Adaptation of buildings to climate change: an overview
- 1SuDesi Lab, Centre for Sustainable Technologies, Indian Institute of Science, Bangalore, Karnataka, India
- 2Department of Architecture and Planning, College of Engineering Trivandrum, Thiruvananthapuram, Kerala, India
Buildings worldwide have evolved based on local resources and skills, evolving form and orientation to ensure a productive and comfortable indoor environment. Traditional habitations rely on passive climate-responsive mechanisms and physiological resilience. At the same time, contemporary buildings rely increasingly on active mechanisms for fine-tuned convenience and comfort. Those buildings are becoming less habitable due to climate change. This paper presents an overview of research into climate-responsive building adaptation, identifying various factors determining a building’s ability to regulate external climatic conditions in providing a habitable indoor environment. The review covers the ability of occupants to manage their thermal environment and adaptation mechanisms, including various adaptation strategies attributed to climate change. Besides a review of relevant research tools and methodologies, the paper also identifies future research challenges. Those challenges include but are not limited to evaluating climate classification provided by building standards given climate change, the need for region-specific climate-change vulnerability assessment of the built environment to develop specific adaptation strategies, a survey of vernacular structures to understand their inherent adaptation capacities, developing a framework to study building adaptation, etc. Thus, this review opens the possibility of further research in building adaptation.
1 Introduction
Climate change is identified through prolonged variations in weather parameters. Its effect, magnitude and timing are uncertain (Sands, 1992; Cissé et al., 2022). With the growing population and increasing future demand for resources, it is critical to deal with the uncertain climate. Otherwise, there could be large-scale losses, including wetlands and forests (U.S. Congress, 1993), human settlements, and the economy itself (Shaw et al., 2023). Human mortality due to heat and cardiovascular/chronic respiratory diseases are increasing, too (P. K. Singh and Dhiman, 2012; Huang et al., 2011; Alessandrini et al., 2019). Preparations are going on around the globe for a warmer future (Roaf et al., 2009; Gosling et al., 2017). As recognized by the Paris Agreement (United Nations Framework Convention on Climate Change, 2015), there is a significant need for adaptation to strengthen resilience and reduce vulnerability to climate change.
Adaptation of a system is its inherent capability to adjust and respond to changes and the effects caused by those changes. It involves learning and flexibility and improving the capacity to deal with the impacts of change. As the adaptive capacity of a system increases, its resilience to face climate stress will also increase, whereas the vulnerability is reduced by moderating sensitivity and exposure. It is done by enabling interactions among the components within a system (Engle, 2011). Functionally, developing survival features allows a system to deal with environmental changes. But in buildings with increasing convenience and finely regulated indoor thermal environments, the adaptive capacity (both physiological and psychological) is restrained as compared to native habitations that provide fundamental protection from the elements of nature (such as rain, biting cold, and intense Sun), predominantly relying on physiological resilience and psychological determination to achieve thermal comfort. IPCC (Intergovernmental Panel on Climate Change) (Denton et al., 2015) defined adaptation as an “adjustment in natural or human systems in response to actual or expected climatic stimuli or their effects, which moderates harm or exploits beneficial opportunities.” IPCC has later updated the definition by splitting it between the natural and human systems (Möller et al., 2023). A few literatures discuss that the adaptation and adaptive capacity concepts are derived from organizational theory and sociology, as against physiological vulnerability and resilience studies. It is a property that affects social and ecological systems (Engle, 2011). It is influenced by the factors mentioned in Figure 1. However, as a subject of focused discussion and research, it became noticeable after the IPCC reports (IPCC, 2014). Currently, it is closely linked with the study of all significant development areas, including but not limited to infrastructure, governance, health, and agriculture. Therefore, there is an imminent need for integrating adaptation measures to ongoing and future projects in buildings as well (Halsnæs and Trærup, 2009), as there is energy saving from 35% of the total global energy consumption as well, caused by commercial, public and residential buildings (International Energy Agency, 2007). This situation demands a need to understand the related research. The current study aims to review the literature that deals with the adaptation of buildings to climate change and critically identify the knowledge gaps and research challenges. This research identified the pertinent literature dealing with the subject to achieve the aim and reviewed it to clarify three significant questions. 1) Why is building adaptation to climate change required? 2) What is the relevance of adaptation in the built environment? and 3) How is adaptation of buildings carried out?
2 Methodology
The overview includes the topics of adaptation and climate change, adaptation mechanisms, thermal adaptation, sustainable building adaptation, and climate-responsive adaptation strategies for the built environment. The following sections are used to showcase each of these topics. The first section covers the definition of the term “adaptation” and its relationship with the built environment in the context of climate change. Adaptation mechanisms are mentioned primarily in social sciences literature and IPCC reports. Categorizing various strategies into different mechanisms will aid in choosing appropriate measures concerning climate change. The current study attempts to translate the available mechanisms into the built environment. This paper tries to identify possible mechanisms at both the building and occupant levels, which is novel to the building adaptation research. An extensive survey of the various tools and methods used to undertake similar studies has been explored in the section, followed by adaptation mechanisms. As risks due to overheating are of huge concern within the buildings compared to other climate change implications, thermal adaptation is given due importance. When the possibilities of utilizing thermal adaptation to combat climate change have been reviewed, an emerging area of sustainable building adaptation is just touched upon to understand the prospects. Given the depth of the implications, discussing and reviewing suitable strategies is necessary. Subsequently, a critical appraisal of the literature identifying the research gaps and future challenges, has been presented.
Building adaptation and energy are closely connected. However, due to the extent of available literature, and as this study aims at the works done primarily on passive adaptation mechanisms and strategies for climate change, this review excludes the research discussing climate change implications focusing on energy use, energy demand, and energy systems. The adaptive reuse of existing buildings is not part of this study for two reasons. Firstly, it is considered more a mitigation measure than adaptation (Aigwi et al., 2023). Secondly, hardly any literature deals with the adaptive reuse of existing buildings for climate change. The structure of the paper is shown in Figure 2. All the referred articles are sourced through Google Scholar using the search string “climate change building adaptation”. Other keywords used for searching articles in the related realm are adaptation mechanisms, adaptation strategies, climate change, climate-responsiveness, thermal adaptation, sustainable building adaptation, and vulnerability assessment. After a preliminary review, the collected articles are sorted based on relevance towards the current study. Only those found suitable to address the research objective are cited in this research. The selected articles were published between 1973 and 2023. The list of references was analyzed using the pivot tables available in MS Excel to arrive at Figure 3 and Figure 4. Figure 3 shows the number of references on the x-axis with its year of publication along the y-axis, and Figure 4 shows the number of references used per publication. The names of the referred publications can also be found in Figure 4.
3 Adaptation of buildings to climate change
The adaptive comfort model or adaptation theory of thermal comfort in buildings emerged after the oil crisis of the 1970s. It has gained importance due to the increased concerns over environmental impact. The word “Adaptation” originates from two Latin words, ad (to) and aptare (fit), and is defined as any alteration to a building, more than maintenance, to alter its capacity or function (Douglas, 2006). It can be an intervention to reuse or uplift a building for new requirements. The other terms that discuss building adaptation include adaptive capacity, adaptive opportunity, adaptive architecture, design for adaptability, and climate adaptive/adaptable/adapted buildings. However, most of these terms do not directly reference the requirement of adapting to existing or future climates (Dave et al., 2012). Such literature is thus excluded. Here, “climate” in the term climate-adaptable buildings refers to being climate-responsive. The words that closely deal with building adaptation for current or future climate include adaptive capacity, adaptive opportunity, and climate-adaptable buildings (Humphreys and Nicol, 2002). In the present study, climate-responsive adaptation in buildings is “the spontaneous (natural) and reactive (passive or active) ability of a building and its inhabitants to sustain a habitable and productive environment in response to uncertain variability in climate.” Addressing it requires addressing each of the associated attributes. Figure 5 shows the adaptation map traced to a cluster of buildings, with the fundamental unit being a building. The four entities that determine the adaptive capacity of a building are its physical characteristics, functional performance, occupant, and the natural environment. Each entity has a bearing on the performance of the other. For instance, thermal comfort would be in demand for an occupant familiarized with an air-conditioned environment, directly impacting the local environment due to increased thermal discharge and rising temperatures (Aysha and Monto, 2017). A similar scenario can be linked to the occupants of mid-latitude cities, where the internal environment cannot provide enough comfort due to the warming outside. Hence, changes in the outside environment also should be dealt with seriously as they affect the indoors. Climate classifications provided by building standards should be reviewed in this context. In 2003, Europe saw the highest morbidity and mortality, especially of the vulnerable group, due to the unusually high temperature and reduced diurnal temperature swing. Hence, estimating the climate change amplification coefficients for buildings is essential to designing better resilient ones (Coley and Kershaw, 2010). de Wilde and Coley, 2012 have clearly outlined the key processes and ways in which the adverse effects of climate change affect both occupants and buildings. A critical review of heatwave impacts on the natural environment and human beings and its measures also unveils the need for building adaptation, especially for dwellings, as most studies focus on commercial buildings (Zuo et al., 2015). In this context, adaptation can be considered a necessary functional requirement of any building.
4 Adaptation mechanisms
Adaptation operates in many ways in different domains. In this section, an attempt has been made to translate those to the built environment (Table 1). Mainly, adaptation occurs through autonomous or planned adaptation (Gosling et al., 2017). The inherent ability to adjust to the environment physiologically is called reactive or autonomous adaptation, which is dominant in climate change. It can be physiological acclimatization or behavioral adaptations such as changes in clothing, etc. In IPCC, autonomous or spontaneous adaptation means responding to the experienced climate and its effects without foresight. With the anticipated changes in climate, proactive measures can be taken to alleviate the impacts. Then, it becomes anticipatory or planned adaptation. It includes public awareness programs, health services, the introduction of subsidies to increase HVAC (Heating, ventilation, and air conditioning) installations, etc. Incremental adaptation is about preserving a system’s integrity, whereas changing a system’s fundamental characteristics based on climatic effects is called transformational adaptation (AR5 2014).
There are also hard and soft adaptations (Coley et al., 2012). Hard adaptations are those with modifications in the structure of a building, whereas soft adaptations are behavioral strategies like opening windows earlier in the day. The Former are likely to add cost to adaptation strategies, but the latter typically do not. When it comes to adaptation responses (U.S. Congress, 1993), they can be passive, deliberate, reactive, or anticipatory. The biological changes in plants or the gradual changes in human tastes and behavior belong to passive responses, and management actions taken after climate change effects are deliberate reactive responses, and planning or regulatory measures taken for climate change preparations are anticipatory responses. Other adaptation and mitigation mechanisms include structural/institutional, technological, and cultural/behavioral (Zuo et al., 2015). If health warnings and enforcing building codes fall under institutional or structural mechanisms, reports and literature with adaptation strategies come under technological mechanisms. Awareness of the occupants and other forms of thermal adaptation constitutes cultural/behavioral mechanisms. Though commercial, technological, and financial adaptation are mentioned in a study as options for house builders in the UK, clarity needs to be improved due to the uncertainty of information available then (Hertin et al., 2003). As discussed in Figure 5, there are three levels to deal with when it comes to adaptation in the built environment. They are cluster, building, and occupant levels. The various adaptation mechanisms prevailing in the literature corresponding to the three levels are identified and listed in Table 1. Different adaptation strategies (see Table 3) can also be found in the literature under one of these mechanisms discussed here.
5 Tools and techniques for building adaptation research
When the climate zone of the building location plays a significant role in undertaking any adaptation, it is inevitable to make it climate-responsive so that the inhabitants feel thermally comfortable indoors. In the literature on building adaptation, only a few have developed a proper methodology to study building adaptation given climate change. However, Figure 6 illustrates the typical process followed in building adaptation literature. Salient studies in this pattern are discussed here. While most literature adopted modeling and simulations, quite a few studies approached detailed questionnaire surveys, physical measurements, and investigation of occupant behavior to study building adaptation. There was a variety in terms of the tools used in the literature. Instead of listing everything in this section, the information has been consolidated and systematically presented in Table 2. As most studies dealing with occupant comfort have used one of the existing thermal comfort models, such as Fanger’s model, Humphrey’s model, or the Adaptive comfort model, it is not included in the table. The time for studies to measure the building’s physical parameters and the occupant behavior survey varied from 2 weeks to 2 years. Another observation was the common use of the “morphing” technique to combine current climate data into future hourly weather data with climate change projections. Climate scenarios for 50 years and 100 years were generated to study the future housing system of New Zealand, given climate change (Jonas et al., 2007). They conducted a social impact study to understand the critical climate changes, potential social impacts, and housing conditions. Thermal simulation and energy performance modeling were done, followed by cost-benefit analysis. When two buildings, a school and a large house, were studied by Coley et al. (2012) for applying hard and soft adaptation strategies, Gupta and Gregg (2012) evaluated the influence of climate change on thermal comfort in suburban English homes. Unlike other studies focusing more on the occupants, the latter research has tried to assess the climate change risks associated with dwellings and the occupants, using projected climate change data for the UK. Considering the potential changes in the houses’ physical characteristics and occupancy, Barbosa et al. (2015) have developed a methodology for evaluating thermal comfort vulnerability in Portugal residences utilizing morphing methods and building performance simulations. An apartment in Taiwan was assessed using building simulation to evaluate thermal performance based on climate change (K. T. Huang and Hwang (2016), Lomas and Giridharan (2012) also conducted a study using real-time measurements and modeling to perceive the appropriateness of current thermal comfort standards in assessing climate change adaptation. But unlike other building or thermal adaptation studies, where the selected buildings are primarily residential dwellings or apartments and office buildings, the authors have studied a hospital building, which brings in the diversity of the building typology. More similar research is required to cover the broad typology of buildings, including industrial ones. Also, too many studies rely only on simulation-based evaluation, which may not include all the facts about the real scenarios. Hence, standardized model calibration should be ensured before approving such studies. The authenticity of the tools/software is questioned if a regulatory body does not support it. More experiment-based research should be encouraged in building adaptation to ascertain the effectiveness of studied strategies.
6 Thermal adaptation
Adaptation of buildings also implies achieving thermal comfort by modifying the environment or behavior based on the expectations and thermal preferences of the occupant, which is otherwise mentioned as thermal adaptation. Adaptive thermal comfort models support the variable indoor temperature standards dealing with the adaptive capacity of occupants. These models help to enhance occupant comfort, reduce energy use, and encourage climate-responsive design of buildings. At the same time, static models deal with fixed indoor temperature standards and support mechanical heating or cooling indoors. The differences in both models formed the context for “thermal adaptation,” which is a combined effect of behavioral, physiological, and psychological processes. Psychological or habituation involves a changed perception of sensory information. Behavioral or technological changes to heat balance include personal adjustment to clothing, activity, postures, food, moving to different locations, modifying surroundings to open or close windows, using HVAC controls, and cultural adjustments such as activity scheduling, dress codes, or siestas. The duration of exposure and delay in response to the thermal conditions determine each of the above adaptation variants (Brager and De Dear, 1998; Dear et al., 1998). Thermal adaptation studies have been ongoing for three decades. With the onset of climate change, a shift can be observed from the studies looking only at the “present outdoor or indoor climatic conditions” to “future scenarios.” Kwok and Rajkovich, 2010 are not limiting the survey to adaptation but claim to include mitigation measures in the building codes and standards. However, the strategies suggested are more adaptive. Their idea of a “mesocomfort zone” must be further explored as there is tremendous scope due to microclimate variability even within a designated climate zone.
Unlike other studies where thermal adaptation is being tested from cold to warm conditions, it is interesting to observe this study testing the adaptation of subjects from warm to cold conditions and vice versa (Luo et al., 2016). It was found that thermal adaptation was not easy, and the study subjects took almost 3 years to re-adapt to the cold environment from a neutral-warm condition. However, their previous studies examining comfort expectations have shown that it is easier for the occupants to shift to an improved thermal condition than to adapt to a lower indoor comfort level, which can be attributed to one of the three adaptation components. Some Indian studies have stated that behavioral adaptation is insufficient, with occupants accustomed to higher comfort levels (Indraganti, 2010; Shastry et al., 2016). While determining the extent of occupant thermal adaptation in free-running or naturally ventilated buildings in Changsha, China, based on physiological and psychological adaptation modes, it is found that it takes 4.25 days for the occupants to adjust to a variation in outdoor temperature and 13 days only if physical mode was considered, implying the importance of psychological adaptation (Liu et al., 2014; Liu et al., 2017 compared the three components of adaptation, behavioral, psychological, and physiological, between natives and migrants of China. It was examined during migration in cold, hot-summer, and warm-winter regions. The study explored the occurrence of the three kinds of adaptation during a short summer trip. Two different locations of migration and time duration of study were fixed, followed by the selection of subjects, three male and four female migrants. Physical measurements include indoor air and globe temperatures, relative humidity (RH), and air velocity, complying with ASHRAE 55. An overall assessment of the thermal environment (TPPA-thermal physiological and psychological assessment) was conducted. Objective evaluation included the subjects’ diet, activity, and behavioral patterns in a day. The subjective assessment had thermal sensation, thermal preference, humid sensation, and humid preference votes. The results showed that the subjects quickly adjusted to the high humidity condition. The integrated neutral temperature of the subjects in the first location was 26.17°C and RH 53.23%. The difference between neutral temperature and relative humidity reduced to 13.05°C and 30.56% between the migrants and the natives after the travel. If the decrease of strain in terms of physiological, behavioral, and psychological is considered a symbol of thermal adaptation, migrants were in the process of it during the travel. However, it decayed when they left the location. Thermal adaptation studies on two groups of people in Japan’s office buildings showed that occupants’ comfort temperature varied considerably between centralized HVAC and free-running buildings. The opportunities for occupants to control their thermal ambiance, such as openable windows, personal fans, and HVAC controls, were discussed as the reasons for the variation, as it would have psychologically or physiologically affected them. However, the differences in the thermal perception of the occupants were found to be insignificant as they were allowed to control their indoor environment at different temperature levels (Goto et al., 2007).
A combination of energy-saving measures and thermal adaptation in buildings have been studied in cities in three different climate zones of India (Dhaka et al., 2012). An institute hostel building was selected, and surface and indoor air temperatures were measured for the study. While analyzing thermal adaptation in composite climate (maximum and minimum temperatures vary from 4°C to 43°C), they observed that the neutral temperature, Tn, varies significantly throughout the months than the constant thermostat of 24°C set for the air conditioner (Tn is the temperature experienced by the occupant over 1 month, either indoor or outdoor, where less variation was stated on subjective scales on mean air temperature or globe temperature (Ti). Tn = 2.56 + 0.83 Ti with r = +0.96) (Humphreys, 1975). This is the temperature at which an occupant feels thermally comfortable (Dhaka, Mathur, and Garg, 2012)). In hot-dry climates, there is a considerable difference between the constant thermostat and neutral temperature because of the extreme summer and winter. However, the difference was not significant for warm-humid climates as there is not much variation in the outdoor temperatures throughout the year. Dhaka and Mathur, 2017, conducted an exclusive study of air-conditioned buildings belonging to the Indian composite climate. Contradictory to the discussions above, a wide range of comfortable temperatures have been observed. It shows that the occupants were satisfied at a higher room temperature than the temperature mentioned in thermal comfort reference studies. Though studies such as Parkinson et al. (2020), López-Pérez et al. (2019), Manu et al. (2016), confirm this inference and show the possibility of better adaptation to a warmer climate, de Dear et al. (2020) seems skeptical about it as there is an evident shift of comfort zone over time towards a narrower range. In a study held in hybrid buildings of Indian hot-dry climate (Anoop Honnekeri et al., 2014), where there are definite zones of air-conditioned as well as naturally ventilated spaces, the indoor temperatures in naturally ventilated zones were always lesser by two to five degree Celsius than the outdoor temperature even if the latter was above 35°C. Irrespective of the variations in the outdoor temperatures, the occupants were found to be comfortable by managing specific adaptive actions such as controlling the openings and usage of fans. This ascertains better thermal performance of the building envelope and higher adaptive capacity of occupants staying inside a naturally ventilated space of hot-dry climate, irrespective of their intermittent visits to the air-conditioned zone. In other words, the occupants are less vulnerable to the outdoor conditions in free-running spaces than in air-conditioned rooms, where the comfortable temperature range is between 23°C and 29°C. Even ISO 7730 mentioned adaptation, wherein people in warm climates can accept hot environments compared to colder climates (Standard, 2005). This is also conforming to the study done by Shastry et al. (2016), Henna, Saifudeen, and Mani (2019), Saifudeen and Mani (2021) where the occupants exhibit a more comprehensive comfortable temperature range in free-running buildings against the adaptation study mentioned in Fanger (1973). An exciting study held during the COVID-19 pandemic period in Italian and French classrooms in the same climate zone observed that the neutral temperature of French students was 3.1°C less than their Italian counterparts. Also, French students showed lesser adaptability during winter, though they are more familiar with colder conditions than Italians (Lamberti et al., 2023). This clearly shows the influence of microclimate and further demands the need for revision of climate zones. The importance of thermal adaptation is emphasized through the literature discussed in this section, especially from the occupant’s point of view, though not every work has included climate change effects. Therefore, this section is not extensive enough to fit all the thermal adaptation studies. But most strategies discussed here are helpful while undertaking climate change adaptation of buildings. However, there is a considerable lack of implementing thermal adaptation strategies in buildings, even though thorough studies have been conducted on such methods of achieving thermal comfort (Hellwig et al., 2019).
7 Sustainable building adaptation
Though few, some studies mention the importance of sustainable building adaptation (Wilkinson et al., 2014), looking at occupants and buildings. By sustainable building adaptation, Wilkinson et al. meant adapting the existing building stock with a reduced environmental footprint and efficient resource usage. A similar attempt was made to understand the issues of low-cost public housing in Malaysia to provide solutions from the perspective of sustainable adaptation (Hashim et al., 2015). An Attribute-Action Matrix was used to assess the public housing in Singapore, and found that retaining buildings with minor maintenance could be a sustainable strategy for adaptation (Teo and Lin, 2011). The impact of the internal environment of the office building on the productivity of workers was studied to highlight the importance of sustainable adaptation measures (Kershaw and Lash 2013; Singh et al., 2008 have attempted to relate bioclimatism (Bioclimatism is an integration of micro-climate and architecture to achieve thermal comfort), socio-cultural setup, local availability of building material, thermal comfort and adaptation of these features in the vernacular buildings, while trying to study sustainability through bioclimatic design at the northeast of India. According to the study, “i) maximize the use of renewable and natural resources in the building environment, ii) climate-responsive building design, iii) minimizing the artificial energy and water use and iv) good building system functions and proper operation and maintenance” will form the basic principles of sustainable design of buildings. Another literature (Sengupta, 2008) presents cost-effective and sustainable construction technologies across India. The techniques include using compressed earth blocks, masonry arches, rat-trap bond walls, and filler slabs. As with many similar methods, these techniques can act as sustainable adaptation strategies but need testing. Given the context of climate change, research should be done to study the effects of sustainable building adaptation to encompass social, economic, and environmental dimensions.
8 Climate-responsive building adaptation strategies
This section lists the adaptation strategies from the literature that could be applied either for a new building or as a retrofitting option for an existing building to deal with climate change. Adaptation and mitigation are the two possible ways to deal with climate change. It can be at a macro scale, such as country-level measures, or a micro scale, such as city/town level or building level. According to IPCC (Denton et al., 2015), mitigation means cutting greenhouse gas sources and descending them through human intervention. Therefore, mitigation measures can be considered actions that help reduce greenhouse gas sources due to buildings. Both steps are vital to deal with the adverse effects of climate change, as it is already underway and imminent. However, rigorous mitigation measures cannot lessen the impact of further climate change. Hence, adaptation strategies are needed to reduce vulnerability, as a climate event always follows mitigation, whereas the former can be planned. Moreover, mitigation can neither be used to push back climate change nor to prepare for it. Still, connecting both measures will enhance the region’s socioeconomic development (Ayers and Saleemul, 2009). Quite a few studies discuss the various adaptation strategies possible in buildings, though hardly a few have been empirically tested and evaluated. For example, Kwok and Rajkovich, 2010 have developed strategies to improve the adaptive capacity of passive and low-energy buildings. Specific strategies were mentioned for each approach to the design and construction of the buildings, including site analysis, form development, daylighting, and passive heating or cooling. Likewise, it is observed that the considerations and challenges for low-energy or green buildings concerning climate change are similar to those of conventional buildings (Kwok and Rajkovich, 2010). But green building practices, including LEED (Leadership in Energy and Environmental Design) certification, offer better adaptation opportunities for climate change (Pyke et al., 2012; Wilson and Ward, 2009 put forth a list of adaptation strategies for US buildings, as given below. Among the different weather parameters, they focus on temperature, as an increase in temperature is critical to climate change.
• Designing building envelopes to reduce the cooling load
• Design buildings for natural ventilation to control humidity
• Control internal gains using efficient lighting and appliances
• Modify simulation models as per warmer climate projections
• Include more landscaping to lessen cooling requirements
• Control urban heat island effect through design and landscaping
Farzan et al. (2017) indicated that by 2090, occupant thermal comfort would be adversely affected if the summer and winter of Germany get warmer and milder, respectively. They have studied a single-family detached house representing a significant part of the German building stock. The authors have assessed three passive adaptation measures: solar protection glazing, natural ventilation, and shading, and found that a combination of these helps reduce uncomfortable overheating hours. When a well-insulated envelope, lesser skylight area, and standard roof with high solar absorption are the optimal strategies proposed for the northern regions of France, non-insulated ground slab, reflective, cool roof, and more skylight areas to increase night natural cooling potential and lighting are recommended for the southern regions (Lapisa et al., 2018). Strategies that could be switched between summer and winter are required to maintain comfort throughout the year, as per the study in the south of France (Machard et al., 2023). If ventilation and solar control are helpful in summer, adjusting the roof optics and percentage of glazing will help in winter. A noticeable study by Porritt (2012) on the different dwelling typologies of the UK found that passive interventions are insufficient to treat Tier 2 dwellings against over-heating compared to Tier 1 houses. In Tier 1 and Tier 2, the outside wall insulation steadily outperformed any internal insulation of walls. The study has also developed a retrofit advice tool kit as part of the study showcasing the various adaptation options. Shaw et al. (2007) give different adaptation options for UK buildings to manage high temperatures, flood risks, water resources and quality, and ground conditions. This comprehensive guide provides solutions at urban, neighborhood, and building scales. Porritt et al. (2011) categorized the interventions into three areas: insulation, solar control, and ventilation while testing the adaptation of Victorian terraced dwellings in the UK for heatwaves. The trials included loft, external and internal wall insulation based on the existing thermal mass, exterior shutters or internal blinds for windows, painting the roofs and walls with a lighter color, and ventilation modifications corresponding to the outside weather. The effective interventions were external wall insulation and lighter color painting of the exterior walls. While most studies recommend that thermal mass modifications can bring better thermal comfort inside, Seyed Masoud and Sharples (2017) suggest that it does not alone provide comfort. Instead, the study agrees with other literature that put forth ventilation strategies and solar control as effective approaches to improve space thermal comfort in UK buildings (Lomas and Ji, 2009; Short et al., 2012). A similar study from the Netherlands that assessed the changes in thermal resistance, thermal capacity, and albedo values along with vegetation roof, solar shading, and additional natural ventilation also found the most effective techniques being shading the building exteriors and improving natural ventilation (Hooff et al., 2015). Another study (Coley, Kershaw, and Eames, 2012) in the UK on a school building and residence tested hard and soft adaptation mechanisms, such as window shading, controlled glazing, controlled window openings, and night ventilation, in both current and future climate. Here, soft (behavioral) adaptation is found to be as least effective as the hard one in both buildings. Gupta and Gregg (2012) suggested a few passive measures for cooling suburban English home interiors, including microclimate management and ventilation design. The authors have also proposed other adaptation techniques, such as wall and roof retrofit. For example, they replaced window glazing with low e-glazing and the roof with a high albedo roof. Of the tested adaptation options, minimizing direct solar radiation’s influence was the most effective. A Passivhaus residence in the UK was evaluated for overheating and found that a 95–110° inclined south façade with glazing can reduce the risk of indoor overheating (Lavafpour and Steve Sharples, 2014).
A low-carbon dioxide adaptable home (LCAH), as the authors call it, which is a near-zero energy house in Ireland, with multiple building technologies (rain-screen cladding, lightweight envelope features, airtight wrapping, prefabricated steel frames), has been tested using various passive solar and daylighting options along with post-occupancy evaluation, to find the reduced overheating hours (Sanders and Phillipson, 2003; Kinnane et al., 2017) suggested enforcing strategies using building standards and codes to develop guidelines and enhance awareness of climate change, periodic maintenance of houses, etc., for the UK building stock, which would have been taken care of by now. Contrary to the existing literature, which mainly discussed the occupants’ adaptability concerning buildings, Kvande and Lisø, 2009 deal with adapting masonry structures in extreme climates. They have comprehensively analyzed the defects of masonry structures in Norway and proposed geographically dependent guidelines for masonry design. They are suggesting the strengthening of policies in every aspect to ensure climate adaptation at the local level. The proposed guidelines include requirements for performance-based procedures, frost resistance needs, masonry mortar compatibility, window positioning approaches, high-performance weather-protective flashings, and rendering recommendations.
A few studies discuss the adaptation of buildings to climate change in Australia. They recognize the significant impacts being health effects from overheating, increased energy consumption, increased damage due to tropical cyclones, damage of foundations and pipe work due to ground movement, flooding and saltwater inundation damages, and increased bushfire risk (Bengtsson et al., 2007). Some of the effective strategies proposed for typical Australian houses include reducing the space volume for air conditioning by partitions, modifying occupant behavior, changes in clothing style, ceiling and roof insulation, gap sealing, and do-it-yourself (DIY) retrofitting of houses based on the type of construction (John James et al., 2017). According to the authors, deeper retrofit is desirable to adapt to the high indoor temperatures apart from the occupant behavioral changes. A study held in ten Australian cities (different climate zones) to investigate the potential of natural ventilation has found varying results during the present and future climates, which ascertains the need to assess the climate before any interventions (Bamdad et al., 2022).
Clothing is a behavioral adaptation technique that can be followed for adapting to climate variations depending upon individual choice. However, it will become difficult if there is a dress code to be followed. A study from Nigeria surveyed 450 participants during dry and rainy seasons to understand how much clothing can affect thermal perception. They found that clothing, relative humidity, and metabolic rate have correlation coefficients of 0.516, 0.115, and 0.020, respectively. This study concludes that clothing helps an occupant to better adapt to the thermal environment of a building during a hotter climate (Meshack, 2017). This helps in less reliance on other psychological and physiological adaptations. Another study in southwest Nigeria on contemporary low-income housing infers that climate change resilience is achievable and thermal comfort can be improved using modifications to the building envelope. They have modeled the house using two envelope options each for floor, roof, and wall. They are timber and concrete floors, pitched and flat roofs using hardwood, hollow heavy concrete, and adobe walls. In contradiction to the conventional studies where adobe and wood are mostly suggested building materials for the humid tropics, this study found that the indoors were more comfortable with hollow heavy concrete walls, concrete floors, and hardwood pitched roofs (Stephanie and Sharples Steve 2017; Hong et al., 2013 have recommended a double skin facade for East Asian monsoon climates where hot summers and cold winters are prevalent. Strategically operating the airflow through the double skin façade as per change in weather can bring in adequate comfort.
Cool roofs (reflective roofs with a high albedo and thermal emittance) seem to have more significant potential in terms of climate change adaptation along with energy efficiency, mitigating heat island effects, improving air quality and reducing heat wave impacts (Bureau of Energy Efficiency, 2011; Garg et al., 2016a; Garg et al., 2016b; Afshari, 2023; Bamdad, 2023). These roofs can be a better strategy in dealing with hotter environments, but further studies are required to understand their performance during winters when heating is the priority.
Mishra and Ramgopal (2013) extensively reviewed human thermal comfort studies. They have observed that people can adapt to their surroundings with enough adaptation opportunities. But compared to free running or naturally ventilated buildings, the comfort zone is narrowed down in air-conditioned buildings. They also observed that air movement and clothing modification are the two primary means of adaptation. Aysha and Monto (2017) have presented the possible adaptation techniques in terms of built environment, built form or structure, building material, fenestrations, site and surroundings, and the urban context. It is understood that there are various options to tackle adaptation concerning buildings and their occupants. The only challenge is testing it scientifically, seeing the performance, and proposing further interventions. Saifudeen and Mani (2017) have considered the possible adaptation techniques for India concerning the variation in weather parameters due to climate change. The methods were identified and collated based on occupant, clothing, and building. The vernacular architecture study from the northeast region of India has found numerous solar passive features that deal with temperature control, shading, and ventilation. They are air gaps in the ceiling, movable blinds, chimneys, increased openings, proper orientation, and compact construction (Singh et al., 2008). High thermal mass construction using local materials such as stone and brick is effective for maintaining comfort in the free-running structures of composite climate in India, especially during extreme winter and summer (Kumar et al., 2017). The vernacular architecture of Bushehr City, Iran, was studied, and the climate-responsive solutions were analyzed for the design of buildings in the future. The solutions included the use of low thermal capacity materials for building envelope, especially wood, proper orientation aligning with wind direction, placing of windows towards the courtyard, and usage of rooftops or terraces during night time (Motealleh et al., 2018). The assessment of climate-responsive design using real-time data of the historic dwellings in Cadiz, Spain, reveals that the passive techniques, including high thermal mass envelopes environmental control systems of openings, will be adequate to provide comfort for those dwellings even in the changing climate (Rubio-Bellido et al., 2015). Extensive research on historic buildings could pool in ample passive strategies to adapt existing buildings, as discussed in Claudia et al. (2023). The lessons from vernacular architecture practices are also of due importance to deal with region-specific climatic variability as well as to intervene with proper adaptive measures (Henna, Saifudeen, and Mani, 2017; Philokyprou et al., 2017; Weber and Yannas, 2013; Ramli, 2012). However, care must be observed in understanding the practices as studies show the reduced efficacy of vernacular passive design techniques in a changing climate (Zune, Rodrigues, and Gillott, 2020). The research by Gauthier et al. (2020) gives much hope to deal with the strategies in a person-centric way once their study progresses. All the possible climate-responsive building adaptation strategies discussed in literature applicable to occupants, buildings, and clusters of buildings have been organized in Table 3, corresponding to the various climate change impacts and the type of adaptation mechanisms. This table helps choose the required strategies for a new or existing construction. For example, “region-specific designs” belong to the “passive or planned/anticipatory” type of adaptation mechanism that applies to new construction, whereas “adaptive usage of balconies/doors/windows/curtains/blinds” is for existing buildings and belongs to “passive, autonomous/reactive/spontaneous” mechanism. Figure 7 shows an attempt to trace the locations where cases have been used by the researchers to study building adaptation.
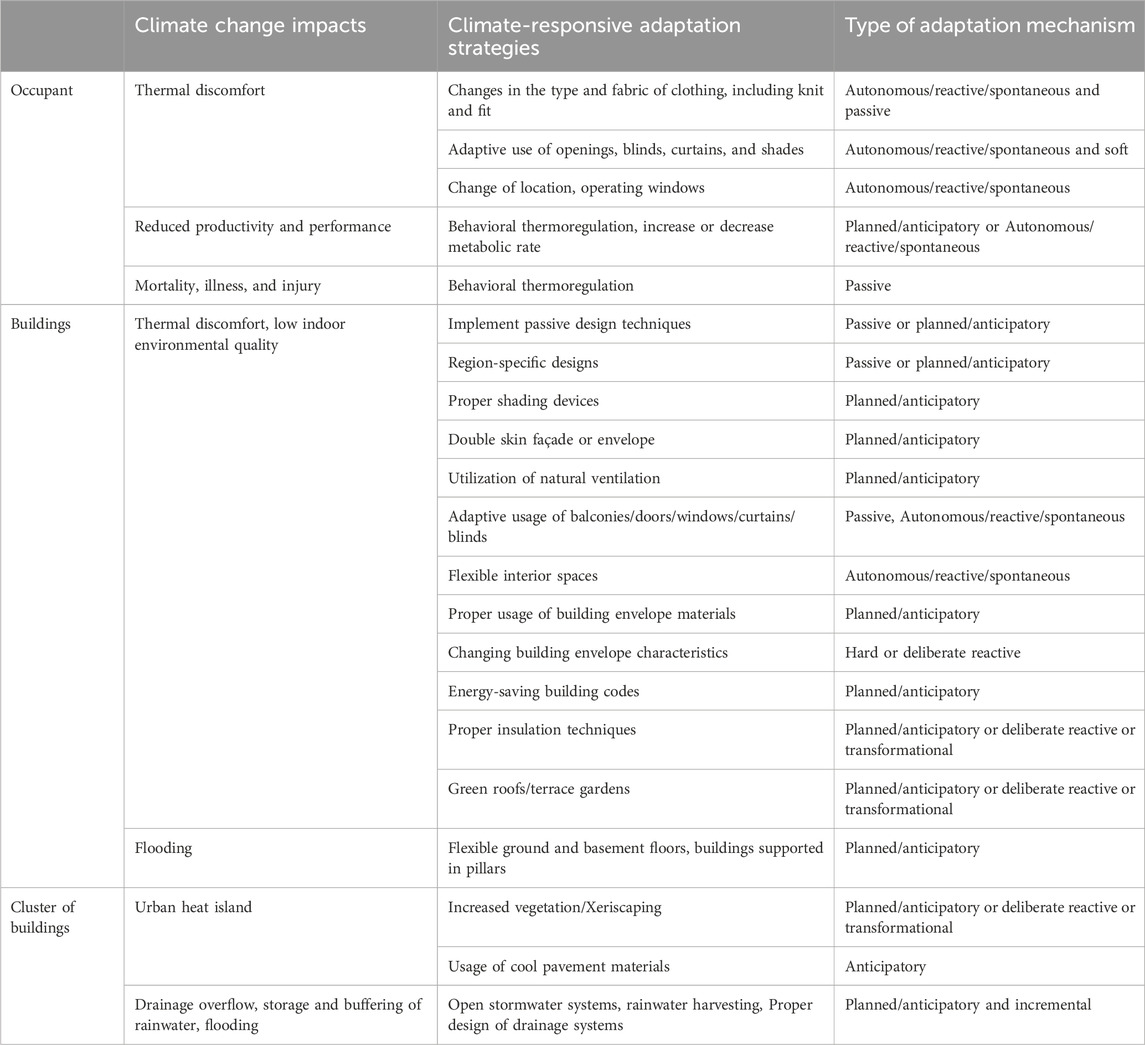
TABLE 3. Climate-responsive building adaptation strategies to deal with specific climate change impacts and their type of adaptation mechanisms to be followed.
Retrofitting is the way forward for existing buildings towards sustainable development, and so is climate change adaptation. However, it is not easy to find out a climate-specific strategy (Daly et al., 2014; Ürge-Vorsatz et al., 2014; Filippín et al., 2018; Elsharkawy and Zahiri, 2020). For sustainable retrofitting, existing bio-climatic and passive features are essential (Bodale et al., 2019). The significant attributes to be considered while retrofitting are the window-to-wall ratio, solar heat gain coefficient of windows, heat transmission coefficient or U-values, and insulation thickness of the building envelopes (Li et al., 2021). These factors are directly linked to energy consumption and thermal comfort. However, care must be observed in adding thermal insulation with increased glazing area to deal with overheating in the future (Filippín et al., 2017). A Machine Learning model, as used by Ma et al. (2023), can be utilized to arrive at retrofitting decisions to avoid redundant measures, and also depending on the sensitivity of the buildings to climate.
9 Critical appraisal
While addressing the potential impacts of climate change, literature, including IPCC, has discussed various systems like forests, species diversity, aquatic ecosystems, coastal resources, coastal wetlands, water resources, agriculture, human health, transportation, and energy. The mention of buildings as part of a system or an independent system is just emerging. For the literature being discussed under the topic of adaptation and mitigation to climate change referred to across the world, it is critical to address the adaptation of the built environment along with the other systems. Revising climate classifications published by building standards could be an initial step towards understanding building adaptation. As understood from various literatures, the physiological resilience of the occupants to temperature variations is higher in the tropical regions as compared to the occupants in the temperate zones, which demands an in-depth survey. Hardly any study has scrutinized the climate change risks or vulnerabilities associated with buildings other than the overall assessment by the IPCC. Consequently, there is a need to assess the region-wise climate change vulnerabilities related to the built environment to develop region-specific measures or microclimate-responsive interventions. Likewise, there is an increasing trend among the rural population to transform their vernacular dwellings to conventional ones by changing the building envelope materials from mud or wood to brick and concrete, thereby losing the thermal comfort achieved inside the climate-responsive vernacular dwellings. This discomfort is expected to increase as an impact of changing climate. More studies focusing on vernacular buildings can be made, as those buildings are known for their inherent climate-responsive adaptation capabilities. A comprehensive outline of the built environment in terms of adaptation needs to be improved in the existing methodologies and hence requires a proper framework. Also, there is enough scope in research to undertake real-time or experiment-based studies to prove whether the mentioned adaptation techniques work. The climate models and building simulation tools fail to accommodate microclimate variability or the region-specific parameters within the module that model calibration must be addressed in studies. Simulation tools can be developed to incorporate region-specific passive solutions for new buildings and passive retrofitting measures for existing buildings to combat climate change. The adaptation guidelines can also be included in building codes and regulations, adding various techniques corresponding to different climates. More climate change impact studies must be conducted to cover a wide range of building typology, configurations, and geographical locations. Further investigation of the concept is necessary to build adaptation research and develop sustainable means of adaptation techniques for climate change. Within the realm of sustainability, social and economic factors also should be given due importance as the environmental factor. Mainly, the buildings in clusters or on the urban scale should only be addressed through sustainable strategies in the future. Given the challenges, the most significant gaps identified by this research include the lack of understanding of climatic variations due to climate change within definite zones, absence of vulnerability research pertinent to the built environment for providing adaptation measures, utilization of more apt tools and techniques including physical experiments, artificial intelligence and machine learning for building adaptation research.
10 Conclusion
The review addresses topics related to adaptation research for the built environment to prepare to fight climate change. It explored whether thermal adaptation can be utilized to better adapt to a changing climate. The behavioral measures to regulate external climatic conditions were identified. Much work is required to understand all the dimensions of sustainable adaptation. The study recommends further exploration of sustainable adaptation strategies such that urban building clusters will benefit. When adaptation mechanisms are understood through the literature on various other systems, this review collated the information to include the built environment. Available cases from across the globe have been discussed to choose suitable adaptation strategies or climate-responsive retrofitting measures specific to new or existing construction. The gaps identified in the review and further scope of research have been dealt with through a critical appraisal.
Extensive research is required in building adaptation to cover the varied geographies and diversity regarding climate, resources, building typology, and lifestyles. Most climate change studies focus on warming. However, it is imperative to address all the extreme climate change events as they have one or other implications for the built environment. And this has to be dealt with using detailed simulations and hands-on experiments to develop appropriate adaptation measures.
Author contributions
AS: Conceptualization, Data curation, Formal Analysis, Investigation, Methodology, Visualization, Writing–original draft, Writing–review and editing. MM: Conceptualization, Supervision, Writing–original draft, Writing–review and editing.
Funding
The author(s) declare that no financial support was received for the research, authorship, and/or publication of this article.
Conflict of interest
The authors declare that the research was conducted in the absence of any commercial or financial relationships that could be construed as a potential conflict of interest.
Publisher’s note
All claims expressed in this article are solely those of the authors and do not necessarily represent those of their affiliated organizations, or those of the publisher, the editors and the reviewers. Any product that may be evaluated in this article, or claim that may be made by its manufacturer, is not guaranteed or endorsed by the publisher.
References
Afshari, A. (2023). Optimization of urban design/retrofit scenarios using a computationally light standalone urban energy/climate model (SUECM) forced by ERA5 data. Energy Build. 287, 112991. doi:10.1016/j.enbuild.2023.112991
Aigwi, I. E., Ahmed, D., and Amarachukwu, N. N. (2023). Adaptive reuse of existing buildings as a sustainable tool for climate change mitigation within the built environment. Sustain. Energy Technol. Assessments 56 (September 2022), 102945. doi:10.1016/j.seta.2022.102945
Alessandrini, J. M., Ribéron, J., and Da Silva, D. (2019). Will naturally ventilated dwellings remain safe during heatwaves? Energy Build. 183, 408–417. doi:10.1016/j.enbuild.2018.10.033
AR5 (2014). “Glossary. Climate change 2013: impacts, adaptation and vulnerability,” in Contribution of working group II to the fifth assessment report of the intergovernmental Panel on climate change, 1757–1776. doi:10.1016/S0959-3780(06)00031-8
Ayers, J. M., and Saleemul, H. (2009). The value of linking mitigation and adaptation: a case study of Bangladesh. Environ. Manag. 43 (5), 753–764. doi:10.1007/s00267-008-9223-2
Aysha, S., and Monto, M. (2017). “Adaptation of buildings to climate change,” in Encyclopedia of sustainable technologies (Elsevier), 331–349. doi:10.1016/B978-0-12-409548-9.10202-7
Bamdad, K. (2023). Cool roofs: a climate change mitigation and adaptation strategy for residential buildings. Build. Environ. 236 (March), 110271. doi:10.1016/j.buildenv.2023.110271
Bamdad, K., Matour, S., Izadyar, N., and Law, T. (2022). Introducing extended natural ventilation index for buildings under the present and future changing climates. Build. Environ. 226 (April), 109688. doi:10.1016/j.buildenv.2022.109688
Barbosa, R., Vicente, R., and Santos, R. (2015). Climate change and thermal comfort in southern Europe housing: a case study from lisbon. Build. Environ. 92, 440–451. doi:10.1016/j.buildenv.2015.05.019
Bengtsson, By J., Clément, V., and Davies, T. (2007). Adaptation to climate change through building regulation. Australia: Edge Environment.
Bodale, A., Catalina, T., and Sima, C. I. (2019). Adaptation of buildings to climate change through bioclimatic strategies, in Romania. E3S Web Conf. 111, 06071. doi:10.1051/e3sconf/201911106071
Brager, G. S., and De Dear, R. J. (1998). Thermal adaptation in the built environment: a literature review. Energy Build. 27 (1), 83–96. doi:10.1016/s0378-7788(97)00053-4
Claudia, E. V., David, B., Arash, B., Bassam, A., and Torres, M. J. (2023). Thermal performance of historic buildings in Mexico: an analysis of passive systems under the influence of climate change. Energy Sustain. Dev. 72 (2022), 100–113. doi:10.1016/j.esd.2022.12.002
Cissé, G., McLeman, R., Adams, H., Aldunce, P., Bowen, K., Campbell-Lendrum, D., et al. (2022). “Health, wellbeing, and the changing structure of communities,” in Climate change 2022: impacts, adaptation and vulnerability. Contribution of working group II to the sixth assessment report of the intergovernmental Panel on climate change. doi:10.1017/9781009325844.009.1042
Coley, D., and Kershaw, T. (2010). Changes in internal temperatures within the built environment as a response to a changing climate. Build. Environ. 45 (1), 89–93. doi:10.1016/j.buildenv.2009.05.009
Coley, D., Kershaw, T., and Eames, M. (2012). A comparison of structural and behavioural adaptations to future proofing buildings against higher temperatures. Build. Environ. 55, 159–166. doi:10.1016/j.buildenv.2011.12.011
Costantine, G., Maalouf, C., Moussa, T., and Polidori, G. (2018). Experimental and numerical investigations of thermal performance of a hemp lime external building insulation. Build. Environ. 131 (December 2017), 140–153. doi:10.1016/j.buildenv.2017.12.037
Daly, D., Cooper, P., and Ma, Z. (2014). Implications of global warming for commercial building retrofitting in Australian cities. Build. Environ. 74, 86–95. doi:10.1016/j.buildenv.2014.01.008
Dave, M., Varshney, A., and Graham, P. (2012). Assessing the climate change adaptability of buildings.
Dear, R., Brager, D. G., and Donna, C. (1998). Developing an adaptive model of thermal comfort and preference. ASHRAE Trans. 104 (1), 1–18.
Dear, R. D., Xiong, J., Kim, J., and Cao, B. (2020). A review of adaptive thermal comfort research since 1998. Energy Build. 214, 109893. doi:10.1016/j.enbuild.2020.109893
Denton, F., Wilbanks, T. J., Abeysinghe, A. C., Burton, I., Gao, Q., Masui, T., et al. (2015). “Climate-resilient pathways: adaptation, mitigation, and sustainable development,” in Climate change 2014 impacts, adaptation, and vulnerability. Editors C. B. Field, V. R. Barros, D. David Jon, K. J. Mach, and M. D. Mastrandrea (Cambridge: Cambridge University Press), 1101–31. doi:10.1017/CBO9781107415379.025
Dhaka, S., and Mathur, J. (2017). Quantification of thermal adaptation in air-conditioned buildings of composite climate, India. Build. Environ. 112, 296–307. doi:10.1016/j.buildenv.2016.11.035
Dhaka, S., Mathur, J., and Garg, V. (2012). Combined effect of energy efficiency measures and thermal adaptation on air conditioned building in warm climatic conditions of India. Energy Build. 55, 351–360. doi:10.1016/j.enbuild.2012.09.038
Elsharkawy, H., and Zahiri, S. (2020). The significance of occupancy profiles in determining post retrofit indoor thermal comfort, overheating risk and building energy performance. Build. Environ. 172 (January), 106676. doi:10.1016/j.buildenv.2020.106676
Engle, N. L. (2011). Adaptive capacity and its assessment. Glob. Environ. Change 21 (2), 647–656. doi:10.1016/j.gloenvcha.2011.01.019
Fanger, P. O. (1973). Assessment of man’s thermal comfort in practice. Br. J. Industrial Med. 30, 313–324. doi:10.1136/oem.30.4.313
Farzan, B., Johannes, M., Julia, B., and Werner, L. (2017). “Effectiveness of passive climate adaptation measures in residential buildings in Germany,” in PLEA Conference Proceedings: Design to Thrive, 18–25.
Filippín, C., Flores Larsen, S., and Ricard, F. (2018). Improvement of energy performance metrics for the retrofit of the built environment. Adaptation to climate change and mitigation of energy poverty. Energy Build. 165 (April), 399–415. doi:10.1016/j.enbuild.2017.12.050
Filippín, C., Ricard, F., Flores Larsen, S., and Santamouris, M. (2017). Retrospective analysis of the energy consumption of single-family dwellings in central Argentina. Retrofitting and adaptation to the climate change. Renew. Energy 101, 1226–1241. doi:10.1016/j.renene.2016.09.064
Garg, V., Kotharkar, R., Sathaye, J., Rallapalli, H., Kulkarni, N., Reddy, N., et al. (2016a). Assessment of the impact of cool roofs in rural buildings in India. Energy Build. 114, 156–163. doi:10.1016/j.enbuild.2015.06.043
Garg, V., Somal, S., Arumugam, R., and Bhatia, A. (2016b). Development for cool roof calculator for India. Energy Build. 114, 136–142. doi:10.1016/j.enbuild.2015.06.022
Gauthier, S., Bourikas, L., Al-Atrash, F., Bae, C., Chun, C., de Dear, R., et al. (2020). The colours of comfort: from thermal sensation to person-centric thermal zones for adaptive building strategies. Energy Build. 216, 109936. doi:10.1016/j.enbuild.2020.109936
Gosling, S. N., Hondula, D. M., Bunker, A., Ibarreta, D., Liu, J., Zhang, X., et al. (2017). Adaptation to climate change: a comparative analysis of modeling methods for heat-related mortality. Environ. Health Perspect. 125 (8), 087008. doi:10.1289/EHP634
Goto, T., Mitamura, T., Yoshino, H., Tamura, A., and Inomata, E. (2007). Long-term field survey on thermal adaptation in office buildings in Japan. Build. Environ. 42 (12), 3944–3954. doi:10.1016/j.buildenv.2006.06.026
Guan, L. (2012). Energy use, indoor temperature and possible adaptation strategies for air-conditioned office buildings in face of global warming. Build. Environ. 55, 8–19. doi:10.1016/j.buildenv.2011.11.013
Gupta, R., and Gregg, M. (2012). Using UK climate change projections to adapt existing English homes for a warming climate. Build. Environ. 55, 20–42. doi:10.1016/j.buildenv.2012.01.014
Halsnæs, K., and Trærup, S. (2009). Development and climate change: a mainstreaming approach for assessing economic, social, and environmental impacts of adaptation measures. Environ. Manag. 43 (5), 765–778. doi:10.1007/s00267-009-9273-0
Hamdy, M., Carlucci, S., Hoes, P. J., Jan, L., and Hensen, M. (2017). The impact of climate change on the overheating risk in dwellings—a Dutch case study. Build. Environ. 122 (August 2003), 307–323. doi:10.1016/j.buildenv.2017.06.031
Hashim, A. E., Aida Samikon, S., Ismail, F., and Ismail, Z. (2015). Managing facilities on Malaysian low-cost public residential for sustainable adaptation. Procedia - Soc. Behav. Sci. 168, 52–60. doi:10.1016/j.sbspro.2014.10.209
Hellwig, R. T., Teli, D., Schweiker, M., Joon Ho Choi, M. C., Mora, R., Rawal, R., et al. (2019). A framework for adopting adaptive thermal comfort principles in design and operation of buildings. Energy Build. 205, 109476. doi:10.1016/j.enbuild.2019.109476
Henna, K., Saifudeen, A., and Mani, M. (2019). “Responsiveness and resilience of existing dwellings in warm-humid climate zone to changing climate,” in Comfort at the extremes 2019 (Dubai: Susan Roaf and Will Finlayson), 758–773.
Hertin, J., Berkhout, F., Gann, D. M., and Barlow, J. (2003). Climate change and the UK house building sector: perceptions, impacts and adaptive capacity. Build. Res. Inf. 31 (3–4), 278–290. doi:10.1080/0961321032000097683
Hong, T., Kim, J., Lee, J., Koo, C., and Park, H. S. (2013). Assessment of seasonal energy efficiency strategies of a double skin façade in a monsoon climate region. Energies 6 (9), 4352–4376. doi:10.3390/en6094352
Honnekeri, A., Brager, G., Dhaka, S., and Mathur, J. (2014). “Comfort and adaptation in mixed-mode buildings in a hot-dry climate,”In Proceedings of 8th Windsor Conference: Counting the Cost of Comfort in a Changing World. Windsor, UK. United Kingdom: Cumberland Lodge. Available at: http://www.cbe.berkeley.edu/research/briefs-survey.htm.
Hooff, T. V., Blocken, B., Hensen, J. L. M., and Timmermans, H. J. P. (2015). Reprint of: on the predicted effectiveness of climate adaptation measures for residential buildings. Build. Environ. 83 (January), 142–158. doi:10.1016/j.buildenv.2014.10.006
Huang, C., Vaneckova, P., Wang, X., FitzGerald, G., Guo, Y., and Tong, S. (2011). Constraints and barriers to public health adaptation to climate change. Am. J. Prev. Med. 40 (2), 183–190. doi:10.1016/j.amepre.2010.10.025
Huang, K. T., and Hwang, R. L. (2016). Future trends of residential building cooling energy and passive adaptation measures to counteract climate change: the case of taiwan. Appl. Energy 184, 1230–1240. doi:10.1016/j.apenergy.2015.11.008
Humphreys, M. A. (1975). “Field studies of thermal comfort compared and applied,” in Building research establishment (UK: Garston, Watford: Building Research Station).
Humphreys, M. A., and Nicol, J. F. (2002). Adaptive thermal comfort and sustainable thermal standards for buildings. Energy Build. 34 (6), 563–572. doi:10.1016/S0378-7788(02)00006-3
Indraganti, M. (2010). Behavioural adaptation and the use of environmental controls in summer for thermal comfort in apartments in India. Energy Build. 42 (7), 1019–1025. doi:10.1016/j.enbuild.2010.01.014
IPCC (2014). “IPCC, 2013: climate change 2013: the physical science basis,” in Contribution of working group I to the fifth assessment report of the intergovernmental Panel on climate change. Editors Bex V., Midgley P. M., Stocker T. F., Qin D., Plattner G.-K., Tignor M.et al. (Cambridge: Cambridge University Press). doi:10.1017/CBO9781107415324
I-Ting, L., Kuo-Tsang, H., and Hwang, R.-L. (2017). “Uncertainty and sensitivity analysis of residential cooling energy against future climate in hot-humid taiwan,” in PLEA Conference Proceedings: Design to Thrive, 97–104.
John James, S., Behdad, M., Richard, A., Adrian, P., and Clarke John, M. (2017). “Adapting to a warmer climate – affordable low-carbon retrofits and occupant options for typical Australian houses,” in PLEA Conference Proceedings: Design to Thrive, 145–152.
Jonas, B., Bennett, J., Stephen, M., Brett, M., and Page, I. (2007). “Climate change impacts in New Zealand: a cross-disciplincary assessment of the need to adapt buildings, with focus on housing,” in World Conference SB08, 252–263.
Jones, R. V., Goodhew, S., and De Wilde, P. (2016). Measured indoor temperatures, thermal comfort and overheating risk: post-occupancy evaluation of low energy houses in the UK. Energy Procedia 88, 714–720. Elsevier B.V. doi:10.1016/j.egypro.2016.06.049
Karimpour, M., Belusko, M., Xing, K., Boland, J., and Bruno, F. (2015). Impact of climate change on the design of energy efficient residential building envelopes. Energy Build. 87, 142–154. doi:10.1016/j.enbuild.2014.10.064
Kershaw, T., and Lash, D. (2013). Investigating the productivity of office workers to quantify the effectiveness of climate change adaptation measures. Build. Environ. 69, 35–43. doi:10.1016/j.buildenv.2013.07.010
Khadeeja, H., Aysha, S., and Mani, M. (2021). Resilience of vernacular and modernising dwellings in three climatic zones to climate change. Sci. Rep. 11 (1), 1–14. doi:10.1038/s41598-021-87772-0
Kinnane, O., Grey, T., and Dyer, M. (2017). Adaptable housing design for climate change adaptation. Proc. Institution Civ. Eng. Eng. Sustain. 170 (5), 249–267. doi:10.1680/jensu.15.00029
Kumar, S., Tewari, P., Mathur, S., and Mathur, J. (2017). Development of mathematical correlations for indoor temperature from field observations of the performance of high thermal mass buildings in India. Build. Environ. 122, 324–342. doi:10.1016/j.buildenv.2017.06.030
Kwok, A. G., and Rajkovich, N. B. (2010). Addressing climate change in comfort standards. Build. Environ. 45 (1), 18–22. doi:10.1016/j.buildenv.2009.02.005
Lamberti, G., Leccese, F., Salvadori, G., Contrada, F., and Kindinis, A. (2023). Investigating the effects of climate on thermal adaptation: a comparative field study in naturally ventilated university classrooms. Energy Build. 294 (June), 113227. doi:10.1016/j.enbuild.2023.113227
Lapisa, R., Bozonnet, E., Salagnac, P., and Abadie, M. O. (2018). Optimized design of low-rise commercial buildings under various climates – energy performance and passive cooling strategies. Build. Environ. 132 (January), 83–95. doi:10.1016/j.buildenv.2018.01.029
Lavafpour, Y., and Steve, S. (2014). Impact of the envelope geometry on cooling demand in very airtight UK dwellings under current and future weather projections. Energy Procedia 62, 421–430. doi:10.1016/j.egypro.2014.12.404
Li, Q., Zhang, L., Zhang, L., and Wu, X. (2021). “Optimizing energy efficiency and thermal comfort in building green retrofit.”, Energy 237: 121509. doi:10.1016/j.energy.2021.121509
Liu, W., Huangfu, H., Xiong, J., and Deng, Q. (2014). Feedback effect of human physical and psychological adaption on time period of thermal adaption in naturally ventilated building. Build. Environ. 76, 1–9. doi:10.1016/j.buildenv.2014.02.012
Liu, Y., Dong, Y., Song, C., Wang, Y., Liu, L., and Liu, J. (2017). A tracked field study of thermal adaptation during a short-term migration between cold and hot-summer and warm-winter areas of China. Build. Environ. 124, 90–103. doi:10.1016/j.buildenv.2017.07.022
Lomas, K. J., and Giridharan, R. (2012). Thermal comfort standards, measured internal temperatures and thermal resilience to climate change of free-running buildings: a case-study of hospital wards. Build. Environ. 55, 57–72. doi:10.1016/j.buildenv.2011.12.006
Lomas, K. J., and Ji, Y. (2009). Resilience of naturally ventilated buildings to climate change: advanced natural ventilation and hospital wards. Energy Build. 41 (6), 629–653. doi:10.1016/j.enbuild.2009.01.001
López-Pérez, L. A., Flores-Prieto, J. J., and Ríos-Rojas, C. (2019). Adaptive thermal comfort model for educational buildings in a hot-humid climate. Build. Environ. 150 (November 2018), 181–194. doi:10.1016/j.buildenv.2018.12.011
Luo, M., Zhou, X., Zhu, Y., Zhang, D., and Cao, B. (2016). Exploring the dynamic process of human thermal adaptation: a study in teaching building. Energy Build. 127, 425–432. doi:10.1016/j.enbuild.2016.05.096
Ma, D., Li, X., Lin, B., Zhu, Y., and Yue, S. (2023). A dynamic intelligent building retrofit decision-making model in response to climate change. Energy Build. 284, 112832. doi:10.1016/j.enbuild.2023.112832
Machard, A., Inard, C., Alessandrini, J. M., Devys-Peyre, F., Martinez, S., Ribéron, J., et al. (2023). Climate change influence on buildings dynamic thermal behavior during summer overheating periods: an in-depth sensitivity analysis. Energy Build. 284, 112758. doi:10.1016/j.enbuild.2022.112758
Manu, S., Shukla, Y., Rawal, R., Thomas, L. E., de Dear, R., and De Dear, R. (2016). Field studies of thermal comfort across multiple climate zones for the subcontinent: India model for adaptive control (IMAC). Build. Environ. 98, 422–426. doi:10.1016/j.buildenv.2015.12.019
Masoud, S. S., and Sharples, S. (2017). “Quantifying the behaviour of modern and traditional construction systems on the basis of thermal comfort,” in PLEA conference proceedings: design to thrive.
Meshack, E. (2017). “The ‘second skin’ and thermal adaptation in changing climate,” in PLEA Conference Proceedings: Design to Thrive, 49–56.
Mishra, A. K., and Ramgopal, M. (2013). Field studies on human thermal comfort - an overview. Build. Environ. 64, 94–106. doi:10.1016/j.buildenv.2013.02.015
Möller, V., van Diemen, R., Matthews, J. B. R., Méndez, C., Semenov, S., Fuglestvedt, J. S., et al. (2023). “IPCC 2022: annex II: glossary.”, In Climate change 2022: impacts adaptation and vulnerability Contribution of working group II to the sixth assessment report of the intergovernmental Panel on climate change. Editors H. O. Pörtner, D. C. Roberts, M. Tignor, E. S. Poloczanska, and K. Mintenbeck (Cambridge University Press), 2897–2930. doi:10.1017/9781009325844.029
Motealleh, P., Zolfaghari, M., and Parsaee, M. (2018). Investigating climate responsive solutions in vernacular architecture of Bushehr city. HBRC J. 14 (2), 215–223. doi:10.1016/j.hbrcj.2016.08.001
Parkinson, T., de Dear, R., and Brager, G. (2020). Nudging the adaptive thermal comfort model. Energy Build. 206, 109559. doi:10.1016/j.enbuild.2019.109559
Philokyprou, M., Aimilios, M., Malaktou, E., and Savvides, A. (2017). Environmentally responsive design in eastern mediterranean. The case of vernacular architecture in the coastal, lowland and mountainous regions of Cyprus. Build. Environ. 111, 91–109. doi:10.1016/j.buildenv.2016.10.010
Porritt, M., Stephen, P. C. C., Shao, Li, and Goodier, C. I. (2013). Heat wave adaptations for UK dwellings and development of a retrofit toolkit. Int. J. Disaster Resil. Built Environ. 4 (3), 269–286. doi:10.1108/IJDRBE-08-2012-0026
Porritt, S., Shao, L., Paul, C., and Goodier, C. (2011). Adapting dwellings for heat waves. Sustain. Cities Soc. 1 (2), 81–90. doi:10.1016/j.scs.2011.02.004
Porritt, S. M. (2012). Adapting dwellings to climate change - retrofit advice tool. Available at: http://www.extreme-weather-impacts.net/toolkit/.
Pyke, C. R., McMahon, S., Larissa, L., Rajkovich, N. B., and Adam, R. (2012). Development and analysis of climate sensitivity and climate adaptation opportunities indices for buildings. Build. Environ. 55, 141–149. doi:10.1016/j.buildenv.2012.02.020
Pyrgou, A., Lucia Castaldo, V., Anna Laura, P., Franco, C., and Santamouris, M. (2017). On the effect of summer heatwaves and urban overheating on building thermal-energy performance in Central Italy. Sustain. Cities Soc. 28, 187–200. doi:10.1016/j.scs.2016.09.012
Ramli, N. H. (2012). Re-adaptation of Malay house thermal comfort design elements into modern building elements – case study of selangor traditional Malay house & low energy building in Malaysia. Iran. J. Energy & Environ. 3, 19–23. doi:10.5829/idosi.ijee.2012.03.05.04
Roaf, S., Crichton, D., and Nicol, F. (2009). Adapting buildings and cities for climate change - a 21st century survival guide second edition. Available at: http://library.uniteddiversity.coop/Ecological_Building/Adapting_Buildings_and_Cities_for_Climate_Change.pdf.
Rubio-Bellido, , Carlos, J. A. P. A., and Cabeza-Lainez, J. M. (2015). Adaptation strategies and resilience to climate change of historic dwellings. Sustain. Switz. 7 (4), 3695–3713. doi:10.3390/su7043695
Saifudeen, A., and Mani, M. (2017). “Formulating a methodology to study adaptability in buildings to climate change: climate-responsive adaptability in Indian conditions.” In PLEA Conference Proceedings: Design to Thrive, 128–135.
Sanders, C. H., and Phillipson, M. C. (2003). UK adaptation strategy and technical measures: the impacts of climate change on buildings. Build. Res. Inf. 31 (3–4), 210–221. doi:10.1080/0961321032000097638
Sands, P. (1992). The united Nations framework convention on climate change. Rev. Eur. Community Int. Environ. Law 1 (3), 270–277. doi:10.1111/j.1467-9388.1992.tb00046.x
Sengupta, N. (2008). Use of cost-effective construction technologies in India to mitigate climate change. Curr. Sci. 94 (1), 38–43.
Shastry, V., Mani, M., and Tenorio, R. (2016). Evaluating thermal-comfort and building climatic-response in warm-humid climates for vernacular dwellings in suggenhalli (India). Archit. Sci. Rev. 59 (1), 12–26. doi:10.1080/00038628.2014.971701
Shaw, R., Colley, M., and Connell, R. (2007). Climate change adaptation by design: a guide for sustainable communities. London: TCPA. http://www.tcpa.org.uk/data/files/bd_cca.pdf.
Shaw, R., Luo, Y., Cheong, T. S., Abdul Halim, S., Chaturvedi, S., Hashizume, M., et al. (2023). “2022: asia climate change 2022: impacts, adaptation and vulnerability,” in Contribution of working group II to the sixth assessment report of the intergovernmental Panel on climate change. Editors H.-O. Pörtner, D. C. Roberts, M. Tignor, E. S. Poloczanska, K. Mintenbeck, A. Alegríaet al. (Cambridge University Press), 1457–1580. doi:10.1017/9781009325844.012
Short, C. A., Lomas, K. J., Giridharan, R., and Fair, A. J. (2012). Building resilience to overheating into 1960’s UK hospital buildings within the constraint of the national carbon reduction target: adaptive strategies. Build. Environ. 55, 73–95. doi:10.1016/j.buildenv.2012.02.031
Singh, M. K., Mahapatra, S., and Atreya, S. K. (2008). “SUSTAINABILITY THROUGH BIOCLIMATIC BUILDING DESIGN IN NORTH-EAST INDIA,” in ISES-AP - 3rd International Solar Energy Society Conference – Asia Pacific Region (ISES-AP-08) Incorporating the 46th ANZSES Conference, 1–10.1
Singh, P. K., and Dhiman, R. C. (2012). Climate change and human health: Indian context. J. Vector Borne Dis. 49 (2), 55–60.
Stephanie, O., and Sharples, S. (2017). “Impact of building envelope construction on thermal comfort: a parametric analysis of modern, low income housing in south-west Nigeria for current and future climates.” In PLEA Conference Proceedings: Design to Thrive, 113–120.
Teo, E. A., and Lin, G. (2011). Determination of strategic adaptation actions for public housing in Singapore. Build. Environ. 46 (7), 1480–1488. doi:10.1016/j.buildenv.2011.01.021
United Nations Framework Convention on Climate Change (2015). United Nations framework convention on climate change. Paris Agreement.
Ürge-Vorsatz, D., Lucon, O., A Zain Ahmed, H. A., Bertoldi, P., Cabeza, L. F., Eyre, N., et al. (2014). “Buildings,” in Mitigation. Working group III contribution to the fifth assessment report of the intergovernmental Panel of climate change, 671–738.
U.S. Congress (1993). Preparing for an uncertain climate. United States: Office of Technology Assessment I.
Wan, K. K. W., Li, D. H. W., and Lam, J. C. (2011). Assessment of climate change impact on building energy use and mitigation measures in subtropical climates. Energy 36 (3), 1404–1414. doi:10.1016/j.energy.2011.01.033
Wang, X., Chen, D., and Ren, Z. (2010). Assessment of climate change impact on residential building heating and cooling energy requirement in Australia. Build. Environ. 45 (7), 1663–1682. doi:10.1016/j.buildenv.2010.01.022
Weber, W., and Yannas, S. (2013). Lessons from vernacular architecture. London and New York: Earthscan from Routledge. doi:10.4324/9780203756164
Wilkinson, S. J., Remøy, H., and Langston, C. (2014). Sustainable building adaptation. Sustainable building adaptation: innovations in decision-making. Oxford: John Wiley & Sons. doi:10.1002/9781118477151
Williams, K., Jennifer, L. R. J., Payne, C., Hopkins, D., and Smith, I. (2012). The conditions for, and challenges of, adapting england’s suburbs for climate change. Build. Environ. 55, 131–140. doi:10.1016/j.buildenv.2011.11.015
Wilson, A., and Ward, A. (2009). Design for adaptation: living in a climate-changing world. Environ. Build. News, 1–9. Available at: http://www.buildinggreen.com/auth/article.cfm/2009/8/28/Design-for-Adaptation-Living-in-a-Climate-Changing-World/.
Zhai, Z. J., and Michael Helman, J. (2019). Implications of climate changes to building energy and design. Sustain. Cities Soc. 44, 511–519. doi:10.1016/j.scs.2018.10.043
Zune, M., Rodrigues, L., and Gillott, M. (2020). Vernacular passive design in Myanmar housing for thermal comfort. Sustain. Cities Soc. 54, 101992. doi:10.1016/j.scs.2019.101992
Keywords: adaptation mechanisms, adaptation strategies, climate change, climate-responsive, thermal adaptation, sustainable building adaptation, vulnerability assessment
Citation: Saifudeen A and Mani M (2024) Adaptation of buildings to climate change: an overview. Front. Built Environ. 10:1327747. doi: 10.3389/fbuil.2024.1327747
Received: 25 October 2023; Accepted: 05 February 2024;
Published: 16 February 2024.
Edited by:
Osama Yousef Ayadi, The University of Jordan, JordanReviewed by:
Hasim Altan, Prince Mohammad Bin Fahd University, Saudi ArabiaMitja Košir, University of Ljubljana, Slovenia
Copyright © 2024 Saifudeen and Mani. This is an open-access article distributed under the terms of the Creative Commons Attribution License (CC BY). The use, distribution or reproduction in other forums is permitted, provided the original author(s) and the copyright owner(s) are credited and that the original publication in this journal is cited, in accordance with accepted academic practice. No use, distribution or reproduction is permitted which does not comply with these terms.
*Correspondence: Aysha Saifudeen, ayshas@iisc.ac.in