- Key Laboratory of Beijing for Identification and Safety Evaluation of Chinese Medicine, State Key Laboratory of Innovative Natural Medicine and TCM Injections, Institute of Chinese Materia Medica, China Academy of Chinese Medical Sciences, Beijing, China
Asarum (Xixin), which contains analogues of aristolochic acid (AA), is the only species of the genus Aristolochia included in the Chinese Pharmacopoeia 2020. However, the contents and nephrotoxic effects of AA analogs in Asarum (Xixin) and its formulations have not been clarified. An automatic, effective solid phase extraction process and UPLC-MS/MS method were established for the pretreatment and quantitative detection of AA analogues in commercially available traditional Chinese patent medicines. The cytotoxicity and DNA damage induced by five analogues of AA were evaluated by CCK8 using human kidney cells (HK-2) and comet assays. HPLC was used to detect the analogues of AA in Asarum heterotropoides F. Schmidt (Xixin). The results showed that the contents of AA I, AA II, and AA IIIa were below the detection limit, while AA IVa and AL I presented relatively high contents of Asarum heterotropoides F. Schmidt (Xixin), within the range of 66.50–121.03 μg/g and 19.73–43.75 μg/g, respectively. The levels of AA analogues were in the nanogram-per-gram level in the main traditional Chinese patent medicines. AA I and AL I exhibited relatively high cytotoxicity at 48 h in CCK8 assays, while AA II, AA IIIa, and AA IVa showed weak cytotoxicity even at 800–1,000 μM. AA I induced significant pathological alterations and direct DNA damage at 40 mg/kg and 20 mg/kg, respectively. No distinct nephrotoxicity or hepatotoxicity was observed in mice treated with AA II, AA IIIa, AA IVa, or AL I at 40 mg/kg in this study. Consumption of Asarum heterotropoides F. Schmidt (Xixin) with controlled doses and periods is relatively safe as the contents of AA analogues in Asarum heterotropoides F. Schmidt (Xixin) and its formulations were far below those causing acute toxicity in this study. But, the long-term toxicity of Asarum heterotropoides F. Schmidt (Xixin) still needs further study.
Introduction
Aristolochic acid (AA) exists mainly in plants of the Aristolochiaceae family that have been used in pharmaceutical formulations for hundreds of years to treat various diseases (Heinrich et al., 2009; Li et al., 2017). Exposure to AA is associated with nephropathy and upper tract urothelial carcinoma (UUC) (Heinrich et al., 2009; Wu and Wang, 2013; Yang et al., 2014). AA is also the causative agent of Balkan Endemic Nephropathy (BEN), a chronic, progressive renal disease, which arises from the consumption of bread in which wheat grain is contaminated with the weed of Aristolochia clematitis (Jelaković et al., 2012; Jelaković et al., 2019). There are various analogues of AA (AAA) in plants of the Aristolochiaceae family (Kuo et al., 2012; Mao et al., 2017). Among these, AA I and AA II are considered the main active components of Aristolochia species (Debelle et al., 2008). AA I and its metabolites can react with DNA to form covalent adducts, such as dA-AL I and dG-AL I, which are characteristic markers of exposure to AAs (Stiborová et al., 2017; Sidorenko, 2020). The adducts can induce adenine-to-thymine (A>T) transversions, which have been detected both in vitro and in vivo (Chen et al., 2012; Chen et al., 2013).
In 2002, AA I was listed as class I carcinogen by WHO due to its nephrotoxicity and carcinogenicity. Subsequently, most Aristolochia plants have been prohibited for clinical use in many countries and districts except Asarum (Xixin), as it contains relatively low levels of AA I and AA II (Jong et al., 2003). Furthermore, some studies have shown that most Asarum (Xixin) products are not cytotoxic in HK-2 cells in vitro (Li Y.-L. et al., 2010; Michl et al., 2017). Recently, traditional Chinese herbal remedies containing AA have been statistically associated with human liver cancer, based on an AA-associated mutation in patients with hepatocellular carcinoma (HCC) patients (Hsieh et al., 2008; Hoang et al., 2013; Ng et al., 2017). The formation of aristolactam–DNA adducts induced significant A>T transversions in TP-53 and JAK1 genes, and initiated liver cancer both in mouse and human liver cancer models (Lu et al., 2020). AA I can also promote the invasion and migration of HCC by activating the complement system C3a/C3aR (Li et al., 2020). Although there is still controversy about the role of AA in HCC, its toxicity should not be ignored. As the only species of the genus Aristolochia allowed in the Chinese Pharmacopoeia 2020, the rationality for the clinical application of Asarum (Xixin) has aroused public concern.
According to our data, there are almost 200 types of traditional Chinese medicines containing Asarum (Xixin) on the Chinese mainland. Since AA I can induce an exceptionally long-term persistence of DNA adducts and irreversible nephrotoxicity, the content of AA I should be strictly controlled (Li et al., 2011; Yang L. et al., 2012; Schmeiser et al., 2014). However, the exact contents of AA I and AA II in Asarum (Xixin) from natural sources and its formulated products are not clear. Besides the trace amounts of AA I and AA II, there are many other AA analogues in Asarum (Xixin) (Wen et al., 2014; Michl et al., 2017). Whether they played a role in the AAN progression was not well known. The toxicities of these analogues of AA may have been neglected (Michl et al., 2014).
One aim of this study was to quantitatively detect the contents of AA I, AA II, AA IIIa, AA IVa, and AL I in Asarum heterotropoides F. Schmidt (Xixin) and its formulated products. The other was to assess their in vitro and in vivo toxicities, to provide a rationale for the clinical application of Asarum heterotropoides F. Schmidt (Xixin). HPLC was usually used for the detection and quantification of AA analogs in crude drugs (Li W. et al., 2004; Xu et al., 2013). However, it is not sensitive enough to detect the trace amounts of AA analogues in traditional Chinese patent medicines (Chen et al., 2020). In this study, an efficient solid phase extraction (SPE) and UPLC-MS/MS method was developed to quantitatively detect the contents of AA I, AA II, AA IIIa, AA IVa, and AL I in Asarum heterotropoides F. Schmidt (Xixin) of different origins and commercially available traditional Chinese patent medicines. Cytotoxicity, acute toxicity, and comet assays were performed to compare the in vitro and in vivo toxicity of different AA analogues, to provide a theoretical basis for the safe clinical application of Asarum heterotropoides F. Schmidt and its formulated products.
Materials and Methods
Samples and Solvents
Fifteen batches of Asarum heterotropoides F. Schmidt (Xixin) samples were purchased from different districts of the Chinese mainland with batch numbers XX01–XX15. All were authenticated as the roots and rhizomes of Asarum heterotropoides F. Schmidt (Xixin) by Prof. Jinda Hao, Institute of Chinese Materia Medica, China Academy of Chinese Medical Sciences. Traditional Chinese patent medicines containing herbs from the Aristolochiaceae family were collected from online and offline pharmacies.
The AA I reference standard (purity >98%) was purchased from the National Institutes of Food and Drug Control (Beijing, China). AA II, AA IIIa, AA IVa, and AL I (purities >98%) reference standards were obtained from Chengdu Push Bio-technology Co., Ltd. (Chengdu, China). Methanol, acetonitrile, formic acid, and ammonium acetate (HPLC and MS/MS grade) were purchased from Fisher Scientific (MA, United States). Aspartate amino transferase (AST), alanine amino transferase (ALT), alkaline phosphatase activities (ALP), creatinine (CREA), blood urea nitrogen (BUN), total bile acid (TBA), and total bilirubin (TBIL) were purchased from Hua Sin Science Co., Ltd (Guangzhou, China).
Chromatographic Separation and ESI-MS/MS
The Agilent Eclipse XDB C18 column (4.6 × 150 mm, 5 μm) was used for chromatographic separations of the analogues of AA in Asarum heterotropoides F. Schmidt (Xixin). The mobile phases were acetonitrile containing 0.1% formic acid (A) and water containing 0.1% formic acid (B). Separations were performed as follows: 0–5 min, 30–30% A; 5–5.01 min, 30–36% A; 5.01–26 min, 36–43% A; 26–28 min, 43–43% A; 28–28.01 min, 43–30% A; 28.01–30 min, 30–30% A. The flow rate was 1.0 ml/min. AA analogues were detected at a wavelength of 254 nm. The column temperature was maintained at 20–23°C. Samples (10 μl) were injected into the HPLC system.
Quantitative determination of analogues of AA in traditional Chinese patent medicines was performed with a UPLC-MS/MS system consisting of an ACQUITY UPLC I-Class coupled to a Xevo-TQS detector (Waters, United States). Chromatographic separation was achieved using an ACQUITY UPLC BEH C18 column (2.1 × 50 mm, 1.7 μm; Waters, United States) with a flow rate of 0.3 ml/min at 35°C. Separation was obtained with the following gradient: 0–1.0 min, 10% B; 1.0–7.0 min, 10–75% B; 7.0–7.2 min, 75–95% B; 7.2–10.2 min, 95% B; 10.2–10.3 min, 95–10% B, 10.3–12.0 min, 10% B (A: water with 0.01% formic acid, B: methanol with 0.01% formic acid). Both mobile phases were added with 5 mM ammonium acetate and treated under ultrasonication for 5 min before usage. The injection volume was 1.0 μl.
Multiple reaction monitoring mode (MRM) and positive electrospray ionization were applied for the quantitative determination. The instrument parameters were as follows: source temperature, 150°C; capillary voltage, 3.5 kV; desolvation gas flow, 800 L/h; cone gas flow, 150 L/h; nebulizer gas, 7.0 bar; desolvation temperature, 550°C. Details of the parent/daughter ions, cone voltages, and collision energies for AA I, AA II, AA IIIa, AA IVa, and AL I are listed in Supplementary Table S2.
Sample Preparation
Asarum heterotropoides F. Schmidt (Xixin) samples were crushed into a powder and 1.0 g was placed in a conical flask, followed by cold immersion with 20 ml of 70% ethanol for 1 h and ultrasonic extraction (250 W, 40 kHz) for 30 min. The supernatant was collected and the residue was extracted again according to the same procedures. Then the supernatants were combined and filtered through a 0.22-μm membrane prior to HPLC determination.
Traditional Chinese patent medicines (including capsules, granules, tablets, powders and water pills) were crushed and filtered through a 60-mesh sieve. Honeyed pill was cut into small pieces. Each 50.0 mg sample was accurately weighed and placed in a 10 ml centrifuge tube, to which 4 ml of methanol was added. The mixture was ultrasonicated for 90 min at room temperature and then centrifuged at 4,000 rpm for 15 min. The supernatant was collected and dried with nitrogen at 45°C. The residue was redissolved with 1.0 ml of 30% methanol and the pH was adjusted to 8.0–9.0 using aqueous ammonia. Biotage Extrahera (Biotage, Sweden) was applied for the automatic solid phase extraction process. Waters Oasis MAX Cartridge 3 cc/60 mg columns (Waters, United States) were first activated by 3.0 ml of methanol and 3.0 ml of water. Then the columns were balanced with 3.0 ml of 30% methanol. The samples were loaded and washed sequentially with 5% aqueous ammonia and 60% methanol, followed by elution with 3.0 ml of methanol and 4.0 ml of methanol containing 8% FA. The eluent was collected and dried with nitrogen. Then the residue was redissolved with 1.0 ml methanol and centrifuged at 13,000×g for 15 min before UPLC-MS/MS detection.
In Vitro Toxicity Assays
Cytotoxicity analysis was determined using the Cell Counting kit 8 (CCK8) (Bioss, China). HK-2 was cultured in DMEM/F12 (containing 10% fetal bovine serum, 100 U/ml penicillin, and 100 μg/ml streptomycin; Gibco, Invitrogen) at 37°C in 5% carbon dioxide incubator. The cells were maintained at 80% confluency and the medium was replaced every 3 days for routine cultivation. HK-2 cells (8,000 cells/well) were seeded in 96-well plates overnight. The cells were then treated with analogues of AA for 24 or 48 h at different concentrations (6.8, 27.0, 54.0, 108.0, 216.0, and 432.0 μM for AA I; 12.5, 25.0, 100.0, 200.0, 400.0, and 800.0 μM for AA II; 15.6, 62.5, 125.0, 250.0, 500.0, and 1000.0 μM for AA IIIa; 15.6, 31.3, 62.5, 125.0, 500.0, and 1,000.0 μM for AA IVa; 8.0, 16.0, 32.0, 64.0, 128.0, and 256.0 μM for AL I). Subsequently, the cells were incubated with 10% CCK8 solution for another 2 h. The final concentration of DMSO in the medium did not exceed 1.0% v/v. The absorbance (OD) of each well was measured with a spectrophotometer (Spark; Tecan, Switzerland) at 450 nm and the IC50 values were obtained by fitting the curve through nonlinear regression with GraphPad Prism 7.0 (GraphPad Software, CA, United States).
In Vivo Toxicity Assays
The animal experiments were approved by the Research Ethics Committee of the Institute of Chinese Materia Medica, China Academy of Chinese Medical Sciences (ICMM, CACMS). The experiment was carried out according to ethical guidelines and regulations for the use of laboratory animals. A total of 160 ICR mice were randomly divided into 16 groups, each comprising 10 mice (5 males and 5 females). The drugs were administered in a single dose by oral gavage and the dose volume was 0.2 ml/10 g. The control group was treated with 0.5% CMC-Na. Other groups received AA I, AA II, AA IIIa, AA IVa, or AL I, at concentrations of 10, 20, and 40 mg/kg, respectively. Animal weights were recorded every two days. The animals were fasted overnight before experiments and sacrificed on day 14. Blood samples were collected and centrifuged for 15 min at 3,500×g. AST, ALT, ALP, CREA, BUN, TBA, and TBIL levels in plasma were examined. SPSS 17.0 (IBM Corp., United States) was used for the statistical and significance analyses. The renal and liver tissues were embedded and stained with H&E. Histological images were obtained through Olympus BX63 (Olympus Corporation, Japan) at a magnification of ×200.
Comet Assays
ICR mice were separated into seven groups (n = 5 for each group) and administrated with saline, EMS (577.6 mg/kg), AA I (20 mg/kg), AA II (20 mg/kg), AA IIIa (20 mg/kg), AA IVa (20 mg/kg), or AL I (20 mg/kg) for three consecutive days, respectively. After anesthetization and perfusion, renal and liver tissues were obtained. Comet analysis was performed 2–6 h after the last lavage and the processes were according to Günter Speit (Speit and Rothfuss, 2012). The Comet Assay software project (CASP) (V1.2.3) was used to analyze the fraction of tail DNA and the Olive Tail Moment. A total of one hundred cells from kidney or liver tissues of each animal were analyzed. The mean, standard deviation (SD) of tail DNA%, and the median of olive tail moment were calculated. Results were analyzed using SPSS 17.0 by one-way analysis of variance (ANOVA) followed by the least significance difference (LSD) test. The significant differences between the groups were tested and defined by p-value < 0.05.
Results
Quantitative Determination of Analogues of AA in Asarum heterotropoides F. Schmidt (Xixin) and Traditional Chinese Patent Medicines
High-performance liquid chromatography (HPLC) was applied to determine the content of AA analogues (Figure 1) in roots and rhizomes of 15 batches of Asarum heterotropoides F. Schmidt (Xixin). The method showed good separation and reproducibility. AA I, AA II, AA IIIa, IVa, and AL I were eluted at 24.36, 20.81, 9.36, 11.23 and 20.31 min, respectively. The quantitative results are shown in Table 1. AA I, AA II, and AA IIIa were not detected in any of the samples, which may be partially attributed to the detection limit of the HPLC instrument. Relative levels of AA IVa and AL I were found in all samples. The contents of AA IVa and AL I were in the ranges of 66.50–121.03 μg/g and 19.73–43.75 μg/g, respectively. XX04 from Liaoning contained the least AA analogues. There were some regional differences in the contents of AA IVa and AL I, but the changes were within the range of 2 fold.
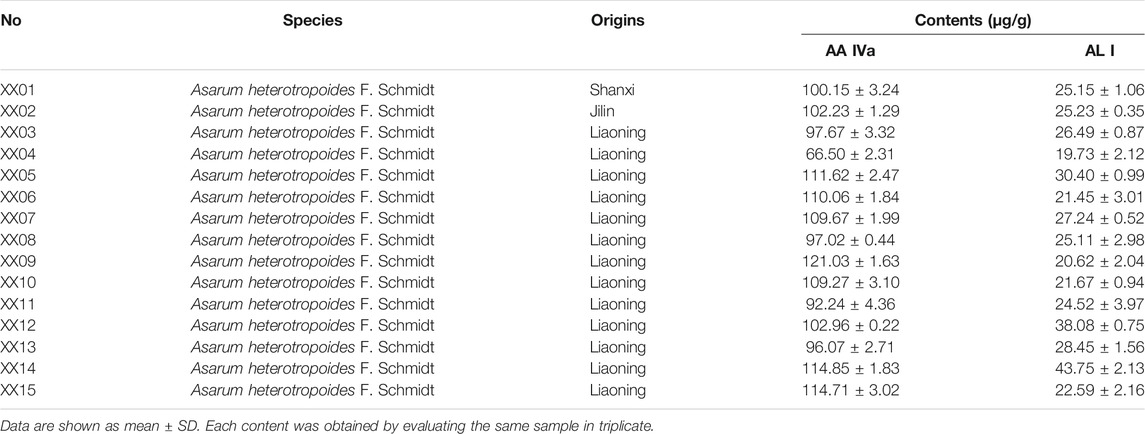
TABLE 1. Plant information and contents of AA IVa and AL I in Asarum heterotropoides F. Schmidt (Xixin)
An effective and automatic SPE method was established and successfully applied in the pretreatment of traditional Chinese patent medicines. Recovery of each analyte was within the range of 86.50–111.88% at three concentration levels (Supplementary Table S4). The contents of AA analogues (AA I, AA II, AA IIIa, AA IVa, and AL I) in 44 commercially available traditional Chinese patent medicines were obtained by UPLC-MS/MS. AA I, AA IVa, and AL I were detected in most traditional Chinese patent medicines evaluated, while AA II and AA IIIa were only detected in few traditional Chinese patent medicines (Table 2, Supplementary Table S5). 70% of the samples tested contained AA I, with concentrations ranging from 0.010–52.450 μg/g. High levels of AA I (3.41–52.45 μg/g) were found in the Ershiwuweisongshi pill, the Duzhongzhuanggu capsule, and the Jingzhikesoutanchuan pill, which contained processed Aristolochia debilis Siebold & Zucc. (Madouling) or Aristolochia mollissima Hance (Xungufeng), which have been reported to have a high content of AA I (Yu et al., 2016; Mao et al., 2017). As for medicines containing only Asarum heterotropoides F. Schmidt (Xixin), a species of the genus Aristolochia, the content of AA I was below 100 ng/g. AA IVa was identified in 86.6% of the samples and the concentrations were within the range of 0.005–1.287 μg/g. Variable content of AL I was detected in all traditional Chinese patent medicines, except for the Shenmei Yangwei and the Shenqi Jianwei Granules. The highest concentration of AL I was found in the Shaqi pill at 5.565 μg/g.
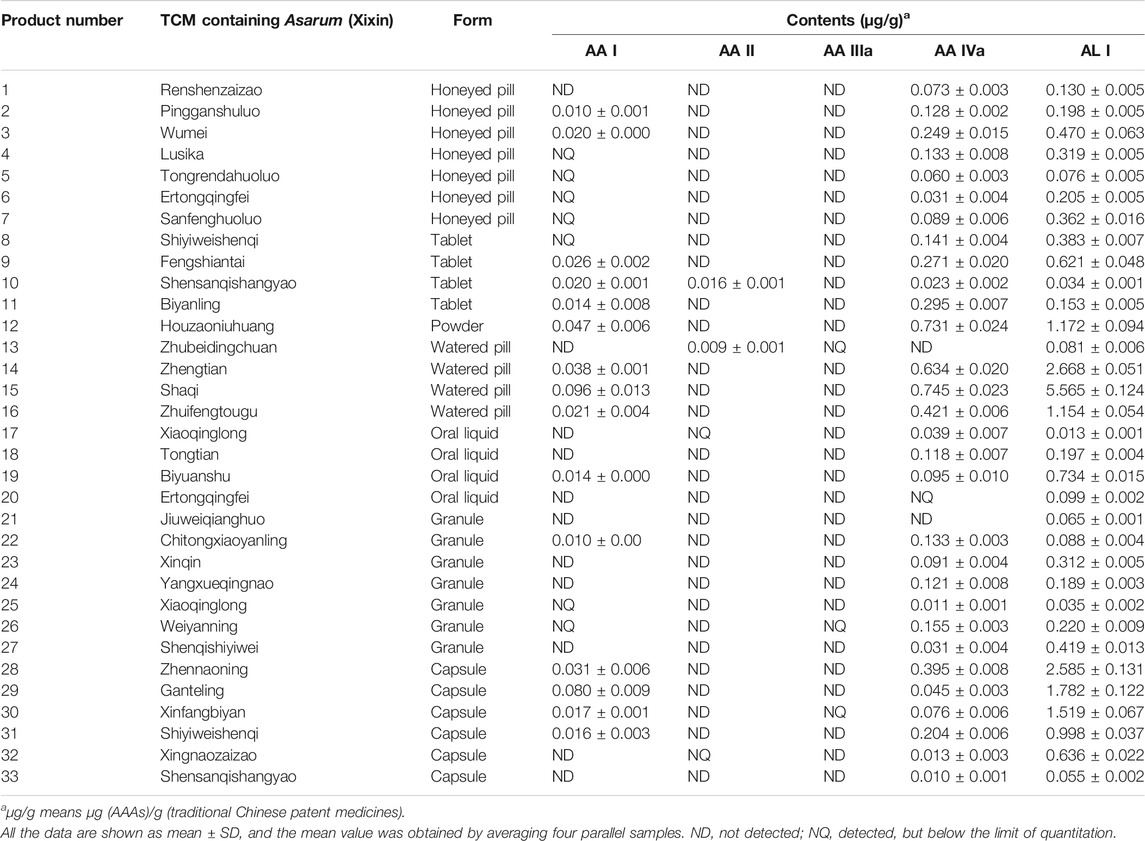
TABLE 2. Quantitative determination of aristolochic acid analogues in traditional Chinese patent medicines containing Asarum heterotropoides F. Schmidt (Xixin)
Different dosage forms of traditional Chinese patent medicines with the same composition contained different amounts of AA analogues. For example, the contents of AA I, AA II, AA IVa and AL I in the Shensanqishangyao tablet were 0.020, 0.016, 0.023, and 0.034 µg/g, respectively. However, AA I or AA II were not detected in the Shensanqishangyao capsule. And the contents of AA IVa and AL I were 0.010 and 0.055 µg/g, respectively. The origins of the herb and the processing method of Asarum heterotropoides F. Schmidt (Xixin) may account for the content differences of the AA analogues.
In Vitro and In Vivo Toxicity of Analogues of AA
According to the quantitative results, the contents of AA I, AA II, and AA IIIa were low, while the levels of AA IVa and AL I were relatively high in most Asarum heterotropoides F. Schmidt (Xixin)–containing medicines. We questioned whether AA IVa and AL I could play a role in the nephrotoxicity of Aristolochia. Thus, we conducted both in vitro and in vivo toxicity assays. The cytotoxicity of the AA analogues was evaluated using the CCK-8 assay. HK-2 cells were treated with AA analogues for 24 or 48 h. AA analogues showed variable degrees of cytotoxic effects in HK-2 cells, with AA I and AL I exhibiting the strongest cytotoxicity (Figure 2). The IC50 values of AA I were 197.3 μM for 24 h and 76.7 μM for 48 h, respectively. AA II showed relatively weak cytotoxicity with IC50 > 800 μM for 24 h and 306.5 μM for 48 h. The cell viability was not lower than 50% of control at a concentration of 1,000 μM for AA IIIa and AA IVa at 24 or 48 h, suggesting low cytotoxicity in HK-2 cells. AL I exhibited relatively low cytotoxicity at 24 h with IC50 >256 μM. However, the toxicity rapidly enhanced with increasing incubation time. After treatment for 48 h, the IC50 value of AL I was reduced to 37.1 μM, exhibiting even stronger cytotoxicity than AA I. Despite the varying cytotoxicity, all AA analogues showed a time- and concentration-dependent inhibition to HK-2 cells.
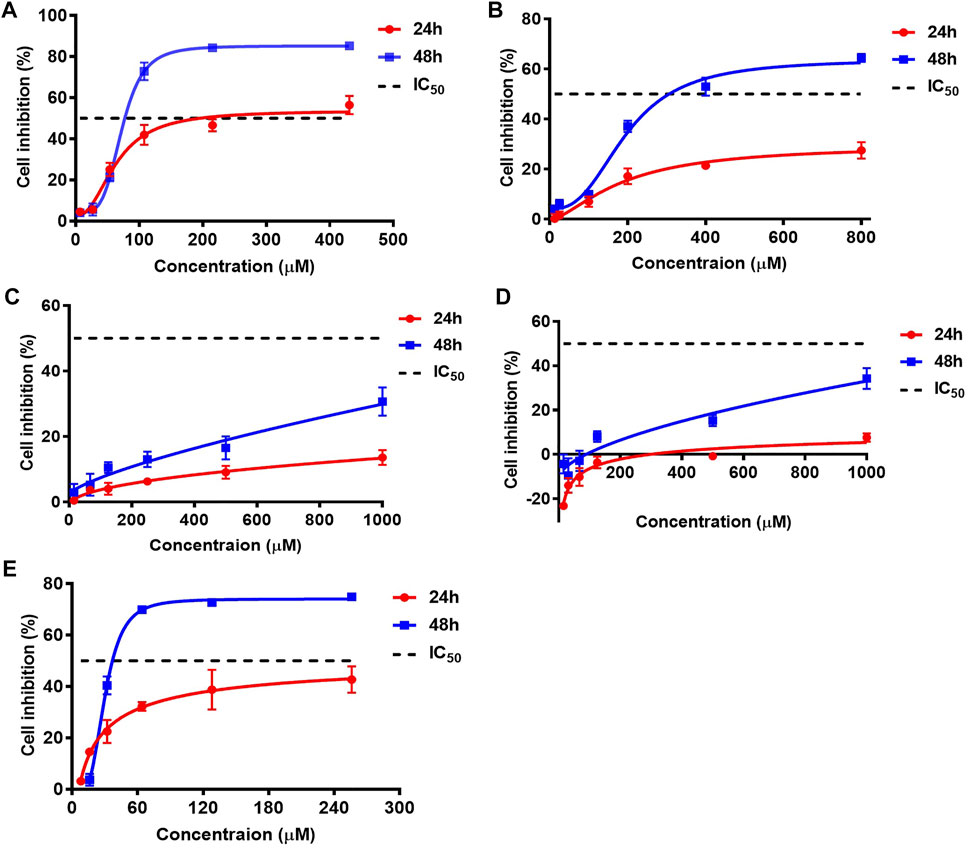
FIGURE 2. Cell inhibitions of HK-2 after treating with AA I (A), AA II (B), AA IIIa (C), AA IVa (D), and AL I (E) for 24 or 48 h. IC50 values of AAAs were obtained by nonlinear regression and least square fitting of the data to [inhibitor] vs. response-variable slope (four parameters) model using GraphPad Prism 7.0. Inhibition data were calculated from five parallels at each concentration and repeated for three times.
To further evaluate the in vivo toxicity of AA analogues, mice were administered single doses of AA I, AA II, AA IIIa, AA IVa, and AL I at 10, 20, and 40 mg/kg, respectively. The control group received 0.05% CMC-Na. Mice administered with AA I achieved a remarkable reduction of body weight compared to the control group. In addition, the body weight decreased with the increasing doses of AA I for both male and female mice (Supplementary Figure S3). Slight weight loss was also observed in male and female mice treated with AA II (40 mg/kg) during the first 10 days. However, the body weight of female mice increased after 10 days and there was no significant difference with the control group. Regarding AA IIIa, AA IVa, and AL I, no distinct changes in body weight were observed between the different dosages and the control group. Female mice treated with AA IVa (40 mg/kg) experienced a drastic weight loss on day 5, but the weight returned to the same level as in the other groups on day 7.
Survival of the control and treated groups were monitored for 14 days. As shown in Figure 3, mice (n = 7 of 10) treated with 40 mg/kg AA I were dead on days 4, 5, and 6 due to the acute kidney failure and the survival rate was 30%. 10% of mice died (n =1 of 10) on day 8 in the group treated with AA II (40 mg/kg). There was no death occurring in control, AA I (10, 20 mg/kg), AA II (10, 20 mg/kg), AA IIIa (10, 20, 40 mg/kg), AA IVa (10, 20, 40 mg/kg), AL I (10, 20, 40 mg/kg) treated groups.
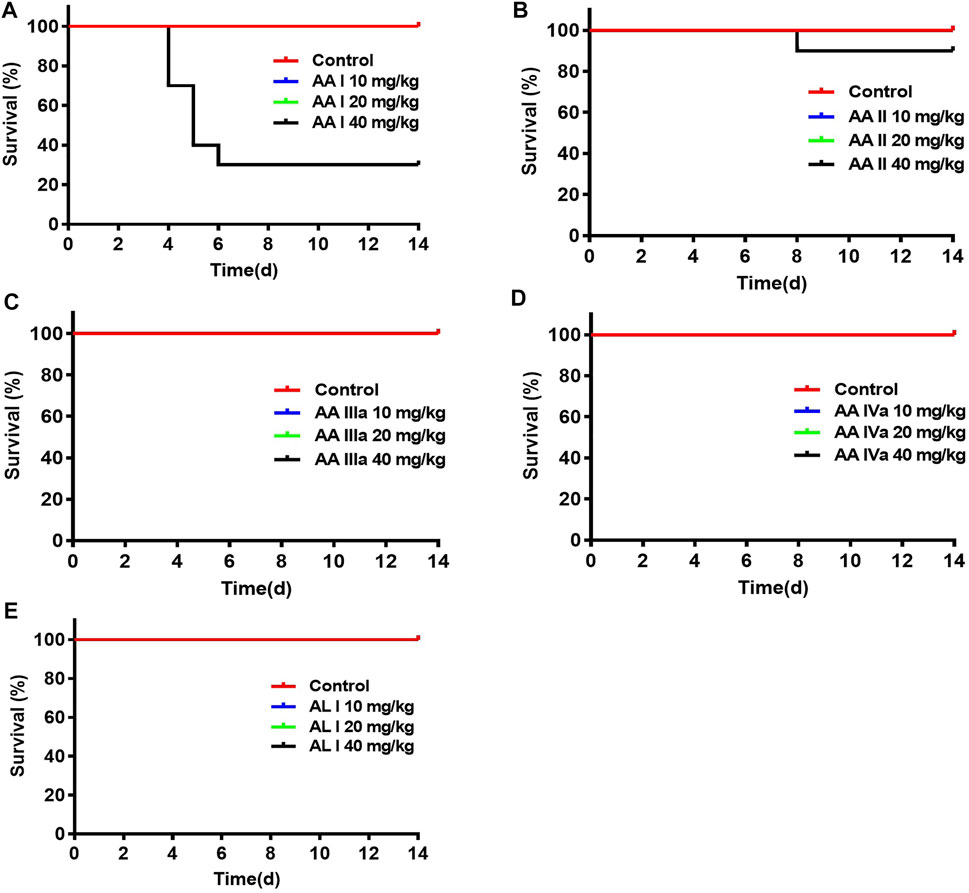
FIGURE 3. Survivals of mice administered with aristolochic acid analogues: AA I (A), AA II (B), AA IIIa (C), AA IVa (D), and AL I (E). ICR mice were administered with aristolochic acid analogues at concentrations of 10, 20, and 40 mg/kg (n = 10 for each group). The curves were created by GraphPad Prism 7.0.
After mice were sacrificed, kidney and liver tissue were obtained for histopathological analysis. Significant lesions were only found in the renal tissues of mice treated with 40 mg/kg AA I. As shown in Figure 4, renal pathological changes were characterized with large area necrosis of renal tubules, epithelial degeneration of renal tubules, and granular casts in renal tubule lumen. Although there were different levels of changes in serum levels of TBIL, ALP, BUN, and CREA in AA I (20 mg/kg, 40 mg/kg) treated mice (Figure 5), no corresponding pathological changes were observed in the liver tissues of the same group (Supplementary Figure S5). The activities of AST, ALT, ALP and the concentrations of TBIL, TBA, CREA and BUN in AA II, AA IIIa, AA IVa, and AL I treated groups showed no significant difference compared to the control group, except for decreased AST, ALT, and ALP activity in the group treated with AL I (10 mg/kg). No pathological changes were observed in the liver tissues of mice treated with AL I.
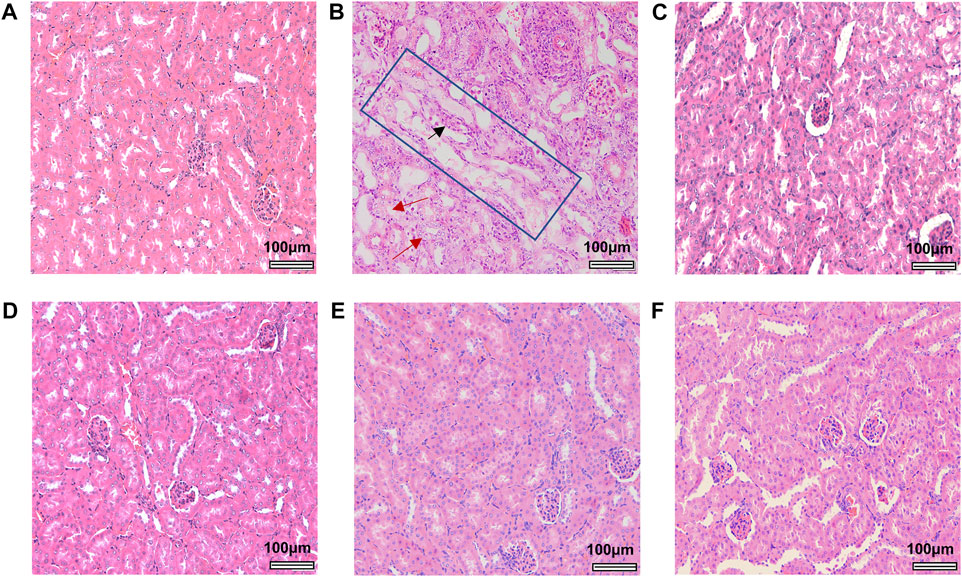
FIGURE 4. Histological images of renal tissues from control and aristolochic acid analogues treated male mice at a concentration of 40 mg/kg. Control (A), AA I (B), AA II (C), AA IIIa (D), AA IVa (E), and AL I (F). Large areas of renal tubule necrosis were outlined in the blue rectangle. Red arrows indicate areas of epithelial degeneration of renal tubules. Black arrow indicates the granular casts in renal tubule lumen. All the images were obtained at the same magnification: ×200, scale bar = 100 μm.
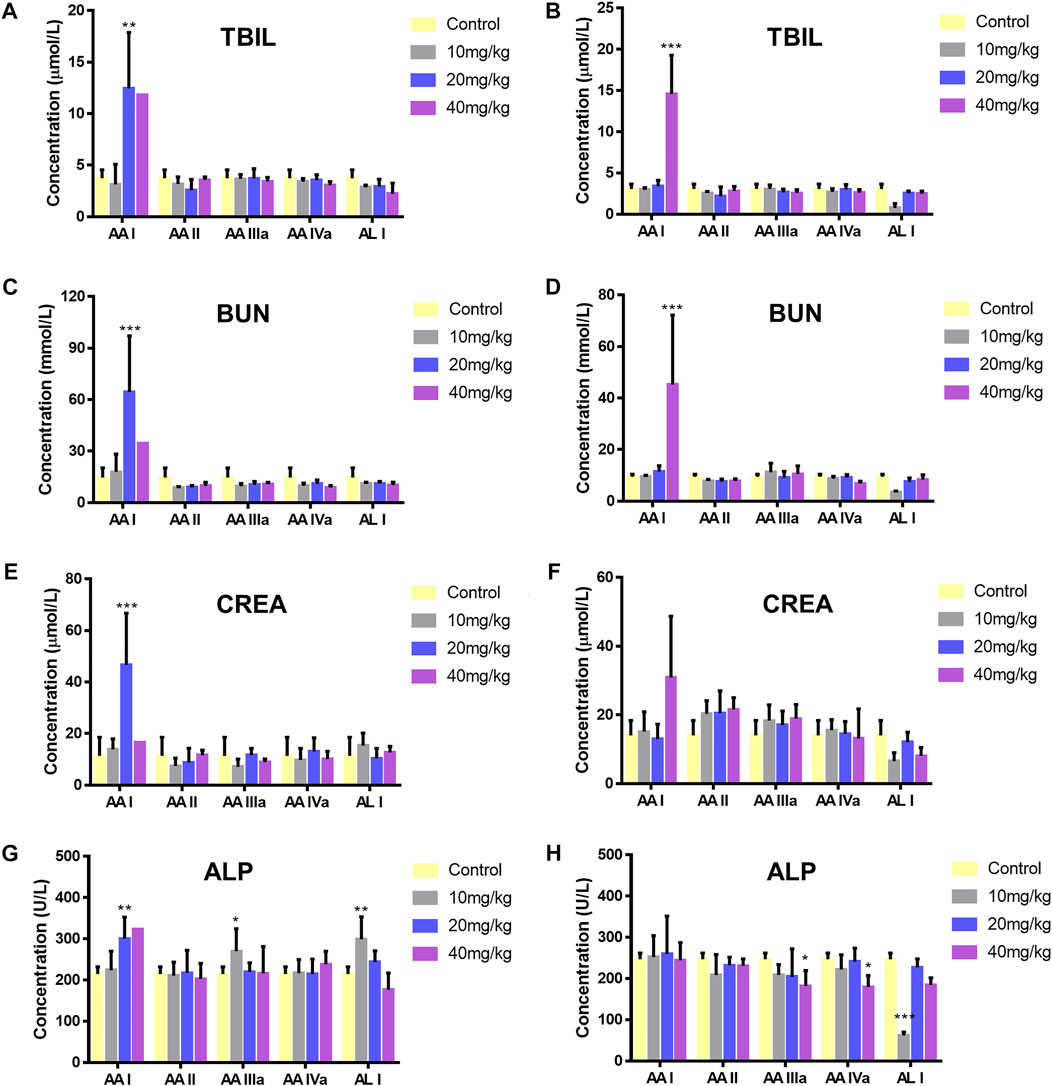
FIGURE 5. Level changes of TBIL (male, (A); female, (B)), BUN (male, (C); female, (D)), CREA (male (E); female (F)), and ALP (male (G); female (H)) of ICR mice administered with 0.5% CMC-Na, 10, 20, and 40 mg/kg aristolochic acid analogues. Significantly different from the control group (*p < 0.05; **p < 0.01, ***p < 0.001).
DNA Damages
The comet assay is a simple and rapid method for detecting DNA damage in single cells. After cell lysis and DNA unwinding, undamaged DNA will remain in the nucleus and appear round during electrophoresis; however, cells with DNA damage resemble the shape of a comet as a result of the migration of negatively charged DNA from the nucleus toward the anode. The extent of DNA migration is positively correlated with the amount of DNA damage in cells (Speit and Rothfuss, 2012). We conducted comet assays to study the DNA damage of AA analogues. Ethyl methanesulfonate (EMS) was a strong genotoxic agent (Gocke et al., 2009) and was used as a positive control in this study. Hepatocyte and renal cells in the control group appeared round during electrophoresis, while EMS-treated hepatocyte and renal cells showed significant comet tailing (Figure 6, Supplementary Figure S6). Renal cells from AA I (20 mg/kg) treated mice showed an evident comet shape. The tail DNA% was 15.39 ± 1.75 (p < 0.001) and the olive tail moment was 7.45 ± 1.94 (p < 0.05), respectively (Supplementary Table S6). However, no DNA damage was observed in hepatocyte cells of mice treated with AA I (20 mg/kg). No DNA damage was observed in renal cells nor in hepatocyte cells in mice treated with AA II, AA IIIa, AA IVa, and AL I. These results indicated that AA I could cause direct DNA damage to the renal tissues, while the ability to induce DNA damage for AA II, AA IIIa, AA IVa, and AL I was relatively low at 20 mg/kg.
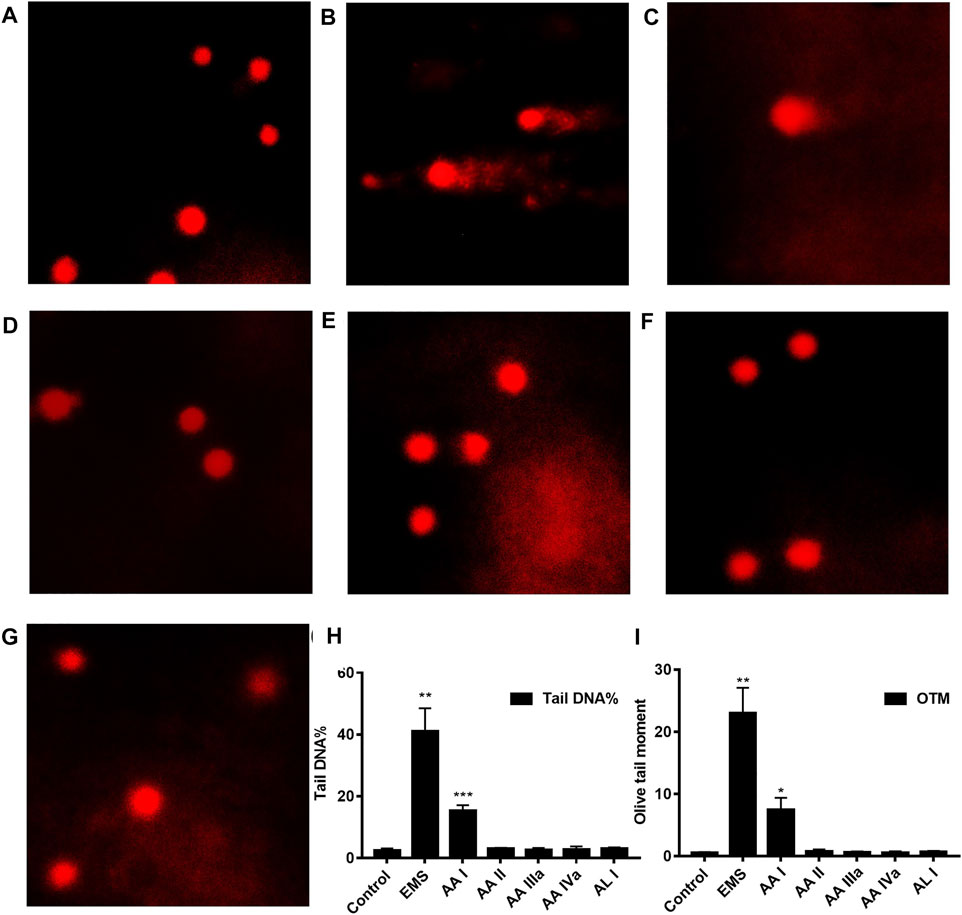
FIGURE 6. Comet assays: control (A), EMS (B), AA I (C), AA II (D), AA IIIa (E), AA IVa (F), AL I (G). The tail DNA% (H) and olive tail moment (OTM) (I) of renal cells from mice treated with AA I (20 mg/kg). Data were calculated from 100 cells and one-way ANOVA was used for statistical and significance analysis. *p < 0.05; **p < 0.01; ***p < 0.001.
Discussion
Plants of Aristolochiaceae have been gradually prohibited in the clinical use due to the nephrotoxicity and carcinogenicity of its component AA, with the exception of Asarum (Xixin), which has been used for centuries as an analgesic and antitussive (Wu et al., 2021). Asarum (Xixin) contains relatively low levels of AA I and AA II in roots and rhizomes; therefore, it is considered as a safe drug and is widely used in traditional Chinese medicines (Xie et al., 2007; Xue et al., 2008). In recent years, AA I was statistically associated with the incidence of hepatocellular carcinoma and the safety of the species of genus Aristolochia has once again aroused public concern (Hoang et al., 2013; Ng et al., 2017). Although the content of AA I in Asarum (Xixin) is required to be <0.001% according to the Chinese Pharmacopoeia 2020, the contents of AA analogues in herbs and their commercial products are still not known. In this study, the analogues of AA were quantitatively determined in 15 batches of Asarum heterotropoides F. Schmidt (Xixin) and 44 traditional Chinese patent medicines. AA I, AA II, and AA IIIa were not detected in Asarum heterotropoides F. Schmidt (Xixin) samples. Relative levels of AA IVa and AL I were found in all samples with species and regional differences, which was in consistent with previous studies (Hao et al., 2006; Zhang et al., 2008). Selecting the suitable species of Asarum heterotropoides F. Schmidt (Xixin) with less AA analogues content was an important way to improve the clinical safety of Asarum heterotropoides F. Schmidt (Xixin) and its formulated products. In this study, XX04 from Liaoning had some advantages on the low content of AA analogues. However, more samples of different species and origins need to be detected, in order to minimize the potential nephrotoxicity and hepatotoxicity.
Traditional Chinese patent medicines contain different types of medical constituents and the complex matrix brings difficulties in quantitative determination. The validation of a previous method is usually estimated based on the analysis of spiked samples of selected products in different forms and compositions, which makes the determination complicated (Vaclavik et al., 2014). In addition, the samples chosen were not representative of all samples. Herein, we established an effective and automatic solid phase extraction method for sample pretreatment, which improved the efficiency of sample processing and the accuracy of detection. In addition, selective enrichment of aristolochic acids and aristolactams can be achieved by adjusting the composition of the eluent. Aristolactams are easily eluted in neutral eluent, whereas AAs tended to be eluted in acidic mobile phase. The recovery of the method for the analogues of AA was within the range of 86.5–107.5% even at a concentration of 0.5–1.0 ng/ml. The method was successfully applied to the extraction of AA analogues and to the determination of traditional Chinese patent medicines. The AA analogues content was at ng/g in most samples. Although processing can reduce the content of Aristolochia AAs to varying degrees (Yang B. et al., 2012; Yuan et al., 2017), the contents of AA I in samples with processed Aristolochia debilis Siebold & Zucc. were still much higher than those with Asarum (Xixin). Thus, special attention should be paid to the potential nephrotoxicity or hepatotoxicity. The AA analogues varied in different dosage forms of the same prescription. Species and origins of Asarum heterotropoides F. Schmidt (Xixin) with different contents of AA analogues may be one of the reasons. The processing method also affected the content of active components, suggesting that optimizing processing may be another approach for reducing the potential toxicity.
AA I and AA II are considered as the main contents and causative agents of the nephrotoxic and carcinogenic effects of Aristolochia species (Debelle et al., 2008). However, in our study, AA IVa and AL I were found to be relatively high contents of Asarum heterotropoides F. Schmidt (Xixin) and its formulated products. Previous studies focused on the toxicity of AA I and AA II, while little is currently known about the toxicity of AA IIIa, AA IVa, and AL I, especially in terms of in vivo toxicity and carcinogenicity. To clarify whether these AA analogues contribute to the process of renal damage, both in vitro and in vivo toxicity assays were performed. Aristolochic acids I, II, IIIa, IVa, and aristolactam I showed varying degrees of cytotoxic effects to HK-2 cells, with AA-I and AL-I exhibiting the strongest cytotoxicity after 48 h of exposure. The localization of functional groups in the structure played a decisive role in cytotoxicity (Balachandran et al., 2005). AL I was the main reductive and genotoxicity metabolite of AA I. The relative cytotoxicity of AA I and AL I was contrary (Balachandran et al., 2005; Li J. et al., 2010). In this study, AA I showed higher cytotoxicity than AL I after 24 h, but the situation reversed after 48 h of incubation. This suggested there was a persistent cytotoxic effect of AL I, which was consistent with the long-term accumulation of AL I in the cytoplasm (Shang et al., 2008). Severe nephrotoxicity with large area necrosis was observed in mice treated with 40 mg/kg AA I. DNA damage was a major cause of nephrotoxicity, and this was confirmed by the comet shape of DNA in the AA I treated group. A high dosage of AA I can also induce hepatotoxicity with a significant increase in serum TBIL, BUN, and CREA levels and ALP and ALT activities. No obvious nephrotoxicity or hepatotoxicity was observed in AA II-, IIIa-, and IVa-treated mice in acute toxicity tests, which was consistent with the low cytotoxicity observed in vitro. Although the direct cytotoxicity of AL I was stronger than AA I, the induced DNA damage or nephrotoxicity of AL I was much lower than that of AA I. The weaker ability of AL I to induce mutagen, apoptosis, TGFβ1, and FN secretion may have played a role (Schmeiser et al., 1986; Li B. et al., 2004). As the metabolite of AA I and the substance that eventually binds to DNA, the toxicity of AL I, especially its long-term mutagenicity, still needs to be further studied, although no renal or DNA damage was observed in the acute toxicity test.
In conclusion, our study revealed that AA IVa and AL I were presented in relatively high levels in Asarum heterotropoides F. Schmidt (Xixin). Most traditional Chinese patent medicines in the study contained AA I, AA IVa, and AL I and the contents were at the ng/g level. Among the AA analogues studied, AA I exerted the strongest nephrotoxicity, while AA II, AA IIIa, and AA IVa showed weak toxicity both in vitro and in vivo. AL I showed significant cytotoxicity to HK-2 cells, but no renal toxicity was observed in vivo at 40 mg/kg. The contents of analogues of AA in Asarum heterotropoides F. Schmidt (Xixin) and its formulated products were far below the concentrations that induce acute nephrotoxicity; therefore, consumption of Asarum heterotropoides F. Schmidt (Xixin) with controlled doses and periods is relatively safe. However, considering the relatively high contents of AA IVa and AL I in Asarum heterotropoides F. Schmidt (Xixin), as well as the high cytotoxicity of AL I, the long-term toxicity of AA IVa and AL I should be further studied. In addition to the above-mentioned findings, the present study provides a basis for a broader quantitative determination of AA analogues and has a guiding implications for the safe clinical application of Asarum heterotropoides F. Schmidt (Xixin) and its formulated products.
Data Availability Statement
The original contributions presented in the study are included in the article/Supplementary Material; further inquiries can be directed to the corresponding author.
Ethics Statement
The animal study was reviewed and approved by the Research Ethics Committee of the Institute of Chinese Materia Medica, China Academy of Chinese Medical Sciences.
Author Contributions
SL and ZX carried out the experiments and wrote the article. LW and JT collected the herbal and TCM samples. CLi, YY, CLiu, DW, JM, SQ, and FW participated in the animal experiments. CP, JH, and YuZ revised the article. AL and YoZ designed the study.
Funding
This work was supported by the National Natural Science Foundation of China 82174073, the Fundamental Research Funds for the Central public welfare research institutes ZZ13-YQ-055, ZZ-13-035-10, ZXKT 20022, ZXKT19014. CACMS Innovation Fund CI2021A04806, CI2021A04801.
Conflict of Interest
The authors declare that the research was conducted in the absence of any commercial or financial relationships that could be construed as a potential conflict of interest.
Publisher’s Note
All claims expressed in this article are solely those of the authors and do not necessarily represent those of their affiliated organizations, or those of the publisher, the editors and the reviewers. Any product that may be evaluated in this article, or claim that may be made by its manufacturer, is not guaranteed or endorsed by the publisher.
Supplementary Material
The Supplementary Material for this article can be found online at: https://www.frontiersin.org/articles/10.3389/fphar.2021.761593/full#supplementary-material
Abbreviations
AAA, aristolochic acid analogues; AA I, aristolochic acid I; AA II, aristolochic acid II; AA IIIa, aristolochic acid IIIa; AA IVa, aristolochic acid IVa; AL I, aristolactam I; ALP, alkaline phosphatase activities; ALT, alanine amino transferase; AST, aspartate amino transferase; BEN, Balkan endemic nephropathy; BUN, blood urea nitrogen; CREA, creatinine; EMS, ethyl methanesulfonate; MRM, multiple reaction monitoring; SPE, solid phase extraction; TBA, total bile acid; TBIL, total bilirubin; UUC, upper tract urothelial carcinoma.
References
Balachandran, P., Wei, F., Lin, R. C., Khan, I. A., and Pasco, D. S. (2005). Structure Activity Relationships of Aristolochic Acid Analogues: Toxicity in Cultured Renal Epithelial Cells. Kidney. Int. 67 (5), 1797–1805. doi:10.1111/j.1523-1755.2005.00277.x
Chen, C. H., Dickman, K. G., Moriya, M., Zavadil, J., Sidorenko, V. S., Edwards, K. L., et al. (2012). Aristolochic Acid-Associated Urothelial Cancer in Taiwan. Proc. Natl. Acad. Sci. U.S.A. 109 (21), 8241–8246. doi:10.1073/pnas.1119920109
Chen, C. H., Dickman, K. G., Huang, C. Y., Moriya, M., Shun, C. T., Tai, H. C., et al. (2013). Aristolochic Acid-Induced Upper Tract Urothelial Carcinoma in Taiwan: Clinical Characteristics and Outcomes. Int. J. Cancer 133 (1), 14–20. doi:10.1002/ijc.28013
Chen, Y. J., Wang, W., and Xiao, H. B. (2020). Monitoring and Quantitative Analysis of Trace Aristolochic acid I in a Qing-Fei-Pai-Du Decoction Using Liquid Chromatography Mass Spectrometry. Acta. Pharm. Sin. 55 (8), 1903–1907. doi:10.16438/j.0513-4870.2020-0197
Debelle, F. D., Vanherweghem, J. L., and Nortier, J. L. (2008). Aristolochic Acid Nephropathy: a Worldwide Problem. Kidney Int. 74 (2), 158–169. doi:10.1038/ki.2008.129
Gocke, E., Bürgin, H., Müller, L., and Pfister, T. (2009). Literature Review on the Genotoxicity, Reproductive Toxicity, and Carcinogenicity of Ethyl Methanesulfonate. Toxicol. Lett. 190 (3), 254–265. doi:10.1016/j.toxlet.2009.03.016
Hao, X. L., Ni, Y., and Li, X. R. (2006). Determination of Aristolochic Acid in Asarum from Different Origins and Varieties by HPLC. Chin. Tradit. Pat. Med. 28 (8), 1209–1210. doi:10.3969/j.issn.1001-1528.2006.08.038
Heinrich, M., Chan, J., Wanke, S., Neinhuis, C., and Simmonds, M. S. (2009). Local Uses of Aristolochia Species and Content of Nephrotoxic Aristolochic Acid 1 and 2--a Global Assessment Based on Bibliographic Sources. J. Ethnopharmacol. 125 (1), 108–144. doi:10.1016/j.jep.2009.05.028
Hoang, M. L., Chen, C. H., Sidorenko, V. S., He, J., Dickman, K. G., Yun, B. H., et al. (2013). Mutational Signature of Aristolochic Acid Exposure as Revealed by Whole-Exome Sequencing. Sci. Transl. Med. 5 (197), 197ra102. doi:10.1126/scitranslmed.3006200
Hsieh, S. C., Lin, I. H., Tseng, W. L., Lee, C. H., and Wang, J. D. (2008). Prescription Profile of Potentially Aristolochic Acid Containing Chinese Herbal Products: an Analysis of National Health Insurance Data in Taiwan between 1997 and 2003. Chin. Med. 3, 13. doi:10.1186/1749-8546-3-13
Jelaković, B., Dika, Ž., Arlt, V. M., Stiborova, M., Pavlović, N. M., Nikolić, J., et al. (2019). Balkan Endemic Nephropathy and the Causative Role of Aristolochic Acid. Semin. Nephrol. 39 (3), 284–296. doi:10.1016/j.semnephrol.2019.02.007
Jelaković, B., Karanović, S., Vuković-Lela, I., Miller, F., Edwards, K. L., Nikolić, J., et al. (2012). Aristolactam-DNA Adducts Are a Biomarker of Environmental Exposure to Aristolochic Acid. Kidney Int. 81 (6), 559–567. doi:10.1038/ki.2011.371
Jong, T. T., Lee, M. R., Hsiao, S. S., Hsai, J. L., Wu, T. S., Chiang, S. T., et al. (2003). Analysis of Aristolochic Acid in Nine Sources of Xixin, a Traditional Chinese Medicine, by Liquid Chromatography/atmospheric Pressure Chemical Ionization/tandem Mass Spectrometry. J. Pharm. Biomed. Anal. 33 (4), 831–837. doi:10.1016/s0731-7085(03)00310-8
Kuo, P. C., Li, Y. C., and Wu, T. S. (2012). Chemical Constituents and Pharmacology of the Aristolochia ( Mădōu Ling) Species. J. Tradit Complement. Med. 2 (4), 249–266. doi:10.1016/s2225-4110(16)30111-0
Li, B., Li, X. M., Zhang, C. Y., Wang, X., and Cai, S. Q. (2004a). Injury in Renal Proximal Tubular Epithelial Cells Induced by Aristololactam I. Zhongguo Zhong Yao Za Zhi 29 (1), 78–83. doi:10.3321/j.issn:1001-5302.2004.01.023
Li, J., Zhang, L., Jiang, Z., Shu, B., Li, F., Bao, Q., et al. (2010a). Toxicities of Aristolochic Acid I and Aristololactam I in Cultured Renal Epithelial Cells. Toxicol. in Vitro 24 (4), 1092–1097. doi:10.1016/j.tiv.2010.03.012
Li, W., Gong, S., Wen, D., Che, B., Liao, Y., Liu, H., et al. (2004b). Rapid Determination of Aristolochic Acid I and II in Aristolochia Plants from Different Regions by Beta-Cyclodextrin-Modified Capillary Zone Electrophoresis. J. Chromatogr. A. 1049 (1-2), 211–217. doi:10.1016/s0021-9673(04)01336-6
Li, Y., Zhu, S., Xue, M., Jing, Y., Liu, X., Cai, D., et al. (2020). Aristolochic Acid I Promotes the Invasion and Migration of Hepatocellular Carcinoma Cells by Activating the C3a/C3aR Complement System. Toxicol. Lett. S0378-4274 (20), 30410. doi:10.1016/j.toxlet.2020.08.014
Li, Y.-L., Tian, M., Yu, J., Shang, M.-Y., and Cai, S.-Q. (2010b). Studies on morphology and aristolochic acid analogue constituents of Asarum campaniflorum and a comparison with two official species of Asari Radix et Rhizoma. J. Nat. Med. 64 (4), 442–451. doi:10.1007/s11418-010-0433-6
Li, Y. M., Li, J., and Yu, F. (2017). Current Situation and Research Progress on the Nephrotoxicity of Aristolochic Acid Compounds. J. Pharm. Res. 36 (9), 534–537. doi:10.13506/j.cnki.jpr.2017.09.011
Li, Z. H., Wei, M., Ding, G. F., and Wang, D. H. (2011). Clinical Characteristics and Long-Term Follow-Up Analysis of Three Cases with Newborn Aristolochic Acid Nephropathy. Zhonghua Er Ke Za Zhi 49 (11), 814–817. doi:10.3760/cma.j.issn.0578-1310.2011.11.005
Lu, Z. N., Luo, Q., Zhao, L. N., Shi, Y., Wang, N., Wang, L., et al. (2020). The Mutational Features of Aristolochic Acid-Induced Mouse and Human Liver Cancers. Hepatology 71 (3), 929–942. doi:10.1002/hep.30863
Mao, W. W., Gao, W., Liang, Z. T., Li, P., Zhao, Z. Z., and Li, H. J. (2017). Characterization and Quantitation of Aristolochic Acid Analogs in Different Parts of Aristolochiae Fructus, Using UHPLC-Q/TOF-MS and UHPLC-QqQ-MS. Chin. J. Nat. Med. 15 (5), 392–400. doi:10.1016/s1875-5364(17)30060-2
Michl, J., Bello, O., Kite, G. C., Simmonds, M. S. J., and Heinrich, M. (2017). Medicinally Used Asarum Species: High-Resolution LC-MS Analysis of Aristolochic Acid Analogs and In Vitro Toxicity Screening in HK-2 Cells. Front. Pharmacol. 8, 215. doi:10.3389/fphar.2017.00215
Michl, J., Ingrouille, M. J., Simmonds, M. S., and Heinrich, M. (2014). Naturally Occurring Aristolochic Acid Analogues and Their Toxicities. Nat. Prod. Rep. 31 (5), 676–693. doi:10.1039/c3np70114j
Ng, A. W. T., Poon, S. L., Huang, M. N., Lim, J. Q., Boot, A., Yu, W., et al. (2017). Aristolochic Acids and Their Derivatives Are Widely Implicated in Liver Cancers in Taiwan and throughout Asia. Sci. Transl. Med. 9 (412), 1. doi:10.1126/scitranslmed.aan6446
Schmeiser, H. H., Nortier, J. L., Singh, R., Gamboa da Costa, G., Sennesael, J., Cassuto-Viguier, E., et al. (2014). Exceptionally Long-Term Persistence of DNA Adducts Formed by Carcinogenic Aristolochic Acid I in Renal Tissue from Patients with Aristolochic Acid Nephropathy. Int. J. Cancer 135 (2), 502–507. doi:10.1002/ijc.28681
Schmeiser, H. H., Pool, B. L., and Wiessler, M. (1986). Identification and Mutagenicity of Metabolites of Aristolochic Acid Formed by Rat Liver. Carcinogenesis. 7 (1), 59–63. doi:10.1093/carcin/7.1.59
Shang, P., Wang, X., Li, X. M., Tang, J. W., and Cai, S. Q. (2008). Observation of Penetration, Distribution and Accumulation in Human Renal Proximal Tubular Epithelial Cells by Aristololactam-I. Zhongguo Zhong Yao Za Zhi 33 (7), 793–797. doi:10.3321/j.issn:1001-5302.2008.07.018
Sidorenko, V. S. (2020). Biotransformation and Toxicities of Aristolochic Acids. Adv. Exp. Med. Biol. 1241, 139–166. doi:10.1007/978-3-030-41283-8_9
Speit, G., and Rothfuss, A. (2012). The Comet Assay: a Sensitive Genotoxicity Test for the Detection of DNA Damage and Repair. Methods Mol. Biol. 920, 79–90. doi:10.1007/978-1-61779-998-3_6
Stiborová, M., Arlt, V. M., and Schmeiser, H. H. (2017). DNA Adducts Formed by Aristolochic Acid Are Unique Biomarkers of Exposure and Explain the Initiation Phase of Upper Urothelial Cancer. Int. J. Mol. Sci. 18 (10), 1. doi:10.3390/ijms18102144
Vaclavik, L., Krynitsky, A. J., and Rader, J. I. (2014). Quantification of Aristolochic Acids I and II in Herbal Dietary Supplements by Ultra-high-performance Liquid Chromatography-Multistage Fragmentation Mass Spectrometry. Food Addit. Contam. A. 31 (5), 784–791. doi:10.1080/19440049.2014.892215
Wen, H., Gao, H.-y., Qi, W., Xiao, F., Wang, L.-l., Wang, D., et al. (2014). Simultaneous Determination of Twenty-Two Components in Asari Radix et Rhizoma by Ultra Performance Liquid Chromatography Coupled with Quadrupole Time-of-Flight Mass Spectrometry. Planta Med. 80 (18), 1753–1762. doi:10.1055/s-0034-1383296
Wu, F., and Wang, T. (2013). Risk Assessment of Upper Tract Urothelial Carcinoma Related to Aristolochic Acid. Cancer Epidemiol. Biomarkers Prev. 22 (5), 812–820. doi:10.1158/1055-9965.epi-12-1386
Wu, H., Wen, X. Y., Yan, P., Shen, S. R., Liu, X. D., and Lai, K. F. (2021). Research Progress on Chemical Constituents and Pharmacological Effects of Asarum Heterotropoides F. Schmidt. Chin. J. Exp. Tradit. Med. Form 27 (4), 186–195. doi:10.13422/j.cnki.syfjx.20202353
Xie, Z. M., Li, S. X., and Liu, J. (2007). Aristolochic Acid A Content in Different Parts of Herba Asari. Chin. Tradit Pat Med. 29 (12), 1808–1810. doi:10.3969/j.issn.1001-1528.2007.12.031
Xu, Y. Q., Li, X. W., Liu, G. X., Wang, X., Shang, M. Y., Li, X. M., et al. (2013). Comparative Study of the Contents of Analogues of Aristolochic Acid in Two Kinds of Aristolochiae Fructus by High-Performance Liquid Chromatography. J. Nat. Med. 67 (1), 113–122. doi:10.1007/s11418-012-0664-9
Xue, Y., Tong, X. H., Wang, F., and Zhao, W. G. (2008). Analysis of Aristolochic Acid A from the Aerial and Underground Parts of Asarum by UPLC-UV. Yao Xue Xue Bao 43 (2), 221–223. doi:10.3321/j.issn:0513-4870.2008.02.020
Yang, B., Li, Z. H., Yang, W. L., Chen, H. F., and Yuan, J. B. (2012a). The Effect of Various Drug Processing Technologies on the Contents of Aristolochic Acid Analogues in Axistolchiae Fructus. Lishizhen Med. Mater. Med. Res. 23 (10), 2553–2555. doi:10.3969/j.issn.1008-0805.2012.10.074
Yang, H. Y., Chen, P. C., and Wang, J. D. (2014). Chinese Herbs Containing Aristolochic Acid Associated with Renal Failure and Urothelial Carcinoma: a Review from Epidemiologic Observations to Causal Inference. Biomed. Res. Int. 2014, 569325. doi:10.1155/2014/569325
Yang, L., Su, T., Li, X. M., Wang, X., Cai, S. Q., Meng, L. Q., et al. (2012b). Aristolochic Acid Nephropathy: Variation in Presentation and Prognosis. Nephrol. Dial. Transplant. 27 (1), 292–298. doi:10.1093/ndt/gfr291
Yu, J., Ma, C. M., Wang, X., Shang, M. Y., Hattori, M., Xu, F., et al. (2016). Analysis of Aristolochic Acids, Aristololactams and Their Analogues Using Liquid Chromatography Tandem Mass Spectrometry. Chin. J. Nat. Med. 14 (8), 626–640. doi:10.1016/s1875-5364(16)30074-7
Yuan, J., Ren, G., Liang, J., Wang, C. Z., Yan, Z., Huang, Q., et al. (2017). Comparative Studies on the Multi-Component Pharmacokinetics of Aristolochiae Fructus and Honey-Fried Aristolochiae Fructus Extracts after Oral Administration in Rats. BMC Complement. Altern. Med. 17 (1), 107. doi:10.1186/s12906-017-1626-2
Keywords: Asarum heterotropoides F. Schmidt, aristolochic acid analogues, content, toxicity, safety
Citation: Liu S, Xian Z, Zhao Y, Wang L, Tian J, Pan C, Han J, Zhang Y, Li C, Yi Y, Liu C, Wang D, Meng J, Qin S, Wang F and Liang A (2021) Quantitative Determination and Toxicity Evaluation of Aristolochic Acid Analogues in Asarum heterotropoides F. Schmidt (Xixin) and Traditional Chinese Patent Medicines. Front. Pharmacol. 12:761593. doi: 10.3389/fphar.2021.761593
Received: 20 August 2021; Accepted: 27 October 2021;
Published: 26 November 2021.
Edited by:
Michał Tomczyk, Medical University of Bialystok, PolandReviewed by:
James A. Fordyce, The University of Tennessee, Knoxville, United StatesChao-Zhan Lin, Guangzhou University of Chinese Medicine, China
Copyright © 2021 Liu, Xian, Zhao, Wang, Tian, Pan, Han, Zhang, Li, Yi, Liu, Wang, Meng, Qin, Wang and Liang. This is an open-access article distributed under the terms of the Creative Commons Attribution License (CC BY). The use, distribution or reproduction in other forums is permitted, provided the original author(s) and the copyright owner(s) are credited and that the original publication in this journal is cited, in accordance with accepted academic practice. No use, distribution or reproduction is permitted which does not comply with these terms.
*Correspondence: Aihua Liang, ahliang@icmm.ac.cn
†These authors have contributed equally to this work and share first authorship