- 1Division d’Hématologie-Oncologie, Centre de Recherche de l’Hôpital Maisonneuve-Rosemont, Montréal, QC, Canada
- 2Département de Microbiologie, Infectiologie et Immunologie, Université of Montréal, Montréal, QC, Canada
For several leukemia patients, allogeneic stem cell transplantation (allogeneic-SCT) is the unique therapeutic modality that could potentially cure their disease. Despite significant progress made in clinical management of allogeneic-SCT, acute graft-versus-host disease (aGVHD) and infectious complications remain the second and third cause of death after disease recurrence. Clinical options to restore immunocompetence after allogeneic-SCT are very limited as studies have raised awareness about the safety with regards to graft-versus-host disease (GVHD). Preclinical works are now focusing on strategies to improve thymic functions and to restore the peripheral niche that have been damaged by alloreactive T cells. In this mini review, we will provide a brief overview about the adverse effects of GVHD on the thymus and the peripheral niche and the resulting negative outcome on peripheral T cell homeostasis. Finally, we will discuss the potential relevance of coordinating our studies on thymic rejuvenation and improvement of the peripheral lymphoid niche to achieve optimal T cell regeneration in GVHD patients.
Key Points
• GVHD effect on the thymus.
• GVHD effect on the peripheral niche.
• Addressing the dysfunction of the thymus and the peripheral niche to improve T cell regeneration in GVHD patients.
Introduction
Allogeneic-SCT was developed to treat leukemia and lymphoma as well as congenital or acquired hematologic conditions. Despite important side effects, allogeneic-SCT remains the only curative treatment for several patients with high risk refractory hematologic cancers. Acute graft-versus-host disease (aGVHD) is a serious complication of allogeneic-SCT that occurs when donor lymphocytes react to normal host-tissues. Paradoxically, alloreactive T cells can improve survival by eliminating residual leukemia cells that survive SCT; a reaction known as graft-versus-leukemia effect (1). Following SCT, donor hematopoietic stem cells home to the bone marrow (BM) and differentiate into white cells, megakaryocytes and erythrocytes. While the recovery of innate immune cells is relatively fast, regeneration of lymphocytes is slower and can be further delayed by aGVHD (2, 3). For several years, it was believed that the failure to recover T cells post-SCT was essentially attributed to thymic dysfunction. Today, it is well known that the size of the lymphocyte pool is controlled by the thymus but also by the peripheral niche that provides resources for T cells to survive in the periphery. Recent functional studies have demonstrated a significant reduction in the bioavailability of peripheral T cell resources in graft-versus-host disease (GVHD) hosts, which contributes to the more important immunosuppression in allogeneic-SCT compared with autologous-SCT. As a result, lymphopenia is typically more severe in GVHD patients and clinical options to accelerate lymphocyte reconstitution are virtually inexistent because of the risk to aggravate aGVHD (4, 5). In this mini-review, we will address how aGVHD affects thymopoiesis and the peripheral niche, and we will propose potential strategies to improve immune reconstitution after allogeneic-SCT.
Immune Reconstitution in a Non-GVHD Setting
Lymphocyte regeneration can occur through thymopoiesis and/or via homeostatic proliferation (HP) of mature lymphocytes (2). In younger patients, thymic regeneration typically occurs during the first-year post-transplantation and is normally followed by rapid normalization of T cell counts (2). In adults, age-related thymic involution combined with therapy-induced cytotoxic insults result in prolonged thymic dysfunction. During this period, T cell regeneration occurs primarily through HP of mature lymphocytes contained in the graft (Figure 1A). In addition to interleukin-7 (IL-7), T cell receptor (TCR) stimulation by major histocompatibility complexes (MHCs) class I or II is necessary for HP of CD8+ and CD4+ lymphocytes, respectively (6). While HP is sufficient for restoring CD8 counts, it is normally insufficient for CD4+ T lymphocytes and the full recovery of the CD4 subset can take several months or years to occur and depends largely on thymic recovery (2). B cell recovery takes between 3 and 6 months to occur (7, 8), whereas DC recovery after autologous-SCT is normally fast. Given that DCs are important for NK cell homeostasis, they likely influenced NK regeneration which also occurs within few weeks post-SCT (9, 10) (Table 1).
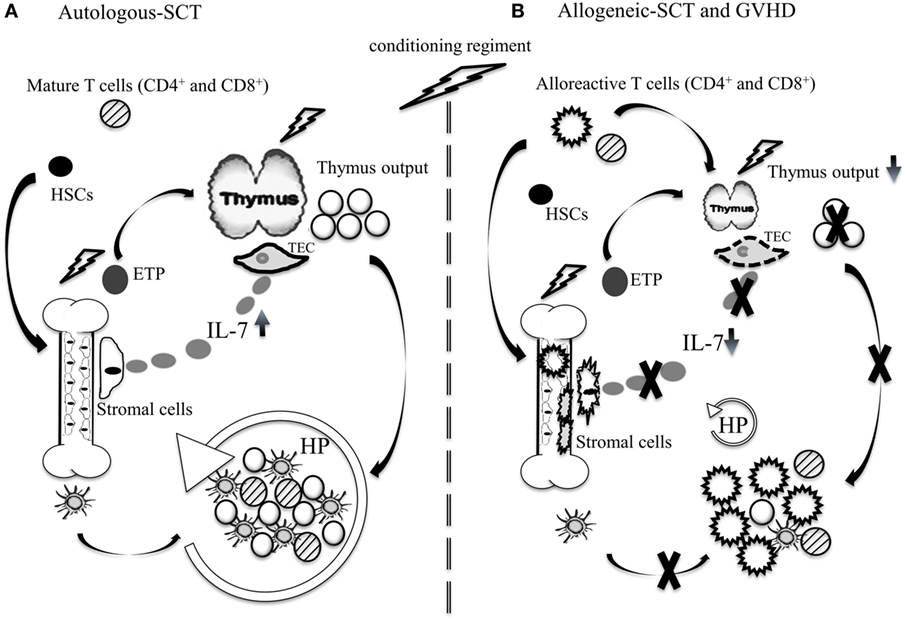
Figure 1. Immune reconstitution after autologous and allogeneic-SCT. (A) Autologous-SCT: chemotherapeutic insults affect the BM and thymopoiesis. During this period, thymopoiesis is inefficient and T cell regeneration occurs primarily through HP of mature T cells contained in the graft. The production of DCs occurs relatively early after autologous SCT and combined with elevated systemic IL-7, produced by stromal cell of primary and secondary lymphoid organs, they induce HP of mature T cells. In younger patients, rapid thymopoiesis recovery contributes to normalize CD4+ T cell counts and T cell receptor diversity. (B) Allogeneic-SCT: the combined GVHD and chemotherapeutic insults to the thymus and the BM induce long-lasting dysfunction of thymopoiesis and the peripheral lymphoid niche. Damages to the BM microenvironment are mediated primarily by alloreactive CD4+ T cells. During GVHD, DC production is reduced and systemic IL-7 is low, which constrain HP of non-alloreactive naïve T cells. Depending on the severity of GVHD and patient’s age, the dysfunction of the thymus can persist for several years.
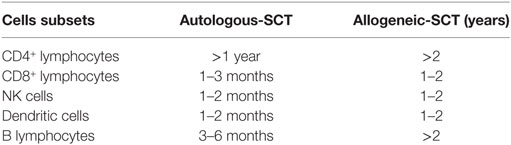
Table 1. Time line of immune reconstitution of immune cells after autologous and allogeneic-SCT (7, 11–17).
Immune Reconstitution after Allogeneic-SCT and GVHD
The immunosuppression that occurs after allogeneic-SCT is typically more important than the level of immunosuppression normally seen after autologous-SCT. Patients undergoing allogeneic-SCT experience a phase of profound lymphopenia that can last several months or years (18, 19). Depending on the severity of the aGVHD, the regeneration of both CD4+ and CD8+ lymphocytes can be further delayed. The current models put forth to explain how aGVHD affects T cell reconstitution relates to two primary factors: GVHD-mediated damage to the thymic microenvironment essential for T cell production (20); and the dysfunction of the peripheral niche essential for the survival and HP of naïve CD4+ and CD8+ T lymphocytes in the periphery (Figure 1B) (21–23). These animal studies have provided a new model to explain the profound immunosuppression typically seen in GVHD patients.
In contrast, the effect of chronic GVHD (cGVHD) on T cell regeneration is not as well understood. cGVHD occurs normally after aGVHD and during this period, T cell regeneration is already compromised. While aGVHD is mediated by mature lymphocytes contained in the graft, the origin of cGVHD appears related to leakage and release of donor-derived autoreactive lymphocytes by the thymus (Figure 2). As a result, clinical manifestations are different from aGVHD with cGVHD symptoms resembling those in patients with systemic autoimmune diseases (24).
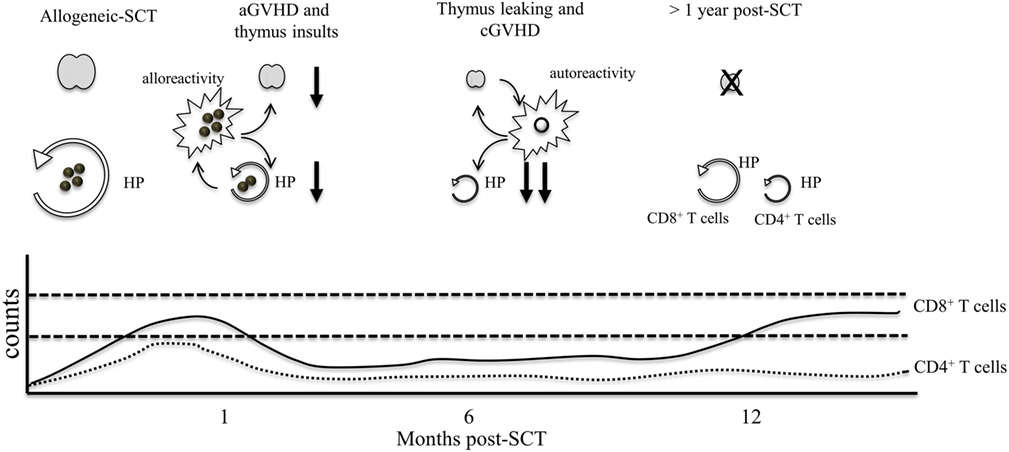
Figure 2. The effect of GVHD on lymphocyte numbers. Following chemotherapy, thymic insults induce thymic involution and loss of thymic output. Early after T cell infusion, CD4+ and CD8+ T cell counts increase as a consequence of HP and alloreactive T cell activation. At 1 month post-allogeneic-SCT, GVHD T cells induce damage to the thymus and the peripheral niche, resulting in severe thymic dysfunction and lower HP of T cells. During this period, patients are profoundly lymphopenic. After several months, the thymus may leak few autoreactive T cells, which contribute to the development of cGVHD. Autoreactive T cells can further damage the thymus and the peripheral niche, resulting in long lasting immunosuppression. At 1 year post-GVHD, rises in CD8 counts can occur through HP whereas HP of CD4+ T cells remains highly inefficient resulting in chronic CD4 lymphopenia that can persist for several years.
Immunosuppressive therapies use to control GVHD can affect lymphocyte reconstitution. Cyclosporine A and methotrexate alter B cell differentiation and thymocytes and peripheral T cell survival by interfering with TCR signaling (7, 25, 26). Novel drugs used to control refractory cGVHD are tyrosine kinase inhibitors, which can interfere with TCR or IL-7 signaling to reduce lymphocyte activation with potential detrimental effects on T cell survival (27–29). Thus, in addition to chemotherapeutic and GVHD insults that affect lymphoid organs, the prophylaxis used to control GVHD can further constrain lymphocyte regeneration after allogeneic-SCT.
GVHD Effects on the Thymus
The rich microenvironment of the thymus in MHC-I and II expression by thymic epithelial cells (TECs) and antigen-presenting cells render the thymus a significant target for alloreactive lymphocytes (30). While studies have demonstrated that the thymic recovery that occurs after autologous-SCT is associated with rapid normalization of the T cell repertoire (2), thymic rebound that occurs after allogeneic-SCT is often insufficient for restoring T cell counts and diversity, even in children (31, 32). Such differences in TCR diversity following autologous and allogeneic-SCT suggest that some elements of the thymus undergo significant damages by alloreactivity. Indeed, thymic-dependent immune reconstitution of T lymphocytes is severely impaired after allogeneic-SCT and further compromised by aGVHD (20, 33). The contribution of cGVHD T cells to thymic insults remains largely unknown since thymus atrophy is normally severe during this period. However, thymus infiltration by autoreactive T cells can occur in mice with systemic autoimmune diseases, suggesting a potential contribution of cGVHD T cells to thymic damage (34).
Evaluation of thymopoiesis can be measured by T-cell receptor excision circles (TREC) that are generated from the rearrangement of the V and J segments at the TCRα locus and thus correlate with thymic output of naïve T cells (35, 36). Depending of the robustness of the thymic output before transplantation, clinical outcomes and incidence of GVHD may vary (37). During GVHD, TREC levels are normally low and correlate with low levels of recent thymic emigrants (RTEs) detected in the blood (36, 38–41). Other factors such as age, preparative regimen, source of stem cells could also affect thymopoiesis (42, 43).
Normal thymopoiesis proceeds in a thymic microenvironment composed of a cortex and a medulla separated by the cortico–medullary junction. TECs are the major component of the thymus stroma that guide thymocyte development. Cortical thymic epithelial cells (cTECs) and medullary thymic epithelial cells (mTECs) have distinct functions and play a critical role during positive and negative selection of thymocytes to produce conventional T cells and non-conventional FOXP3+ regulatory CD4+ T cells (44–48). Following allogeneic-SCT, aGVHD induces loss of the delimitation between the cortex and the medulla (49, 50). Alloreactive lymphocytes can eliminate cTECs and mTECs, which explains the reduction of the number of mTECs expressing the transcription factor AIRE “autoimmune regulator” during GVHD (51–53). Loss of TECs is associated with diminished IL-7 production, which contributes to thymocyte death and loss of thymic output during aGVHD (54). While GVHD insults to cTECs can impair positive selection, GVHD alteration to mTECs is more insidious and could impair the negative selection of autoreactive thymocytes and contribute to cGVHD (55, 56). Furthermore, several DC subsets collaborate with mTECs to induce negative selection of self-reactive thymocytes and during GVHD, they are eliminated by alloreactive T cells, which lead to the development of cGVHD (57–60). Thus, although thymic recovery is an essential step toward normalization of T cell counts, the production of self-reactive T cells during/after aGVHD could negatively impact the benefit of thymic dependent T cell regeneration. Effective thymic recovery in a GVHD setting will need to include means to ensure adequate positive and negative selection of thymocytes to preclude the generation of potentially autoreactive lymphocytes that could exacerbate the pathology.
GVHD Effects on the Peripheral Niche
Several studies have demonstrated that GVHD induces significant damage to the peripheral niche that controls T cell homeostasis (21–23). During lymphopenia, IL-7 accumulates due to a reduced consumption by the limited number of T cells (61). In addition, T cells have increased accessibility to MHC-expressing DCs and the increase in both IL-7 and MHC accessibility promotes T lymphocyte HP (61). During GVHD, however, the lymphopenic environment is strikingly different and survival and HP of T cells are impaired (23, 62–64). For one, alloreactivity induces damages to stromal cells of primary and secondary lymphoid organs, the primary source of IL-7 (22, 65–67). In addition, DC regeneration is decreased in GVHD (23). CD4+ T cell HP depends on MHC-II presentation by DCs, such that this effect alone could explain the lack of CD4 regeneration. However, it does not necessarily explain lack of CD8+ T cell HP, as MHC-I is ubiquitously expressed, and CD8 HP is probably not exclusively dependent on MHC-I expressed by DCs. Additional studies are needed to identify elements of the peripheral niche that limit CD8 recovery in GVHD hosts.
Several studies have demonstrated that DC numbers are reduced during GVHD and depletion/inactivation of recipient DCs before allogeneic-SCT can reduce GVHD (63, 68–71). The mechanism preventing DC regeneration during GVHD is not fully understood (23, 64, 72). However, two potential mechanisms have been proposed to explain diminished DC counts in GVHD hosts: the elimination of DCs by alloreactive lymphocytes and the disruption of the BM microenvironment that constrains DC production. Indeed, T cells can eliminate DCs after priming (73–75) and following allogeneic-SCT, recipient and donor-derived DCs can be eliminated by alloreactive lymphocytes (23). However, the long-lasting DC depletion that occurs during GVHD is unlikely only due to the allo-immune response against mature DCs since the elimination of alloreactive lymphocytes post-GVHD does not restore DC counts.
Damage to the BM microenvironment can induce myelosuppression after allogeneic-SCT. BM stromal cells provide growth factors such as GM-CSF, M-CSF, and Flt3-L, which are essential for HSC differentiation into mature cells. However, little is known about how these factors are affected by GVHD in the BM. While serum analysis can provide some insight about variation of these factors, the evaluation of BM biopsy is probably more accurate since several of these factors are also produced by immune cells (76). For example, Flt3-L levels in the BM differ from those seen in the blood of GVHD mice (23). The retention/egress of BM cells is also important for the differentiation of DCs. Stromal derived factor-1 alpha (SDF-1α) regulates integrin expression on HSCs and during GVHD, SDF-1α is diminished (23). Low SDF-1α levels inside the BM likely explain the accumulation of poorly differentiated DC precursors found in the blood during GVHD (64). The low SDF-1α levels in the BM are line with the low documented B cell levels in GVHD (77). Thus, access to BM specimens is essential to define the factors that limit DC regeneration necessary for maintaining both T and B cell homeostasis.
Restoring Thymopoiesis
Thymus rejuvenation represents the best option to restore T cell repertoire diversity in transplanted patients. Today, the adoptive transfer of T cell precursors and the transplantation of thymic tissues are two interesting options to achieve this goal. The administration of T cell precursors has demonstrated its efficacy at correcting intrinsic defects to common lymphoid progenitors (CLPs) to improve thymopoiesis. However, these studies were performed in a non-GVHD setting and although CLPs are decreased during GVHD (23), it is unclear whether the administration of T cell precursors could improve thymopoiesis if the thymic epithelium has been damaged by alloreactive lymphocytes. Nonetheless, interaction between T cell precursors and TECs may help TECs to recover post-GVHD (78, 79). The administration of keratinocyte growth factor (KGF) protects the thymic epithelium from GVHD insults in mice (80). In humans, however, KGF (Palifermin) has been tested for CD4+ T cell recovery in HIV patients but the benefits were quite modest (81). Thymic restoration is currently under investigation using embryonic stem cells and promising preclinical studies in rodents have demonstrated that the expansion of cTECs and mTECs, lead to increased numbers of functional T cells in the periphery (82).
Another approach to restore thymopoiesis is the use of postnatal allogeneic cultured thymus tissue, an approach that improved thymic output in patients with DiGeorge syndrome (83, 84). These patients are profoundly immunocompromised, which allow thymus engraftment without significant graft-rejection. Although such strategy has not been tested in allogeneic-SCT patients, it was investigated in HIV patients (85). However, high rate of thymus rejection was observed. Immunosuppression appears essential for successful thymic engraftment and T cell depletion prior to thymus transplant could lower the rate of graft rejection in GVHD patients. However, it is not clear whether lower numbers of CLPs observed after aGVHD could affect thymic output. Interestingly, IL-21 can improve thymopoiesis by expanding BM Lin−Sca1+c-kit+ lymphoid progenitors after allogeneic-SCT (86). Thus, combining thymus transplantation with either IL-21 therapy or T cell precursor therapy could yield better thymic recovery following allogeneic-SCT.
Restoring the Peripheral Niche
Approaches to improve HP of mature lymphocytes after allogeneic-SCT have demonstrated that the benefit on T cell regeneration is frequently offset by excessive GVHD (4, 23, 87). IL-7 therapy has been shown to expand T cells with a predominant effect on naïve CD8+ cells (88). When administered early post-SCT, IL-7 could expand GVHD precursors and worsen aGVHD (4). At later time points, alloreactive T cells are activated and express lower IL-7Rα levels, which could explain why GVHD severity is less affected by IL-7 therapy (23, 89). Similarly, IL-15 can improve lymphocyte reconstitution after T cell depleted allogeneic-SCT (90), but it can also worsen GVHD (5, 91). DCs are the most potent cell type to initiate T cell activation and forcing their recovery following allogeneic-SCT can present significant risks. For instance, Flt3-L treatment can expand most DC subsets in mice and humans (92) and when administered after allogeneic-SCT, it worsens GVHD (23). In contrast, the expansion of DCs by SDF-1α therapy is largely restricted to the DC1 subset and resulted in a decrease in GVHD severity (23). Host DC1 have been shown to protect against aGVHD (93, 94). DC1 cells have an intrinsic ability to cross present antigens on MHC-I molecules and they are poor activators of CD4+ T cells (95, 96), a property that is likely important for preventing unwanted T cell activation during homeostatic stimulation of naïve lymphocytes. However, there are several different subsets of DC with distinct functions, and it is not clear which subset specifically controls CD4 homeostasis, if any (97). Thus, depending of the cocktail of cytokines used to improve T and DC regeneration, caution is needed as the risk to aggravate GVHD may surpass the benefit on T cell regeneration.
Mesenchymal stem cells (MSCs) can be administered in patients to diminish GVHD (98, 99). MSCs have immunosuppressive properties on T cells and on innate immune cells (100–102). MSCs produce a vast array of cytokines and growth factors known to affect hematopoiesis (103–107). While IL-7 production by MSCs may help to improve T cell survival, the secretion of other factors like SDF-1α could promote DC regeneration (108). The infusion of HLA mismatch MSCs was first reported to reduce alloreactivity in patients with steroid-refractory aGVHD (109). However, studies that follow showed a benefit highly variable and often transient with response rate higher in patients with mild GVHD (Grade-II) (110–112). MSC therapy can effectively induce complete response in 72% of patients (Grade II) with partial response for patients with higher grade GVHD (99, 113). Importantly, the use of MSCs generated from pooled BM mononuclear cells obtained from multiple allogeneic donors showed surprising efficacy in patients with grade III-IV refractory GVHD (114, 115). Thus, the use of multiple donors to generate MSCs could perhaps reduce the variability inherent to individual donor. The development of effective strategies to lower GVHD severity is clearly an important milestone in our attempt to restore T cell homeostasis. Such a reduction could diminish GVHD insults to the thymus and the peripheral niche, facilitating our future immune interventions to accelerate lymphocyte reconstitution in patients.
Restoring T Cell Homeostasis after GVHD
In most clinical settings of human lymphopenia, the dysfunction of the thymus represents the primary limiting factor for T cell regeneration. However, the dysfunction of the peripheral niche is now well documented and added to the dysfunction of the thymus and together they contribute to the very poor immune reconstitution that typically occurs in GVHD patients. In a setting where the peripheral niche remains dysfunctional, it is not clear whether restoring thymopoiesis would be sufficient to increase peripheral T cell counts. DCs and IL-7 provide critical survival signals to RTEs for their expansion in the periphery. Depending of the robustness of the thymic output, T cell immune reconstitution may vary considerably when the peripheral niche is dysfunctional. The absence of peripheral DCs could result into greater competition between T cells for access to MHC class I–II, which could affect the number and the diversity of the T cell repertoire. Under these conditions, the repertoire may be skewed toward lymphocytes with the highest levels of self-reactivity. In addition, the absence of peripheral DCs could impede self-tolerance imposed by thymopoiesis since the peripheral DCs pick up peripheral self-antigens, migrate to the thymus and induce negative selection of developing self-reactive thymocytes (116, 117). The failure to present self-antigens through MHC-II has been shown to induce severe autoimmunity in mice transplanted with MHC-II−/− BM cells (118). As a result, the feasibility and the safety of restoring thymopoiesis in GVHD patients remain largely unknown. Animal models used to study T cell homeostasis have not yet addressed the capacity of the thymus to restore T cell counts in the absence of a functional peripheral niche. The use of thymic transplantation in GVHD hosts could provide significant insights about the potential benefits and limitations of restoring thymopoiesis in GVHD patients.
Conclusion and Perspectives
Despite significant progress in our understanding of the biology of T cell depletion, therapies aimed at accelerating T cell regeneration remain limited and are still in clinical development (119, 120). The effect of GVHD on T cell homeostasis is multiple and addressing the dysfunction of the thymus or the peripheral niche alone may yield disappointing results in patients undergoing allogeneic-SCT. Under these circumstances, the coordinate use of IL-7, SDF-1α, T cell precursors, embryonic stem cells, tissues engineering to recreate an artificial thymus and thymus transplantation could provide superior benefits with regards to T cell regeneration in GVHD patients. In parallel, we need to address the recovery of other immune cell subsets such as B- and/or NK cells, which also provide immunocompetence to transplanted patients. Collaboration between clinicians and scientists will be instrumental to the successful development of new animal models of thymic rejuvenation in GVHD hosts to address the dysfunction of peripheral niche in order to guide our future immune interventions to accelerate and restore the T cell compartment in humans.
Author Contributions
All authors contributed intellectually to the writing of this work.
Conflict of Interest Statement
The authors declare no commercial or financial relationships that could constitute a conflict of interest.
Funding
This work was supported by grants from the Cancer Research Society of Canada (grants no. 16255 and no. 22669 to MG and no. 21006 to SL) and in part by the Foundation de l’Hôpital Maisonneuve-Rosemont, Montreal, QC, CAN.
References
1. Horowitz MM, Gale RP, Sondel PM, Goldman JM, Kersey J, Kolb HJ, et al. Graft-versus-leukemia reactions after bone marrow transplantation. Blood (1990) 75(3):555–62.
2. Mackall CL, Fleisher TA, Brown MR, Andrich MP, Chen CC, Feuerstein IM, et al. Age, thymopoiesis, and CD4+ T-lymphocyte regeneration after intensive chemotherapy. N Engl J Med (1995) 332(3):143–9. doi:10.1056/NEJM199501193320303
3. Fry TJ, Mackall CL. Immune reconstitution following hematopoietic progenitor cell transplantation: challenges for the future. Bone Marrow Transplant (2005) 35(Suppl 1):S53–7. doi:10.1038/sj.bmt.1704848
4. Sinha ML, Fry TJ, Fowler DH, Miller G, Mackall CL. Interleukin 7 worsens graft-versus-host disease. Blood (2002) 100(7):2642–9. doi:10.1182/blood-2002-04-1082
5. Blaser BW, Roychowdhury S, Kim DJ, Schwind NR, Bhatt D, Yuan W, et al. Donor-derived IL-15 is critical for acute allogeneic graft-versus-host disease. Blood (2005) 105(2):894–901. doi:10.1182/blood-2004-05-1687
6. Guimond M, Fry TJ, Mackall CL. Cytokine signals in T-cell homeostasis. J Immunother (2005) 28(4):289–94. doi:10.1097/01.cji.0000165356.03924.e7
7. Storek J, Wells D, Dawson MA, Storer B, Maloney DG. Factors influencing B lymphopoiesis after allogeneic hematopoietic cell transplantation. Blood (2001) 98(2):489–91. doi:10.1182/blood.V98.2.489
8. Simonelli C, Zanussi S, Pratesi C, Rupolo M, Talamini R, Caffau C, et al. Immune recovery after autologous stem cell transplantation is not different for HIV-infected versus HIV-uninfected patients with relapsed or refractory lymphoma. Clin Infect Dis (2010) 50(12):1672–9. doi:10.1086/652866
9. Guimond M, Freud AG, Mao HC, Yu J, Blaser BW, Leong JW, et al. In vivo role of Flt3 ligand and dendritic cells in NK cell homeostasis. J Immunol (2010) 184(6):2769–75. doi:10.4049/jimmunol.0900685
10. Dean R, Masci P, Pohlman B, Andresen S, Serafino S, Sobecks R, et al. Dendritic cells in autologous hematopoietic stem cell transplantation for diffuse large B-cell lymphoma: graft content and post transplant recovery predict survival. Bone Marrow Transplant (2005) 36(12):1049–52. doi:10.1038/sj.bmt.1705183
11. Storek J, Witherspoon RP, Storb R. T cell reconstitution after bone marrow transplantation into adult patients does not resemble T cell development in early life. Bone Marrow Transplant (1995) 16(3):413–25.
12. Mackall CL, Fleisher TA, Brown MR, Andrich MP, Chen CC, Feuerstein IM, et al. Distinctions between CD8+ and CD4+ T-cell regenerative pathways result in prolonged T-cell subset imbalance after intensive chemotherapy. Blood (1997) 89(10):3700–7.
13. Steingrimsdottir H, Gruber A, Bjorkholm M, Svensson A, Hansson M. Immune reconstitution after autologous hematopoietic stem cell transplantation in relation to underlying disease, type of high-dose therapy and infectious complications. Haematologica (2000) 85(8):832–8.
14. Storek J, Dawson MA, Storer B, Stevens-Ayers T, Maloney DG, Marr KA, et al. Immune reconstitution after allogeneic marrow transplantation compared with blood stem cell transplantation. Blood (2001) 97(11):3380–9. doi:10.1182/blood.V97.11.3380
15. Williams KM, Gress RE. Immune reconstitution and implications for immunotherapy following haematopoietic stem cell transplantation. Best Pract Res Clin Haematol (2008) 21(3):579–96. doi:10.1016/j.beha.2008.06.003
16. Storek J, Geddes M, Khan F, Huard B, Helg C, Chalandon Y, et al. Reconstitution of the immune system after hematopoietic stem cell transplantation in humans. Semin Immunopathol (2008) 30(4):425–37. doi:10.1007/s00281-008-0132-5
17. de Koning C, Plantinga M, Besseling P, Boelens JJ, Nierkens S. Immune reconstitution after allogeneic hematopoietic cell transplantation in children. Biol Blood Marrow Transplant (2016) 22(2):195–206. doi:10.1016/j.bbmt.2015.08.028
18. Denham S, Attridge SR, Barfoot RK. The effect of limited courses of cyclosporine on survival and immunocompetence of allogeneic bone marrow chimeras. Transplantation (1985) 40(5):477–82. doi:10.1097/00007890-198511000-00002
19. Einsele H, Ehninger G, Steidle M, Fischer I, Bihler S, Gerneth F, et al. Lymphocytopenia as an unfavorable prognostic factor in patients with cytomegalovirus infection after bone marrow transplantation. Blood (1993) 82(5):1672–8.
20. Muller-Hermelink HK, Sale GE, Borisch B, Storb R. Pathology of the thymus after allogeneic bone marrow transplantation in man. A histologic immunohistochemical study of 36 patients. Am J Pathol (1987) 129(2):242–56.
21. Dulude G, Roy DC, Perreault C. The effect of graft-versus-host disease on T cell production and homeostasis. J Exp Med (1999) 189(8):1329–42. doi:10.1084/jem.189.8.1329
22. Shono Y, Ueha S, Wang Y, Abe J, Kurachi M, Matsuno Y, et al. Bone marrow graft-versus-host disease: early destruction of hematopoietic niche after MHC-mismatched hematopoietic stem cell transplantation. Blood (2010) 115(26):5401–11. doi:10.1182/blood-2009-11-253559
23. Gauthier SD, Leboeuf D, Manuguerra-Gagne R, Gaboury L, Guimond M. Stromal-derived factor-1alpha and interleukin-7 treatment improves homeostatic proliferation of naive CD4(+) T cells after allogeneic stem cell transplantation. Biol Blood Marrow Transplant (2015) 21(10):1721–31. doi:10.1016/j.bbmt.2015.06.019
24. Martin PJ. Biology of chronic graft-versus-host disease: implications for a future therapeutic approach. Keio J Med (2008) 57(4):177–83. doi:10.2302/kjm.57.177
25. Hannam-Harris AC, Taylor DS, Nowell PC. Cyclosporin A directly inhibits human B-cell proliferation by more than a single mechanism. J Leukoc Biol (1985) 38(2):231–9. doi:10.1002/jlb.38.2.231
26. Gratama JW, Wursch AM, Nissen C, Gratwohl A, D’Amaro J, de Gast GC, et al. Influence of graft-versus-host disease prophylaxis on early T-lymphocyte regeneration following allogeneic bone marrow transplantation. Br J Haematol (1986) 62(2):355–65.
27. Chen GL, Arai S, Flowers ME, Otani JM, Qiu J, Cheng EC, et al. A phase 1 study of imatinib for corticosteroid-dependent/refractory chronic graft-versus-host disease: response does not correlate with anti-PDGFRA antibodies. Blood (2011) 118(15):4070–8. doi:10.1182/blood-2011-03-341693
28. Legros L, Ebran N, Stebe E, Rousselot P, Rea D, Cassuto JP, et al. Imatinib sensitizes T-cell lymphocytes from chronic myeloid leukemia patients to FasL-induced cell death: a brief communication. J Immunother (2012) 35(2):154–8. doi:10.1097/CJI.0b013e318243f238
29. Thiant S, Moutuou MM, Laflamme P, Sidi Boumedine R, Leboeuf DM, Busque L, et al. Imatinib mesylate inhibits STAT5 phosphorylation in response to IL-7 and promotes T cell lymphopenia in chronic myelogenous leukemia patients. Blood Cancer J (2017) 7(4):e551. doi:10.1038/bcj.2017.29
30. Fukushi N, Arase H, Wang B, Ogasawara K, Gotohda T, Good RA, et al. Thymus: a direct target tissue in graft-versus-host reaction after allogeneic bone marrow transplantation that results in abrogation of induction of self-tolerance. Proc Natl Acad Sci U S A (1990) 87(16):6301–5. doi:10.1073/pnas.87.16.6301
31. Olkinuora H, Talvensaari K, Kaartinen T, Siitonen S, Saarinen-Pihkala U, Partanen J, et al. T cell regeneration in pediatric allogeneic stem cell transplantation. Bone Marrow Transplant (2007) 39(3):149–56. doi:10.1038/sj.bmt.1705557
32. Merindol N, Champagne MA, Duval M, Soudeyns H. CD8(+) T-cell reconstitution in recipients of umbilical cord blood transplantation and characteristics associated with leukemic relapse. Blood (2011) 118(16):4480–8. doi:10.1182/blood-2011-04-349241
33. Lum LG. The kinetics of immune reconstitution after human marrow transplantation. Blood (1987) 69(2):369–80.
34. Ashman RF, Singh N, Lenert PS. Abnormal thymic maturation and lymphoproliferation in MRL-Fas (lpr/lpr) mice can be partially reversed by synthetic oligonucleotides: implications for systemic lupus erythematosus and autoimmune lymphoproliferative syndrome. Lupus (2017) 26(7):734–45. doi:10.1177/0961203316676381
35. Douek DC, Betts MR, Hill BJ, Little SJ, Lempicki R, Metcalf JA, et al. Evidence for increased T cell turnover and decreased thymic output in HIV infection. J Immunol (2001) 167(11):6663–8. doi:10.4049/jimmunol.167.11.6663
36. Douek DC, Koup RA, McFarland RD, Sullivan JL, Luzuriaga K. Effect of HIV on thymic function before and after antiretroviral therapy in children. J Infect Dis (2000) 181(4):1479–82. doi:10.1086/315398
37. Saglio F, Cena S, Berger M, Quarello P, Boccasavia V, Ferrando F, et al. Association between thymic function and allogeneic hematopoietic stem cell transplantation outcome: results of a pediatric study. Biol Blood Marrow Transplant (2015) 21(6):1099–105. doi:10.1016/j.bbmt.2015.02.010
38. Hazenberg MD, Otto SA, de Pauw ES, Roelofs H, Fibbe WE, Hamann D, et al. T-cell receptor excision circle and T-cell dynamics after allogeneic stem cell transplantation are related to clinical events. Blood (2002) 99(9):3449–53. doi:10.1182/blood.V99.9.3449
39. Krenger W, Schmidlin H, Cavadini G, Hollander GA. On the relevance of TCR rearrangement circles as molecular markers for thymic output during experimental graft-versus-host disease. J Immunol (2004) 172(12):7359–67. doi:10.4049/jimmunol.172.12.7359
40. Liu C, He M, Rooney B, Kepler TB, Chao NJ. Longitudinal analysis of T-cell receptor variable beta chain repertoire in patients with acute graft-versus-host disease after allogeneic stem cell transplantation. Biol Blood Marrow Transplant (2006) 12(3):335–45. doi:10.1016/j.bbmt.2005.09.019
41. Przybylski GK, Kreuzer KA, Siegert W, Schmidt CA. No recovery of T-cell receptor excision circles (TRECs) after non-myeloablative allogeneic hematopoietic stem cell transplantation is correlated with the onset of GvHD. J Appl Genet (2007) 48(4):397–404. doi:10.1007/BF03195239
42. Hakim FT, Cepeda R, Kaimei S, Mackall CL, McAtee N, Zujewski J, et al. Constraints on CD4 recovery postchemotherapy in adults: thymic insufficiency and apoptotic decline of expanded peripheral CD4 cells. Blood (1997) 90(9):3789–98.
43. Hakim FT, Memon SA, Cepeda R, Jones EC, Chow CK, Kasten-Sportes C, et al. Age-dependent incidence, time course, and consequences of thymic renewal in adults. J Clin Invest (2005) 115(4):930–9. doi:10.1172/JCI200522492
44. Anderson G, Owen JJ, Moore NC, Jenkinson EJ. Thymic epithelial cells provide unique signals for positive selection of CD4+CD8+ thymocytes in vitro. J Exp Med (1994) 179(6):2027–31. doi:10.1084/jem.179.6.2027
45. Surh CD, Sprent J. T-cell apoptosis detected in situ during positive and negative selection in the thymus. Nature (1994) 372(6501):100–3. doi:10.1038/372100a0
46. Liston A, Lesage S, Wilson J, Peltonen L, Goodnow CC. Aire regulates negative selection of organ-specific T cells. Nat Immunol (2003) 4(4):350–4. doi:10.1038/ni906
47. Nakagawa Y, Ohigashi I, Nitta T, Sakata M, Tanaka K, Murata S, et al. Thymic nurse cells provide microenvironment for secondary T cell receptor alpha rearrangement in cortical thymocytes. Proc Natl Acad Sci U S A (2012) 109(50):20572–7. doi:10.1073/pnas.1213069109
48. Daley SR, Hu DY, Goodnow CC. Helios marks strongly autoreactive CD4+ T cells in two major waves of thymic deletion distinguished by induction of PD-1 or NF-kappaB. J Exp Med (2013) 210(2):269–85. doi:10.1084/jem.20121458
49. Seemayer TA, Lapp WS, Bolande RP. Thymic epithelial injury in graft-versus-host reactions following adrenalectomy. Am J Pathol (1978) 93(2):325–38.
50. Ghayur T, Seemayer TA, Xenocostas A, Lapp WS. Complete sequential regeneration of graft-vs.-host-induced severely dysplastic thymuses. Implications for the pathogenesis of chronic graft-vs.-host disease. Am J Pathol (1988) 133(1):39–46.
51. Gardner JM, Fletcher AL, Anderson MS, Turley SJ. AIRE in the thymus and beyond. Curr Opin Immunol (2009) 21(6):582–9. doi:10.1016/j.coi.2009.08.007
52. Dertschnig S, Hauri-Hohl MM, Vollmer M, Hollander GA, Krenger W. Impaired thymic expression of tissue-restricted antigens licenses the de novo generation of autoreactive CD4+ T cells in acute GVHD. Blood (2015) 125(17):2720–3. doi:10.1182/blood-2014-08-597245
53. Hassan MN, Waller EK. GVHD clears the Aire in thymic selection. Blood (2015) 125(17):2593–5. doi:10.1182/blood-2015-03-630871
54. Hauri-Hohl MM, Keller MP, Gill J, Hafen K, Pachlatko E, Boulay T, et al. Donor T-cell alloreactivity against host thymic epithelium limits T-cell development after bone marrow transplantation. Blood (2007) 109(9):4080–8. doi:10.1182/blood-2006-07-034157
55. Desbarats J, Lapp WS. Thymic selection and thymic major histocompatibility complex class II expression are abnormal in mice undergoing graft-versus-host reactions. J Exp Med (1993) 178(3):805–14. doi:10.1084/jem.178.3.805
56. Wu T, Young JS, Johnston H, Ni X, Deng R, Racine J, et al. Thymic damage, impaired negative selection, and development of chronic graft-versus-host disease caused by donor CD4+ and CD8+ T cells. J Immunol (2013) 191(1):488–99. doi:10.4049/jimmunol.1300657
57. Hollander GA, Widmer B, Burakoff SJ. Loss of normal thymic repertoire selection and persistence of autoreactive T cells in graft vs host disease. J Immunol (1994) 152(4):1609–17.
58. van den Brink MR, Moore E, Ferrara JL, Burakoff SJ. Graft-versus-host-disease-associated thymic damage results in the appearance of T cell clones with anti-host reactivity. Transplantation (2000) 69(3):446–9. doi:10.1097/00007890-200002150-00026
59. Zhang Y, Hexner E, Frank D, Emerson SG. CD4+ T cells generated de novo from donor hemopoietic stem cells mediate the evolution from acute to chronic graft-versus-host disease. J Immunol (2007) 179(5):3305–14. doi:10.4049/jimmunol.179.5.3305
60. Sakoda Y, Hashimoto D, Asakura S, Takeuchi K, Harada M, Tanimoto M, et al. Donor-derived thymic-dependent T cells cause chronic graft-versus-host disease. Blood (2007) 109(4):1756–64. doi:10.1182/blood-2006-08-042853
61. Guimond M, Veenstra RG, Grindler DJ, Zhang H, Cui Y, Murphy RD, et al. Interleukin 7 signaling in dendritic cells regulates the homeostatic proliferation and niche size of CD4+ T cells. Nat Immunol (2009) 10(2):149–57. doi:10.1038/ni.1695
62. Takebayashi M, Amakawa R, Tajima K, Miyaji M, Nakamura K, Ito T, et al. Blood dendritic cells are decreased in acute graft-versus-host disease. Bone Marrow Transplant (2004) 33(10):989–96. doi:10.1038/sj.bmt.1704406
63. Giraud S, Dhedin N, Gary-Gouy H, Lebon P, Vernant JP, Dalloul A. Plasmacytoid dendritic cell reconstitution following bone marrow transplantation: subnormal recovery and functional deficit of IFN-alpha/beta production in response to herpes simplex virus. J Interferon Cytokine Res (2005) 25(3):135–43. doi:10.1089/jir.2005.25.135
64. Banovic T, Markey KA, Kuns RD, Olver SD, Raffelt NC, Don AL, et al. Graft-versus-host disease prevents the maturation of plasmacytoid dendritic cells. J Immunol (2009) 182(2):912–20. doi:10.4049/jimmunol.182.2.912
65. Horny HP, Horst HA, Ehninger G, Kaiserling E. Lymph node morphology after allogeneic bone marrow transplantation for chronic myeloid leukemia: a histological and immunohistological study focusing on the phenotype of the recovering lymphoid cells. Blut (1988) 57(1):31–40. doi:10.1007/BF00320632
66. Chewning JH, Zhang W, Randolph DA, Swindle CS, Schoeb TR, Weaver CT. Allogeneic Th1 cells home to host bone marrow and spleen and mediate IFNgamma-dependent aplasia. Biol Blood Marrow Transplant (2013) 19(6):876–87. doi:10.1016/j.bbmt.2013.03.007
67. Shono Y, Shiratori S, Kosugi-Kanaya M, Ueha S, Sugita J, Shigematsu A, et al. Bone marrow graft-versus-host disease: evaluation of its clinical impact on disrupted hematopoiesis after allogeneic hematopoietic stem cell transplantation. Biol Blood Marrow Transplant (2014) 20(4):495–500. doi:10.1016/j.bbmt.2013.12.568
68. Aractingi S, Gluckman E, Dauge-Geffroy MC, Le Goue C, Flahaut A, Dubertret L, et al. Langerhans’ cells are depleted in chronic graft versus host disease. J Clin Pathol (1997) 50(4):305–9. doi:10.1136/jcp.50.4.305
69. Chan GW, Gorgun G, Miller KB, Foss FM. Persistence of host dendritic cells after transplantation is associated with graft-versus-host disease. Biol Blood Marrow Transplant (2003) 9(3):170–6. doi:10.1016/S1083-8791(03)70006-8
70. Arpinati M, Chirumbolo G, Urbini B, Bonifazi F, Bandini G, Saunthararajah Y, et al. Acute graft-versus-host disease and steroid treatment impair CD11c+ and CD123+ dendritic cell reconstitution after allogeneic peripheral blood stem cell transplantation. Biol Blood Marrow Transplant (2004) 10(2):106–15. doi:10.1016/j.bbmt.2003.09.005
71. Vakkila J, Thomson AW, Hovi L, Vettenranta K, Saarinen-Pihkala UM. Circulating dendritic cell subset levels after allogeneic stem cell transplantation in children correlate with time post transplant and severity of acute graft-versus-host disease. Bone Marrow Transplant (2005) 35(5):501–7. doi:10.1038/sj.bmt.1704827
72. Leveque-El Mouttie L, Koyama M, Le Texier L, Markey KA, Cheong M, Kuns RD, et al. Corruption of dendritic cell antigen presentation during acute GVHD leads to regulatory T-cell failure and chronic GVHD. Blood (2016) 128(6):794–804. doi:10.1182/blood-2015-11-680876
73. Loyer V, Fontaine P, Pion S, Hetu F, Roy DC, Perreault C. The in vivo fate of APCs displaying minor H antigen and/or MHC differences is regulated by CTLs specific for immunodominant class I-associated epitopes. J Immunol (1999) 163(12):6462–7.
74. Hermans IF, Ritchie DS, Yang J, Roberts JM, Ronchese F. CD8+ T cell-dependent elimination of dendritic cells in vivo limits the induction of antitumor immunity. J Immunol (2000) 164(6):3095–101. doi:10.4049/jimmunol.164.6.3095
75. Yang J, Huck SP, McHugh RS, Hermans IF, Ronchese F. Perforin-dependent elimination of dendritic cells regulates the expansion of antigen-specific CD8+ T cells in vivo. Proc Natl Acad Sci U S A (2006) 103(1):147–52. doi:10.1073/pnas.0509054103
76. Chklovskaia E, Nissen C, Landmann L, Rahner C, Pfister O, Wodnar-Filipowicz A. Cell-surface trafficking and release of flt3 ligand from T lymphocytes is induced by common cytokine receptor gamma-chain signaling and inhibited by cyclosporin A. Blood (2001) 97(4):1027–34. doi:10.1182/blood.V97.4.1027
77. Shimabukuro-Vornhagen A, Hallek MJ, Storb RF, von Bergwelt-Baildon MS. The role of B cells in the pathogenesis of graft-versus-host disease. Blood (2009) 114(24):4919–27. doi:10.1182/blood-2008-10-161638
78. Awong G, Singh J, Mohtashami M, Malm M, La Motte-Mohs RN, Benveniste PM, et al. Human proT-cells generated in vitro facilitate hematopoietic stem cell-derived T-lymphopoiesis in vivo and restore thymic architecture. Blood (2013) 122(26):4210–9. doi:10.1182/blood-2012-12-472803
79. Smith MJ, Reichenbach DK, Parker SL, Riddle MJ, Mitchell J, Osum KC, et al. T cell progenitor therapy-facilitated thymopoiesis depends upon thymic input and continued thymic microenvironment interaction. JCI Insight (2017) 2(10):1–14. doi:10.1172/jci.insight.92056
80. Rossi S, Blazar BR, Farrell CL, Danilenko DM, Lacey DL, Weinberg KI, et al. Keratinocyte growth factor preserves normal thymopoiesis and thymic microenvironment during experimental graft-versus-host disease. Blood (2002) 100(2):682–91. doi:10.1182/blood.V100.2.682
81. Jacobson JM, Wang H, Bordi R, Zheng L, Gross BH, Landay AL, et al. A randomized controlled trial of palifermin (recombinant human keratinocyte growth factor) for the treatment of inadequate CD4+ T-lymphocyte recovery in patients with HIV-1 infection on antiretroviral therapy. J Acquir Immune Defic Syndr (2014) 66(4):399–406. doi:10.1097/QAI.0000000000000195
82. Hu R, Liu Y, Su M, Song Y, Rood D, Lai L. Transplantation of donor-origin mouse embryonic stem cell-derived thymic epithelial progenitors prevents the development of chronic graft-versus-host disease in mice. Stem Cells Transl Med (2017) 6(1):121–30. doi:10.5966/sctm.2016-0012
83. Markert ML, Boeck A, Hale LP, Kloster AL, McLaughlin TM, Batchvarova MN, et al. Transplantation of thymus tissue in complete DiGeorge syndrome. N Engl J Med (1999) 341(16):1180–9. doi:10.1056/NEJM199910143411603
84. Markert ML, Devlin BH, McCarthy EA. Thymus transplantation. Clin Immunol (2010) 135(2):236–46. doi:10.1016/j.clim.2010.02.007
85. Markert ML, Hicks CB, Bartlett JA, Harmon JL, Hale LP, Greenberg ML, et al. Effect of highly active antiretroviral therapy and thymic transplantation on immunoreconstitution in HIV infection. AIDS Res Hum Retroviruses (2000) 16(5):403–13. doi:10.1089/088922200309061
86. Tormo A, Khodayarian F, Cui Y, Al-Chami E, Kanjarawi R, Noe B, et al. Interleukin-21 promotes thymopoiesis recovery following hematopoietic stem cell transplantation. J Hematol Oncol (2017) 10(1):120. doi:10.1186/s13045-017-0490-3
87. Blazar BR, McKenna HJ, Panoskaltsis-Mortari A, Taylor PA. Flt3 ligand (FL) treatment of murine donors does not modify graft-versus-host disease (GVHD) but FL treatment of recipients post-bone marrow transplantation accelerates GVHD lethality. Biol Blood Marrow Transplant (2001) 7(4):197–207. doi:10.1053/bbmt.2001.v7.pm11349806
88. Hennion-Tscheltzoff O, Leboeuf D, Gauthier SD, Dupuis M, Assouline B, Gregoire A, et al. TCR triggering modulates the responsiveness and homeostatic proliferation of CD4+ thymic emigrants to IL-7 therapy. Blood (2013) 121(23):4684–93. doi:10.1182/blood-2012-09-458174
89. Alpdogan O, Muriglan SJ, Eng JM, Willis LM, Greenberg AS, Kappel BJ, et al. IL-7 enhances peripheral T cell reconstitution after allogeneic hematopoietic stem cell transplantation. J Clin Invest (2003) 112(7):1095–107. doi:10.1172/JCI200317865
90. Sauter CT, Bailey CP, Panis MM, Biswas CS, Budak-Alpdogan T, Durham A, et al. Interleukin-15 administration increases graft-versus-tumor activity in recipients of haploidentical hematopoietic SCT. Bone Marrow Transplant (2013) 48(9):1237–42. doi:10.1038/bmt.2013.47
91. Thiant S, Moutuou MM, Leboeuf D, Guimond M. Homeostatic cytokines in immune reconstitution and graft-versus-host disease. Cytokine (2016) 82:24–32. doi:10.1016/j.cyto.2016.01.003
92. Maraskovsky E, Brasel K, Teepe M, Roux ER, Lyman SD, Shortman K, et al. Dramatic increase in the numbers of functionally mature dendritic cells in Flt3 ligand-treated mice: multiple dendritic cell subpopulations identified. J Exp Med (1996) 184(5):1953–62. doi:10.1084/jem.184.5.1953
93. Weber M, Rudolph B, Stein P, Yogev N, Bosmann M, Schild H, et al. Host-derived CD8(+) dendritic cells protect against acute graft-versus-host disease after experimental allogeneic bone marrow transplantation. Biol Blood Marrow Transplant (2014) 20(11):1696–704. doi:10.1016/j.bbmt.2014.08.005
94. Markey K, Kuns RD, Browne DJ, Gartlan KH, Robb RJ, Martins JP, et al. Flt-3L expansion of recipient CD8alpha+ dendritic cells deletes alloreactive donor T cells and represents an alternative to post-transplant cyclophosphamide for the prevention of GVHD. Clin Cancer Res (2018) 24(7):1604–16. doi:10.1158/1078-0432.CCR-17-2148
95. den Haan JM, Lehar SM, Bevan MJ. CD8(+) but not CD8(-) dendritic cells cross-prime cytotoxic T cells in vivo. J Exp Med (2000) 192(12):1685–96. doi:10.1084/jem.192.12.1685
96. Kronin V, Fitzmaurice CJ, Caminschi I, Shortman K, Jackson DC, Brown LE. Differential effect of CD8(+) and CD8(-) dendritic cells in the stimulation of secondary CD4(+) T cells. Int Immunol (2001) 13(4):465–73. doi:10.1093/intimm/13.4.465
97. Audiger C, Rahman MJ, Yun TJ, Tarbell KV, Lesage S. The importance of dendritic cells in maintaining immune tolerance. J Immunol (2017) 198(6):2223–31. doi:10.4049/jimmunol.1601629
98. Kurtzberg J, Prockop S, Teira P, Bittencourt H, Lewis V, Chan KW, et al. Allogeneic human mesenchymal stem cell therapy (remestemcel-L, Prochymal) as a rescue agent for severe refractory acute graft-versus-host disease in pediatric patients. Biol Blood Marrow Transplant (2014) 20(2):229–35. doi:10.1016/j.bbmt.2013.11.001
99. Introna M, Rambaldi A. Mesenchymal stromal cells for prevention and treatment of graft-versus-host disease: successes and hurdles. Curr Opin Organ Transplant (2015) 20(1):72–8. doi:10.1097/MOT.0000000000000158
100. Spaggiari GM, Capobianco A, Becchetti S, Mingari MC, Moretta L. Mesenchymal stem cell-natural killer cell interactions: evidence that activated NK cells are capable of killing MSCs, whereas MSCs can inhibit IL-2-induced NK-cell proliferation. Blood (2006) 107(4):1484–90. doi:10.1182/blood-2005-07-2775
101. Nemeth K, Leelahavanichkul A, Yuen PS, Mayer B, Parmelee A, Doi K, et al. Bone marrow stromal cells attenuate sepsis via prostaglandin E(2)-dependent reprogramming of host macrophages to increase their interleukin-10 production. Nat Med (2009) 15(1):42–9. doi:10.1038/nm.1905
102. Bouchlaka MN, Moffitt AB, Kim J, Kink JA, Bloom DD, Love C, et al. Human mesenchymal stem cell-educated macrophages are a distinct high IL-6-producing subset that confer protection in graft-versus-host-disease and radiation injury models. Biol Blood Marrow Transplant (2017) 23(6):897–905. doi:10.1016/j.bbmt.2017.02.018
103. Haynesworth SE, Baber MA, Caplan AI. Cytokine expression by human marrow-derived mesenchymal progenitor cells in vitro: effects of dexamethasone and IL-1 alpha. J Cell Physiol (1996) 166(3):585–92. doi:10.1002/(SICI)1097-4652(199603)166:3<585::AID-JCP13>3.0.CO;2-6
104. Van Overstraeten-Schlogel N, Beguin Y, Gothot A. Role of stromal-derived factor-1 in the hematopoietic-supporting activity of human mesenchymal stem cells. Eur J Haematol (2006) 76(6):488–93. doi:10.1111/j.1600-0609.2006.00633.x
105. Wagner W, Saffrich R, Ho AD. The stromal activity of mesenchymal stromal cells. Transfus Med Hemother (2008) 35(3):185–93. doi:10.1159/000128956
106. Li T, Wu Y. Paracrine molecules of mesenchymal stem cells for hematopoietic stem cell niche. Bone Marrow Res (2011) 2011:353878. doi:10.1155/2011/353878
107. Zhao K, Liu Q. The clinical application of mesenchymal stromal cells in hematopoietic stem cell transplantation. J Hematol Oncol (2016) 9(1):46. doi:10.1186/s13045-016-0276-z
108. Fujii S, Miura Y, Fujishiro A, Shindo T, Shimazu Y, Hirai H, et al. Graft-versus-host disease amelioration by human bone marrow mesenchymal stromal/stem cell-derived extracellular vesicles is associated with peripheral preservation of naive T cell populations. Stem Cells (2018) 36(3):434–45. doi:10.1002/stem.2759
109. Le Blanc K, Rasmusson I, Sundberg B, Gotherstrom C, Hassan M, Uzunel M, et al. Treatment of severe acute graft-versus-host disease with third party haploidentical mesenchymal stem cells. Lancet (2004) 363(9419):1439–41. doi:10.1016/S0140-6736(04)16104-7
110. Bernardo ME, Ball LM, Cometa AM, Roelofs H, Zecca M, Avanzini MA, et al. Co-infusion of ex vivo-expanded, parental MSCs prevents life-threatening acute GVHD, but does not reduce the risk of graft failure in pediatric patients undergoing allogeneic umbilical cord blood transplantation. Bone Marrow Transplant (2011) 46(2):200–7. doi:10.1038/bmt.2010.87
111. Chen X, Wang C, Yin J, Xu J, Wei J, Zhang Y. Efficacy of mesenchymal stem cell therapy for steroid-refractory acute graft-versus-host disease following allogeneic hematopoietic stem cell transplantation: a systematic review and meta-analysis. PLoS One (2015) 10(8):e0136991. doi:10.1371/journal.pone.0136991
112. Dotoli GM, De Santis GC, Orellana MD, de Lima Prata K, Caruso SR, Fernandes TR, et al. Mesenchymal stromal cell infusion to treat steroid-refractory acute GvHD III/IV after hematopoietic stem cell transplantation. Bone Marrow Transplant (2017) 52(6):859–62. doi:10.1038/bmt.2017.35
113. Introna M, Lucchini G, Dander E, Galimberti S, Rovelli A, Balduzzi A, et al. Treatment of graft versus host disease with mesenchymal stromal cells: a phase I study on 40 adult and pediatric patients. Biol Blood Marrow Transplant (2014) 20(3):375–81. doi:10.1016/j.bbmt.2013.11.033
114. Kuci Z, Bonig H, Kreyenberg H, Bunos M, Jauch A, Janssen JW, et al. Mesenchymal stromal cells from pooled mononuclear cells of multiple bone marrow donors as rescue therapy in pediatric severe steroid-refractory graft-versus-host disease: a multicenter survey. Haematologica (2016) 101(8):985–94. doi:10.3324/haematol.2015.140368
115. Bader P, Kuci Z, Bakhtiar S, Basu O, Bug G, Dennis M, et al. Effective treatment of steroid and therapy-refractory acute graft-versus-host disease with a novel mesenchymal stromal cell product (MSC-FFM). Bone Marrow Transplant (2018). doi:10.1038/s41409-018-0102-z
116. Goldschneider I, Cone RE. A central role for peripheral dendritic cells in the induction of acquired thymic tolerance. Trends Immunol (2003) 24(2):77–81. doi:10.1016/S1471-4906(02)00038-8
117. Li J, Park J, Foss D, Goldschneider I. Thymus-homing peripheral dendritic cells constitute two of the three major subsets of dendritic cells in the steady-state thymus. J Exp Med (2009) 206(3):607–22. doi:10.1084/jem.20082232
118. Teshima T, Reddy P, Liu C, Williams D, Cooke KR, Ferrara JL. Impaired thymic negative selection causes autoimmune graft-versus-host disease. Blood (2003) 102(2):429–35. doi:10.1182/blood-2003-01-0266
119. Rosenberg SA, Sportes C, Ahmadzadeh M, Fry TJ, Ngo LT, Schwarz SL, et al. IL-7 administration to humans leads to expansion of CD8+ and CD4+ cells but a relative decrease of CD4+ T-regulatory cells. J Immunother (2006) 29(3):313–9. doi:10.1097/01.cji.0000210386.55951.c2
Keywords: interleukin-7, dendritic cells, lymphopenia, lymphocytes, stem cell transplantation, GVHD, IL-7, SDF-1α
Citation: Moutuou MM, Pagé G, Zaid I, Lesage S and Guimond M (2018) Restoring T Cell Homeostasis After Allogeneic Stem Cell Transplantation; Principal Limitations and Future Challenges. Front. Immunol. 9:1237. doi: 10.3389/fimmu.2018.01237
Received: 02 March 2018; Accepted: 17 May 2018;
Published: 18 June 2018
Edited by:
Hans-Jochem Kolb, Kolb Consulting UG, GermanyReviewed by:
Benedetto Bruno, Università degli Studi di Torino, ItalyEvelyn Ullrich, Goethe-Universität Frankfurt am Main, Germany
Copyright: © 2018 Moutuou, Pagé, Zaid, Lesage and Guimond. This is an open-access article distributed under the terms of the Creative Commons Attribution License (CC BY). The use, distribution or reproduction in other forums is permitted, provided the original author(s) and the copyright owner are credited and that the original publication in this journal is cited, in accordance with accepted academic practice. No use, distribution or reproduction is permitted which does not comply with these terms.
*Correspondence: Martin Guimond, martin.guimond@umontreal.ca