Introduction
Peanut (Arachis hypogaea L.) is an important oilseed and cash crop in the United States. The United States is the fourth largest producer of peanut in the world with a market value of more than $1 billion (USDA-NASS 2022). Peanut production in the United States increased from 2,800 kg ha−1 in the 1970s to 4,600 kg ha−1 in 2021 (USDA-NASS 2022) due to improved cultivars and cultural practices, more effective pest management, and increased per capita consumption (Holbrook Reference Holbrook2019). Although more-effective herbicides are available, weed interference remains a major constraint for peanut production in the United States despite continuous research efforts in weed science. Season-long weed interference can cause 60% to 80% peanut yield reduction through competition for light, water, and nutrients and decreased harvest efficiency (Everman et al. Reference Everman, Burke, Clewis, Thomas and Wilcut2008a, Reference Everman, Clewis, Thomas, Burke and Wilcut2008b).
Peanut has characteristics that make weed control challenging compared with other row crops such as cotton (Gossypium hirsutum L.), corn (Zea mays L.), or soybean [Glycine max (L.) Merr.]. It has a low canopy, allowing weeds to be more competitive for light, particularly during the early stage of crop growth (Wilcut et al. Reference Wilcut, York, Grichar and Wehtje1995). Peanut also requires a long growing season (140 to 160 d) for development and maturity (Chaudhari et al. Reference Chaudhari, Jordan, Grey, Prostko and Jennings2018), and residual herbicides registered for use in peanut do not provide season-long weed control, leading to heavy late-season weed infestation (Grichar Reference Grichar2007). The prostrate growth habit of peanut, with its stems growing parallel to the soil, restricts cultivation to the early season (Boyer et al. Reference Boyer, Ferrell, MacDonald, Tillman and Rowland2011). Cultivation can also introduce wounds to the plant tissue, which can increase access for pathogens and disease incidence (Wilcut et al. Reference Wilcut, York, Grichar and Wehtje1995). Weed control could be accomplished by hand weeding, but this is expensive, time-consuming, laborious, and impractical under modern-day circumstances (Johnson et al. Reference Johnson, Boudreau and Davis2012a, Reference Johnson, Boudreau and Davis2012b). Consequently, weed management in peanut is overwhelmingly achieved with herbicides, and research in the United States has focused primarily on chemical weed control. Although herbicides are not a complete solution to the complex weed management challenges in peanut production, they are effective and have contributed significantly to increased peanut yields (Gianessi and Reigner Reference Gianessi and Reigner2007).
Numerous studies have evaluated various herbicides for weed control in peanut in the United States. However, a systematic review of existing literature on this subject is lacking. The biology and management of weeds in peanut, including chemical weed control, was reviewed by Wilcut et al. (Reference Wilcut, York, Grichar and Wehtje1995), but this review was published almost three decades ago. Since that publication, several herbicides such as acetochlor, carfentrazone, diclosulam, dimethenamid-P, flumioxazin, fluridone, fluazifop-P, imazapic, lactofen, pyroxasulfone, and S-metolachlor have been registered in peanut (Anonymous 2017, 2023; Prostko et al. Reference Prostko, Grey, Webster and Kemerait2011). Considerable progress has been made in developing herbicides to increase the number of tools available for weed control in peanut, including the recent registration of one new herbicide mode of action (Group 12, fluridone) (Anonymous 2023).
No single herbicide application can provide sufficient weed control in all situations due to a narrow window of application, low residual activity, variability in weed control, and rotational restrictions. Therefore, effective weed control in peanut is generally obtained by using herbicide programs that consist of herbicide mixtures and sequential applications of preplant-incorporated or preemergence, early-postemergence, and/or late-postemergence herbicides (Chaudhari et al. Reference Chaudhari, Jordan, Grey, Prostko and Jennings2018). Growers use a broad combination of preplant-incorporated, preemergence, and postemergence herbicides based on the weed community composition, dominant weed species, rotational restrictions, environmental conditions, and economic benefits (Leon et al. Reference Leon, Jordan, Bolfrey-Arku, Dzomeku, Korres, Burgos and Duke2019).
In addition to the earlier review by Wilcut et al. (Reference Wilcut, York, Grichar and Wehtje1995), Leon et al. (Reference Leon, Jordan, Bolfrey-Arku, Dzomeku, Korres, Burgos and Duke2019) provided an overview of sustainable weed management in peanut, but focused particularly on weed prevention, avoidance, monitoring, and suppression as parts of successful integrated weed management in peanut. The current paper presents a systematic review of weed management research in peanut in the United States in the last five decades, specifically focusing on chemical weed management methods. This review aims to compile existing literature and access the research progress and achievements in peanut chemical weed management, highlight the strengths and weaknesses of various herbicides used in peanut to assist in augmenting herbicide recommendations for research and extension, and identify current research gaps and prospects for future research.
Systematic Literature Search
The literature search was done using a four-step filtering process.
Step 1
The databases of Scopus, Web of Science, and Peanut Science (journal of the American Peanut Research and Education Society) covering 53 yr (from 1970 to July 2022, accessed July 12, 2022) were searched using predefined search terms (Table 1). Peanut Science was included because it is currently not indexed in Scopus or Web of Science but publishes peer-reviewed results of peanut research.
Table 1. Search terms and exclusion criteria used to identify relevant articles in the databases of Scopus, Web of Science, and Peanut Science (accessed: July 12, 2022).
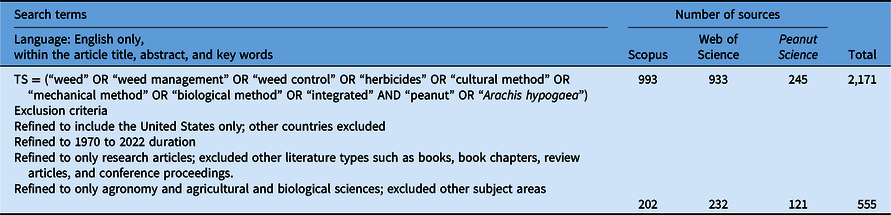
Step 2
The total record (2,171 peer-reviewed articles) from the three databases was screened to identify each article’s relevance for the review by refining the search terms based on exclusion criteria (Table 1). This resulted in a refined cohort of 555 peer-reviewed publications.
Step 3
The refined cohort of 555 peer-reviewed publications from the three databases was exported and combined in Excel, with the year of publication as rows and contents (journal, research focus, weeds studied, herbicides tested, study type [field, greenhouse, or laboratory], study location, number of site-years, research methods, and abstract) as columns.
Step 4
Duplicates (78 peer-reviewed publications) were removed, and the remaining publications (477) were further screened by two independent researchers for their relevance by reviewing the titles and abstracts. This resulted in 317 unique and relevant publications that were subsequently reviewed. Of the 317 publications reviewed, 245 (77%) focused on chemical weed management, while the remaining 72 (23%) focused on nonchemical weed management. Only the 245 peer-reviewed publications focused on chemical weed management are discussed in the current paper. To help the readers determine specific herbicide/weed efficacy data and associated publication, tables for each herbicide MOA and application method have been constructed (Tables 2–10). Discussions on nonchemical weed management are covered in the first part of this publication series (Daramola et al. Reference Daramola, Iboyi, MacDonald, Kanissery, Singh, Tillman and Devkota2023a).
Chemical Weed Control in Peanut in the United States
Peanut can tolerate herbicides from various modes of action, including acetyl-CoA carboxylase (ACCase), acetolactate synthase (ALS), carotenoid biosynthesis, fatty-acid and lipid biosynthesis, mitosis, phytoene desaturase (fluridone), photosystem I (PSI) electron diverter, and protoporphyrinogen oxidase (PPO) inhibitors, and the synthetic auxin 2,4-DB, allowing selective weed control with these herbicides in peanut (Leon et al. Reference Leon, Jordan, Bolfrey-Arku, Dzomeku, Korres, Burgos and Duke2019). However, peanut is susceptible to herbicides such as photosystem II (PSII) inhibitors (e.g., metribuzin, atrazine), enolpyruvyl shikimate3-phosphate synthase (EPSPS) inhibitors (e.g., glyphosate), and glutamine synthase inhibitors (e.g., glufosinate), which are commonly used in crops such as cotton, corn, and soybean in rotation with peanut (Daramola et al. Reference Daramola, Kharel, Iboyi and Devkota2023b, Reference Daramola, Iboyi, MacDonald, Kanissery, Singh, Tillman and Devkota2023c; Leon et al. Reference Leon, Jordan, Bolfrey-Arku, Dzomeku, Korres, Burgos and Duke2019).
Research progress and developments in chemical weed control over the years have led to significant changes in the herbicide options available for weed control in peanut. Wilcut et al. (Reference Wilcut, York, Grichar and Wehtje1995) presented a historical perspective describing the herbicide changes that occurred between the late 1940s and early 1990s along with a list of herbicide registrations and cancellations. Except for an overview, we will not duplicate this information. Rather, we will discuss new developments in peanut weed control since that publication. Alachlor, benefin, chloramben, dinoseb, metolachlor, naptalam, naptalam plus dinoseb, pendimethalin, trifluralin, and vernolate are among the first group of herbicides evaluated for efficacy and peanut tolerance between the late 1940s and early 1980s (Wilcut et al. Reference Wilcut, York, Grichar and Wehtje1995). During this period, preplant-incorporated applications of the dinitroaniline herbicides benefin, pendimethalin, and trifluralin (used only in Texas and Oklahoma and not in the U.S. Southeast region) or preemergence applications of the chloroacetamide herbicides alachlor and metolachlor were used to control annual grasses and small-seeded broadleaf weeds. Vernolate applied preplant incorporated provided effective control of yellow (Cyperus esculentus L.) and purple (Cyperus rotundus L.) nutsedge (Buchanan et al. Reference Buchanan, Murray and Hauser1982; Wilcut et al. Reference Wilcut, York, Grichar and Wehtje1995), while broadleaf weeds were controlled with the postemergence herbicides acifluorfen, bentazon, chloramben, dinoseb, dinoseb plus naptalam, and 2,4-DB (Wilcut et al. Reference Wilcut, York, Grichar and Wehtje1995). Dinoseb was identified as one of the most promising postemergence herbicides in peanut in early research and was used extensively for selective weed control, particularly for troublesome weeds species such as Florida beggarweed [Desmodium tortuosum (Sw.) DC.] and sicklepod [Senna obtusifolia (L.) Irwin & Barneby] until 1986, when dinoseb registration was cancelled due to toxicity issues (Wilcut et al. Reference Wilcut, York, Grichar and Wehtje1995). Similarly, toxicity or injury concerns have eliminated alachlor, benefin, chloramben, naptalam, and vernolate from U.S. peanut production. The removal of these herbicides encouraged the development and registration of safer herbicides such as bentazon, chlorimuron, ethalfluralin, fenoxaprop, imazethapyr, norflurazon, paraquat, pyridate, and sethoxydim between the late 1980s and early 1990s (Wilcut et al. Reference Wilcut, York, Grichar and Wehtje1995). Reviews on the use of these herbicides in peanut can be found elsewhere (Buchanan et al. Reference Buchanan, Murray and Hauser1982; Wilcut et al. Reference Wilcut, York, Grichar and Wehtje1995). The past two decades have also witnessed significant progress in chemical weed control in peanut with registration of more herbicides such as acetochlor, carfentrazone, diclosulam, dimethenamid-P, fluazifop-P, fluridone, flumioxazin, imazapic, lactofen, pyroxasulfone, and S-metolachlor (Table 2). However, there are still gaps and limitations in chemical weed control in peanut with the currently registered herbicides.
Table 2. Mode of action (MOA), chemical family, application timings, and application rates of herbicides labeled for use in peanut since 1995.
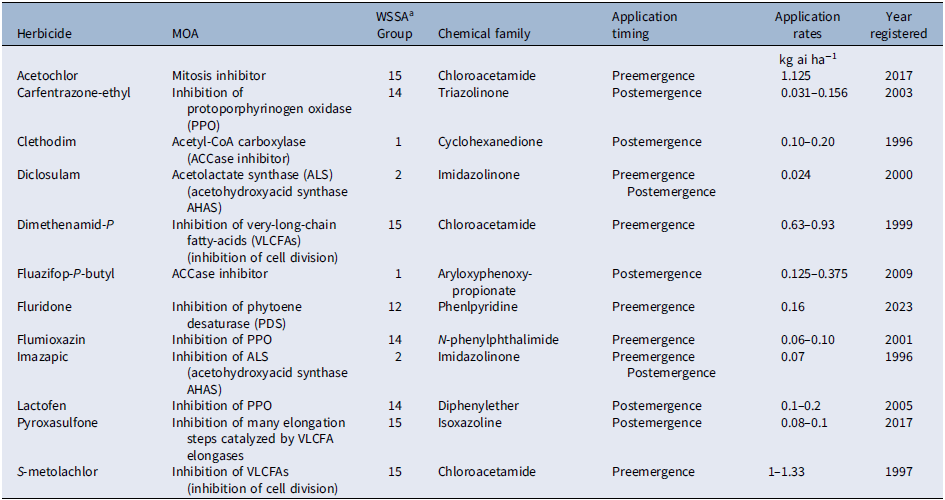
a Weed Science Society of America, https://wssa.net/wssa/weed/herbicides.
Soil-applied Herbicides
Soil-applied herbicides are used to provide residual weed control and prevent weed establishment. Residual weed control in peanut is achieved mainly with very-long-chain fatty-acid (VLCFA) inhibitors from the chloroacetamide chemical family (e.g., acetochlor, dimethenamid-P, pyroxasulfone, and S-metolachlor), mitosis inhibitors from the dinitroaniline chemical family (e.g., ethalfluralin and pendimethalin), and less frequently with the carotenoid inhibitor norflurazon from the pyridazinone chemical family. The use of the PPO inhibitor flumioxazin from the N-phenylphthalimide chemical family and ALS inhibitors from the triazolopyrimidine (e.g., diclosulam) and imidazolinone (e.g., imazapic, imazethapyr) chemical families for residual weed control have increased considerably. Likewise, the phytoene desaturase inhibitor fluridone has recently been registered for preemergence application in peanut (Anonymous 2017).
Chloroacetamides (Acetochlor, Dimethenamid-P, Pyroxasulfone, and S-metolachlor)
The chloroacetamides acetochlor, dimethenamid-P, pyroxasulfone, and S-metolachlor are soil-applied herbicides that inhibit long-chain fatty-acid biosynthesis (Shaner Reference Shaner2014). They are applied preplant incorporated, preemergence, or in conjunction with postemergence herbicides in peanut to control annual grasses and small-seeded broadleaf weeds and provide suppression of nutsedge (Cyperus spp.) and large-seeded broadleaf weeds such as bristly starbur (Acanthospermum hispidum DC.), common ragweed (Ambrosia artemisiifolia L.), D. tortuosum, and S. obtusifolia (Basinger et al. Reference Basinger, Randell and Prostko2021; Clewis et al. Reference Clewis, Everman, Jordan and Wilcut2007; Grichar et al. Reference Grichar, Lemon, Sestak and Brewer2000; Robinson et al. Reference Robinson, Clewis and Wilcut2006; Wehtje and Brecke Reference Wehtje and Brecke2004). Incorporating these herbicides into the soil ensures activity in situations where preemergence applications may fail from the absence of irrigation or inadequate rainfall; however, compared with activated preemergence applications, control or suppression of some weeds is lower (Grichar et al. Reference Grichar, Lemon, Sestak and Brewer2000). The microencapsulated formulation of acetochlor registered for use in peanut provides longer residual activity and higher crop safety than emulsifiable concentrate formulations (Anonymous 2010; Grichar et al. Reference Grichar, Dotray and Etheredge2015). In general, most preemergence herbicide applications, such as S-metolachlor and acetochlor applied preemergence, in peanut require adequate rainfall or irrigation for activation and optimum efficacy. S-metolachlor (12:80 mixture of R-inactive and S-active stereoisomers formulation) was registered in 1997 and provides weed control efficacy similar to metolachlor (50:50 mixture of R and S stereoisomers formulation) in peanut (Grichar et al. Reference Grichar, Lemon, Brewer and Minton2001, Reference Grichar, Dotray and Baughman2008). It is applied at lower rates (1.1 to 1.4 kg ha−1) due to a greater concentration of S-stereoisomers in the formulation (Anonymous 2004; O’Connell et al. Reference O’Connell, Harms and Allen1998).
Our systematic review of the literature showed that acetochlor and S-metolachlor provide >70% control of barnyardgrass [Echinochloa crus-galli (L.) P. Beauv.], hemp sesbania [Sesbania herbacea (Mill.) McVaugh; syn.: Sesbania exaltata (Raf.) Rydb. ex A.W. Hill], horse purslane (Trianthema portulacastrum L.), Palmer amaranth (Amaranthus palmeri S. Watson), pitted morningglory (Ipomoea lacunosa L.), and prickly sida (Sida spinosa L.), but control of several other annual grasses and small-seeded broadleaf weeds with acetochlor and S-metolachlor is often <70% (Tables 3 and 4). Peanut growers generally do not observe >70% control of I. lacunosa and S. exaltata with S-metolachlor; however, Chaudhari et al. (Reference Chaudhari, Jordan, Grey, Prostko and Jennings2018) and Seale at al. (Reference Seale, Bararpour, Bond, Gore and Golden2020) observed >70% control, probably due to low weed pressure in the soil seedbank or because control was evaluated very early in the season (19 to 21 d after planting). Dimethenamid-P controls some weed species with efficacy similar to acetochlor and S-metolachlor; however, dimethenamid-P is less effective on Ipomoea spp., S. spinosa, and C. esculentus (Burke et al. Reference Burke, Askew and Wilcut2002; Clewis et al. Reference Clewis, Askew and Wilcut2002; Robinson et al. Reference Robinson, Clewis and Wilcut2006). The available literature showed that dimethenamid-P provides poor (0% to 67%) control of entireleaf morningglory (Ipomoea hederacea var. integriuscula A. Gray), ivyleaf morningglory [Ipomoea hederacea (L.) Jacq.], I. lacunosa, and S. spinosa in peanut (Tables 3 and 4). Although acetochlor, dimethenamid-P, and S-metolachlor provide good control of most annual grasses, they have limited activity on Texas panicum [Urochloa texana (Buckley) R. Webster; syn.: Panicum texanum Buckley], which can be problematic in peanut (Clewis et al. Reference Clewis, Everman, Jordan and Wilcut2007; Grichar et al. Reference Grichar, Colburn and Kearney1994; Johnson et al. Reference Johnson, Prostko and Mullinix2002). Both acetochlor and S-metolachlor applied preemergence provided <70% control of U. texana in irrigated strip-tillage peanut (Grichar et al. Reference Grichar, Colburn and Kearney1994; Johnson et al. Reference Johnson, Prostko and Mullinix2002). Similarly, dimethenamid-P applied preemergence did not control U. texana compared with a nontreated control in strip-tillage peanut despite optimum activation with irrigation (Johnson et al. Reference Johnson, Prostko and Mullinix2002).
Table 3. Cumulative results on efficacy of very-long-chain fatty-acid inhibitors applied preemergence in peanut.
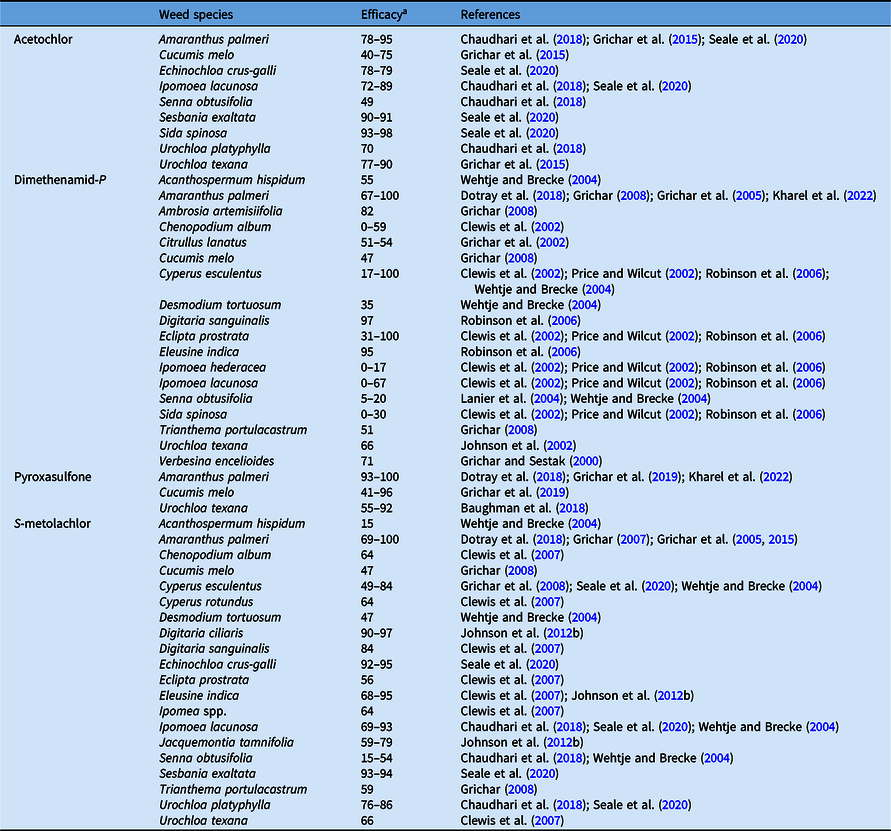
a Efficacy range across all references listed from 0% to 100%, where 0 = no control, 100 = complete control.
Table 4. Cumulative results on efficacy of acetolactate synthase (diclosulam and imazethapyr), seedling root growth (ethalfluralin and pendimethalin), very-long-chain fatty-acid (dimethenamid-P, pyroxasulfone, and S-metolachlor), and protoporphyrinogen oxidase (sulfentrazone) inhibitors applied preplant incorporated in peanut.
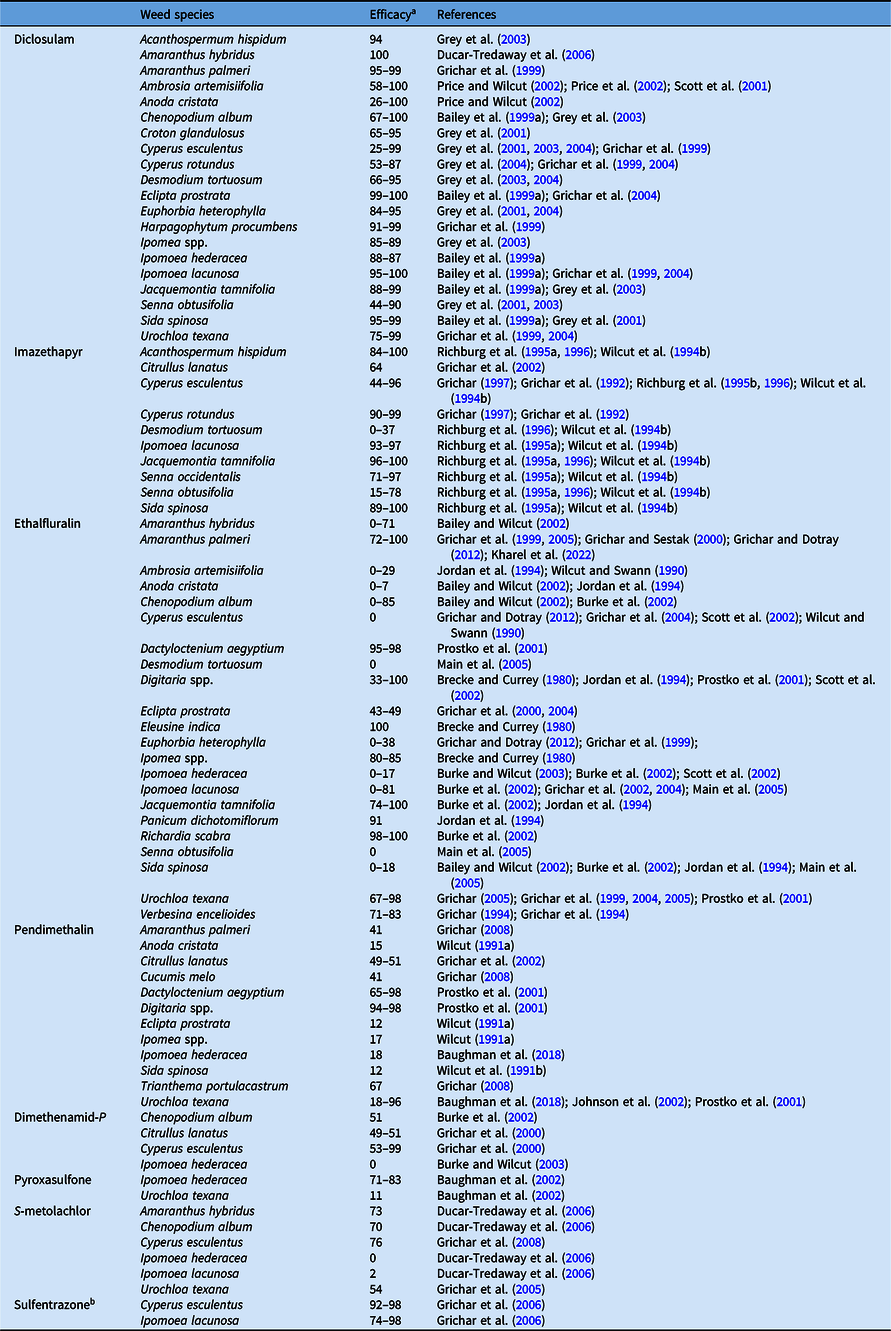
a Efficacy range across all references listed from 0% to 100%, where 0 = no control, 100 = complete control.
b No longer registered for U.S. peanut production.
While these herbicides provide effective weed control, peanut injury, stunting, and delayed emergence can occur depending on the method, timing, and rate of application (Chaudhari et al. Reference Chaudhari, Jordan, Grey, Prostko and Jennings2018; Grichar and Dotray Reference Grichar and Dotray2012). Peanut injury is also influenced by environmental factors such as soil moisture, temperature, pH, and organic matter (Cardina and Swann Reference Cardina and Swann1988; Chaudhari et al. Reference Chaudhari, Jordan, Grey, Prostko and Jennings2018). S-metolachlor has been observed to cause greater injury and growth suppression at higher rates under wet conditions (Basinger et al. Reference Basinger, Randell and Prostko2021; Chaudhari et al. Reference Chaudhari, Jordan, Grey, Prostko and Jennings2018). Variable peanut injury ranging from <5% to 33% was reported from preemergence application of S-metolachlor up to 1.40 kg ai ha−1 (Basinger et al. Reference Basinger, Randell and Prostko2021; Chaudhari et al. Reference Chaudhari, Jordan, Grey, Prostko and Jennings2018; Clewis et al. Reference Clewis, Everman, Jordan and Wilcut2007; Grichar et al. Reference Grichar, Dotray and Baughman2008). Although various levels of peanut injury have been observed from chloroacetamide herbicides, yields were not negatively impacted, except at rates higher than label recommended (Chaudhari et al. Reference Chaudhari, Jordan, Grey, Prostko and Jennings2018; Clewis et al. Reference Clewis, Everman, Jordan and Wilcut2007; Grichar et al. Reference Grichar, Dotray and Etheredge2015). In studies evaluating peanut tolerance to preemergence applications of S-metolachlor at 1.1, 1.4, and 2.8 kg ha−1, Basinger et al. (Reference Basinger, Randell and Prostko2021) observed yield reduction (8.9%) only at the 2.6X (2.8 kg ha−1) recommended rate.
Pyroxasulfone is another chloroacetamide herbicide used to control grasses and small-seeded broadleaf weeds in peanut (Dotray et al. Reference Dotray, Baughman, Grichar and Woodward2018; Eure et al. Reference Eure, Prostko and Merchant2015) and is similar in activity to acetochlor, dimethenamid-P, and S-metolachlor. Pyroxasulfone has a similar weed control spectrum but has a higher specific activity, allowing for a much lower application rate compared with dimethenamid-P (Grichar et al. Reference Grichar, Dotray and Baughman2019). Peanut generally has good tolerance to pyroxasulfone; however, preemergence applications caused 8% to 18% early-season peanut stunting (Eure et al. Reference Eure, Prostko and Merchant2015; Grichar et al. Reference Grichar, Dotray and Baughman2019; Prostko et al. Reference Prostko, Grey, Webster and Kemerait2011). Several factors, including rates, soil type, environmental conditions, and cultivars, may influence early-season stunting or injury, with greater stunting following preemergence applications in heavy soils under wet conditions (Prostko et al. Reference Prostko, Grey, Webster and Kemerait2011). Prostko et al. (Reference Prostko, Grey, Webster and Kemerait2011) reported significant peanut stunting preemergence (48% at 480 g ai ha−1, representing 4X use rate) in a sandy loam soil with 16% clay content, but no significant injury in a Tifton sand soil type with 2% clay content. Eure et al. (Reference Eure, Prostko and Merchant2015) observed significant stunting (38% to 55%) following preemergence applications at 240 g ai ha−1 (2X use rate) when peanut emergence coincided with heavy rain events.
Dinitroaniline (Ethalfluralin and Pendimethalin)
Dinitroaniline herbicides such as ethalfluralin and pendimethalin are Group 3 herbicides that block mitosis through inhibition of microtubule polymerization. These herbicides are readily absorbed by roots and emerging shoots and are commonly used to control annual grasses and small-seeded broadleaf weeds in peanut (Johnson and Mullinix Reference Johnson and Mullinix1999). Dinitroanilines have low water solubility (<1 ppm) and are moderately volatile and susceptible to photodegradation (Weber Reference Weber1990). As such, these herbicides are usually incorporated with rainfall, irrigation, or mechanically to prevent loss via evaporation (Johnson and Mullinix Reference Johnson and Mullinix1999). They can also be applied preemergence, but results are inconsistent compared with control by preplant-incorporated applications (Brecke and Currey Reference Brecke and Currey1980; Johnson and Mullinix Reference Johnson and Mullinix1999). Ethalfluralin and pendimethalin provide >80% control of annual grasses such as crowfootgrass [Dactyloctenium aegyptium (L.) Willd.], Digitaria spp., E. crus-galli, fall panicum (Panicum dichotomiflorum Michx.), and U. texana (data from conventional tillage) and small-seeded broadleaf weeds such as Amaranthus spp., common lambsquarters (Chenopodium album L.), and Florida pusley (Richardia scabra L.) (Tables 4 and 5). Dinitroanilines do not provide adequate control of A. artemisiifolia, Cyperus spp., D. tortuosum, eclipta [Eclipta prostrata (L.) L.], Ipomoea spp., S. spinosa, S. obtusifolia, and tropic croton (Croton glandulosus L.) (Grichar and Colburn Reference Grichar and Colburn1996; Scott et al. Reference Scott, Askew, Wilcut and Bennett2002; Wilcut et al. Reference Wilcut, York, Grichar and Wehtje1995). Grichar et al. (Reference Grichar, Colburn and Kearney1994) found dinitroaniline herbicides were ineffective in controlling U. texana in conservation-tillage peanut production. Both ethalfluralin and pendimethalin applied preemergence or preplant incorporated provided poor early-season control of U. texana in non-irrigated minimum-tillage peanut (Wilcut et al. Reference Wilcut, Wehtje and Hicks1990b). Similarly, in irrigated strip-tillage peanut, U. texana control with pendimethalin was not >75%, while control in plots treated with ethalfluralin was not better compared with the untreated control (Johnson et al. Reference Johnson, Prostko and Mullinix2002). The reduced efficacy of these dinitroaniline herbicides in conservation-tillage peanut was attributed to adsorption by cover crop residues and organic matter, resulting in greater concentration of the herbicide in the seed germination zone (Johnson et al. Reference Johnson, Prostko and Mullinix2002).
Table 5. Cumulative results on the efficacy of seedling root growth (ethalfluralin and pendimethalin) and protoporphyrinogen oxidase (flumioxazin and sulfentrazone) inhibitors applied preemergence in peanut.
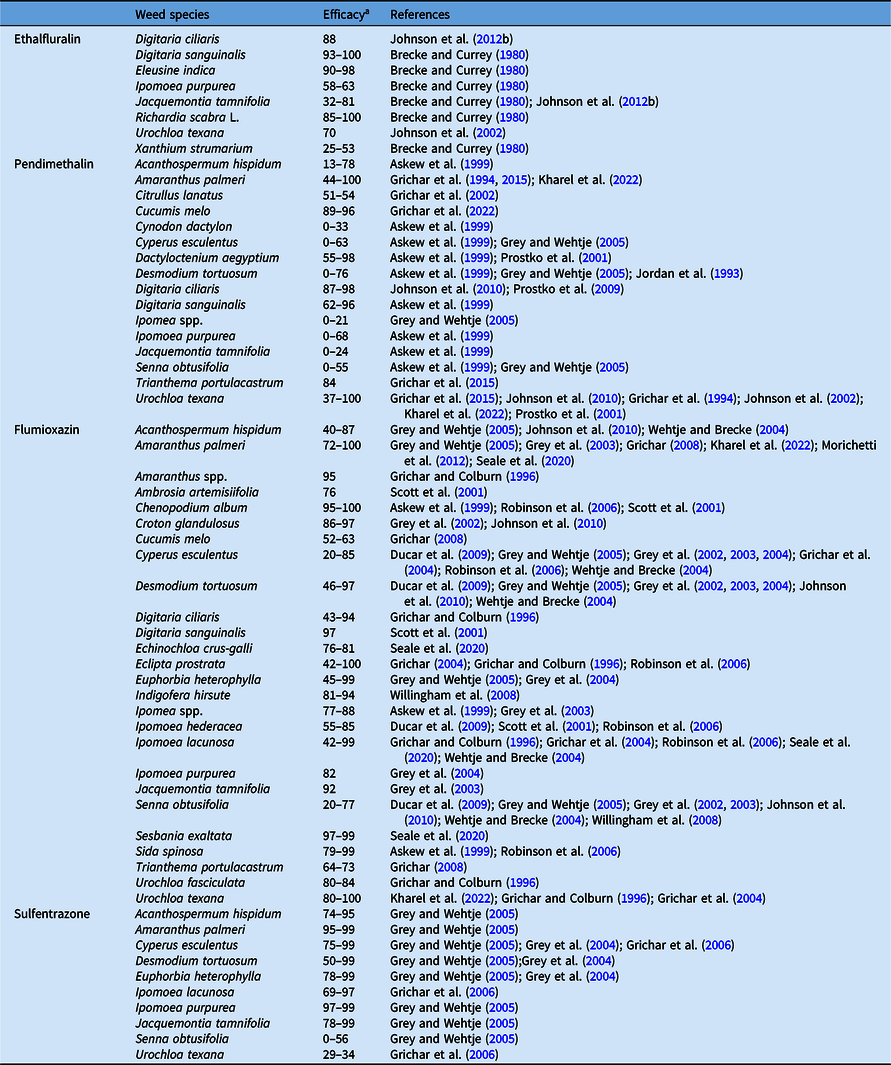
a Efficacy range across all references listed from 0% to 100%, where 0 = no control, 100 = complete control.
Peanut injury, expressed as stunting, swollen hypocotyls, and abnormal lateral root growth, has been observed with dinitroaniline herbicides (Johnson et al. Reference Johnson, Colvin, Wehtje, Littlefield and Mullinix1997; Johnson and Mullinix Reference Johnson and Mullinix1999). The level of injury from these herbicides can vary depending on the method, timing, and rates of application (Johnson and Mullinix Reference Johnson and Mullinix1999; Johnson et al. Reference Johnson, Prostko and Davis2011). Ethalfluralin and pendimethalin were reported to be more injurious to peanut when applied preplant incorporated than preemergence, and injury increased with increasing rate of application from 0.6 to 2.2 kg ai ha−1 for each herbicide (Johnson and Mullinix Reference Johnson and Mullinix1999). Similarly, applications of ethalfluralin and pendimethalin delayed until 3 wk after emergence caused significant injury and reduced peanut yield compared with preemergence applications (Johnson et al. Reference Johnson, Prostko and Davis2011). Dinitroaniline injury can also be undetected until harvest, because the vegetative growth may appear unaffected, but injured plants often have large numbers of pegs and very few pods (Johnson et al. Reference Johnson, Prostko and Davis2011). Peanut gynophores (pegs) form aboveground and grow downward to penetrate the soil surface (pegging), where they contact damaging levels of the herbicide at the soil surface. These herbicides block cell division in the developing peg, thus preventing the peg from penetrating the soil and forming the pod (Johnson et al. Reference Johnson, Colvin, Wehtje, Littlefield and Mullinix1997).
Although injury has been observed, several studies, especially in conventional tillage systems, reported preplant-incorporated or preemergence applications of ethalfluralin, pendimethalin, and trifluralin do not affect peanut yield when used at the recommended application timings and rates (Brecke and Currey Reference Brecke and Currey1980; Dotray et al. Reference Dotray, Keeling, Grichar, Prostko, Lemon and Everrit2003; Grichar and Colburn Reference Grichar and Colburn1993; Johnson et al. Reference Johnson, Colvin, Wehtje, Littlefield and Mullinix1997). In a 3-yr study, Grichar and Colburn (Reference Grichar and Colburn1993) observed that yield and grade of five runner peanut cultivars were not affected by preplant-incorporated application of ethalfluralin, pendimethalin, and trifluralin. Similarly, peanut yield was not affected by preplant-incorporated application of ethalfluralin and pendimethalin at 0.67 to 1.68 kg ai ha−1 and trifluralin at 0.56 and 0.71 kg ai ha−1 in five runner-type cultivars. However, Johnson et al. (Reference Johnson, Prostko and Davis2011) showed that both ethalfluralin and pendimethalin have the potential to inhibit pod formation and reduce peanut yield in strip-tillage systems, especially when the application is delayed. Studies have also reported that cover crop debris present in strip-tillage systems can influence the availability of herbicide, which may cause the herbicide to be more injurious to peanut (Johnson et al. Reference Johnson, Prostko and Mullinix2002; Weber Reference Weber1990). The retention of cover crop residue in strip-tillage systems can increase soil-water content and cause cooler soil temperatures, which possibly resulted in higher peanut injury from the herbicides at ground cracking.
PPO Inhibitor (Flumioxazin)
Flumioxazin is an N-phenylphthalimide herbicide registered only for preemergence applications in peanut (Askew et al. Reference Askew, Wilcut and Cranmer1999; Grichar and Colburn Reference Grichar and Colburn1996). This herbicide is absorbed by plants mainly through seedling root and shoot uptake (Yoshida et al. Reference Yoshida, Sakaki, Sato, Haga, Nagano, Oshio and Kamoshita1991) and can provide 4 to 6 wk of broad-spectrum residual weed control. It is particularly effective against broadleaf weeds that are difficult to control in peanut such as D. tortuosum (Ducar et al. Reference Ducar, Clewis, Wilcut, Jordan, Brecke, Grichar, Johnson and Wehtje2009; Grey et al. Reference Grey, Bridges, Prostko, Eastin, Johnson, Vencill, Brecke, MacDonald, Ducar, Everest and Wehtje2003; Johnson et al. Reference Johnson, Prostko and Davis2010). Flumioxazin was also reported to provide >70% control of A. artemisiifolia, A. hispidum, A. palmeri, C. album, C. glandulosus, citron melon [Citrullus lanatus (Thunb.) Matsum. & Nakai], large crabgrass [Digitaria sanguinalis (L.) Scop.], E. crus-galli, E. prostrata, Ipomoea spp., S. spinosa, smellmelon (Cucumis melo L.), and U. texana in peanut (Table 5). It can be used to provide effective control of ALS inhibiting herbicide–resistant weeds, particularly A. palmeri (Grichar and Dotray Reference Grichar and Dotray2013; Seale et al. Reference Seale, Bararpour, Bond, Gore and Golden2020). Flumioxazin does not effectively control C. esculentus (Ducar et al. Reference Ducar, Clewis, Wilcut, Jordan, Brecke, Grichar, Johnson and Wehtje2009; Grey et al. Reference Grey, Bridges, Hancock and Davis2004), S. obtusifolia (Grey and Wehtje Reference Grey and Wehtje2005; Johnson et al. Reference Johnson, Prostko and Davis2010; Willingham et al. Reference Willingham, Brecke, Treadaway-Ducar and MacDonald2008), and wild poinsettia (Euphorbia heterophylla L.) (Grey et al. Reference Grey, Bridges, Hancock and Davis2004), and annual grass control is inconsistent (Grichar and Colburn Reference Grichar and Colburn1996).
Flumioxazin selectivity in tolerant species and peanut is achieved through rapid metabolism (Yoshida et al. Reference Yoshida, Sakaki, Sato, Haga, Nagano, Oshio and Kamoshita1991). In a laboratory study evaluating differential tolerance of I. hederacea, S. obtusifolia, and peanut, Price et al. (Reference Price, Wilcut and Cranmer2004) observed more rapid metabolism of root-absorbed [14C]flumioxazin in peanut and S. obtusifolia (tolerant species) compared with I. hederacea (susceptible species). Peanut metabolism was three times faster than I. hederacea metabolism, with only 11% of the parent compound retained 72 h after treatment compared with 41% retained in I. hederacea at the same time point (Price et al. Reference Price, Wilcut and Cranmer2004). This suggests peanut metabolizes root-absorbed flumioxazin before any visible injury is observed. Price et al. (Reference Price, Wilcut and Cranmer2004) also showed that peanut germination was not affected by the direct exposure of peanut seed to flumioxazin at field application rates. Flumioxazin injury characterized by stunting and leaflet discoloration was <30% when applied preemergence at recommended rates (Askew et al. Reference Askew, Wilcut and Cranmer1999; Burke et al. Reference Burke, Askew and Wilcut2002; Grey et al. Reference Grey, Bridges, Hancock and Davis2004; Grey and Wehtje Reference Grey and Wehtje2005; Hurdle et al. Reference Hurdle, Grey, Pilon, Monfort and Prostko2020; Teuton et al. Reference Teuton, Main, MacDonald, Ducar and Brecke2004; Umphres et al. Reference Umphres, Steckel and Mueller2018; Wilcut et al. Reference Wilcut, Askew, Bailey, Spears and Isleib2001). However, delaying application until germination initiation increases the risk of direct contact with emerged plants, which is highly injurious to peanut (Burke et al. Reference Burke, Askew and Wilcut2002; Jordan et al. Reference Jordan, Lancaster, Lanier, Lassiter and Johnson2009a). Flumioxazin applied at 6, 8, and 10 d after planting caused significant peanut injury (20% to 59%) compared with applications at 0, 2, and 4 d after planting (0% to 29%) (Burke et al. Reference Burke, Askew and Wilcut2002).
Peanut injury from flumioxazin is also influenced by soil organic matter and clay content (Leon and Tillman Reference Leon and Tillman2015). Planting depth and flumioxazin placement depth has also been shown to influence injury potential (Ferrell et al. Reference Ferrell, Vencill, Xia and Grey2005). Greater injury is also associated with cool weather or rainfall occurring during or soon after flumioxazin application due to increased absorption and decreased metabolism (Hurdle et al. Reference Hurdle, Grey, Pilon, Monfort and Prostko2020; Umphres et al. Reference Umphres, Steckel and Mueller2018; Wilcut et al. Reference Wilcut, Askew, Bailey, Spears and Isleib2001). In studies conducted with three runner-type peanut cultivars, Wilcut et al. (Reference Wilcut, Askew, Bailey, Spears and Isleib2001) reported flumioxazin injury caused season-long reduction in peanut canopy, but yield was not adversely affected. Similarly, Burke et al. (Reference Burke, Askew and Wilcut2002) observed delayed peanut pod development due to injury from flumioxazin, but no reduction in peanut yield. Hurdle et al. (Reference Hurdle, Grey, Pilon, Monfort and Prostko2020) reported yield reduction due to flumioxazin injury in one of three locations in a study conducted in North Carolina. At this site, injury of 50% to 67% was observed due to cool and wet conditions at the time of peanut emergence compared with <2% injury from the same studies at two other locations where environmental conditions were favorable for peanut emergence.
Heavy rainfall that results in splashing of flumioxazin-treated soil on peanut foliage can cause temporary injury (Grey et al. Reference Grey, Dotray and Grichar2007). Generally, peanut injury from flumioxazin is transient, with recovery between 5 and 8 wk after treatment with no effect on peanut grade and pod yield regardless of flumioxazin application rate, timing, or peanut cultivar (Askew et al. Reference Askew, Wilcut and Cranmer1999; Basinger et al. Reference Basinger, Randell and Prostko2021; Ducar et al. Reference Ducar, Clewis, Wilcut, Jordan, Brecke, Grichar, Johnson and Wehtje2009; Johnson et al. Reference Johnson, Prostko and Mullinix2006; Main et al. Reference Main, Ducar, Whitty and MacDonald2005; Seale et al. Reference Seale, Bararpour, Bond, Gore and Golden2020; Umphres et al. Reference Umphres, Steckel and Mueller2018; Wilcut et al. Reference Wilcut, Askew, Bailey, Spears and Isleib2001). Despite preemergence activity, flumioxazin has relatively low soil persistence and does not have carryover concerns for rotation with corn, cotton, and soybean (Grey et al. Reference Grey, Bridges, Eastin and MacDonald2002).
Sulfentrazone is another soil-applied PPO-inhibiting herbicide that has been evaluated but is no longer registered in peanut (Grey et al. Reference Grey, Dotray and Grichar2007). Sulfentrazone applied preemergence or preplant incorporated provided effective control of many broadleaf weeds, including A. hispidum, A. palmeri, D. tortuosum, Ipomoea spp., and E. heterophylla (Tables 4 and 5). However, significant peanut injury and yield reduction from sulfentrazone was reported across the major peanut-growing regions (Grey et al. Reference Grey, Bridges, Hancock and Davis2004; Grichar et al. Reference Grichar, Besler and Dotray2006; Johnson and Mullinix Reference Johnson and Mullinix1994). Although sulfentrazone is effective for weed control, the unacceptable injury and rotational concerns, especially under coarse-textured soil typical of most peanut-growing regions, resulted in the registration being cancelled for peanut (Grey and Wehtje Reference Grey and Wehtje2005; Grichar et al. Reference Grichar, Besler and Dotray2006).
Foliar-applied Broadleaf Herbicides
Postemergence broadleaf weed herbicides are critical for successful weed management programs in peanut. Control of late-emerging broadleaf weeds and those that escape early-season control from soil-applied herbicides is critical to maintaining good yields and proper harvesting (Everman et al. Reference Everman, Clewis, Taylor and Wilcut2006; Wilcut et al. Reference Wilcut, York, Grichar and Wehtje1995). Peanut growers often make two or more postemergence broadleaf herbicide applications due to peanut’s long growing season and continuous broadleaf weed germination (Chaudhari et al. Reference Chaudhari, Jordan, Grey, Prostko and Jennings2018). Postemergence broadleaf weed control in peanut is achieved using bentazon and paraquat (photosynthetic inhibitors), acifluorfen and lactofen (PPO inhibitors), 2,4-DB (synthetic auxin), and chlorimuron, diclosulam, imazapic, and imazethapyr (ALS inhibitors). The application timing of these herbicides is important for effective control to ensure efficacy and reduce the need for multiple applications. Acifluorfen, bentazon, lactofen, and paraquat have contact activity and only kill tissues with which the spray comes into contact. Therefore, maximum weed control is achieved when applied to smaller weeds between peanut ground crack (GC) and 2 to 3 wk after GC (Grey et al. Reference Grey, Bridges and Eastin2001; Jordan et al. Reference Jordan, Culpepper, Grichar, Ducar, Brecke and York2003a). Conversely, chlorimuron, diclosulam, imazapic, imazethapyr, and 2,4-DB have systemic activity, and weed size is not as critical, as the herbicide is absorbed and translocated to meristematic tissues, killing underground structures (Dotray and Keeling Reference Dotray and Keeling1997; Everman et al. Reference Everman, Clewis, Taylor and Wilcut2006).
Photosynthetic Inhibitors (Bentazon and Paraquat)
Bentazon kills susceptible species by blocking electron flow in PSII, inhibiting the production of NADPH + H and ATP needed for CO2 fixation in the light-independent reactions (Shaner Reference Shaner2014). The blockage of electrons also elicits massive radical production, and this severe oxidative stress causes membrane disruption and cellular breakdown. Affected plants show chlorosis followed by necrosis and rapid plant death. Bentazon applied postemergence controls several broadleaf weeds in peanut (Table 6) and has activity on C. esculentus (Grichar Reference Grichar1992). However, it lacks residual activity and is ineffective for postemergence control of Amaranthus spp., D. tortuosum, Ipomoea spp., and S. obtusifolia (Grey et al. Reference Grey, Bridges and Eastin2001; Richburg et al. Reference Richburg, Wilcut, Culbreath and Kvien1993a). Bentazon is often applied in mixture with chloroacetamide herbicides such as metolachlor and dimethenamid-P to provide residual control and with other postemergence herbicides such as acifluorfen, lactofen, paraquat, and 2,4-DB to improve control of broadleaf weeds and increase weed control spectrum (Grichar et al. Reference Grichar, Colburn and Kearney1994). Bentazon applied in tank mixture with acifluorfen provided >90% control of A. artemisiifolia, C. album, C. glandulosus, and Ipomoea spp. (Wilcut Reference Wilcut1991b) and >95% control of A. palmeri compared with <60% control from bentazon alone (Grichar Reference Grichar1997a). While the tank mixture of bentazon with residual or postemergence broadleaf herbicides increased weed control spectrum and provided a residual effect for an extended period, considerable injury to peanut can occur (Grichar et al. Reference Grichar, Jordan and Prostko2012; Jordan et al. Reference Jordan, Spears and Wilcut2003b). Grichar et al. (Reference Grichar, Jordan and Prostko2012) reported 5% to 20% peanut injury with bentazon plus imazapic, while Jordan et al. (Reference Jordan, Spears and Wilcut2003b) observed greater peanut injury with early postemergence application of bentazon plus acifluorfen and bentazon plus acifluorfen plus 2,4-DB. In addition, acifluorfen plus bentazon and acifluorfen plus bentazon plus 2,4-DB reduced yield by 200 and 150 kg ha−1, respectively, when compared with a nontreated control in a 3-yr weed-free trial (Jordan et al. Reference Jordan, Spears and Wilcut2003b). Similarly, late postemergence application of bentazon plus acifluorfen reduced peanut yield by at least 23% compared with a weed-free control (Jordan et al. Reference Jordan, Wilcut and Swann1993). Wilcut (Reference Wilcut1991a) also observed up to 37% reduction in peanut yield with delayed application of bentazon plus acifluorfen until 4 wk after GC compared with application at GC.
Table 6. Cumulative results on efficacy of photosynthesis inhibitors applied postemergence in peanut.
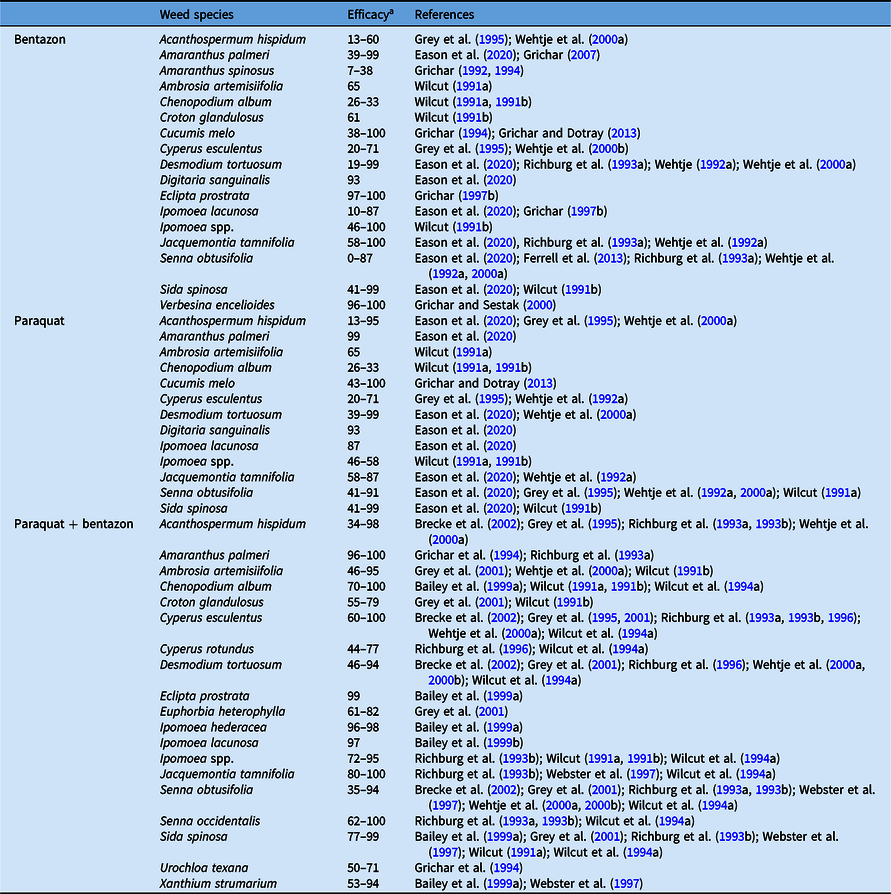
a Efficacy range across all references listed from 0% to 100%, where 0 = no control, 100 = complete control.
Paraquat is a nonselective postemergence herbicide commonly used in peanut production, particularly in the southeastern United States (Eason et al. Reference Eason, Grey, Tubbs, Prostko and Li2020; Wilcut et al. Reference Wilcut, York, Grichar and Wehtje1995; Wehtje et al. Reference Wehtje, Wilcut, McGuire and Hicks1991). Paraquat was demonstrated to be a suitable replacement for dinoseb for control of annual broadleaf weeds in peanut (Wilcut et al. Reference Wilcut, York, Grichar and Wehtje1995). It is rapidly absorbed into plant foliage, killing susceptible species by diverting electrons in PSI and the production of highly reactive oxygen species (Shaner Reference Shaner2014). It can be applied from peanut hypocotyl emergence until 28 d after emergence to control early-emerging weeds (Jordan et al. Reference Jordan, Lancaster, Lanier, Lassiter and Johnson2003a). Paraquat provides good to excellent control of several annual broadleaf weeds, including D. tortuosum, S. obtusifolia, and Ipomoea spp. (Table 7) in addition to its activity on grass weed species (Wilcut Reference Wilcut1991b). However, it does not control A. hispidum, coffee senna [Senna occidentalis (L.) Link], S. spinosa, and smallflower morningglory [Jacquemontia tamnifolia (L.) Griseb.] (Wilcut et al. Reference Wilcut, Wehtje, Hicks and Cole1990c, Reference Wilcut, York, Grichar and Wehtje1995). Paraquat lacks residual activity; therefore, subsequent herbicide applications are often required to maintain season-long weed control. While paraquat provides consistent control of several annual grass and broadleaf weeds prevalent in the southeastern United States, it causes peanut stunting and foliar injury characterized by chlorosis, necrosis, and bronzing. However, plants recover rapidly under good environmental conditions, and yield is not affected in most cases if it is applied before pegging and fruit development (Carley et al. Reference Carley, Jordan, Brandenburg and Dharmasri2009; Eason et al. Reference Eason, Grey, Tubbs, Prostko and Li2020; Knauft et al. Reference Knauft, Colvin and Gorbet1990; Wehtje et al. Reference Wehtje, Brecke and Bostick1994). Conversely, studies conducted with Virginia peanut types showed paraquat may affect peanut grade by delaying maturity (Carley et al. Reference Carley, Jordan, Brandenburg and Dharmasri2009; Knauft et al. Reference Knauft, Colvin and Gorbet1990). In addition, paraquat injury can interact with other stressors, resulting in significant yield reduction. Brecke et al. (Reference Brecke, Funderburk, Teare and Gorbet1996) reported significant reduction in peanut yield when paraquat was applied to peanut with damage from thrips. Similarly, paraquat application after 28 d of peanut emergence increases the chances of significant yield reduction (Brecke et al. Reference Brecke, Funderburk, Teare and Gorbet1996; Johnson et al. Reference Johnson, Colvin and Mullinix1993). When paraquat application was delayed until 2 wk after GC, A. artemisiifolia control was reduced by 30% and peanut yield by 1,200 kg ha−1, with a consequent net loss of about $400 ha−1 compared with application at GC (Wilcut and Swann Reference Wilcut and Swann1990).
Table 7. Cumulative results on efficacy of protoporphyrinogen oxidase inhibitors (acifluorfen and lactofen) and synthetic auxin (2,4-DB) applied postemergence in peanut.
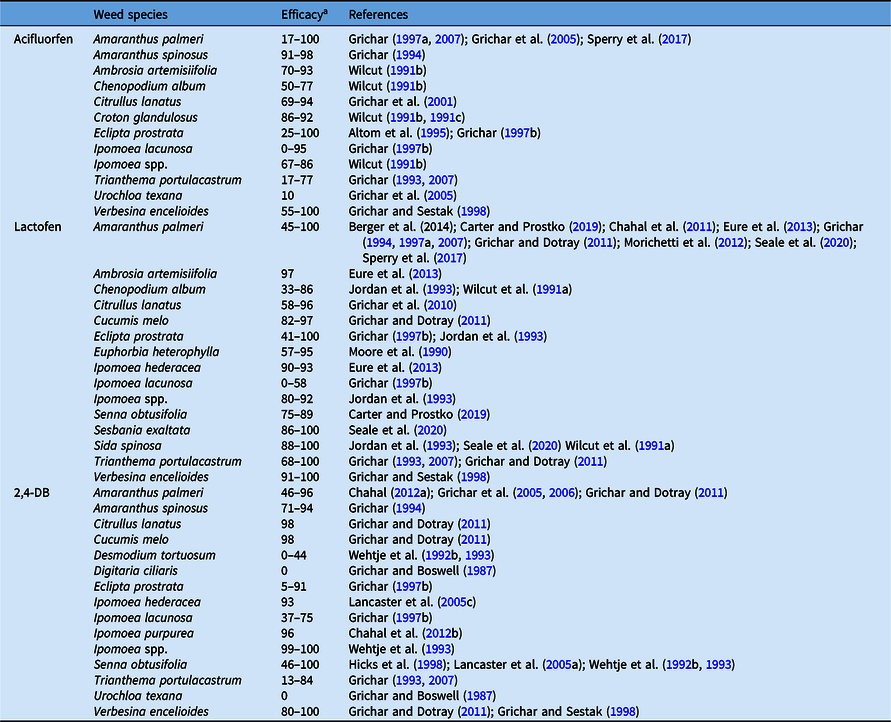
a Efficacy range across all references listed from 0% to 100%, where 0 = no control, 100 = complete control.
Depending on weed community composition, paraquat is often combined with imazapic or S-metolachlor to increase residual activity (Carley et al. Reference Carley, Jordan, Brandenburg and Dharmasri2009; Grichar and Dotray Reference Grichar and Dotray2012; Wehtje et al. Reference Wehtje, Brecke and Martin2000a, Reference Wehtje, Padgett and Martin2000b). Although tank mixtures of paraquat and residual herbicides can improve weed control, significant injury and stunting may occur (Eason et al. Reference Eason, Grey, Tubbs, Prostko and Li2020; Grichar and Dotray Reference Grichar and Dotray2013; Grichar et al. Reference Grichar, Jordan and Prostko2012). Eason et al. (Reference Eason, Grey, Tubbs, Prostko and Li2020) reported significant peanut stunting up to 25% with paraquat plus S-metolachlor compared with paraquat alone (6% to 15%). Paraquat is also commonly applied in tank mixture with other postemergence broadleaf herbicides such as bentazon and 2,4-DB to reduce foliar injury to peanut, improve control of larger weeds, and broaden the weed control spectrum (Eason et al. Reference Eason, Grey, Tubbs, Prostko and Li2020; Price et al. Reference Price, Li, Price, Chen and Grey2020; Wehtje et al. Reference Wehtje, Wilcut and McGuire1992a, Reference Wehtje, Wilcut and McGuire1992b). Co-application of paraquat and 2,4-DB improved control of larger S. obtusifolia plants than paraquat applied alone (Wehtje et al. Reference Wehtje, Wilcut and McGuire1992b). Similarly, paraquat applied in tank mixture with bentazon controlled a broader spectrum of broadleaf weeds including such as A. hispidum, C. rotundus, common cocklebur (Xanthium strumarium L.), S. occidentalis, S. spinosa, and J. tamnifolia, (Table 7) and reduced foliar injury from paraquat compared with paraquat applied alone (Eason et al. Reference Eason, Tubbs, Grey and Li2019; Grey et al. Reference Grey, Wehtje, Walker and Paudel1995; Wehtje et al. Reference Wehtje, Wilcut and McGuire1992a; Wilcut et al. Reference Wilcut, Walls and Horton1991b, Reference Wilcut, Richburg, Eastin, Wiley, Walls and Newell1994a). However, bentazon applied with paraquat can act as an antagonist by reducing the absorption of paraquat on the leaf surface, thereby reducing paraquat efficacy on certain species (Wehtje et al. Reference Wehtje, Wilcut and McGuire1992a). In greenhouse and field studies, bentazon reduced the efficacy of paraquat for the control of D. tortuosum, S. obtusifolia, and U. texana in peanut (Wehtje et al. Reference Wehtje, Wilcut and McGuire1992a).
Paraquat is not commonly used in the southwestern United States because peanut injury from paraquat applied during the hotter periods can significantly reduce peanut yield and grade characteristics (Knauft et al. Reference Knauft, Colvin and Gorbet1990; Wilcut and Swann Reference Wilcut and Swann1990; Wilcut et al. Reference Wilcut, York, Grichar and Wehtje1995). Likewise, paraquat alone or in mixture with bentazon or 2,4-DB is not a common herbicide program in the Virginia-North Carolina region because paraquat does not provide adequate control of A. artemisiifolia, C. album, C. glandulosus and spurred anoda [Anoda cristata (L.) Schltdl.] which are commonly found in the region (Wilcut et al. Reference Wilcut, Richburg, Eastin, Wiley, Walls and Newell1994a, Reference Wilcut, York, Grichar and Wehtje1995).
PPO Inhibitors (Acifluorfen, Carfentrazone, and Lactofen)
Acifluorfen and lactofen are used for postemergence control of annual broadleaf weeds in peanut. They are classified as diphenyl ether (cell membrane disrupter) that kill weeds rapidly by interfering with protoporphyrinogen IX oxidase synthesis and causing accumulation of protoporphyrin IX in the cytoplasm (Shaner Reference Shaner2014). This molecule reacts with light, resulting in the production of toxic singlet oxygen species, which deteriorate cell membranes. Acifluorfen and lactofen provide >70% control of several annual broadleaf weeds such as A. palmeri, A. spinosus, A. artemisiifolia, C. glandulosus, C. melo, and S. spinosa (Table 7). They also provide effective control of ALS herbicide– resistant weeds in peanut and rotated crops such as cotton and soybean especially, in the southwestern and Virgina-North Carolina peanut growing regions of the United States (Culpepper et al. Reference Culpepper, Grey, Vencill, Kichler, Webster, Brown, York, Davis and Hanna2006; Wise et al. Reference Wise, Grey, Prostko, Vencill and Webster2009). Acifluorfen and lactofen do not have residual activity at the rate used postemergence in peanut, thus sequential applications are often required for season long weed control (Dotray et al. Reference Dotray, Grichar, Baughman, Prostko, Grey and Gilbert2012; Wilcut et al. Reference Wilcut, Swann and Hagwood1990a). Sequential application of lactofen provides better weed control than single application (Jordan et al. Reference Jordan, Wilcut and Swann1993; Sperry et al. Reference Sperry, Ferrell, Smith, Fernandez, Leon and Smith2017) but can cause reduction in peanut yield (Sperry et al. Reference Sperry, Ferrell, Smith, Fernandez, Leon and Smith2017; Wilcut Reference Wilcut1991b).
The efficacy of acifluorfen and lactofen is influenced by weed size or stage of weed growth at the time of application (Wilcut and Swann Reference Wilcut and Swann1990; Wilcut Reference Wilcut1991a, Reference Wilcut1991b). For example, efficacy of acifluorfen and lactofen on E. prostrata decreased with delayed applications, as larger weeds (>10 cm) escaped control (Grichar Reference Grichar1997b). Lactofen controlled C. album 86% when applied at the cotyledon to 2-leaf stage, but control declined to 34% when C. album was 10-cm high (Wilcut Reference Wilcut1991b). In the same study, lactofen controlled S. spinosa 87% to 95% and Ipomoea spp. 83% to 86% when applied at the 2-leaf stage, but control declined to 0% when applications were made at the 3- to 7-leaf stages.
Acifluorfen and lactofen can cause reduced canopy growth and visible peanut injury characterized by leaf bronzing, cupping and crinkling of leaf margins, and necrotic spots/lesions. Plant recover within 2 to 4 wk after application, and yield penalties are rarely observed if applications are made before full seed fill (Chaudhari et al. Reference Chaudhari, Jordan, Grey, Prostko and Jennings2018; Ferrell et al. Reference Ferrell, Leon, Sellers, Rowland and Brecke2013; Grichar Reference Grichar1994). However, yield reduction can occur with sequential applications and applications made later in the season, especially between the R5 (beginning seed) to R6 (full seed) growth stages (Grichar Reference Grichar1997a; Jordan et al. Reference Jordan, Wilcut and Swann1993; Sperry et al. Reference Sperry, Ferrell, Smith, Fernandez, Leon and Smith2017; Wilcut Reference Wilcut1991b). Peanut yield was reduced by 49% with late postemergence application of acifluorfen plus bentazon and by 45% with sequential application of lactofen (Jordan et al. Reference Jordan, Wilcut and Swann1993). Wilcut et al. (Reference Wilcut, Wehtje and Hicks1990b) reported 39% and 45% reduction in peanut yield when application of acifluorfen plus bentazon and lactofen was delayed until 4 wk after GC compared with GC application, respectively. Other studies have reported similar yield reductions following sequential application of lactofen in peanut (Boyer et al. Reference Boyer, Ferrell, MacDonald, Tillman and Rowland2011; Grichar Reference Grichar1997b: Sperry et al. Reference Sperry, Ferrell, Smith, Fernandez, Leon and Smith2017; Wilcut et al. Reference Wilcut, Wehtje and Hicks1990b). Acifluorfen and lactofen are frequently tank mixed with 2,4-DB and crop oil concentrate (COC) (Ferrell et al. Reference Ferrell, Leon, Sellers, Rowland and Brecke2013; Grichar Reference Grichar1997b). Applying acifluorfen or lactofen with 2,4-DB increases efficacy, especially for ALS herbicide– resistant weeds, because 2,4-DB provides systemic activity. However, these herbicide treatments can cause visible peanut injury, although yield is not affected in most cases (Boyer et al. Reference Boyer, Ferrell, MacDonald, Tillman and Rowland2011; Ferrell et al. Reference Ferrell, Leon, Sellers, Rowland and Brecke2013). Application of lactofen with COC resulted in 48% injury and 10-d delay in peanut canopy closure (Boyer et al. Reference Boyer, Ferrell, MacDonald, Tillman and Rowland2011).
Carfentrazone is another PPO-inhibiting herbicide labeled for use in peanut. It has little or no residual effect but exhibits rapid contact activity, causing desiccation of susceptible weed species within hours of treatment and, consequently, plant death within days (Anonymous 2008, 2020). Carfentrazone is used to control Ipomoea species in peanut (Grichar et al. Reference Grichar, Dotray and Baughman2021; Kharel et al. Reference Kharel, Devkota, Macdonald, Tillman and Mulvaney2022), but only as a burndown treatment before planting (Anonymous 2008; Grichar et al. Reference Grichar, Dotray and Baughman2010). Carfentrazone has been shown to cause stunting and peanut injury ranging from 7% to 62% and significant yield reduction in various studies (Chaudhari et al. Reference Chaudhari, Jordan and Jennings2017; Dotray et al. Reference Dotray, Baughman and Grichar2010, Grichar et al. Reference Grichar, Dotray and Baughman2010, Reference Grichar, Dotray and Baughman2021; Kharel et al. Reference Kharel, Devkota, Macdonald, Tillman and Mulvaney2022; Price et al. Reference Price, Li, Price, Browne, Balkcom and Chen2021). Injury from carfentrazone can be substantially greater than injury from lactofen and paraquat plus bentazon, which is often considered as unacceptable by peanut growers (Dotray et al. Reference Dotray, Baughman and Grichar2010; Grichar et al. Reference Grichar, Dotray and Baughman2010). Several factors, including rates and timing of application, planting dates, and environmental factors, can influence peanut stunting or injury from carfentrazone. Carfentrazone causes more severe injury to peanut when applied early season rather than late season (Dotray et al. Reference Dotray, Baughman and Grichar2010; Grichar et al. Reference Grichar, Dotray and Baughman2010). Dotray et al. (Reference Dotray, Baughman and Grichar2010) reported greater peanut injury ranging from 14% to 19% following early postemergence (28 to 51 d after planting) application of carfentrazone at 27 and 36 g ai ha−1 compared with 6% to 8% injury from late postemergence (93 to 121 d after planting) application. Similarly, Grichar et al. (Reference Grichar, Dotray and Baughman2010) reported greater peanut injury ranging from 7% to 52% following early postemergence (35 d after planting) application of carfentrazone at 30 and 40 g ai ha−1 compared with 9% to 16% injury from late postemergence (56 d after planting) application. Injury from carfentrazone at these application rates and timings resulted in as much as 22% reduction in peanut yield, but peanut grade characteristics were not affected (Grichar et al. Reference Grichar, Dotray and Baughman2010). Yield losses up 27% were observed with carfentrazone-ethyl plus a high surfactant oil concentrate at 75 and 90 d after planting as compared with the nontreated check (Price et al. Reference Price, Li, Price, Browne, Balkcom and Chen2021). Similarly, carfentrazone application during the pod-filling stage (4 wk before digging) caused 10% reduction in peanut yield, whereas yield was not affected when applied at 1 or 2 wk before digging (Chaudhari et al. Reference Chaudhari, Jordan and Jennings2017). This research suggests carfentrazone may be an appropriate herbicide for late-season weed control to reduce weed interference with peanut digging and inversion.
Carfentrazone plus pyroxasulfone has recently been labeled as a commercially available premixed herbicide combination for post emergence application in peanut (Anonymous 2020). Available literature showed that carfentrazone plus pyroxasulfone can control small-seeded annual broadleaf weeds, including ALS herbicide– resistant A. palmeri in peanut (Grichar et al. Reference Grichar, Dotray and Baughman2021). Amaranthus palmeri control with carfentrazone plus pyroxasulfone preemergence was at least 78% season-long, while postemergence applications were inconsistent (24% to 100%). Pendimethalin plus premixed carfentrazone plus pyroxasulfone controlled C. melo at least 80% late season.
Synthetic Auxin (2,4-DB)
The herbicide 2,4-DB is a selective systemic phenoxyalkanoic acid Group 4 herbicide registered for use in peanut throughout the growing season (Jordan Reference Jordan2004). Several troublesome broadleaf weeds, including A. palmeri, C. melo, S. obtusifolia, Ipomoea spp., T. portulacastrum, golden crownbeard [Verbesina encelioides (Cav.) Benth. & Hook. f. ex A. Gray], and X. strumarium, are controlled with 2,4-DB applied postemergence in peanut (Table 7). However, 2,4-DB rarely provides complete control of S. obtusifolia and X. strumarium with a single application (Wilcut et al. Reference Wilcut, York, Grichar and Wehtje1995). Phenoxy herbicides are generally toxic to broadleaf weeds or crops, but legumes such as peanut exhibit high tolerance to 2,4-DB, and significant foliar injury is rarely observed (Dotray et al. Reference Dotray, Porter and Keeling2004; Faircloth and Prostko Reference Faircloth and Prostko2010). Tolerant legumes cannot convert the butyric acid side chain as readily as other broadleaf plants (Hawf and Behrens Reference Hawf and Behrens1974). Peanut tolerance to 2,4-DB has also been attributed to reduced spray retention, absorption, and translocation and less beta-oxidation within plant tissue, resulting in less conversion to the phytotoxic secondary metabolite (2,4-D) (Hawf and Behrens Reference Hawf and Behrens1974; Ketchersid et al. Reference Ketchersid, Boswell and Merkle1978). Earlier studies indicated that 2,4-DB was not readily absorbed by peanut leaves, was slowly metabolized to 2,4-D, and was not accumulated in the nut at harvest (Ketchersid et al. Reference Ketchersid, Boswell and Merkle1978). In contrast, 2,4-DB was rapidly absorbed and converted to 2,4-D and subsequently translocated to the apical region in redroot pigweed (Amaranthus retroflexus L.), a susceptible species, resulting in reduced growth and death of the plant (Ketchersid et al. Reference Ketchersid, Boswell and Merkle1978). Application of 2,4-DB is restricted within 60 d before peanut harvest. Peanut yield was not impacted when 2,4-DB was applied within recommended rates and timing (Baughman et al. Reference Baughman, Grichar and Jordan2002; Faircloth and Prostko Reference Faircloth and Prostko2010; Ferrell et al. Reference Ferrell, Leon, Sellers, Rowland and Brecke2013; Grichar et al. Reference Grichar, Sestak and Besler1997; Jordan et al. Reference Jordan, Spears and Wilcut2003b; Lancaster et al. Reference Lancaster, Jordan, York, Wilcut, Brandenburg and Monks2005c). In earlier studies, application of 2,4-DB at 0.95 kg ai ha−1 up to 62 d after planting reduced pod development in a Spanish cultivar, but yield was not reduced with sequential application at 0.45 kg ai ha−1 during pod fill (Ketchersid et al. Reference Ketchersid, Boswell and Merkle1978). However, peanut yield and grade characteristics were not adversely affected when 2,4-DB was applied at 0.45 kg ai ha−1 up to 120 d after planting in runner and Virginia market-type cultivars (Baughman et al. Reference Baughman, Grichar and Jordan2002; Grichar et al. Reference Grichar, Sestak and Besler1997). Similarly, Jordan et al. (Reference Jordan, Spears and Wilcut2003b) reported that 2,4-DB at 0.14 kg ai ha−1 did not adversely affect peanut pod yield and seed germination when applied at 3, 5, or 7 wk before digging.
The herbicide 2,4-DB is often applied in combination with other postemergence broadleaf weed herbicides such as acifluorfen, bentazon, lactofen, and paraquat to improve weed control spectrum (Burke et al. Reference Burke, Askew and Wilcut2002; Jordan et al. Reference Clewis, Everman, Jordan and Wilcut2007; Wilcut et al. Reference Wilcut, Richburg, Wiley, Walls, Jones and Iverson1994b). Application of 2,4-DB with these herbicides can also improve control of weeds larger than the recommended size for treatment with broadleaf herbicides (Ferrell et al. Reference Ferrell, Leon, Sellers, Rowland and Brecke2013; Jordan et al. Reference Jordan, Culpepper, Grichar, Ducar, Brecke and York2003a). Application of 2,4-DB in tank mixture with acifluorfen plus bentazon improved the control of A. palmeri between 10- to 20-cm height (Ferrell et al. Reference Ferrell, Leon, Sellers, Rowland and Brecke2013). However, these herbicide treatments can cause visible peanut injury (Ferrell et al. Reference Ferrell, Leon, Sellers, Rowland and Brecke2013; Jordan et al. Reference Jordan, Spears and Wilcut2003b) and stunting manifested as reduced canopy width (Ferrell et al. Reference Ferrell, Leon, Sellers, Rowland and Brecke2013), but yield penalties are rarely observed (Baughman et al. Reference Baughman, Grichar and Jordan2002; Dotray et al. Reference Dotray, Porter and Keeling2004; Ferrell et al. Reference Ferrell, Leon, Sellers, Rowland and Brecke2013).
Foliar-applied Graminicides (Clethodim, Fluazifop-P-Butyl, and Sethoxydim)
Clethodim, fluazifop-P-butyl, and sethoxydim are postemergence-applied herbicides commonly referred to as graminicides. They selectively inhibit lipid biosynthesis in susceptible species and are applied postemergence to control annual and perennial grasses in peanut throughout the majority of the growing season, especially grasses that escape control from soil-applied herbicides (Anonymous 2000a, 2000b; Chahal et al. Reference Chahal, Jordan, York, Brandenburg, Shew, Burton and Danehower2013; Lancaster et al. Reference Lancaster, Jordan, York, Wilcut, Brandenburg and Monks2005c; Prostko et al. Reference Prostko, Johnson and Mullinix2001). The effectiveness of these herbicides in controlling annual and perennial grasses is important to minimize interference and increase the efficiency of peanut digging and inversion, because the dense, fibrous root systems of grasses can cause peanut pods to be stripped from the vines (Wilcut et al. Reference Wilcut, York, Grichar and Wehtje1995). Clethodim and sethoxydim can provide >80% control of the most prevalent grass weed species in peanut, including broadleaf signalgrass [Urochloa platyphylla (Munro ex C. Wright) R.D. Webster], common bermudagrass [Cynodon dactylon (L.) Pers.], E. crus-galli, goosegrass [Eleusine indica (L.) Gaertn.], D. sanguinalis, P. dichotomiflorum, southern crabgrass [Digitaria ciliaris (Retz.) Koeler], and U. texana (Table 8). Similarly, >80% control of C. dactylon, D. ciliaris, U. platyphylla, and U. texana can be achieved with fluazifop-P-butyl (Table 8). Additionally, sequential application(s) can provide excellent (>90%) control of perennial grasses such as johnsongrass [Sorghum halepense (L.) Pers.] (York et al. Reference York, Wilcut and Grichar1993).
Table 8. Cumulative results on the efficacy of acetyl-CoA carboxylase inhibitors applied postemergence in peanut.
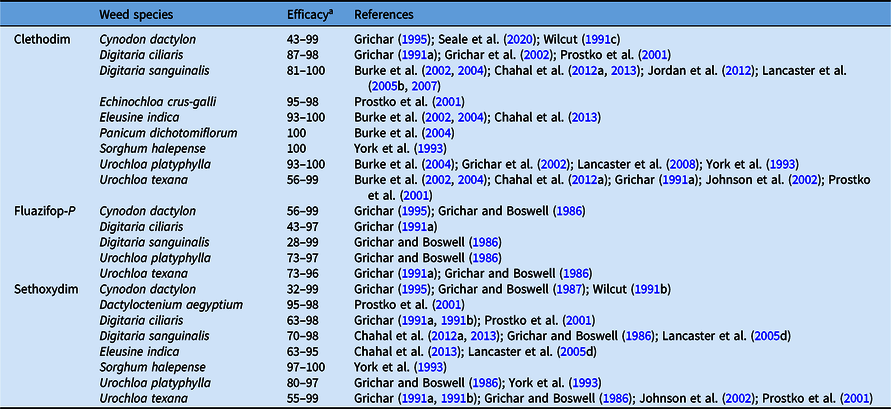
a Efficacy range across all references listed from 0% to 100%, where 0 = no control, 100 = complete control.
Graminicides are often applied in mixture with broadleaf herbicides and other pesticides to reduce the number of application trips over the field, saving time and fuel and increasing the spectrum of weed control and overall weed management (Burke et al. Reference Burke, Price, Wilcut, Jordan, Culpepper and Tredaway-Ducar2004; Grichar et al. Reference Grichar, Besler, Brewer and Baughman2002; Holshouser and Coble Reference Holshouser and Coble1990). However, this practice can affect weed control when the tank mix contains herbicides that are not compatible (Chahal et al. Reference Chahal, Jordan, Shew, Brandenburg, York, Burton and Danehower2012b, Reference Chahal, Jordan, York, Brandenburg, Shew, Burton and Danehower2013; Lancaster et al. Reference Lancaster, Jordan and Johnson2008). Efficacy of the graminicides clethodim and sethoxydim may be reduced when applied in tank mixture with broadleaf herbicides such as acifluorfen, bentazon, acifluorfen plus bentazon, phenoxyalkanoic acid, 2,4-DB, or herbicides that inhibit ALS such as imazapic and imazethapyr (Burke and Wilcut Reference Burke and Wilcut2003; Burke et al. Reference Burke, Price, Wilcut, Jordan, Culpepper and Tredaway-Ducar2004; Jordan Reference Jordan1995; York et al. Reference York, Wilcut and Grichar1993), due to reduced absorption, translocation, or metabolism (Ferreira et al. Reference Ferreira, Burton and Coble1995). Weed species, weed size at the time of herbicide application, rates, adjuvants, and environmental conditions can affect the interaction between graminicides and broadleaf herbicides (Burke and Wilcut Reference Burke and Wilcut2003; Jordan Reference Jordan1995). Chlorimuron antagonized clethodim for E. crus-galli and S. halepense control but U. platyphylla control with clethodim was not affected (Jordan Reference Jordan1995). In the same study, Jordan (Reference Jordan1995) reported reduction in E. crus-galli and U. platyphylla control with fluazifop-P when applied in tank mixture with chlorimuron compared with fluazifop-P alone. Also, bentazon antagonized sethoxydim and clethodim efficacy for E. crus-galli, S. halepense, and U. platyphylla control (Jordan Reference Jordan1995). Imazapic in mixture with clethodim reduced clethodim efficacy on D. sanguinalis, E. indica, P. dichotomiflorum, and U. texana, but U. platyphylla was not affected (Burke et al. Reference Burke, Price, Wilcut, Jordan, Culpepper and Tredaway-Ducar2004). Imazapic applied 1 d before and up to 3 d after clethodim reduced efficacy of clethodim on P. dichotomiflorum and D. sanguinalis >30 cm in height (Burke et al. Reference Burke, Price, Wilcut, Jordan, Culpepper and Tredaway-Ducar2004). Similarly, imazapic applied 3 d before and up to 7 d after clethodim reduced E. indica control compared with clethodim alone (Burke et al. Reference Burke, Price, Wilcut, Jordan, Culpepper and Tredaway-Ducar2004). Burke and Wilcut (Reference Burke and Wilcut2003) showed imazapic did not affect absorption or translocation of clethodim in treated E. indica but antagonized imazapic efficacy by reducing the photosynthetic rate of E indica and therefore the sensitivity of ACCase to clethodim (Burke and Wilcut Reference Burke and Wilcut2003). Bentazon and 2,4-DB tank mixed with clethodim reduced P. dichotomiflorum and U. platyphylla control compared with clethodim alone (Burke et al. Reference Burke, Price, Wilcut, Jordan, Culpepper and Tredaway-Ducar2004). In a similar study, U. platyphylla control with clethodim was <65% when applied in mixture with acifluorfen, acifluorfen plus bentazon, imazethapyr, imazapic, or lactofen (Grichar et al. Reference Grichar, Besler, Brewer and Baughman2002). Ammonium sulfate and other adjuvants can be tank mixed to alleviate the antagonistic effect of broadleaf herbicides on the efficacy of clethodim and sethoxydim (Burke et al. Reference Burke, Price, Wilcut, Jordan, Culpepper and Tredaway-Ducar2004; Jordan Reference Jordan1995).
Herbicides with Soil and Foliar Activity—ALS Inhibitors (Chlorimuron, Diclosulam, Imazapic, and Imazethapyr)
The introduction of the ALS inhibitors diclosulam, chlorimuron, imazapic, and imazethapyr is one of the most important developments in the history of weed control in peanut production in the United States. These herbicides provide effective control of monocotyledonous and dicotyledonous weed species and exhibit residual activity for preventing weed seedling emergence and foliar activity for control of emerged weeds (Grey and Wehtje Reference Grey and Wehtje2005; Grey et al. Reference Grey, Bridges, Prostko, Eastin, Johnson, Vencill, Brecke, MacDonald, Ducar, Everest and Wehtje2003). In addition to broad-spectrum weed control, considerable safety to peanut is achieved (Grey and Wehtje Reference Grey and Wehtje2005). These features may explain the peanut growers’ high reliance on these herbicides during the last 20 yr, which in fact favored increased selection pressure and subsequently the evolution of resistance in important and problematic species, including A. palmeri (Clewis et al. Reference Clewis, Everman, Jordan and Wilcut2007; Everman et al. Reference Everman, Clewis, Taylor and Wilcut2006). However, the application of these herbicides is limited by rotational restrictions when rotation to sensitive crops such as cotton is anticipated (Grichar et al. Reference Grichar, Dotray and Sestak1999). Numerous researchers have reported the weed control spectrum of ALS-inhibiting herbicides in peanut (Tables 9 and 10), as these continue to be the backbone of weed control in peanut.
Table 9. Cumulative results on efficacy of acetolactate synthase inhibitors applied preemergence in peanut.
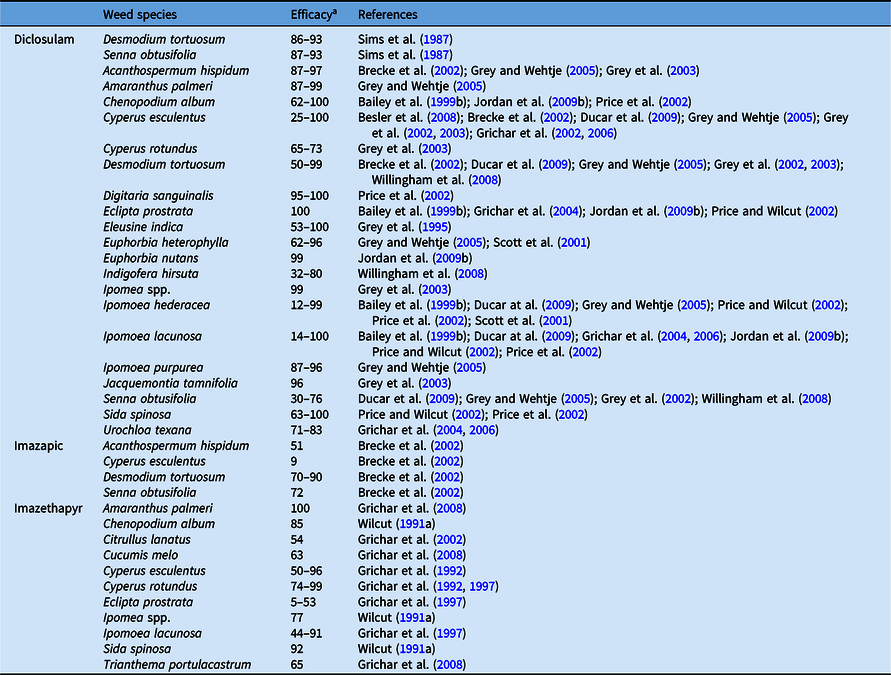
a Efficacy range across all references listed from 0% to 100%, where 0 = no control, 100 = complete control.
Table 10. Cumulative results on the efficacy of acetolactate synthase inhibitors applied postemergence in peanut.
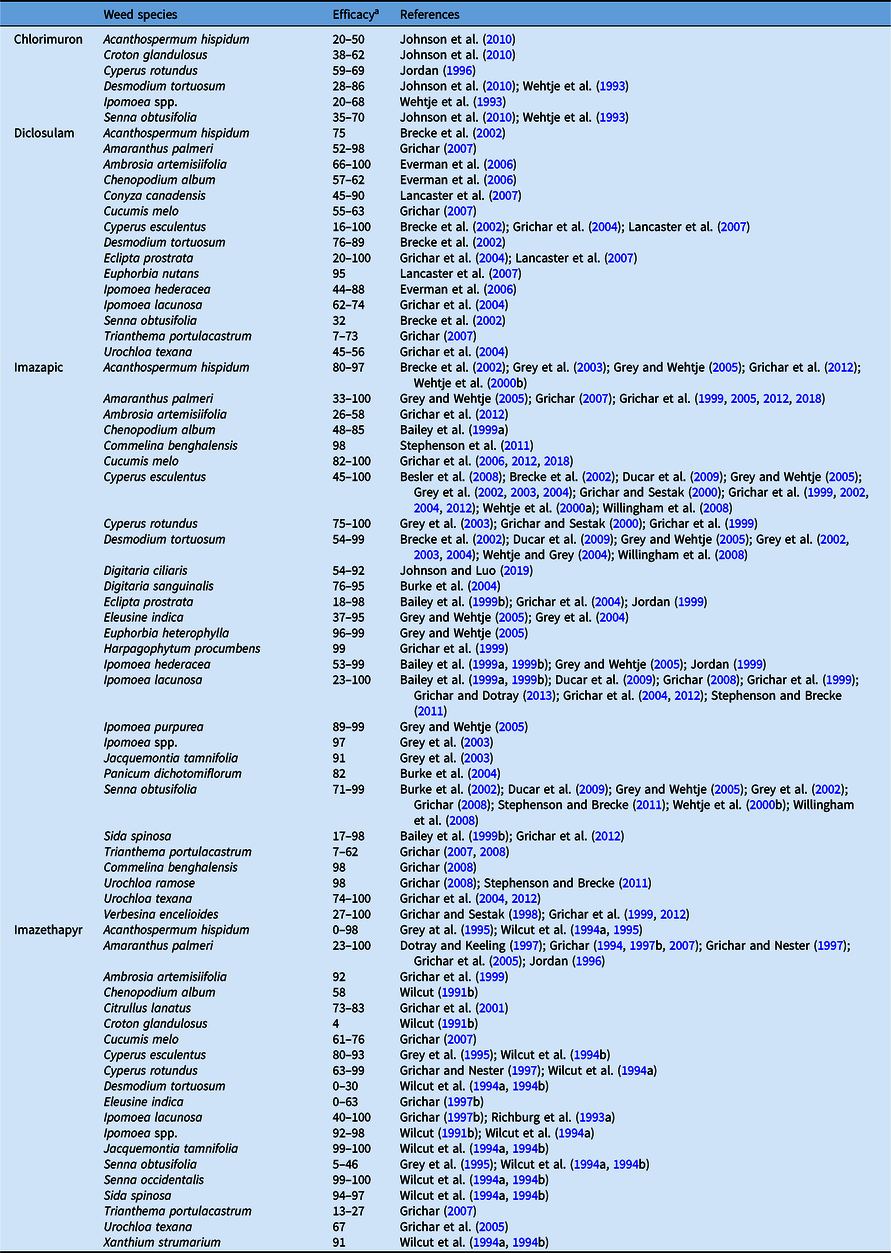
a Efficacy range across all references listed from 0% to 100%, where 0 = no control, 100 = complete control.
Chlorimuron is labeled for late postemergence application in peanut and is primarily used to control D. tortuosum to prevent yield loss and harvest interference (Wehtje et al. Reference Wehtje, Brecke and Martin2000a, Reference Wehtje, Padgett and Martin2000b; Wilcut et al. Reference Wilcut, Richburg, Eastin, Wiley, Walls and Newell1994a). Chlorimuron is restricted to use only from 60 d after emergence to 45 d before peanut harvest (Johnson et al. Reference Johnson, Mullinix and Brown1992a, Reference Johnson, Holbrook, Mullinix and Cardina1992b). During this application window, chlorimuron absorption in peanut is minimal and readily metabolized (Wilcut et al. Reference Wilcut, Wehtje, Patterson, Cole and Hicks1989). However, by 60 d after peanut emergence, D. tortuosum plants are often about 90 to 120 cm (Cardina and Brecke Reference Cardina and Brecke1991), which is significantly larger than the 25-cm height recommended on the chlorimuron label (Anonymous 2009). The application restriction of chlorimuron was established based on peanut injury and yield reductions following early-season applications in studies conducted using ‘Florunner’—a now-obsolete cultivar that has been replaced by newer cultivars in the U.S. Southeast peanut-growing region (Wilcut et al. Reference Wilcut, Wehtje, Patterson, Cole and Hicks1989). However, chlorimuron can cause peanut injury even when applied at the recommended timing (Wehtje and Grey Reference Wehtje and Grey2004). Reduction in peanut growth has been reported following chlorimuron application at the recommended timing, although no consistent reduction in peanut yield was observed (Johnson et al. Reference Johnson, Holbrook, Mullinix and Cardina1992b; Prostko et al. Reference Prostko, Kemerait, Jost, Johnson, Brown and Webster2009). However, studies conducted with newer cultivars such as ‘Georgia-06G’ and ‘Tifguard’ indicated 7% to 11% peanut yield reduction following chlorimuron application at 60 to 99 d after emergence, which is within the recommended window for application (Prostko et al. Reference Prostko, Kemerait and Webster2012). Similarly, Grichar and Dotray (Reference Grichar and Dotray2010) in one of two trials, observed significant yield reduction in ‘Tamrun 96’ peanut cultivar following chlorimuron application at 60 to 95 d after peanut emergence. Additionally, incidence of tomato spotted wilt virus (TSWV) caused by thrips moving from dying weeds was reported following chlorimuron application in ‘AP-3’, ‘Georgia-02C’, and ‘Georgia Green’ peanut cultivars in 15 field trials in Georgia (Prostko et al. Reference Prostko, Kemerait, Jost, Johnson, Brown and Webster2009).
It is also noteworthy that the recommended window of chlorimuron application is later than the 4- to 6-wk critical period of D. tortuosum control in peanut (Hauser et al. Reference Hauser, Buchanan and Ethredge1975). This indicates that an irreversible yield reduction is expected, even if chlorimuron controls D. tortuosum late in the growing season. Wehtje et al. (Reference Wehtje, Brecke and Martin2000a) showed late-season control with chlorimuron after significant early-season interference did not improve peanut yield but resulted in a significant reduction in net return. Research conducted to evaluate the possibility of applying chlorimuron earlier than 60 d after peanut emergence showed that peanut cultivars such as ‘AT 201’ and Georgia Green exhibited acceptable tolerance to chlorimuron applied early in the growing season in three of four trials (Wehtje and Grey Reference Wehtje and Grey2004). In that study, peanut yield reduction from early-season application of chlorimuron was observed only in one of four trials associated with plant stress, which suggests that chlorimuron possesses yield-reducing risk only when the crop has been stressed by other factors (Wehtje and Grey Reference Wehtje and Grey2004). Differences in TSWV tolerance among cultivars may also be a confounding factor in accessing tolerance to chlorimuron. In another study, Johnson et al. (Reference Johnson, Prostko and Davis2010) reported better D. tortuosum control and greater yield in ‘C99R’ and Georgia Green peanut cultivars when chlorimuron was applied at 21 and 35 d after peanut emergence compared with the recommended application window. This suggests that the risk of early-season application of chlorimuron may be worth the potential benefits of controlling D. tortuosum during the critical period of interference when the weeds are smaller in size, particularly in situations with dense populations.
Chlorimuron can be applied in tank mixture with other late-season herbicides, especially 2,4-DB. The interaction of chlorimuron and co-applied herbicides may be beneficial or detrimental to crop safety and weed control depending on the herbicide, application timing, and weed species (Wehtje et al. Reference Wehtje, Wilcut and McGuire1993). The tank mixture of chlorimuron and 2,4-DB improved the control of D. tortuosum, Ipomoea spp., and S. obtusifolia, but peanut injury was not affected (Wehtje et al. Reference Wehtje, Wilcut and McGuire1993). In the same study, a tank mixture of chlorimuron and 2,4-DB improved peanut yield when applied at 11 wk after planting, but yield was reduced when the tank mix was applied at 7 wk after planting (Wehtje et al. Reference Wehtje, Wilcut and McGuire1993).
Diclosulam is a triazolopyrimidine sulfonanilide ALS-inhibiting herbicide (Bailey et al. Reference Bailey, Wilcut, Jordan, Swann and Langston1999a). It is often used in peanut as a preplant-incorporated or preemergence treatment to provide broadleaf and perennial sedge weed control, but it can also be used early postemergence (Baily and Wilcut Reference Bailey and Wilcut2002; Grey and Wehtje Reference Grey and Wehtje2005; Lancaster et al. Reference Lancaster, Beam, Lanier, Jordan and Johnson2007). Diclosulam provides broad-spectrum weed control at a much lower recommended use rate (27 g ha−1) than other herbicides used in peanut, and it is less restrictive compared with other ALS inhibitors with respect to cotton rotational limitations (Anonymous 2000c; Brecke et al. Reference Brecke, Wehtje and Paudel2002). The rotation interval for cotton following imazethapyr and imazapic is 18 mo but it is only 10 mo for diclosulam (Anonymous 2000c). Our systematic review of the literature showed that diclosulam applied preplant incorporated or preemergence provides >70% control of Amaranthus spp., A. artemisiifolia, A. hispidum, C. album, D. tortuosum, D. sanguinalis, E. prostrata, Ipomoea spp., nodding spurge [Chamaesyce nutans (Lag.) Small], S. spinosa, U. texana, V. encelioides, and E. heterophylla in peanut (Tables 4 and 9). However, it does not provide effective control of S. obtusifolia, a major problematic weed in peanut in the United States (Brecke et al. Reference Brecke, Wehtje and Paudel2002; Grey and Wehtje Reference Grey and Wehtje2005; Main et al. Reference Main, Ducar, Whitty and MacDonald2005). Also, control of annual grasses and C. esculentus with diclosulam is inconsistent (Baily and Wilcut Reference Bailey and Wilcut2002; Brecke et al. Reference Brecke, Wehtje and Paudel2002; Grichar et al. Reference Grichar, Dotray and Sestak1999). Some studies reported >80% C. esculentus control with diclosulam applied preplant incorporated or preemergence (Baily and Wilcut Reference Bailey and Wilcut2002; Clewis et al. Reference Clewis, Askew and Wilcut2002; Grey et al. Reference Grey, Bridges, Hancock and Davis2004; Price and Wilcut Reference Price and Wilcut2002; Price et al. Reference Price, Wilcut and Swann2002), whereas others observed <70% control (Brecke et al. Reference Brecke, Wehtje and Paudel2002; Grey et al. Reference Grey, Bridges and Eastin2001; Grichar et al. Reference Grichar, Dotray and Sestak1999; Price and Wilcut Reference Price and Wilcut2002). Variations in C. esculentus control with diclosulam are attributed mainly to differences in application rates, with greater control observed with increasing rate of application (Ducar-Tredaway et al. Reference Ducar-Tredaway, Wilcut, Jordan, Faircloth and Swann2006; Grey et al. Reference Grey, Bridges and Eastin2001; Grichar et al. Reference Grichar, Dotray and Sestak1999), and application method, with preplant-incorporated applications often resulting in more consistent control than preemergence applications (Grey and Wehtje Reference Grey and Wehtje2005; Grey et al. Reference Grey, Bridges and Eastin2001, Reference Grey, Bridges, Hancock and Davis2004; Grichar et al. Reference Grichar, Dotray and Sestak1999; Main et al. Reference Main, Ducar and Macdonald2002). The efficacy of diclosulam applied preemergence depends on the availability of soil moisture from rainfall or irrigation to move the herbicide to the active zone of weed germination (Grey and Wehtje Reference Grey and Wehtje2005; Grey et al. Reference Grey, Bridges, Hancock and Davis2004; Main et al. Reference Main, Ducar and Macdonald2002). Although diclosulam is mostly applied preplant incorporated or preemergence, research has indicated that timely postemergence applications can provide effective control of several problematic weeds in peanut (Brecke et al. Reference Brecke, Wehtje and Paudel2002; Everman et al. Reference Everman, Clewis, Taylor and Wilcut2006; Lancaster et al. Reference Lancaster, Beam, Lanier, Jordan and Johnson2007). Lancaster et al. (Reference Lancaster, Beam, Lanier, Jordan and Johnson2007) reported good to excellent control of A. artemisiifolia, C. esculentus, C. nutans, and I. hederacea following a postemergence application of diclosulam at 3 wk after planting at 9, 13, 18, and 27 g ha−1, but smooth pigweed (Amaranthus hybridus L.) and C. album control was <35%. Diclosulam applied early postemergence controlled A. palmeri at least 85%, whereas control was 75% with late postemergence application at 30 g ai ha−1 (Grichar Reference Grichar2007). Diclosulam applied postemergence at 4, 9, 13, or 27 g ai ha−1 controlled A. artemisiifolia 92% when applied within 7 wk after planting, whereas control was 89% with 13 and 27 g ai ha−1 rates and 63% to 66% with 4 and 9 g ai ha−1 rates when the application was delayed until 9 wk after planting (Everman et al. Reference Everman, Clewis, Taylor and Wilcut2006). Regardless of application rate, I. hederacea control with diclosulam was reduced by >50% when applied at 7 to 9 wk after planting (8- to 10-leaf stage) as compared with early application at 5 wk after planting (Everman et al. Reference Everman, Clewis, Taylor and Wilcut2006).
Peanut cultivars exhibit good tolerance to diclosulam due to their ability to metabolize the herbicide (Bailey and Wilcut Reference Bailey and Wilcut2002; Grey et al. Reference Grey, Bridges and Eastin2001; Grichar et al. Reference Grichar, Dotray and Sestak1999). However, minor, and transient injury characterized by necrosis and peanut stunting has been reported in Florida (Main et al. Reference Main, Ducar and Macdonald2002; Teuton et al. Reference Teuton, Main, MacDonald, Ducar and Brecke2004), Georgia (Grey et al. Reference Grey, Bridges and Eastin2001, Reference Grey, Bridges, Prostko, Eastin, Johnson, Vencill, Brecke, MacDonald, Ducar, Everest and Wehtje2003), North Carolina (Bailey et al. Reference Bailey, Wilcut, Jordan, Swann and Langston1999a, 2000; Price et al. Reference Price, Wilcut and Swann2002), and south Texas (Grichar et al. Reference Grichar, Dotray and Sestak1999). Diclosulam injury resulted in reduced peanut root biomass and canopy diameter (Bailey et al. Reference Bailey, Wilcut, Spears, Isleib and Langston2000; Grey et al. Reference Grey, Dotray and Grichar2007), but yield and grade characteristics are generally not affected in popular Virginia- and runner-type cultivars (Bailey et al. Reference Bailey, Wilcut, Spears, Isleib and Langston2000; Grey et al. Reference Grey, Dotray and Grichar2007; Main et al. Reference Main, Ducar and Macdonald2002, Reference Main, Ducar, Whitty and MacDonald2005). Main et al. (Reference Main, Ducar and Macdonald2002) reported peanut canopy width, yield, and percentage of extra-large kernels were not affected by diclosulam applied preplant incorporated at 18, 27, or 54 g ai ha−1 in peanut runner-type cultivars: Georgia Green, ‘C99R’, and ‘MDR-98’. Similarly, Bailey et al. (Reference Bailey, Wilcut, Spears, Isleib and Langston2000) reported diclosulam applied preplant incorporated at 36 g ai ha−1 did not reduce peanut yields in eight Virginia-type cultivars. Diclosulam applied postemergence at 27 and 54 g ha−1 caused peanut injury ranging from 11% to 30%, but the injury was transient, and peanut yield was not affected (Everman et al. Reference Everman, Clewis, Taylor and Wilcut2006)
Imazapic and imazethapyr are Group 2 imidazolinone herbicides that kill susceptible weed species by inhibiting ALS, an enzyme involved in the biosynthesis of branched-chain amino acids (Senseman Reference Senseman2007). Imazapic and imazethapyr are registered in peanut for annual broadleaf weed and Cyperus spp. control (Richburg et al. Reference Richburg, Wilcut and Wiley1995c). Imazethapyr applied preplant incorporated, preemergence, or postemergence and imazapic applied preemergence or postemergence are used to provide residual weed control in peanut in addition to their foliar activity (Richburg et al. Reference Richburg, Wilcut and Wehtje1994, Reference Richburg, Wilcut and Wiley1995c; Wilcut et al. Reference Wilcut, Richburg, Wiley and Walls1996). Imazethapyr applied preplant incorporated or preemergence at rates ranging from 35 to 72 g ai ha−1 provides >70% control of A. cristata, A. hispidum, C. album, C. lanatus, C. melo, E. prostrata, Ipomoea spp., S. occidentalis, S. spinosa, X. strumarium, and Amaranthus species, including A. palmeri (Tables 4 and 9). Wilcut et al. (Reference Wilcut, Walls and Horton1991b) reported >90% control of A. cristata, E. prostrata, Ipomoea spp., and S. spinosa with preplant-incorporated and preemergence applications of imazethapyr at 71 g ai ha−1. Similarly, postemergence application of imazethapyr provided >90% control of these weed species (Table 10), particularly when applied to small weeds within 10 d of weed emergence (Grey et al. Reference Grey, Wehtje, Walker and Paudel1995; Wilcut et al. Reference Wilcut, Richburg, Eastin, Wiley, Walls and Newell1994a). Regardless of application timing, imazethapyr does not provide good control of D. tortuosum and S. obtusifolia, the two most prevalent and troublesome weeds in peanut in the southeastern United States (Klingaman et al. Reference Klingaman, King and Oliver1992; Richburg Reference Richburg, Wilcut, Culbreath and Kvien1995a, Reference Richburg, Wilcut and Eastin1995b, Reference Richburg, Wilcut and Vencill1996; Wilcut et al. Reference Wilcut, Walls and Horton1991b, Reference Wilcut, Richburg, Eastin, Wiley, Walls and Newell1994a). It also shows poor control of A. artemisiifolia, a prevalent weed species in North Carolina and Virginia peanut fields (York et al. Reference York, Wilcut, Swann, Jordan and Walls1995). Regardless of application method, A. artemisiifolia control with imazethapyr at 70 g ha−1 was not >67% even with sequential applications consisting of preplant incorporated before GC or preemergence followed by postemergence application (York et al. Reference York, Wilcut, Swann, Jordan and Walls1995). Similarly, imazethapyr did not control D. tortuosum and S. obtusifolia adequately with preplant-incorporated (Richburg et al. Reference Richburg, Wilcut and Vencill1996) or early postemergence (Wilcut et al. Reference Wilcut, Richburg, Wiley, Walls, Jones and Iverson1994b) applications, with control generally <50%. In a greenhouse study evaluating differential tolerance of D. tortuosum, S. obtusifolia, and A. retroflexus to imazethapyr, Cole et al. (Reference Cole, Wehtje, Wilcut and Hicks1989) observed reduced root absorption and greater half-life for foliar-applied [14C]imazethapyr in D. tortuosum, and S. obtusifolia compared with A. retroflexus (a susceptible species). This suggests that D. tortuosum and S. obtusifolia tolerance of imazethapyr is based on reduced root absorption of the herbicide when applied preplant incorporated or preemergence and a greater capacity to metabolically inactivate the herbicide when applied postemergence (Cole et al. Reference Cole, Wehtje, Wilcut and Hicks1989).
The efficacy of imazethapyr for weed control in peanut is influenced by weed size; environmental factors; and method, timing, and rates of application (Dotray and Keeling Reference Dotray and Keeling1997; Grichar et al. Reference Grichar, Nester and Colburn1992; Richburg et al. Reference Richburg, Wilcut and Wehtje1993b, Reference Richburg, Wilcut and Vencill1996). As discussed previously, tolerance to imazethapyr is based on differential metabolism among weed species. The amount of imazethapyr metabolized and, subsequently, the efficacy of the herbicide can vary with weed species and the site of uptake, which is influenced by the application method (Wilcut et al. Reference Wilcut, Walls and Horton1991b). Grichar et al. (Reference Grichar, Nester and Colburn1992) reported a greater and more consistent C. esculentus and C. rotundus control with imazethapyr applied preplant incorporated compared with preemergence application. Similarly, York et al. (Reference York, Wilcut, Swann, Jordan and Walls1995) observed a greater (>90%) and more consistent control of A. cristata and S. spinosa with imazethapyr applied preplant incorporated or preemergence compared with postemergence application (<60%). Environmental factors may account for the variation in the efficacy of imazethapyr following different application methods. Exposure to sunlight has been reported to degrade or alter the structure of imazethapyr (Basham and Lavy Reference Basham and Lavy1987), which may explain the reduced efficacy of the herbicide on Cyperus spp. when applied preemergence compared with preplant incorporated application. Furthermore, the efficacy of soil applied imazethapyr depend on the availability of moisture from rainfall or irrigation within a few days of application to activate the herbicide and enhance root absorption (Wilcut et al. Reference Wilcut, Richburg, Eastin, Wiley, Walls and Newell1994a). Also, water stress that enhances the development of thicker cuticles can reduce the uptake of foliar-applied herbicides (Shaner Reference Shaner1989), which may explain the reduced efficacy of imazethapyr applied postemergence compared with preplant-incorporated or preemergence applications. In addition, imazethapyr applied postemergence often does not kill weeds completely but rather causes distorted terminal growth and inhibits further weed development (York et al. Reference York, Wilcut, Swann, Jordan and Walls1995). However, postemergence application of imazethapyr has been shown to provide more consistent weed control in highly susceptible species such as A. hispidum (Wilcut et al. Reference Wilcut, Richburg, Eastin, Wiley, Walls and Newell1994a) and Ipomoea spp. (Grichar et al. Reference Grichar, Nester and Colburn1992) compared with preplant-incorporated or preemergence applications. Postemergence application of imazethapyr provided >80% control of A. hispidum and Ipomoea spp. even at 0.5X the label rate (Richburg et al. Reference Richburg, Wilcut and Wiley1995c). Poor weed control following postemergence application of imazethapyr may also be due to larger weed size (Wilcut et al. Reference Wilcut, Walls and Horton1991b). Efficacy of translocated herbicides is influenced by weed size (York et al. Reference York, Wilcut, Swann, Jordan and Walls1995). Foliar absorption of imidazolinone herbicides is limited by the amount of herbicide that passes through the cuticle (Shaner Reference Shaner1989). Weed species such as A. cristata, C. album, S. spinosa, and S. occidentalis become tolerant of imazethapyr when larger after the 2-leaf growth stage (Wilcut et al. Reference Wilcut, Walls and Horton1991a, Reference Wilcut, Walls and Horton1991b, Reference Wilcut, Richburg, Wiley, Walls, Jones and Iverson1994b).
Peanut has been shown to have excellent tolerance to imazethapyr due to its high capacity for metabolizing the herbicide (Cole et al. Reference Cole, Wehtje, Wilcut and Hicks1989; Grichar et al. Reference Grichar, Sestak and Besler1997). Therefore, peanut injury from imazethapyr is usually minor and transient with no impact on yield and grade characteristics (Jordan et al. Reference Daramola, Iboyi, MacDonald, Kanissery, Singh, Tillman and Devkota2003b; Richburg et al. Reference Richburg, Wilcut and Wiley1995c; Wilcut et al. Reference Wilcut, Walls and Horton1991a, Reference Wilcut, Walls and Horton1991b; York et al. Reference York, Wilcut, Swann, Jordan and Walls1995). Richburg et al. (Reference Richburg, Wilcut and Wiley1995c) observed only 10% peanut injury following postemergence application of imazethapyr at 72 g ha−1. Peanut injury was not >11% in studies conducted with five runner-, three Virginia-, and four Spanish-type peanut cultivars in the southeastern and southwestern United States, although imazethapyr caused a slight reduction in canopy width in the Southeast (Richburg et al. Reference Richburg, Wilcut and Grichar2006). Similarly, peanut injury from imazethapyr was not >11% even at 2X the label rate (140 g ha−1) (York et al. Reference York, Wilcut, Swann, Jordan and Walls1995). However, peanut stunting is associated with cool weather, high humidity, dew, or rainfall occurring during or soon after imazethapyr application due to increased absorption and slowed metabolism, but yield and grade are often not affected (Klingaman et al. Reference Klingaman, King and Oliver1992; Grichar Reference Grichar1997a, Reference Grichar1997b).
Imazethapyr is often tank mixed with acifluorfen, bentazon, metolachlor, and 2,4-DB to increase the weed spectrum controlled and with paraquat to improve D. tortuosum and S. obtusifolia control (Grey et al. Reference Grey, Wehtje, Walker and Paudel1995; Grichar et al. Reference Grichar, Nester and Colburn1992; Wilcut et al. Reference Wilcut, Walls and Horton1991b, Reference Wilcut, Richburg, Eastin, Wiley, Walls and Newell1994a). However, the interaction of imazethapyr and co-applied herbicides may be additive, synergistic, or antagonistic on weed control depending on the targeted weed species and method of application (Grey et al. Reference Grey, Wehtje, Walker and Paudel1995; Grichar et al. Reference Grichar, Nester and Colburn1992). Imazethapyr plus metolachlor as preemergence application improved control of Ipomoea spp. by 14% compared with imazethapyr applied alone (Wilcut et al. Reference Wilcut, Walls and Horton1991b). Control of S. obtusifolia with imazethapyr applied early postemergence was not >24%, whereas imazethapyr plus paraquat provided at least 53% control (Wilcut et al. Reference Wilcut, Richburg, Eastin, Wiley, Walls and Newell1994a). Similarly, D. tortuosum control with early postemergence application of imazethapyr was not >30%, whereas imazethapyr plus paraquat provided 53% to 74% control (Wilcut et al. Reference Wilcut, Richburg, Eastin, Wiley, Walls and Newell1994a). In contrast, early postemergence application of imazethapyr plus paraquat reduced A. hispidum control by 15% compared with imazethapyr applied alone, whereas C. esculentus, C. rotundus, Ipomoea spp., S. halepense, S. occidentalis, S. spinosa, and X. strumarium were not affected (Wilcut et al. Reference Wilcut, Richburg, Eastin, Wiley, Walls and Newell1994a). Grichar et al. (Reference Grichar, Nester and Colburn1992) also reported that the addition of metolachlor to imazethapyr applied preplant incorporated did not result in any improvement in C. esculentus and C. rotundus control.
Imazapic is probably the preferred postemergence herbicide for most peanut growers in the United States. Since its introduction in 1996, imazapic has been widely utilized, because it provides effective control of the most troublesome weed species in peanut without the need to tank mix it with other herbicides (Grey et al. Reference Grey, Bridges, Prostko, Eastin, Johnson, Vencill, Brecke, MacDonald, Ducar, Everest and Wehtje2003). Additionally, it has a longer residual effect compared with other ALS-inhibiting herbicides registered for use in peanut (Richburg et al. Reference Richburg, Wilcut and Wehtje1994). Imazapic applied postemergence provides good (80%) to excellent (99%) control of a wide range of weed species, including A. hispidum, A. palmeri, A. spinosus, C. melo, C. esculentus, C. rotundus, S. obtusifolia, Ipomoea spp., L. lacunosa, S. occidentalis, S. spinosa, U. texana, and V. encelioides in peanut (Table 10). Imazapic controls C. esculentus and C. rotundus better than diclosulam and imazethapyr and also provides suppression of D. tortuosum and S. obtusifolia, which are not adequately controlled by imazethapyr (Grey et al. Reference Grey, Bridges and Eastin2001, Reference Grey, Bridges, Hancock and Davis2004). Imazapic generally provides effective weed control and exhibits excellent safety on peanut when applied postemergence (Grichar and Nester Reference Grichar and Nester1997). However, Richburg et al. (Reference Richburg, Wilcut and Wehtje1994) reported excellent control of C. esculentus and C. rotundus with imazapic regardless of application method (preemergence, postemergence, or preemergence and postemergence), indicating imazapic is readily absorbed by both roots and foliage of targeted weeds (Richburg et al. Reference Richburg, Wilcut and Wehtje1994). Grichar et al. (Reference Grichar, Jordan and Prostko2012) also reported at least 92% control of A. hispidum from imazapic applied preemergence, similar to the control reported from postemergence application in other studies (Table 10). In addition to effective broadleaf weed control, imazapic can suppress or in some instances control annual and perennial grasses such as D. ciliaris, D. sanguinalis, and P. texana (Wilcut et al. Reference Wilcut, York, Grichar and Wehtje1995).
Imazapic’s recommended use rate is 71 g ai ha−1; however, a rate as low as 40 g ai ha−1 provided at least 95% control of A. palmeri, similar to a 50 g ai ha−1 application rate (Grichar Reference Grichar1997a). Similarly, imazapic at 40 g ai ha−1 controlled C. esculentus at least 90% (Grichar and Nester Reference Grichar and Nester1997). In a similar study, imazapic at 1/2X the recommended rate provided at least 82% control of A. hispidum, C. esculentus, C. rotundus, S. occidentalis, S. spinosa, J. tamnifolia, and X. strumarium regardless of application method (preplant incorporated, preemergence, or postemergence) (Richburg et al. Reference Richburg, Wilcut and Wiley1995c; Webster et al. Reference Webster, Wilcut and Coble1997). Ducar et al. (Reference Ducar, Clewis, Wilcut, Jordan, Brecke, Grichar, Johnson and Wehtje2009) also observed >87% I. hederacea control with imazapic applied early postemergence at 1/2X the recommended rate. Although imazapic is one of the most expensive herbicides for weed control in peanut, it provides the greatest net return due to improved weed control when compared with most other herbicides (Grichar et al. Reference Grichar, Besler, Lemon and Brewer2005). However, it does not provide adequate control of A. artemisiifolia, C. album, and E. prostrata (Jordan et al. Reference Jordan, Lancaster, Lanier, Lassiter and Johnson2009a, Reference Jordan, Lancaster, Lanier, Lassiter and Johnson2009b; Wilcut et al. Reference Wilcut, York, Grichar and Wehtje1995). Jordan et al. (Reference Jordan, Lancaster, Lanier, Lassiter and Johnson2009b) reported only 25% and 33% control of C. album and E. prostrata, respectively, from imazapic at 72 g ai ha−1, while Grichar et al. (Reference Grichar, Jordan and Prostko2012) observed <70% control of A. artemisiifolia with imazapic. In addition, control of D. tortuosum with imazapic is inconsistent across years and locations (Richburg et al. Reference Richburg, Wilcut and Wiley1995c, Reference Richburg, Wilcut and Vencill1996; Webster et al. Reference Webster, Wilcut and Coble1997; Wilcut et al. Reference Wilcut, Richburg, Wiley and Walls1996; Willingham et al. Reference Willingham, Brecke, Treadaway-Ducar and MacDonald2008). In some instances, imazapic controlled D. tortuosum up to 90% (Brecke et al. Reference Brecke, Wehtje and Paudel2002; Richburg et al. Reference Richburg, Wilcut and Wiley1995c, Reference Richburg, Wilcut and Vencill1996; Wehtje et al. Reference Wehtje, Padgett and Martin2000b), but control was sometimes <50% in other studies (Grey and Wehtje Reference Grey and Wehtje2005; Richburg et al. Reference Richburg, Wilcut and Wiley1995c, Reference Richburg, Wilcut and Vencill1996; Wilcut et al. Reference Wilcut, Richburg, Wiley and Walls1996). Moisture variability across years and locations and differential weed size were hypothesized as possible reasons for the inconsistent D. tortuosum control with imazapic (Richburg et al. Reference Richburg, Wilcut and Eastin1995b, Reference Richburg, Wilcut and Wiley1995c; Wehtje et al. Reference Wehtje, Brecke and Martin2000a, Reference Wehtje, Padgett and Martin2000b; Wilcut et al. Reference Wilcut, Richburg, Wiley and Walls1996). Wilcut et al. (Reference Wilcut, Richburg, Wiley and Walls1996) reported that D. tortuosum control with imazapic was reduced when the plants were growing under moisture stress conditions compared with plants growing under adequate soil moisture. Reduction in root absorption and, consequently, efficacy of imazapic under drought stress conditions have also been reported with other weed species such as C. esculentus and C. rotundus (Richburg et al. Reference Richburg, Wilcut and Wehtje1994). In a greenhouse experiment, imazapic was less effective on D. tortuosum when the seedlings produced trifoliate rather than unifoliate leaves (Wehtje et al. Reference Wehtje, Padgett and Martin2000b). Although absorption of [14C]imazapic by unifoliate and trifoliate D. tortuosum was not different, Wehtje et al. (Reference Wehtje, Padgett and Martin2000b) observed reduced translocation of [14C]imazapic in trifoliate compared with unifoliate D. tortuosum, which possibly contributed to imazapic tolerance in older D. tortuosum seedlings (Wehtje et al. Reference Wehtje, Brecke and Martin2000a). Similarly, research with other weed species such as A. palmeri, A. hispidum, and Ipomoea spp. showed that control with imazapic is less effective when the weeds are large (Chahal et al. Reference Chahal, Jordan, York and Prostko2011; Lancaster et al. Reference Lancaster, Jordan, York, Burke, Corbin, Sheldon, Wilcut and Monks2005b, Reference Lancaster, Beam, Lanier, Jordan and Johnson2007). Imazapic applied to A. hispidum taller than 4 cm killed only the terminal stem, resulting in rapid plant recovery (Richburg et al. Reference Richburg, Wilcut and Wiley1995c).
Imazapic has been evaluated in tank mixtures with other herbicides such as acifluorfen, bentazon, diclosulam, and paraquat for weed control in peanut with variable results. Imazapic applied in tank mixture with acifluorfen, diclosulam, and 2,4-DB did not affect D. sanguinalis, C. esculentus, C. rotundus, I. hederacea, and I. lacunosa control with imazapic (Jordan et al. Reference Jordan, Lancaster, Lanier, Lassiter and Johnson2009b). Similarly, imazapic applied in combination with bentazon or paraquat did not improve C. esculentus control compared with imazapic alone (Grichar et al. Reference Grichar, Jordan and Prostko2012; Wilcut et al. Reference Wilcut, Richburg, Wiley and Walls1996). However, imazapic in combination with paraquat increased T. portulacastrum control to at least 96% compared with 37% to 45% control from imazapic alone (Grichar et al. Reference Grichar, Jordan and Prostko2012). Co-application of imazapic and 2,4-DB also increased annual grass control in peanut compared with imazapic alone (Clewis et al. Reference Clewis, Everman, Jordan and Wilcut2007). Imazapic in combination with bentazon resulted in reduced D. tortuosum control compared with imazapic applied alone (Wilcut et al. Reference Wilcut, Richburg, Wiley and Walls1996).
Peanut exhibits excellent tolerance to imazapic, with no reports of significant long-term injury or yield reduction in popular Spanish-, Virginia-, and runner-type cultivars (Brecke et al. Reference Brecke, Wehtje and Paudel2002; Ducar et al. Reference Ducar, Clewis, Wilcut, Jordan, Brecke, Grichar, Johnson and Wehtje2009; Grichar et al. Reference Grichar, Besler, Brewer and Langston2004; Wehtje et al. Reference Wehtje, Padgett and Martin2000b) even at 2X the label rate (Brecke et al. Reference Brecke, Wehtje and Paudel2002). Yield, grade, or incidence of TSWV were not affected by imazapic applied at 71 g ha−1 in research conducted with Georgia Green, Georgia 01K, and C99R peanut cultivars (Faircloth and Prostko Reference Faircloth and Prostko2010). However, early-season injury from imazapic ranging from 3% to 23% (Dotray et al. Reference Dotray, Baughman, Keeling, Grichar and Lemon2001; Grey and Wehtje Reference Grey and Wehtje2005; Teuton et al. Reference Teuton, Main, MacDonald, Ducar and Brecke2004; Wilcut et al. Reference Wilcut, Richburg, Wiley and Walls1996) and reduction in canopy width and percentage of jumbo and extra-large kernels in Florunner and ‘Sunrunner’ peanut cultivars has been reported (Richburg et al. Reference Richburg, Wilcut, Culbreath and Kvien1995a).
Herbicide-Resistance Issues
The spread of ALS herbicide– resistant and PPO herbicide-resistant Amaranthus species has been prevalent since the 1990s (Heap Reference Heap2023). The increased incidence of herbicide-resistant weeds is due mainly to the repeated use of herbicides from the same mechanisms of action not only within peanut fields but also in rotational crops and inadequate integration with other forms of weed control (e.g., cultural and mechanical). Most of the postemergence herbicides used in peanut, especially those with both residual and systemic activity, are ALS inhibitors, without which there are only a few alternatives. These herbicides are more susceptible to resistance selection due to their extended residual activity and active-site mutation (Saari et al. Reference Saari, Cotterman and Thill2018). Repeated exposure of weeds to these herbicides led to increased selection pressure and the selection of resistant individuals. There are currently 159 weed species resistant to ALS-inhibiting herbicides, some of which seriously threaten peanut production (Berger et al. Reference Berger, Ferrell, Dittmar and Leon2015; Heap Reference Heap2023). Amaranthus palmeri resistance to ALS-inhibiting herbicides was reported in 21 peanut-growing counties in Georgia (Wise et al. Reference Wise, Grey, Prostko, Vencill and Webster2009) and 97% of the agronomic counties in Florida and North Carolina (Poirier et al. Reference Poirier, York, Jordan, Chandi, Everman and Whitaker2014; Sperry et al. Reference Sperry, Ferrell, Smith, Fernandez, Leon and Smith2017). Resistance to ALS-inhibiting herbicides in A. artemisiifolia has also been confirmed in peanut fields across the southeastern United States (Berger et al. Reference Berger, Ferrell, Dittmar and Leon2015; Chandi et al. Reference Chandi, Jordan, York and Lassiter2012). While there are more options for alternative weed control with herbicides from other mechanisms of action in corn, cotton, and soybean, only a few alternatives, particularly the PPO-inhibiting herbicides, are available in peanut. Although the PPO inhibitors such as acifluorfen, flumioxazin, and lactofen have improved the control of ALS herbicide– resistant weeds in peanut fields, there is a possibility of overusing this group of herbicides, which could result in resistance evolution and, consequently, limited options for weed control in peanut. Resistance to PPO-inhibiting herbicides has been reported in soybean (Heap Reference Heap2023), suggesting that the use of PPO-inhibiting herbicides to manage ALS herbicide– resistant weeds in peanut might not be sustainable. The weed resistance situation is one of the reasons residual herbicides will continue to play a critical role in peanut weed management programs. Although paraquat remains an important option for weed control in peanut, as discussed previously, it is limited to use only within the first 28 d after peanut emergence. It is apparent, therefore, that growers cannot continue to rely only on chemical weed control, which will necessitate the integration of nonchemical weed control methods and a diverse and properly designed integrated weed management program in peanut production systems.
Synthesis, Conclusion, and Future Outlook
Although there is increased advocacy for integrated weed management, herbicides remain the dominant tool for weed management in peanut in the United States, as in most other field crops. Hence, the need to synthesize research results to understand the strengths, weaknesses, and effects of different herbicides to develop optimal, field-specific weed management programs. Several herbicides are available for weed control in peanut. However, our systematic review of the literature showed that no single herbicide application can provide sufficient weed control in all situations due to a narrow window of application, low residual activity, variability in weed control, and rotational restrictions. The chloroacetamide herbicides acetochlor, dimethenamid-P, and S-metolachlor and dinitroanilines such as ethalfluralin and pendimethalin provide residual control of many annual grasses and small-seeded broadleaf weeds in peanut but have limited activity on U. texana, especially in conservation-tillage peanut, which can be problematic. Flumioxazin, a PPO inhibitor, is particularly effective against troublesome broadleaf weeds such as D. tortuosum but is not effective on other important species, including C. esculentus and S. obtusifolia. The photosynthetic inhibitors bentazon and paraquat applied alone or in combination provide postemergence control of a wide range of annual grasses and broadleaf weeds, including dominant and troublesome species such as A. palmeri, D. tortuosum, S. obtusifolia, and Ipomoea spp., but lack residual activity and can only be applied within 28 d after peanut cracking. Similarly, the PPO inhibitors acifluorfen and lactofen provide excellent control of numerous annual broadleaf weeds and have improved control of ALS herbicide– resistant weeds in peanut fields. However, they do not provide residual effects at the rate used postemergence in peanut. The synthetic auxin 2,4-DB provides broadleaf weed control but also lacks residual activity and cannot be applied within 60 d before peanut harvest. Chlorimuron, an ALS inhibitor, provides late postemergence control of several broadleaf weeds, including D. tortuosum and S. obtusifolia, but cannot be applied until 60 d after crop emergence due to phytotoxicity to peanut. Other ALS inhibitors such as diclosulam, imazapic, and imazethapyr also provide effective control of many broadleaf, grassy, and sedge weed species. They have both residual and foliar activity and exhibit considerable safety to peanut. However, crop rotational restrictions must be considered before the application of these herbicides. Graminicides such as clethodim, fluazifop-P, and sethoxydim control annual and perennial grasses but do not control dicot weeds and lack residual activity. Due to these limitations, effective weed control in peanut often requires herbicide mixtures and/or sequential applications of preplant incorporated, preemergence, early postemergence, and/or late postemergence herbicides. Mixture of two or more herbicides in peanut often increases weed control and reduces the number of application trips over the field, saving time and fuel. However, this practice can affect weed control when the tank mix contains herbicides that are not compatible. Depending on the targeted weed species, weed size, application rates, and environmental factors, efficacy of graminicides on grass weed species can be reduced when applied in tank mixture with broadleaf herbicides such as acifluorfen, bentazon, acifluorfen plus bentazon, 2,4-DB, imazapic, and imazethapyr due to reduced absorption and translocation of graminicides. Adjuvants can alleviate the antagonistic effect of broadleaf herbicides on efficacy of graminicides, but the response can be inconsistent with the herbicide chemistry and weed species. Although there are numerous publications on weed control with herbicides in peanut in the United States, there is a substantive range in efficacy and weed spectrum controlled due to variations in environmental conditions and flushes of weed germination across years and locations. Most studies provided soil characteristics of the research location; however, in some cases, background information on weed pressure and detailed weather conditions before, at, and after herbicide application were not provided. This information is important to understand the differences in efficacy or peanut injury among the studies.
Despite the relatively high efficacy of herbicides, the evolution of herbicide-resistant weeds is another area of increasing concern. There are currently 159 weed species resistant to ALS-inhibiting herbicides, some of which seriously threaten peanut production (Berger et al. Reference Berger, Ferrell, Dittmar and Leon2015; Heap Reference Heap2023). The weed resistance situation highlights the need for greater stewardship of the active ingredients available as well as the need for investing in further research of nonchemical alternatives and new effective active ingredients. The available options for nonchemical weed control and their potential limitations in peanut are covered in the first part of this publication series (Daramola et al. Reference Daramola, Iboyi, MacDonald, Kanissery, Singh, Tillman and Devkota2023a). Although integrating herbicides with nonchemical weed management strategies and applying tank mixes of herbicides from various mode of actions is important to reduce the incidence of herbicide-resistant weeds, future research should focus on developing new strategies for preventing or delaying the development of resistance. For long-term effect, these strategies should be addressed within the context of climate change and emerging constraints such as water shortages, drought, and flooding and the effects of rising temperatures and increased CO2 concentration on peanut–weed interactions and herbicide efficacy.
Acknowledgments
This research received no specific grant from any funding agency or the commercial or not-for-profit sectors. No competing interests have been declared.