- 1Department of Clinical Sciences and Community Health, University of Milan, Milan, Italy
- 2Neurosurgery Unit, Fondazione Istituto di Ricovero e Cura a Carattere Scientifico (IRCCS) Ca’ Granda Ospedale Maggiore Policlinico, Milan, Italy
- 3Department of Pathophysiology and Transplantation, University of Milan, Milan, Italy
- 4Department of Biomedical Sciences, Humanitas University, Pieve Emanuele, Italy
- 5Endocrinology and Diabetology Unit, Istituto di Ricovero e Cura a Carattere Scientifico (IRCCS) Humanitas Research Hospital, Rozzano, Italy
- 6Endocrinology Unit, Fondazione Istituto di Ricovero e Cura a Carattere Scientifico (IRCCS) Ca’ Granda Ospedale Maggiore Policlinico, Milan, Italy
The mammalian target of rapamycin (mTOR) inhibitor everolimus has been shown to display antiproliferative effects on a wide spectrum of tumors. In vitro studies demonstrated that everolimus inhibited pituitary neuroendocrine tumor (PitNET) cell growth in a subset of patients. Sensitivity to everolimus is reduced by an escape mechanism that increases AKT phosphorylation (p-AKT), leading to pro-survival pathway activation. Dopamine receptor type 2 (DRD2) mediates a reduction of p-AKT in a subgroup of non-functioning PitNETs (NF-PitNETs) and in prolactin-secreting tumor cells (MMQ cells) through a β-arrestin 2-dependent mechanism. The aim of this study was to investigate the efficacy of everolimus combined with DRD2 agonist cabergoline in reducing NF-PitNET primary cells and MMQ cell proliferation and to evaluate AKT phosphorylation and a possible role of β-arrestin 2. We found that 9 out of 14 NF-PitNETs were resistant to everolimus, but the combined treatment with cabergoline inhibited cell proliferation in 7 out of 9 tumors (-31.4 ± 9.9%, p < 0.001 vs. basal) and reduced cyclin D3 expression. In the everolimus-unresponsive NF-PitNET group, everolimus determined a significant increase of p-AKT/total-AKT ratio (2.1-fold, p < 0.01, vs. basal) that was reverted by cabergoline cotreatment. To investigate the molecular mechanism involved, we used MMQ cells as a model of everolimus escape mechanism. Indeed everolimus did not affect MMQ cell proliferation and increased the p-AKT/total-AKT ratio (+1.53 ± 0.24-fold, p < 0.001 vs. basal), whereas cabergoline significantly reduced cell proliferation (-22.8 ± 6.8%, p < 0.001 vs. basal) and p-AKT. The combined treatment of everolimus and cabergoline induced a reduction of both cell proliferation (-34.8 ± 18%, p < 0.001 vs. basal and p < 0.05 vs. cabergoline alone) and p-AKT/total-AKT ratio (-34.5 ± 14%, p < 0.001 vs. basal and p < 0.05 vs. cabergoline alone). To test β-arrestin 2 involvement, silencing experiments were performed in MMQ cells. Our data showed that the lack of β-arrestin 2 prevented the everolimus and cabergoline cotreatment inhibitory effects on both p-AKT and cell proliferation. In conclusion, this study revealed that cabergoline might overcome the everolimus escape mechanism in NF-PitNETs and tumoral lactotrophs by inhibiting upstream AKT activation. The co-administration of cabergoline might improve mTOR inhibitor antitumoral activity, paving the way for a potential combined therapy in β-arrestin 2-expressing NF-PitNETs or other PitNETs resistant to conventional treatments.
Introduction
In recent years, PI3K–AKT–mTOR inhibitors have been proposed as an alternative therapeutic strategy for tumors resistant to conventional drugs. Indeed mTOR inhibitors, like everolimus, torin-1, or rapamycin, displayed successful results in a wide spectrum of tumors. Specifically, everolimus showed a promising perspective in tumors such as HER2/neu negative advanced breast cancer, bronchial and renal cell carcinoma, unresectable or metastatic neuroendocrine pancreatic tumors, or nonfunctional gastrointestinal and lung-originating ones (1).
The PI3K–AKT–mTOR pathway is known to have a fundamental role in regulating cell proliferation, survival, and metabolism. In particular, this pathway has been found overactivated in pituitary neuroendocrine tumors (PitNETs) compared with normal pituitary (2–4), supporting its contribution to PitNET progression (5).
Non-functioning (NF)-PitNETs cause visual field deficits and neurologic manifestations due to mass spread effects, and even if generally benign, they may invade surrounding structures and present resistance to medical treatments. Surgical tumor debulking is currently considered the first-line approach, and to date, NF-PitNETs are still orphans of effective medical therapy (6, 7).
In vitro studies displayed that mTOR inhibitors induced a reduction of cell growth and viability in primary cultured NF-PitNET cells (8–11). On the other hand, several different mechanisms have been demonstrated in literature to explain how sensitivity to mTOR inhibitors could be reduced in different kinds of tumors (12).
Among these, one of the most outlined is an escape mechanism that induces an upstream increase of AKT phosphorylation levels (p-AKT) through insulin receptor substrate-1 (IRS-1) triggering, leading to pro-survival pathway activation (13). Indeed the PI3K–AKT–mTOR pathway activation results in a negative feedback loop, mediated by the mTOR/S6K-dependent loss of IRS-1 expression (14). This mechanism of negative feedback is lost when mTOR activity is inhibited with everolimus, flowing into an increase of p-AKT levels and thereby in an enhancement of cell proliferation (13, 15).
The inhibition of mTORC1 has been reported to induce AKT activation in numerous cell types (16–18), unveiling the fact that mTOR inhibitors block mTORC1 activity but do not alter mTORC2 assembly, accordingly with the direct phosphorylation of AKT at Ser 473 (19). In this regard, an increase of AKT activity has been shown to be one of the major contributors that diminished mTOR inhibitors’ anticancer activity (20).
This limited efficacy of everolimus due to p-AKT re-activation could be overcome by upstream AKT phosphorylation inhibition.
In this connection, a new molecular mechanism that contributes to confer sensitivity or resistance to dopamine agonists (DAs) in PitNETs was recently revealed (21). More specifically, it was demonstrated that, in a subset of NF-PitNETs and in rat prolactin (PRL)-secreting tumoral cells (MMQ), the treatment with a DRD2-selective agonist (BIM53097) leads to p-AKT inhibition, flowing into a reduction of cell proliferation through a β-arrestin 2-dependent mechanism. Briefly, in this work, it was demonstrated that β-arrestin 2 transfection in NF-PitNETs lacking its expression restored the ability of the dopamine agonist to exert its antimitotic action (21). Moreover, in MMQ cells silenced for β-arrestin 2, an opposite effect of BIM53097 on p-AKT was observed, together with a complete loss of its antiproliferative activity. These data unveiled a new molecular mechanism that contributes to confer sensitivity or resistance to DAs in pituitary tumors. In tumoral lactotrophs and NF-PitNETs, the lack of β-arrestin 2 prevents the inhibitory effect of DRD2 on AKT pathway activation with a consequent resistance to the antimitotic action of DAs.
Based on the above-mentioned premises, the aim of this study was to test the efficacy of cabergoline in increasing the sensitivity of human primary cultured NF-PitNET cells to the antiproliferative effects of everolimus and to investigate the contribution of β-arrestin 2.
Materials and Methods
Cell Cultures
The local ethics committee previously approved the study, and each patient involved gave informed consent to the use of his/her tumor sample. A brief description of the patient and tumor characteristics is reported in Table 1. Human pituitary cells were obtained by trans-sphenoidal surgery from patients with NF-PitNET. The tissues were partially frozen for subsequent molecular analysis and partially enzymatically dissociated as previously described (22). The dispersed cells were cultured in Dulbecco’s modified Eagle’s medium supplemented with 10% fetal bovine serum (FBS), 2 mM glutamine, and antibiotics (Gibco, Invitrogen, Life Technologies Inc., Carlsbad, CA, USA). Rat tumoral pituitary MMQ cells (ATCC CRL-10609) were grown in RPMI medium (Life Technologies, Thermo Fisher, Carlsbad, CA, USA) supplemented with 15% horse serum, 2.5% FBS, 2 mM glutamine, and antibiotics.
Chemicals
Everolimus and cabergoline were both purchased from Sigma-Aldrich. The powders were suspended in sterile dimethyl sulfoxide, stocked at -80°C, and diluted immediately before use in complete culture medium.
Proliferation Assay
Cell proliferation was determined by the colorimetric measurement of 5-bromo-2′-deoxyuridine (BrdU) incorporation during DNA synthesis in proliferating cells as previously described (23). MMQ and NF-PitNET cells were seeded in starved medium in a 96-well polylysine-coated plate at a density of 2 or 5 × 104 cells/well, respectively. The cells were incubated for 72 h in complete medium with cabergoline, everolimus, or their combination. BrdU incorporation in newly synthesized DNA was then allowed at 37°C for 24 h (NF-PitNETs) or 2 h (MMQ). Therefore, the assay was performed in accordance with the instruction of the manufacturer (Cytiva, Life Science, Marlborough, MA, USA).
Western Blot Analysis
MMQ and NF-PitNETs cells were seeded at a density of 3 × 105 cells/well in a 6-well plate for western blot analysis. After 24 h, the cells were treated with cabergoline and everolimus, alone or in combination, for 3 h at 37°C. Total proteins were quantified by bicinchoninic acid assay; 60 µg were separated on SDS/polyacrylamide gels and transferred to a nitrocellulose filter. Total-AKT and cyclin D3 antibodies were used at 1:1,000, phospho-AKT (Ser473) and β-arrestin 2 antibodies at 1:2,000 (Cell Signaling, Danvers, MA, USA). Primary antibodies were incubated overnight at 4°C; secondary antibodies anti-rabbit or anti-mouse (Cell Signaling, Danvers, MA, USA) was used at 1:2,000 at room temperature for 1 h. Anti-GAPDH antibody (Invitrogen, Thermo Fisher, CA, USA) were used at 1:4,000 for 1 h at room temperature. Chemiluminescence was detected using ChemiDOC-IT Imaging System (UVP, Upland, CA, USA) and analyzed with NIH ImageJ software. β-arrestin 2 expression level analysis was performed on frozen samples of human NF-PitNETs.
β-Arrestin 2 Silencing
MMQ cells were silenced with rat β-arrestin 2 smart pool siRNAs and Dharmafect transfection agent 4 (Dharmacon, GE Healthcare Life Sciences, Chicago, IL, USA) as previously described (21). In each experiment, a negative control siRNA (non-targeting sequence without a significant homology to the sequence of human, mouse, or rat transcripts) was used. For each experiment, the silencing efficiency was tested by western blot analysis, and only experiments with at least 70% silencing efficiency were considered.
Transcription Factor Analysis
Total RNA was extracted from human NF-PitNET frozen tissues conserved at -80°C with Trizol Reagent (Life Technologies, Carlsbad, CA, USA) using standard methods. Moreover, 2 μg of total RNA were reverse-transcribed with RevertAid H Minus First Strand cDNA Synthesis Kit (Thermo Fisher Scientific, Whaltam, MA, USA). Then, 1 μl of cDNA was used for PCR with a specific primer amplifying human SF-1 (Fw: 5′-AGCTGCAAGGGCTTCTTCAA-3′; Rw: 5′-GAATCTGTGCCTTCTTCTGC-3′) and DRD2 (Fw: 5′-AGACCAGAACGAGTGCATCA-3′; Rw: 5′-CGCCAAACCAGAGAAGAATG-3′). A GAPDH transcript was used as the housekeeping standard.
Statistical Analysis
The results are expressed as mean ± SD. A paired two-tailed Student’s t-test was used to detect the significance between two series of data. Two-way ANOVA test was used to analyze the significance between two different groups of data. In addition, p <0.05 was accepted as statistically significant.
Results
In Vitro Responsiveness of NF-PitNET Primary Cultures to Cabergoline, Everolimus, and Their Cotreatment
The everolimus treatment was effective in reducing cell proliferation in 5 out of 14 NF-PitNET primary cultured cells (-39.2 ± 25.8% at 1 nM, p < 0.01 vs. basal) (Figure 1A). An everolimus dose was chosen based on literature data (8), and preliminary dose–response experiments were performed in NF-PitNET cultured cells (Supplementary Figure S1A). In unresponsive tumors, no reduction of cell proliferation was observed even at higher doses of everolimus (10 nM, 100 nM, and 1 µM) (Supplementary Figure S1B). Cabergoline incubation determined a reduction of NF-PitNET primary cell proliferation in 5 out of 14 samples (-32 ± 21.2% at 100 nM, p < 0.01 vs. basal, Figure 1B). In total, 4 out of these 5 tumors were responsive to both drugs. In responsive tumors, the cotreatment of everolimus and cabergoline did not enhance the efficacy of the drugs administered singularly (Figures 1A, B). Moreover, 8 out of 14 NF-PitNETs were resistant to both cabergoline and everolimus (Table 2).
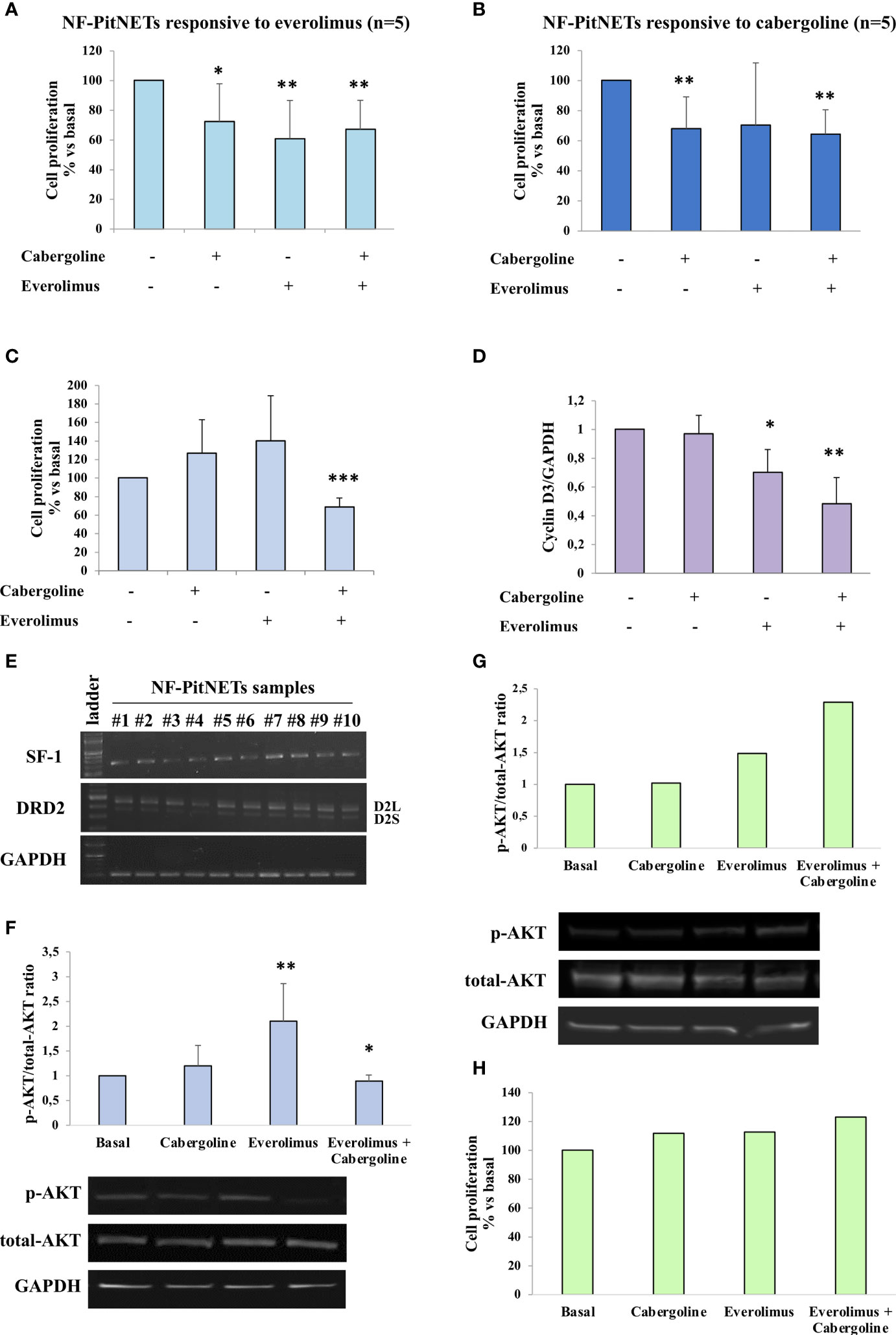
Figure 1 NF-PitNET cell primary culture response to everolimus, cabergoline, and cotreatment on AKT phosphorylation and cell proliferation. Primary cultured of NF-PitNET cells (A–H) were treated with 100 nM cabergoline and 1 nM everolimus for 72 h (A–C, H) or 3 h (D, F, G) at 37°C, administered singularly or in combination. (A–C) BrdU incorporation in newly synthesized DNA was measured. Data represent the mean ± SD normalized vs. respective basal of 14 different NF-PitNETs primary cultures: n = 5 responsive to everolimus (A), n = 5 responsive to cabergoline (B), n = 7 resistant to both drugs but responsive to the cotreatment (C). Each determination was done in triplicate. *p < 0.05, **p < 0.01, ***p < 0.001 vs. corresponding basal. (D) The graph shows the quantification of cyclin D3 normalized to GAPDH (mean value ± SD from 3 primary cultures of NF-PitNETs cells). *p < 0.05, **p < 0.01 vs. corresponding basal. (E) RT-PCR analysis of NF-PitNET samples (n = 10) in order to detect SF-1, D2L, and D2S DRD2 isoform expression. GAPDH expression was analyzed as control. Representative images are shown. (F) The graph shows the quantification of p-AKT/total AKT normalized to the basal. Data represent mean ± SD of 6 different NF-PitNET samples. Representative immunoblots are shown. *p < 0.05; **p < 0.01, vs. corresponding basal. (G, H) NF-PitNET primary cultured cells resistant to cabergoline, everolimus, and cotreatment were analyzed. (G) The graph shows the quantification of p-AKT/total AKT normalized to the basal and representative immunoblots. (H) Measurement of BrdU incorporation in newly synthesized DNA.
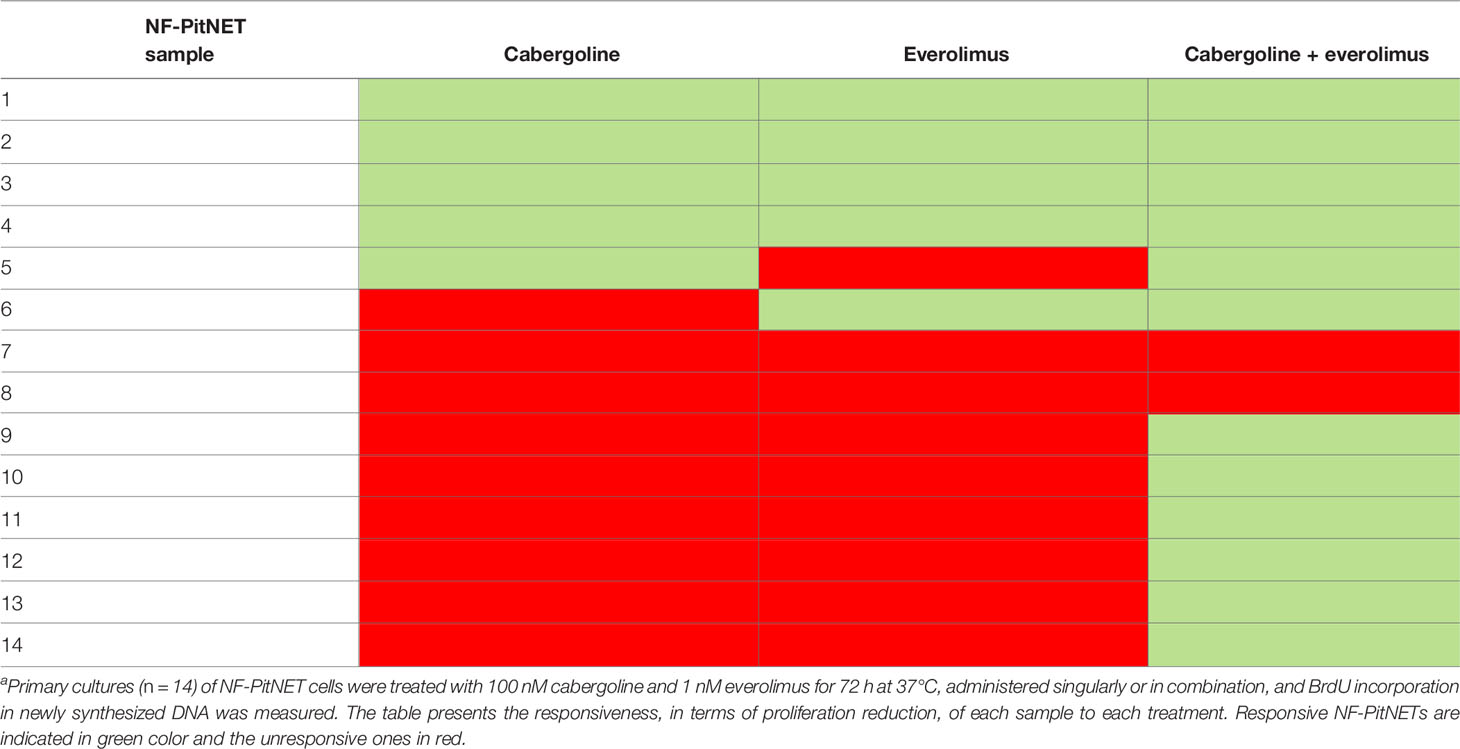
Table 2 NF-PitNETs primary cultured responsiveness to everolimus, cabergoline, and their cotreatment.
Interestingly, in NF-PitNETs resistant to everolimus, the coadministration of cabergoline was effective in inhibiting cell proliferation in 7 out of 9 tumors (-31.4 ± 9.9%, p < 0.001 vs. basal) (Figure 1C and Table 2). Similarly, the combined treatment exerted a strong reduction of cyclin D3 expression (-52 ± 18%, mean of 3 different tumors, p < 0.01 vs. basal, Figure 1D).
In order to establish if NF-PitNET lineage derivation might affect the responsiveness to cabergoline, everolimus, or both, the expression of transcription factor steroidogenic factor-1 (SF-1), marker of a gonadotrophic lineage (24), was evaluated by RT-PCR analysis. Our results showed a positive expression of SF-1 in all tumor samples (Figure 1E). Moreover, all tumors expressed both the long (D2L) and short (D2S) isoforms of DRD2 (Figure 1E).
Everolimus and Cabergoline Effects on AKT Phosphorylation in NF-PitNETs
We then evaluated the AKT activity, testing its phosphorylation status on Ser473 (p-AKT). Our data unveiled that in 6 out of 7 everolimus-unresponsive NF-PitNET group, the 3h everolimus treatment determined a significant increase of the p-AKT/total-AKT ratio (2.1-fold, p < 0.01 vs. basal), and this effect was reverted by cabergoline cotreatment (Figure 1F).
Interestingly, cabergoline was unable to revert the increase of AKT phosphorylation in one everolimus-resistant sample that was also resistant to the antiproliferative effects of the everolimus-and-cabergoline combined treatment, suggesting that the ability to reduce everolimus-dependent AKT phosphorylation is required for the antiproliferative effect (Figures 1G, H).
Cabergoline and Everolimus Cotreatment Reduced Cell Proliferation and AKT Phosphorylation in Everolimus-Resistant MMQ Cells
In MMQ cells, everolimus administration did not affect cell proliferation in a range of doses from 0.1 to 100 nM (Supplementary Figure S1C), while cabergoline inhibited cell growth (-22.8 ± 6.8%, p < 0.001 vs. basal), and a greater inhibition was reached after cabergoline and everolimus coincubation (-34.8 ± 18%, p < 0.001 vs. basal and p < 0.05 vs. cabergoline) (Figure 2A).
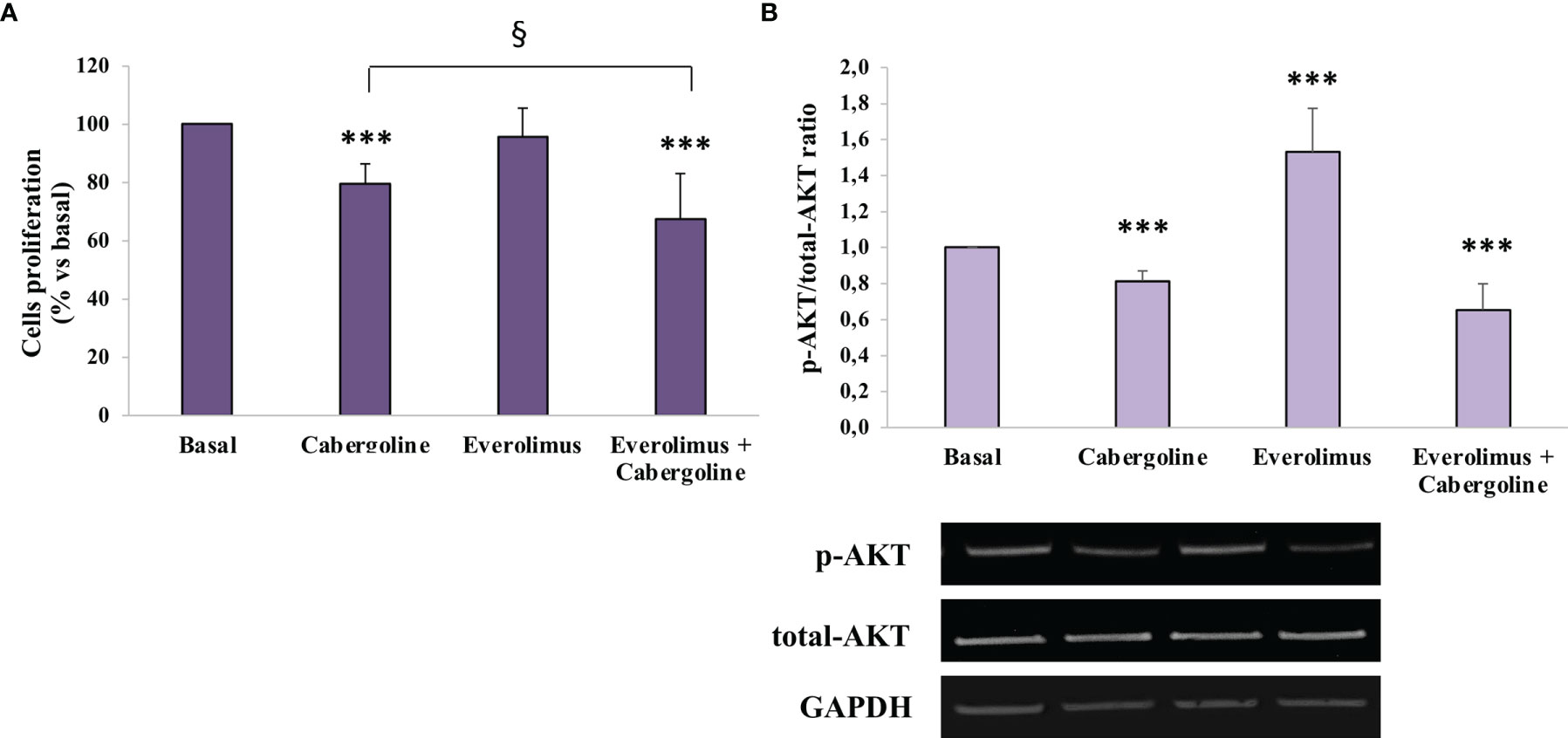
Figure 2 Cabergoline and everolimus cotreatment reduced both cell proliferation and AKT phosphorylation in MMQ cells. MMQ cells were cultured with 100 nM cabergoline and 1 nM everolimus for 72 h (A) or 3 h (B), administered singularly or in combination. (A) BrdU incorporation in newly synthesized DNA was measured. The experiments were repeated 4 times, and each determination was done in quadruplicate. Values represent mean (± SD) normalized vs. respective basal. *** p < 0.001 vs. corresponding basal; § p < 0.05 vs. cabergoline administered alone. (B) The graph shows the quantification of p-AKT on Ser473 normalized to total AKT (mean value ± SD from 4 independent experiments). Representative immunoblots are shown. *** p < 0.001 vs. corresponding basal.
In order to test everolimus’ effects on AKT activity, the AKT phosphorylation levels on Ser473 were evaluated by western blot analysis after 3 h of incubation with everolimus and/or cabergoline. As shown in Figure 3B, everolimus significantly increased the p-AKT/total-AKT ratio (+1.53 ± 0.24-fold, p < 0.001 vs. basal), while cabergoline induced a reduction (-18.6 ± 5.6%, p < 0.001 vs. basal). Cotreatment with cabergoline and everolimus resulted in a strong decrease of p-AKT/total-AKT ratio (-34.5 ± 14%, p < 0.001 vs. basal) (Figure 2B).
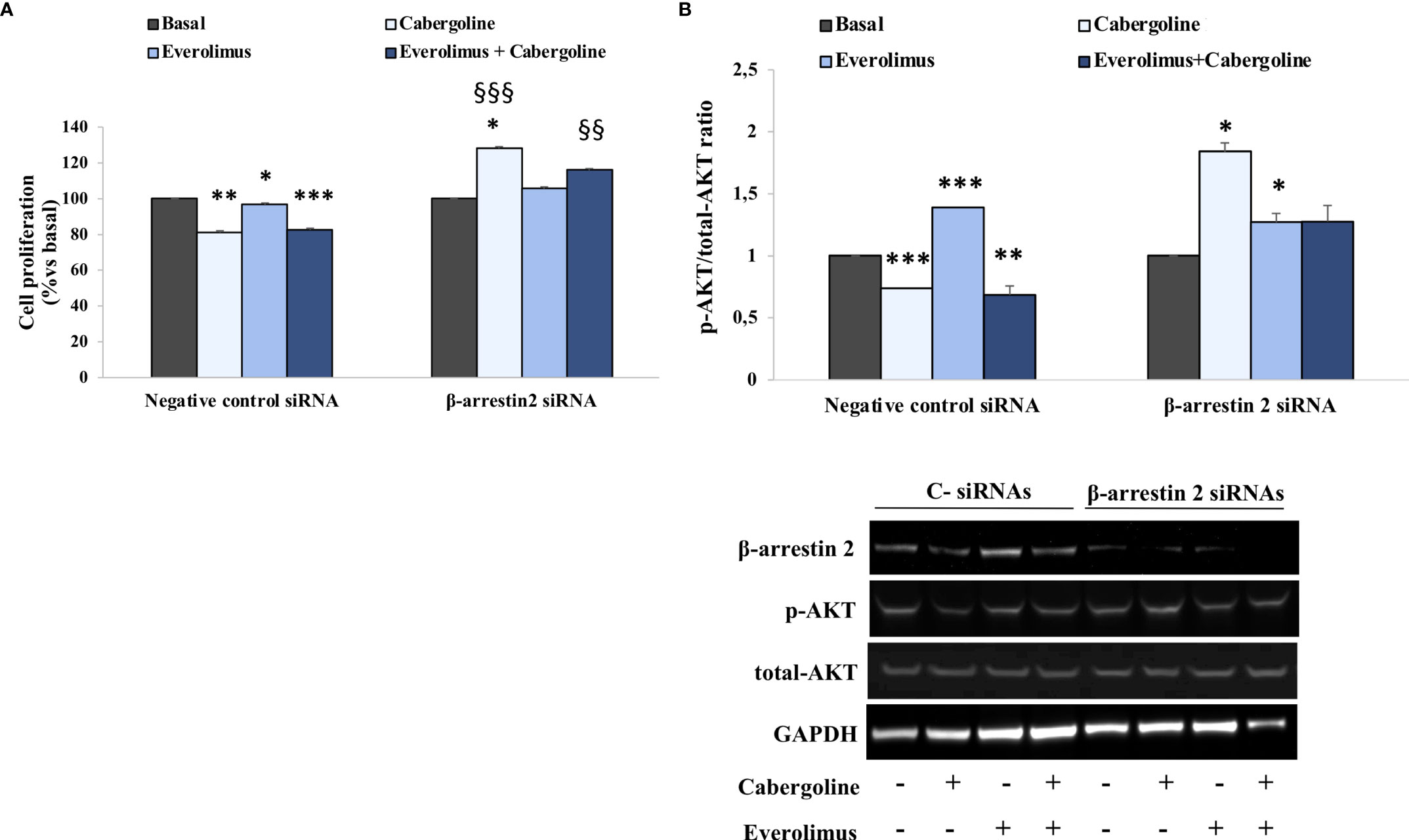
Figure 3 β-arrestin 2 lack reverted everolimus and cabergoline cotreatment ability in reducing both p-AKT and cell proliferation in MMQ cells. The MMQ cells were transiently transfected with β-arrestin 2 siRNAs or negative control siRNAs (C-) for 72 h and then incubated with 100 nM cabergoline and 1 nM everolimus for 72 h (A) or 3 h (B), administered singularly or in combination. (A) BrdU incorporation in newly synthesized DNA was measured. The experiments were repeated 4 times, and each determination was done in quadruplicate. Values represent mean ± SD normalized vs. respective basal. *p < 0.05, ***p < 0.001 vs. corresponding basal, §§ p < 0.01; §§§ p < 0.001 vs. corresponding treated negative control. (B) Densitometrical analysis of p-AKT (Ser473)/total AKT ratio normalized vs. respective basal (mean ± SD from 3 independent experiments), and representative immunoblots are shown. The equal amount of proteins was confirmed by stripping and reprobing with an anti-GAPDH antibody. *p < 0.05, **p < 0.01, ***p < 0.001 vs. corresponding basal.
β-Arrestin 2 Silencing Prevented Everolimus and Cabergoline Cotreatment Inhibitory Effects on Both p-AKT and Cell Proliferation in MMQ Cells
To test a possible involvement of β-arrestin 2 in mediating cabergoline’s inhibition of everolimus-induced AKT phosphorylation, genetic silencing experiments were performed in MMQ cells. Our data show that cell proliferation inhibition induced by cabergoline, both alone and in combination with everolimus, was reverted by β-arrestin 2 silencing (Figure 3A). Accordingly, the lack of β-arrestin 2 prevented the ability of cabergoline to reduce the p-AKT/total-AKT ratio after 3 h of exposure to everolimus (Figure 3B).
In addition, in cells silenced for β-arrestin 2, cabergoline induced a stimulatory effect on AKT according to the observed increase in cell proliferation (Figures 3A, B).
Discussion
This study demonstrated that the cotreatment with the mTOR inhibitor everolimus and DRD2 agonist cabergoline is able to overcome the resistance of a consistent subgroup of NF-PitNETs to everolimus’ antiproliferative effects by preventing the upstream activation of AKT.
This work’s results highlighted that about two-thirds of NF-PitNETs were resistant to everolimus treatment in terms of cell proliferation inhibition, and almost all tumors of this subgroup were also resistant to cabergoline. However, the coadministration of everolimus and cabergoline was able to significantly reduce cell proliferation, while it did not potentiate the effects of each agent singularly administered in responsive tumors.
In agreement, it has been previously shown that a subset of NF-PitNETs is resistant to the antiproliferative in vitro effects of everolimus (8, 11) or rapamycin (10). In particular, Zatelli and co-authors demonstrated that everolimus, used at a higher concentration compared to the present investigation, was effective in reducing cell proliferation in about 70% of NF-PitNETs, and cabergoline did not potentiate the effect of everolimus in responsive tumors (8). Moreover, a study by Rubinfeld et al. unveiled that the limited tumoral responsiveness to mTOR inhibitors in human-derived NF-PitNET cells could be overtaken by combining different kinds of drug or targeting multiple players of the PI3K–AKT–mTOR pathway, emphasizing the cell type-specific effects of these treatments (11). Another work suggested that mTOR inhibitors’ efficacy could be improved by cotreatment with other drugs. Particularly, it was shown that, in NF-PitNETs, octreotide cotreatment potentiated the effects of rapamycin and conferred responsiveness to resistant NF-PitNETs (10).
A lot of potential mechanisms leading to mTOR inhibitor resistance have been described in literature in various kinds of tumors (12)—for instance, earlier studies suggested that tumors with PTEN, FKBP-12, or FKB domain mutations or constitutive PI3K activity displayed scarce responses to mTOR inhibitors. Alterations in protein translation (decreased 4E-BP1 or increased eIF4E) have also been demonstrated to interfere with the effects of mTOR inhibitors on protein synthesis. The stimulation of autophagy and the increased levels of anti-apoptotic molecules, such as Bcl-2, represent additional mechanisms of resistance. Moreover, non-functional apoptotic pathways have been highlighted to potentially confer resistance together with modulation of apoptotic regulators’ stimulation of autophagy and enhanced angiogenesis. In addition, another way can be addressed to the increase in ERK/MAPK signaling, activation of the serin/threonine PIM kinases, the activation status of PDK1, or the altered expression levels of 4E-BP1, a downstream substrate of mTOR, which suppresses eIF4E activity. Furthermore, it was demonstrated that preventing the downregulation of p27Kip1 levels can lead to a less response to this kind of drugs (12). Nevertheless, the increase in AKT activation has been shown to be one of the major contributors that diminished everolimus’ anticancer activity effectiveness, which was induced by an escape mechanism.
Specifically, in different human-derived tumoral cell lines, everolimus blocked mTOR’s ability in inhibiting the ribosomal protein S6 kinase (p706SK), determining an activation of IRS-1 that leads to AKT phosphorylation. This cascade of events flows into an increase of AKT activity and, consequently, into an enhancement of cell proliferation (13).
This suggests that a combined therapy that inhibits mTOR function and prevents AKT activation might have improved the antitumoral activity.
A possible therapeutic potential of DRD2 as a target in PitNETs has been also reported in a previous study in which it was stated that, in ACTH-secreting PitNET cell model, an association of 9-cis retinoic acid and the DRD2 agonist bromocriptine modulates the receptor’s signaling in terms of hormone secretion and cell viability (25).
We have recently demonstrated that the DRD2 agonist cabergoline was effective in inhibiting AKT phosphorylation through a β-arrestin 2-dependent mechanism in tumoral lactotrophs and NF-PitNETs (21). Here we showed that cabergoline improved everolimus’ efficacy by blocking the AKT upstream reactivation.
The AKT phosphorylation levels were analyzed in order to study its activation status after everolimus and cabergoline treatment, singularly and in combination. Our data demonstrated that everolimus-unresponsive NF-PitNETs showed a substantial enhancement of the p-AKT/total-AKT ratio when incubated with everolimus alone, in agreement with the escape mechanism mentioned above. On the other side, the combined treatment with cabergoline strongly reduced this increase of AKT activity, and this ability is correlated with its antimitotic effect. In order to test the relevance of DRD2 expression for NF-PitNETs’ responsiveness to cabergoline and to evaluate the different expressions in DRD2 or its isoforms, long (D2L) and short (D2S), RT-PCR was performed. From our analysis, it emerged that all tumors positively expressed both isoforms of DRD2, regardless of responsiveness to dopamine agonist, ruling out a possible a role of both specific DRD2 isoforms in mediating opposite effects on the everolimus escape mechanism. Our results are in accordance with the observation that the clinical sensitivity to DAs was not associated with the expression of DRD2 or its isoforms (26).
In this sense, we might assume that everolimus’ poor efficacy in NF-PitNETs might be caused by the loss of the negative upstream feedback on AKT, thereby determining the resistance to treatment.
An effect of cabergoline in potentiating the effect of everolimus administration by a reduction of the mTOR inhibitor-induced escape mechanisms was previously reported in lung carcinoid tumoral cells (27).
In PRL-secreting PitNET MMQ cells, cabergoline determined a significant reduction of both cell proliferation and the p-AKT/total-AKT ratio as previously reported (21). On the contrary, everolimus was unable to reduce cell proliferation due to a significant increase of AKT phosphorylation. This effect was reverted by the combined treatment with cabergoline. The co-incubation with everolimus and cabergoline significantly induced a reduction of both cell proliferation and the p-AKT/total-AKT ratio with respect to cabergoline treatment alone, suggesting a possible synergic effect. These observations allow us to consider MMQ cells as a model of “everolimus escape”. Conversely, in rat somatolactotroph tumoral GH3 cells that are responsive to everolimus’ antiproliferative effects, no increase of AKT activity was detected after the everolimus treatment (28). With regard to this, a previous study demonstrated that, in aggressive PRL-PitNETs, everolimus exhibited an antiproliferative action in vitro, suggesting it as a novel therapeutic option in PRL-PitNETs resistant to conventional therapy with cabergoline. Moreover, they demonstrated how the everolimus and cabergoline combination determined in vivo tumor size reduction and PRL level normalization (29).
In addition, we demonstrated the involvement of β-arrestin 2 in mediating cabergoline’s inhibition of AKT activity and cell proliferation after everolimus cotreatment.
Genetic silencing experiments in MMQ cells revealed that the lack of β-arrestin 2 reverted the antimitotic effect induced by the combined treatment with everolimus and cabergoline as well as the inhibition of AKT. It should be noted that MMQ cells only express D2L (21, 30), and studies in literature seem to attribute the postsynaptic phenomena to D2L, such as AKT regulation (31).
However, a specific role of DRD2 isoforms in β-arrestin 2 recruitment has not yet been specifically investigated.
In conclusion, the present study revealed that cabergoline might overcome the everolimus escape mechanism in NF-PitNETs and tumoral lactotrophs by inhibiting the upstream AKT activation. The coadministration of cabergoline might improve mTOR inhibitors’ antitumoral activity, paving the way for a potential combined therapy in β-arrestin 2-expressing NF-PitNETs or other PitNETs resistant to conventional treatments.
Data Availability Statement
The raw data supporting the conclusions of this article will be made available by the authors without undue reservation.
Author Contributions
FM: conceptualization, methodology, investigation, data curation, writing—original draft, writing—review and editing, and formal analysis. EE, DT, RC, GM, GDM, and AMB: investigation. ML and AL: resources, review, and editing; AM, AS, and MA: review and editing. EP: conceptualization, validation, data curation, funding acquisition, supervision, writing—original draft, writing—review and editing, project administration, and formal analysis. GM: conceptualization, supervision, funding acquisition, project administration, and writing—review and editing.
Funding
This work was supported by Associazione Italiana Ricerca Cancro grant to GM (IG 2017-20594), Italian Ministry of Health grant to GM (PE-2016-02361797), and Ricerca Corrente Funds from the Italian Ministry of Health and Progetti di Ricerca di Interesse Nazionale grant to EP (2017N8CK4K).
Conflict of Interest
The authors declare that the research was conducted in the absence of any commercial or financial relationships that could be construed as a potential conflict of interest.
Publisher’s Note
All claims expressed in this article are solely those of the authors and do not necessarily represent those of their affiliated organizations, or those of the publisher, the editors and the reviewers. Any product that may be evaluated in this article, or claim that may be made by its manufacturer, is not guaranteed or endorsed by the publisher.
Supplementary Material
The Supplementary Material for this article can be found online at: https://www.frontiersin.org/articles/10.3389/fendo.2022.867822/full#supplementary-material
References
1. Hasskarl J. Everolimus. Recent Results Cancer Res (2018) 211:101–23. doi: 10.1007/978-3-319-91442-8_8
2. Musat M, Korbonits M, Kola B, Borboli N, Hanson MR, Nanzer AM, et al. Enhanced Protein Kinase B/AKT Signalling in Pituitary Tumours. Endocr Relat Cancer (2005) 2):423–33. doi: 10.1677/erc.1.00949
3. Xu M, Ahorts-Cary L, Knox AJ, Kleinsmidt-DeMasters B, Lillehei K, Wierman ME. Epidermal Growth Factor Receptor Pathway Substrate 8 Is Ovexpressed in Human Pituitary Tumors: Role in Proliferation and Survival. Endocrinology (2009) 150:2064–71. doi: 10.1210/en.2008-1265
4. Noh TW, Jeong HJ, Lee MK, Kim TS, Kim SH, Lee EJ. Predicting Recurrence of Nonfunctioning Pituitary Adenomas. J Clin Endocrinol Metab (2009) 94(11):4406–13. doi: 10.1210/jc.2009-0471
5. Monsalves E, Juraschka K, Tateno T, Agnihotri S, Asa SL, Ezzat S, et al. The PI3K/AKT/mTOR Pathway in the Pathophysiology and Treatment of Pituitary Adenomas. Endocr Relat Cancer (2014) 21(4):R331–344. doi: 10.1530/ERC-14-0188
6. Esposito D, Olsson DS, Ragnarsson O, Buchfelder M, Skoglund T, Johannsson G. Non-Functioning Pituitary Adenomas: Indications for Pituitary Surgery and Post-Surgical Management. Pituitary (2019) 22(4):422–34. doi: 10.1007/s11102-019-00960-0
7. Ntali G, Wass JA. Epidemiology, Clinical Presentation and Diagnosis of non-Functioning Pituitary Adenomas. Pituitary (2018) 21(2):111–8. doi: 10.1007/s11102-018-0869-3
8. Zatelli MC, Minoia M, Filieri C, Tagliati F, Buratto M, Ambrosio MR, et al. Effect of Everolimus on Cell Viability in Nonfunctioning Pituitary Adenomas. J Clin Endocrinol Metab (2010) 95(2):968–76. doi: 10.1210/jc.2009-1641
9. Lee M, Wiedemann T, Gross C, Leinhäuser I, Roncaroli F, Braren R, et al. Targeting PI3K/mTOR Signaling Displays Potent Antitumor Efficacy Against Nonfunctioning Pituitary Adenomas. Clin Cancer Res (2015) 21(14):3204–15. doi: 10.1158/1078-0432.CCR-15-0288
10. Cerovac V, Monteserin-Garcia J, Rubinfeld H, Buchfelder M, Losa M, Florio T, et al. The Somatostatin Analogue Octreotide Confers Sensitivity to Rapamycin Treatment on Pituitary Tumor Cells. Cancer Res (2010) 70(2):666–74. doi: 10.1158/0008-5472.CAN-09-2951
11. Rubinfeld H, Cohen O, Kammer A, Yang G, Cohen ZR, Hadani M, et al. Combination of mTOR Inhibitors Augments Potency While Activating PI3K Signaling in Pituitary Tumors. Neuroendocrinology (2016) 103(5):592–604. doi: 10.1159/000442205
12. Carew JS, Kelly KR, Nawrocki ST. Mechanisms of mTOR Inhibitor Resistance in Cancer Therapy. Target Oncol (2011) 6(1):17–27. doi: 10.1007/s11523-011-0167-8
13. O'Reilly KE, Rojo F, She QB, Solit D, Mills GB, Smith D, et al. mTOR Inhibition Induces Upstream Receptor Tyrosine Kinase Signaling and Activates Akt. Cancer Res (2006) 66(3):1500–8. doi: 10.1158/0008-5472
14. Oldham S, Hafen E. Insulin/IGF and Target of Rapamycin Signaling: A TOR De Force in Growth Control. Trends Cell Biol (2003) 13(2):79–85. doi: 10.1016/s0962-8924(02)00042-9
15. Shi Y, Yan H, Frost P, Gera J, Lichtenstein A. Mammalian Target of Rapamycin Inhibitors Activate the AKT Kinase in Multiple Myeloma Cells by Up-Regulating the Insulin-Like Growth Factor Receptor/Insulin Receptor Substrate-1/Phosphatidylinositol 3-Kinase Cascade. Mol Cancer Ther (2005) 4(10):1533–40. doi: 10.1158/1535-7163.MCT-05-0068
16. Bhatt AP, Bhende PM, Sin SH, Roy D, Dittmer DP, Damania B. Dual Inhibition of PI3K and mTOR Inhibits Autocrine and Paracrine Proliferative Loops in PI3K/Akt/mTOR-Addicted Lymphomas. Blood (2010) 115(22):4455–63. doi: 10.1182/blood-2009-10-251082
17. Kharas MG, Janes MR, Scarfone VM, Lilly MB, Knight ZA, Shokat KM, et al. Ablation of PI3K Blocks BCR-ABL Leukemogenesis in Mice, and a Dual PI3K/mTOR Inhibitor Prevents Expansion of Human BCR-ABL+ Leukemia Cells. J Clin Invest (2017) 127(6):2438. doi: 10.1172/JCI95182
18. Tamburini J, Chapuis N, Bardet V, Park S, Sujobert P, Willems L, et al. Mammalian Target of Rapamycin (mTOR) Inhibition Activates Phosphatidylinositol 3-Kinase/Akt by Up-Regulating Insulin-Like Growth Factor-1 Receptor Signaling in Acute Myeloid Leukemia: Rationale for Therapeutic Inhibition of Both Pathways. Blood (2008) 111(1):379–82. doi: 10.1182/blood-2007-03-080796
19. Sarbassov DD, Guertin DA, Ali SM, Sabatini DM. Phosphorylation and Regulation of Akt/PKB by the rictor-mTOR Complex. Science (2005) 307(5712):1098–101. doi: 10.1126/science.1106148
20. Gupta M, Ansell SM, Novak AJ, Kumar S, Kaufmann SH, Witzig TE. Inhibition of Histone Deacetylase Overcomes Rapamycin-Mediated Resistance in Diffuse Large B-Cell Lymphoma by Inhibiting Akt Signaling Through Mtorc2. Blood (2009) 114(14):2926–35. doi: 10.1182/blood-2009-05-220889
21. Mangili F, Giardino E, Treppiedi D, Barbieri AM, Catalano R, Locatelli M, et al. Beta-Arrestin 2 Is Required for Dopamine Receptor Type 2 Inhibitory Effects on AKT Phosphorylation and Cell Proliferation in Pituitary Tumors. Neuroendocrinology (2021) 111(6):568–79. doi: 10.1159/000509219
22. Peverelli E, Olgiati L, Locatelli M, Magni P, Fustini MF, Frank G, et al. The Dopamine-Somatostatin Chimeric Compound BIM-23A760 Exerts Antiproliferative and Cytotoxic Effects in Human non-Functioning Pituitary Tumors by Activating ERK1/2 and P38 Pathways. Cancer Lett (2010) 288(2):170–6. doi: 10.1016/j.canlet.2009.06.034
23. Peverelli E, Giardino E, Treppiedi D, Meregalli M, Belicchi M, Vaira V, et al. Dopamine Receptor Type 2 (DRD2) and Somatostatin Receptor Type 2 (SSTR2) Agonists are Effective in Inhibiting Proliferation of Progenitor/Stem-Like Cells Isolated From Nonfunctioning Pituitary Tumors. Int J Cancer (2017) 140(8):1870–80. doi: 10.1002/ijc.30613
24. Lopes MBS. The 2017 World Health Organization Classification of Tumors of the Pituitary Gland: A Summary. Acta Neuropathol (2017) 134(4):521–35. doi: 10.1007/s00401-017-1769-8
25. Occhi G, Regazzo D, Albiger NM, Ceccato F, Ferasin S, Scanarini M, et al. Activation of the Dopamine Receptor Type-2 (DRD2) Promoter by 9-Cis Retinoic Acid in a Cellular Model of Cushing's Disease Mediates the Inhibition of Cell Proliferation and ACTH Secretion Without a Complete Corticotroph-To-Melanotroph Transdifferentiation. Endocrinology (2014) 155(9):3538–49. doi: 10.1210/en.2013-1820
26. Greenman Y, Cooper O, Yaish I, Robenshtok E, Sagiv N, Jonas-Kimchi T, et al. Treatment of Clinically Nonfunctioning Pituitary Adenomas With Dopamine Agonists. Eur J Endocrinol (2016) 175(1):63–72. doi: 10.1530/EJE-16-0206
27. Pivonello C, Rousaki P, Negri M, Sarnataro M, Napolitano M, Marino FZ, et al. Effects of the Single and Combined Treatment With Dopamine Agonist, Somatostatin Analog and mTOR Inhibitors in a Human Lung Carcinoid Cell Line: An In Vitro Study. Endocrine (2017) 56(3):603–20. doi: 10.1007/s12020-016-1079-2
28. Gorshtein A, Rubinfeld H, Kendler E, Theodoropoulou M, Cerovac V, Stalla GK, et al. Mammalian Target of Rapamycin Inhibitors Rapamycin and RAD001 (Everolimus) Induce Anti-Proliferative Effects in GH-Secreting Pituitary Tumor Cells In Vitro. Endocr Relat Cancer (2009) 16(3):1017–27. doi: 10.1677/ERC-08-0269
29. Zhang D, Way JS, Zhang X, Sergey M, Bergsneider M, Wang MB, et al. Effect of Everolimus in Treatment of Aggressive Prolactin-Secreting Pituitary Adenomas. J Clin Endocrinol Metab (2019) 104(6):1929–36. doi: 10.1210/jc.2018-02461
30. Ventra C, Meucci O, Grimaldi M, Scorziello A, Porcellini A, Schettini G. Absence of D2S Dopamine Receptor in the Prolactin-Secreting MMQ Pituitary Clone: Characterization of a Wild D2L Receptor Coupled to Native Transduction Mechanisms. J Mol Endocrinol (1995) 14(3):375–89. doi: 10.1038/onc.2016.264
Keywords: pituitary neuroendocrine tumors, mTOR inhibitors, everolimus, AKT phosphorylation, dopamine receptor type 2, cabergoline
Citation: Mangili F, Esposito E, Treppiedi D, Catalano R, Marra G, Di Muro G, Barbieri AM, Locatelli M, Lania AG, Mangone A, Spada A, Arosio M, Peverelli E and Mantovani G (2022) DRD2 Agonist Cabergoline Abolished the Escape Mechanism Induced by mTOR Inhibitor Everolimus in Tumoral Pituitary Cells. Front. Endocrinol. 13:867822. doi: 10.3389/fendo.2022.867822
Received: 01 February 2022; Accepted: 04 April 2022;
Published: 03 June 2022.
Edited by:
Antonino Belfiore, University of Catania, ItalyReviewed by:
Teresa Gagliano, University of Udine, ItalyFederica Barbieri, University of Genoa, Italy
Filippo Ceccato, University of Padua, Italy
Copyright © 2022 Mangili, Esposito, Treppiedi, Catalano, Marra, Di Muro, Barbieri, Locatelli, Lania, Mangone, Spada, Arosio, Peverelli and Mantovani. This is an open-access article distributed under the terms of the Creative Commons Attribution License (CC BY). The use, distribution or reproduction in other forums is permitted, provided the original author(s) and the copyright owner(s) are credited and that the original publication in this journal is cited, in accordance with accepted academic practice. No use, distribution or reproduction is permitted which does not comply with these terms.
*Correspondence: Erika Peverelli, erika.peverelli@unimi.it