Abstract
Objective
To compare pressure–volume (P–V) curves obtained with the Galileo ventilator with those obtained with the CPAP method in patients with acute lung injury (ALI) or acute respiratory distress syndrome (ARDS).
Design
Prospective, observational study.
Setting
General critical care center.
Patients and participants
Patients with ALI/ARDS and receiving mechanical ventilation.
Interventions
Pressure–volume curves were obtained in random order with the CPAP technique and with the software PV Tool-2 (Galileo ventilator).
Measurements and results
In ten consecutive patients, airway pressure was measured by a pressure transducer and changes in lung volume were measured by respiratory inductive plethysmography. P–V curves were fitted to a sigmoidal equation with a mean R 2 of 0.994 ± 0.003. Intraclass correlation coefficients were all >0.75 (P < 0.001 at all pressure levels). Lower (LIP) and upper inflection (UIP), and deflation maximum curvature (PMC) points calculated from the fitted variables showed a good correlation between methods with intraclass correlation coefficients of 0.98 (0.92, 0.99), 0.92 (0.69, 0.98), and 0.97 (0.86, 0.98), respectively (P < 0.001 in all cases). Bias and limits of agreement for LIP (0.51 ± 0.95 cmH2O; −1.36 to 2.38 cmH2O), UIP (0.53 ± 1.52 cmH2O; −2.44 to 3.50 cmH2O), and PMC (−0.62 ± 0.89 cmH2O; −2.35 to 1.12 cmH2O) obtained with the two methods in the same patient were clinically acceptable. No adverse effects were observed.
Conclusion
The PV Tool-2 built into the Galileo ventilator is equivalent to the CPAP method for tracing static P–V curves of the respiratory system in critically ill patients receiving mechanical ventilation.
Similar content being viewed by others
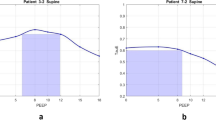
Avoid common mistakes on your manuscript.
Introduction
Pressure–volume (P–V) curves analyze static mechanical properties of the respiratory system by relating airway pressure in no-flow conditions with lung volume at the same pressure [1]. Selecting a positive end-expiratory pressure (PEEP) above the lower inflection point (LIP) improves survival in patients with acute lung injury (ALI) or acute respiratory distress syndrome (ARDS) [2, 3] and lessens the inflammatory response in ARDS patients [4]. Similarly, airway plateau pressure is a determinant of lung injury in ARDS patients [5]. Therefore, the second point of maximum curvature, the upper inflection point, (UIP) should be the maximum plateau pressure [6]. Moreover, the point of maximum curvature (PMC) on the deflation limb of the curve could be used to adjust PEEP to avoid repeated end-expiratory alveolar collapse [7–9].
We proposed the continuous positive airway pressure (CPAP) method to trace inspiratory and expiratory P–V curves. This method is simple, does not require ventilator disconnection, and concorded well with the super-syringe method [10]. The manufacturer Hamilton developed the PV Tool-2 built into the Galileo ventilator (Hamilton Medical) to trace static P–V curves of the entire respiratory system. The Galileo ventilator’s built-in PV Tool-2 simplifies P–V curve tracing and enables physicians without specific training to elaborate P–V curves; thus, it is important to know the accuracy of this method before incorporating it into clinical practice. We hypothesized that P–V curves traced with the PV Tool-2 method at slow pressure/time ramp (3 cmH2O/s) would be equivalent to P–V curves traced with the static CPAP method in mechanically ventilated patients with ALI/ARDS. Preliminary results of this study have been reported in abstract form [11].
Materials and methods
Patients
Informed, written, next-of-kin consent was obtained for all patients. We enrolled ten consecutive, intubated, mechanically ventilated (Servo Ventilator 900 C, Siemens) patients who met the American-European Consensus Conference criteria for ALI/ARDS [12]. Exclusion criteria were age under 18 years, pregnancy, intracranial hypertension, hemodynamic instability, chest wall abnormalities, or air leaks. Patients were deeply sedated with midazolam and morphine and relaxed with vecuronium bromide if needed [13]. ECG, invasive blood pressure, and pulse oximetry were continuously monitored (Hewlett Packard M1166A). Airway pressure was measured by pressure transducer (MP45, Valydine). End-expiratory lung volume was measured by respiratory inductive plethysmography (RIP) (NIMS) with a thoracic strip. RIP was calibrated by performing a linear procedure that included a first point at functional residual capacity and a second point at the end of inspiration. Once calibrated, RIP measurements were checked by comparing the volume values obtained by RIP and flow integration during tidal ventilation (mean tidal volume, 419 ± 132 mL vs. 444 ± 147 mL measured using RIP and the pneumotachograph, respectively, R 2 = 0.96). PEEP was not applied during calibration. A data acquisition system (Windaq 200, Data Q) connected to each monitor allowed analog-to-digital conversion and storage of pressure and RIP signals sampled at 100 Hz. After 10 min baseline ventilation and stabilization, P–V curves were traced in random order with the CPAP technique and with the PV Tool-2, separated by a 10-min period to restore baseline conditions.
P–V curves with CPAP technique
After volume history standardization (three 10 ml/kg breaths), the Servo Ventilator 900 C was switched to CPAP mode, a complete exhalation, until expiratory flow reached zero (6–10 s) was performed, and the expiratory volume measured with RIP was recorded. Airway pressure was raised from 0 to 35 cmH2O in 5 cmH2O steps. Then, CPAP was decreased from 35 to 0 cmH2O. At each step, airway pressure and volume were recorded. To ensure static conditions, each change in airway pressure was effected only when flow reached zero.
P–V curves with the PV Tool-2 built into the Galileo ventilator
This method is based on a linear, pressure-controlled ramp, adjustable for ramp speed and maximum pressure. After volume history standardization, we used a pressure ramp of 3 cmH2O/s and a maximum pressure of 35 cmH2O. Flow was measured using the ventilator’s proximal pneumotachograph (PN279331, Hamilton Medical) inserted between the endotracheal tube and the Y-piece. Volume was obtained from integration of flow. Pressure, flow, and volume data were recorded in a personal computer. At the start of the maneuver, the ventilator performs a prolonged exhalation that lasts for five expiratory time constants to achieve functional residual capacity. In order to study the influence of resistive pressures in the shape of the P–V curve, after elaborating the P–V curve with the pressure ramp of 3 cmH2O/s, a new P–V curve was traced with pressure ramp of 5 cmH2O/s in patients 2, 3, 5, 9, and 10 (Table 1).
P–V curves analysis
P–V curves were constructed by plotting airway pressure against RIP volume in the CPAP technique and airway pressure against ventilator-measured volume in the PV Tool-2 technique. As pressure and volume steps differed between methods, pressure–volume data pairs were fitted to a sigmoidal model modified from the equation proposed by Venegas et al. [14], and volumes corresponding to airway pressure of 0–35 cmH2O in 5 cmH2O steps were interpolated. The equation is Vol = b/{1 + EXP [−(Paw − c)/d]}, where Vol is volume, Paw airway pressure, b the upper asymptote of the curve, c the pressure where curvature changes sign, and d the pressure range where most volume change occurs. This model allows objective, reproducible calculation of the inflection points as follows. On the inspiratory limb, LIP was equal to c −1.317d and the upper inflection point was equal to c +1.317d. On the deflation limb, PMC was calculated as c +1.317d. These points corresponded to the airway pressure where the P–V curve has its maximal upward or downward curvature. Following the same equation makes it possible to calculate the maximal inspiratory and expiratory compliance as: b/4d.
Statistical analysis
The model was fitted using nonlinear regression (least squares method). The two methods of measurement were correlated by calculating the bias and limits of agreement (bias ± 1.96 SD) as proposed by Bland and Altman [15]. The intraclass correlation coefficient (ICC) for absolute agreement was calculated for each pressure level. An ICC >0.75 was considered very good agreement [16]. Data are expressed as mean ± SD or median and interquartile range (IQR), except values from the Bland–Altman analysis, which are expressed as mean (95% confidence interval) and limits of agreement. We considered P < 0.05 significant. We used SPSS 12.0 (SPSS) for all tests.
Results
Table 1 summarizes patients’ characteristics. No deleterious hemodynamic or respiratory effects were identified in any patient. Obtaining the total (inspiratory and expiratory loops) P–V curve took 55 ± 6 s with CPAP technique, 34 ± 3 s with the PV Tool-2 pressure ramp of 3 cmH2O/s and 17 ± 3 s with the PV Tool-2 pressure ramp of 5 cmH2O/s (P < 0.01 for each comparison).
End-expiratory lung volume
End-expiratory lung volume measured before P–V curve tracing with each method in each patient was recorded. No significant differences were found (Table 2).
Mathematical fitting of P–V curves
All P–V curves were fitted to the mathematical model with a mean R 2 of 0.994 ± 0.003. Correlation between curves obtained with the two methods was excellent, and the two curves were almost identical (Fig. 1a). All ICCs were >0.75 (P < 0.001) at all pressure levels (Fig. 1a). However, curves obtained with PV tool-2 pressure ramp of 5 cmH2O/s were different compared with those obtained with pressure ramp of 3 cmH2O/s and the ICCs were <0.75 (Fig. 1b).
a Pressure–volume curves obtained with the two methods (CPAP method and Hamilton method at 3 cmH2O/s of pressure/time ramp) in ten patients. Intraclass correlation coefficients (average and 95% CI) for each point are presented. b Pressure–volume curves obtained with the PV Tool-2 method, with two different pressure/time ramps: 3 and 5 cmH2O/s in five patients (patients 2, 3, 5, 9, and 10) Intraclass correlation coefficients (average and 95% CI) for each point are presented
Correlations for inflection points and compliance
LIP, UIP, PMC, and inspiratory and expiratory compliance calculated from the fitted variables showed good correlation between methods, with ICCs of 0.98 (0.92, 0.99); 0.92 (0.69, 0.98); 0.97 (0.86, 0.98); 0.99 (0.97, 0.99), and 0.95 (0.83, 0.98), respectively, (P < 0.001) (Table 3). Bias for LIP measurements was 0.51 ± 0.95 cmH2O and limits of agreement were −1.36 and 2.38 cmH2O. For UIP measurements, bias was 0.53 ± 1.52 cmH2O and limits of agreement were −2.44 and 3.50 cmH2O. For PMC measurements, bias was −0.62 ± 0.89 cmH2O and limits of agreement were −2.35 and 1.12 cmH2O. Bland–Altman plots for LIP, UIP, and PMC values are represented in Fig. 2a,b, and c, respectively.
Concordance analysis plots showing bias (thick line) and limits of agreement (dashed lines) between the two methods. a Plot for LIP (lower inflection point), b plot for UIP (upper inflection point), and c plot for PMC (point of maximum curvature); CPAP continuous positive airway pressure, HAM Hamilton
Discussion
P–V curves traced with the PV Tool-2 seem equivalent to those obtained with the CPAP technique. However, the PV Tool-2 method is faster and requires no additional equipment. The super-syringe method (generally considered the gold standard) requires the patient to be disconnected from the ventilator, involving possible adverse effects, and the entire maneuver (inspiratory and expiratory limbs) is time consuming (in some studies longer than 100 s [17], twice as long as the CPAP method). Furthermore, this technique requires additional equipment. Techniques to avoid these drawbacks (multiple occlusions and quasi-static low-flow inflation) usually do not allow tracing of the deflation limb. Moreover, the multiple occlusion technique takes several minutes and PEEP must be set at 0 cmH2O, so it is cumbersome in clinical practice and can be transiently detrimental [18–21]. The low-flow inflation technique requires considering the resistive pressure component when flow is >9 L/min [1, 19, 20]. Two solutions to obviate the resistive component in quasi-static methods are: subtracting the resistive pressure in tubing and airways from the total pressure [22, 23], and reducing the constant flow [20]. The first requires complex computerized systems [22], and limiting inspiratory airflow to <9 L/min does not allow high tidal volumes in conventional ventilators, making it very difficult to explore the upper part of the P–V curve [20]. In the PV Tool-2 method, flow is non-constant in order to achieve a designed pressure/time ramp. Airflow changes during the maneuver in function of the patient’s respiratory mechanics. In our study, we calculated the average flow for each curve between 1.5 and 7.7 L/m, all of which were <9 L/min. With this extremely low flow, the resistive pressures can be ignored [1, 20]. To illustrate this point, we performed PV curves using the PV Tool-2 method with two different pressure/time ramps: 3 and 5 cmH2O/s with the latter ramp average flows in our patients were higher (2.4–18 L/m) and we found a clear shift to the right in the faster curve (Fig. 1b and Figs. 1e–5e in electronic supplementary material).
Critique of the methods
The P–V curve obtained with the CPAP technique is time consuming and needs a well-trained clinician because changes in CPAP are difficult (operator-or machine-limited) in some ventilators. Moreover, some conventional ventilators need RIP to measure volume [10], and RIP has limitations to accuracy [24] and requires further analysis for interpretation. Furthermore, the use of a single thoracic band requires muscle paralysis [25].
In contrast, the PV Tool-2 requires no special learning. The complete P–V curve can be traced in less than 35 s without ventilator disconnection and results are immediate. Inflation and deflation limbs can be obtained, and minimal PEEP can be used to avoid lung derecruitment. P–V curve repeatability with both methods was not assessed because alveolar recruitment and derecruitment phenomena inherent to each P–V curve maneuver would make data difficult to interpret.
Finally, a limitation of the Galileo ventilator’s built-in PV Tool-2 is that the inflection points must be determined by eye fitting or by exporting the data. This makes a “well-trained physician” necessary, just like in the CPAP method.
Conclusions
The PV Tool-2 built into the Galileo ventilator is a valid alternative for bedside total respiratory system P–V curve tracing. Moreover, drawbacks inherent in other techniques are avoided. The technique is simple and needs no additional equipment or specialized learning.
References
Lu Q, Rouby JJ (2000) Measurement of pressure volume curves in patients on mechanical ventilation: methods and significance. Crit Care 4:91–100
Amato MBP, Amato MB, Barbas CS, Medeiros DM, Magaldi RB, Schettino GP, Lorenzi-Filho G, Kairalla RA, Deheinzelin D, Munoz C, Oliveira R, Takagaki TY, Carvalho CR (1998) Effect of a protective-ventilation strategy on mortality in the acute respiratory distress syndrome. N Engl J Med 38:347–354
Villar J, Kacmarek RM, Perez-Mendez L, Aguirre-Jaime A (2006) A high positive end-expiratory pressure, low tidal volume ventilatory strategy improves outcome in persistent acute respiratory distress syndrome: a randomized, controlled trial. Crit Care Med 34:1311–1318
Ranieri VM, Suter PM, Tortorella C, De Tullio R, Dayer JM, Brienza A, Bruno F, Slutsky AS (1999) Effect of mechanical ventilation on inflammatory mediators in patients with acute respiratory distress syndrome: a randomized controlled trial. JAMA 282:54–61
Terragni PP, Rosboch G, Tealdi A, Corno E, Menaldo E, Davini O, Gandini G, Herrmann P, Mascia L, Quintel M, Slutsky AS, Gattinoni L, Ranieri VM (2007) Tidal hyperinflation during low tidal volume ventilation in acute respiratory distress syndrome. Am J Respir Crit Care Med 175:160–166
Roupie E, Dambrosio M, Sevillo G, Mentec H, el Atrous S, Beydon L, Brun-Buisson C, Lemaire F, Brochard L (1995) Titration of tidal volume and induced hypercapnia in acute respiratory distress syndrome. Am J Respir Crit Care Med 152:121–128
Albaiceta GM, Luyando LH, Parra D, Menendez R, Calvo J, Pedreira PR, Taboada F (2005) Inspiratory vs expiratory pressure–volume curves to set end-expiratory pressure in acute lung injury. Intensive Care Med 31:1370–1378
DiRocco JD, Carney DE, Nieman GF (2007) Correlation between alveolar recruitment/derecruitment and inflection points on the pressure–volume curve. Intensive Care Med 33:1204–1211
Bayle F, Guerin C, Debord S, Badet M, Lemasson S, Poupelin JC, Richard JC (2006) Assessment of airway closure from deflation lung volume–pressure curve: sigmoidal equation revisited. Intensive Care Med 32:894–898
Albaiceta GM, Piacentini E, Villagra A, Lopez-Aguilar J, Taboada F, Blanch L (2003) Application of continuous positive airway pressure to trace static pressure–volume curves of the respiratory system. Crit Care Med 31:2514–2519
Piacentini E, Wysocki M, Ll Blanch (2007) Validation of a new commercially available method to measure static-pressure volume curves at the bedside. Intensive Care Med 33(S2):207
Bernard GR, Artigas A, Brigham KL, Carlet J, Falke K, Hudson L, Lamy M, Le Call JR, Morris A, Spragg R, The Consensus Committee (1994) The American–European consensus conference on ARDS. Definition, mechanisms, relevant outcomes, and clinical trial coordination. Am J Respir Crit Care Med 149:818–824
Decailliot F, Demoule A, Maggiore SM, Jonson B, Duvaldestin P, Brochard L (2006) Pressure–volume curves with and without muscle paralysis in acute respiratory distress syndrome. Intensive Care Med 32:1322–1328
Venegas JG, Harris RS, Simon BA (1998) A comprehensive equation the pulmonary pressure volume curve. J Appl Physiol 84:389–395
Bland JM, Altman DG (1986) Statistical methods for assessing agreement between two methods of clinical measurement. Lancet 8476:307–310
Bartko JJ (1966) The intraclass correlation coefficient as a measure of reliability. Psychol Rep 19:3–11
Chiumello D, Carlesso E, Aliverti A, Dellaca RL, Pedotti A, Pelosi P, Gattinoni L (2007) Effects of volume shift on the pressure–volume curve of the respiratory system in ALI/ARDS patients. Minerva Anestesiol 73:109–118
Fernandez R, Blanch L, Artigas A (1993) Inflation static pressure–volume curves of the total respiratory system determined without any instrumentation other than the mechanical ventilator. Intensive Care Med 19:33–38
Servillo G, Svantesson C, Beydon L, Roupie E, Brochard L, Lemaire F, Jonson B (1997) Pressure–volume curves in acute respiratory failure. Automated low flow inflation versus occlusion. Am J Respir Crit Care Med 155:1629–1636
Lu Q, Vieira S, Richecoeur J, Puybasset L, Kalfon P, Coriat P, Rouby JJ (1999) A simple automated method for measuring pressure volume curve during mechanical ventilation. Am J Respir Crit Care Med 159:275–282
Ranieri VM, Giuliani R, Flore T, Dambrosio M, Milic-Emili J (1994) Volume–pressure curve of the respiratory system predicts effects of PEEP in ARDS: ‘occlusion’ versus ‘constant flow’ technique. Am J Respir Crit Care Med 149:19–27
Jonson B, Richard JC, Straus C, Mancebo J, Lemaire F, Brochard L (1999) Pressure–volume curves and compliance in acute lung injury: evidence of recruitment above the lower inflection point. Am J Respir Crit Care Med 159:1172–1178
Blanch L, Lopez-Aguilar J, Villagra A (2007) Bedside evaluation of pressure–volume curves in patients with acute respiratory distress syndrome. Curr Opin Crit Care 13:332–337
Markhorst DG, Van Gestel JP, Van Genderingen HR, Haitsma JJ, Lachmann B, Van Vught AJ (2006) Respiratory inductive plethysmography accuracy at varying PEEP levels and degrees of acute lung injury. J Med Eng Technol 30:166–175
Dall’ava-Santucci J, Armaganidis A, Brunet F, Dhainaut JF, Chelucci G, Monsallier J, Lockhart A (1988) Causes of error of respiratory pressure–volume curves in paralyzed subjects. J Appl Physiol 64:42–49
Acknowledgments
This work was supported in part by an Educational grant from Hamilton. CIBER of respiratory diseases is an initiative of ISCIII.
Author information
Authors and Affiliations
Corresponding author
Additional information
An erratum to this article is available at http://dx.doi.org/10.1007/s00134-010-2024-0.
Electronic supplementary material
Below is the link to the electronic supplementary material.
Rights and permissions
About this article
Cite this article
Piacentini, E., Wysocki, M. & Blanch, L. A new automated method versus continuous positive airway pressure method for measuring pressure–volume curves in patients with acute lung injury. Intensive Care Med 35, 565–570 (2009). https://doi.org/10.1007/s00134-008-1322-2
Received:
Accepted:
Published:
Issue Date:
DOI: https://doi.org/10.1007/s00134-008-1322-2