Bioaccumulation of PCBs, OCPs and PBDEs in Marine Mammals From West Antarctica
- 1Graduate School of Oceanography, University of Rhode Island, Narragansett, RI, United States
- 2Department of Environmental Sciences, Faculty of Science, Alexandria University, Alexandria, Egypt
- 3Department of Ocean Sciences, University of California, Santa Cruz, Santa Cruz, CA, United States
- 4Virginia Institute of Marine Science, Gloucester Point, VA, United States
- 5Department of Biological and Environmental Sciences, University of Gothenburg, Gothenburg, Sweden
- 6Department of Environmental Research and Monitoring, Swedish Museum of Natural History, Stockholm, Sweden
- 7Institute for Terrestrial and Aquatic Wildlife Research, University of Veterinary Medicine Hannover, Büsum, Germany
- 8Marine Mammal Research, Department of Ecoscience, Aarhus University, Roskilde, Denmark
To understand the bioaccumulation and food web dynamics of persistent organic pollutants (POPs) as a function of species, age and sex in Antarctic mammals, blubber samples of 3 killer whales (Type C) and 77 pinnipeds (Weddell, Ross and crabeater seals) were collected from the Southern Ocean, Antarctica. They were analyzed for polychlorinated biphenyls (PCBs), organochlorine pesticides (OCPs) and polybrominated diphenyl ethers (PBDEs). ΣDDTs, Σ29PCBs and chlordanes (12 – 4,600, 13 – 1,600, and < 1.5 – 1,700 ng/g lipid, respectively) were the most abundant POPs. Killer whales typically displayed several times greater concentrations of POPs compared to seals, except for PBDEs. PCBs and PBDEs were consistently higher in adult crabeater and Weddell seal males, and in adult female Ross seals than in other sex and age groups reflecting an age accumulation and possible influence of segregated diet, foraging areas, and metabolic transformation rates. POPs concentrations significantly correlated with gene transcription of nuclear receptors involved in detoxification of contaminants and immune relevant cell mediators in the crabeater seals, indicating possible immunotoxic and deleterious health effects. This represents one of the largest studies on POPs in Antarctic marine predators and highlights the complexity of POPs bioaccumulation.
HIGHLIGHTS
- OCPs, PCBs and PBDEs were measured in 3 seal species and Type C killer whales in Western Antarctica.
- Killer whales displayed strong bioaccumulation of PCBs and OCPs, but not of PBDEs.
- Weddell and Ross seals displayed similar POPs profiles, different from crabeater seals.
- POPs are influenced by variations in habitat, diet, and metabolic transformations.
- Biomarkers for immunotoxicity were related to PCB concentrations in crabeater seals.
Introduction
Antarctica has long been considered a pristine environment given its distance from human populations and pollution sources. For persistent organic pollutants (POPs), this view changed when organochlorine pesticides (OCPs) (George and Frear, 1966) and polychlorinated biphenyls (PCBs), formerly used in electronics and other industries (Risebrough and Rieche, 1968), were detected in 1966. Since then, studies were performed to investigate the presence of POPs in water/snow (Tanabe et al., 1983; Dickhut et al., 2005; Hale et al., 2008; Vecchiato et al., 2015; Khairy et al., 2016) and biota (Kawano et al., 1984; Larsson et al., 1992; Corsolini et al., 2007; Bargagli, 2008; Krahn et al., 2008; Corsolini, 2009; Schiavone et al., 2009; Cipro et al., 2012; Trumble et al., 2012; Brault et al., 2013; Dorneles et al., 2015). Long range atmospheric transport (Tanabe et al., 1983; Larsson et al., 1992; Montone et al., 2003), local emissions from the research stations (Larsson et al., 1992; Wild et al., 2014; Khairy et al., 2016) and/or the re-volatilization from snow as it melts or metamorphoses (Khairy et al., 2016) have been suggested as the major sources of POPs in Antarctica.
Due to the lipophilic and persistent nature of POPs, cold temperatures in polar regions, and the tendency of polar organisms to store lipid as an energy source, bioaccumulation of POPs in the Antarctic organisms is expected (Loganathan and Kannan, 1991; Schiavone et al., 2009). Additionally, biomagnification can result in higher concentrations of POPs in top predators, and render them more prone to adverse health effects (Schiavone et al., 2009). Upper trophic level predators, like marine mammals, are good bioindicators of the quality of their environment since they have relatively long-life spans and poor metabolism of POPs (Schiavone et al., 2009; Trumble et al., 2012). Weddell (Leptonychotes weddellii) and crabeater seals (Lobodon carcinophagus), are especially good bioindicators for Antarctica, as their habitat range is constrained to Antarctic waters (Laws, 1977). Recent research indicates that Ross seals (Ommatophoca rossii) spend a considerable portion of the year in pelagic regions, traveling up to ∼1,300 km from the ice edge (Arcalís-Planas et al., 2015), and winter migrations of Antarctic fur seals (Arctocephalus gazella) are diverse and may reach the Patagonian Shelf (Hinke et al., 2012; Staniland et al., 2012).
Thus, in Ross and Antarctic fur seals, POP-levels represent the combined contribution from Antarctic and subantarctic environments. Continuous monitoring of POP concentrations in these marine mammals will therefore indicate any shift or change in the environmental quality of their habitats. This is valuable information and can lead to the discovery of new sources of emission since threshold levels for POPs were exceeded, potentially leading to endocrine disruption and immunotoxicity and indicating threats for marine mammal populations (Noël et al., 2009).
In Antarctica, some seal species have been widely used for monitoring POPs: Weddell seals for example were used to monitor the levels of OCPs (Kawano et al., 1984; Karolewski et al., 1987; Larsson et al., 1992; Vetter et al., 2003; Corsolini, 2009; Cipro et al., 2012; Trumble et al., 2012), PCBs (Karolewski et al., 1987; Larsson et al., 1992; Vetter et al., 2003; Corsolini, 2009; Trumble et al., 2012) and to a lesser extent, polybrominated diphenyl ether (PBDEs) (Hale et al., 2008; Schiavone et al., 2009; Cipro et al., 2012; Trumble et al., 2012). Antarctic fur seals (Corsolini, 2009; Schiavone et al., 2009; Brault et al., 2013) were occasionally used to monitor the chlorinated organic compounds. However, crabeater seals (Corsolini, 2009), Ross seals and killer whales (Orcinus orca) (Krahn et al., 2008) have rarely been used to monitor POPs.
In the current study, we measured the concentrations of OCPs, PCBs and PBDEs in blubber biopsies collected from Weddell, crabeater, and Ross seals and killer whale of different age and sex. This is an extension to the previous work that reported on mercury (Hg) concentrations in seal fur (hair) of some of the same samples (Aubail et al., 2011; Lehnert et al., 2017) and investigated the difference across the Arctic and Antarctic continents as well as the influence of Hg exposure on their immune systems by quantifying gene transcription of selected molecular biomarkers. Our goals were to: (i) determine the concentrations of different POPs in seals and killer whales; (ii) compare the concentrations and profiles of POPs in the four investigated species and elucidate the influences of sex, growth stage, lipid content and body weight on contaminant concentrations and patterns by applying multivariate and univariate statistical techniques; (iii) investigate the influence of the exposure to POPs on the immune systems of the seal species; and (iv) calculate the bioaccumulation and trophic magnification factors for the OCPs and PCBs in the Antarctic food web.
Materials and Methods
Detailed description of the sampling methods for phytoplankton, krill and fish, extraction and cleanup, isotope analysis in biota and physicochemical properties of pollutants can be found in the Supplementary Information. A summary is given below.
Sampling
During the “Oden Southern Ocean” expeditions in December–January 2008/09 and 2010/11 in the Amundsen and Ross seas, Antarctica, skin, and blubber biopsies were taken from Weddell seals (n = 33), Ross seals (n = 11), crabeater seals (n = 33) and killer whales (n = 3) [Supplementary Figure 1, for ship track and sampling stations see Lehnert et al. (2017)]. Seals hauling out on the ice were spotted and caught from (1) the mother ship, when moored to an ice floe, (2) from a zodiac, that was launched when the mother ship was moored to an ice floe, (3) during ski trips departing from the mother ship when moored to an ice floe or (4) from a helicopter that departed from the mothership at any time when moored or when moving through the ice. When seals were spotted during surveys from a helicopter, the helicopter landed on the ice within 100–500 m, a distance where no apparent reaction to the helicopter was seen. From all platforms the seals were slowly approached and either caught using a lightweight pole-net made of two carbon fiber wind surfer masts and a triangular 2 × 2 cm nylon net tied to the masts, and physically restrained (seals in 2008/09 and some in 2020/11) or immobilized with Zoletil 100 using a Daninject airgun and anaesthetizing darts. The handling/sampling procedure of each seal took 30–60 min in total. For the seals, standard length and circumference were measured, weight taken during ventral recumbency using a lightweight tripod and a digital scale (Salter) and sex and life stage (subadult, adult) estimated (Table 1). For each seal a set of samples were collected. A 5 mm biopsy skin punch (PFM Medical) was used to collect a 0.4 g skin/blubber biopsy at the lower part of the back near the hip, blood samples were taken from the epidural intravertebral vein and hair samples were cut with a scissor at the lower part of the back. Samples were always stored frozen until they were analyzed (Supplementary Figure 2).
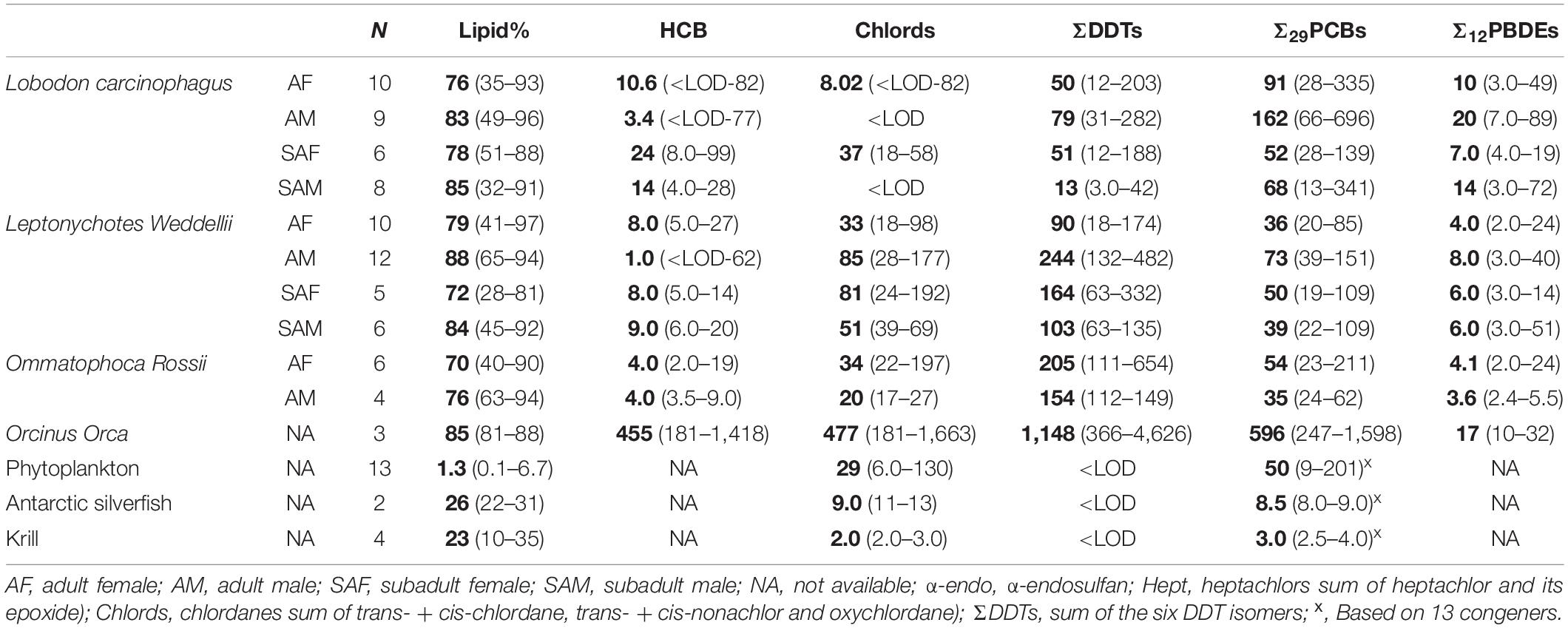
Table 1. Geometric means (in bold) and range (in brackets) of OCPs (HCB, Chlords and DDT), PCBs and PBDEs ng/g lipid) in the blubber samples of the three seal species and killer whales, Values from phytoplankton, the Antarctic silverfish and krill are from Brault (2012).
The whole procedure is shown in two YouTube videos:
https://www.youtube.com/watch?v=lZEH-bsz18w&t=1s
https://www.youtube.com/watch?v=QhE1JXUwUew
From a helicopter the killer whales were spotted spy hopping at the ice edge in the channel made by the ice breaking mothership (Oden). The helicopter landed on the ice within 500 m of the whales, a distance where no apparent reaction to the helicopter was observed. The whales were approached by foot from the ice edge and biopsied using the air gun and darts at < 20 m range and the darts were retrieved in the water from the ice edge. More details about the samples are given in Supplementary Table 1.
For trophic magnification factors (TMF) calculations, we used concentrations of POPs and stable nitrogen isotope values (δ15N) in Antarctic krill, phytoplankton and silverfish from the same study region (Brault, 2012).
Extraction, Cleanup, and Instrumental Analysis
After homogenization with anhydrous Na2SO4, each sample was spiked with surrogate standards and extracted on a table shaker for 1 day using a n-hexane/dichloromethane (DCM) solvent mixture (1:1, v: v) followed by another 1-day extraction with DCM only. Lipid content was determined gravimetrically by taking aliquots from the concentrated extracts. Cleanup of the extracts was done using sulfuric acid treatment followed by neutral silica gel (1.0 g). After eluting the target analytes, samples were concentrated to a final volume of ∼ 50 μL in n-hexane after spiking with the injection standards.
Twenty nine PCB congeners (PCB-8, 11, 18, 28, 44, 52, 66, 77, 81, 101, 105, 114, 118, 123, 126, 128, 138, 153, 156, 157, 167, 169, 170, 180, 187, 189, 195, 206, and 209), 19 OCPs including hexachlorobenzene (HCB), α-, β-, γ- and δ- hexachlorocyclohexane (HCH), heptachlor and its epoxide, oxychlordane, trans- and cis-chlordane, trans- and cis-nonachlor, α-endosulfan, o,p′-DDE, o,p′-DDD, o,p′-DDT, p,p′-DDE, p,p′-DDD and p,p′-DDT and 12 PBDE congeners (BDE-2, 8, 15, 28, 30, 47, 49, 99, 100, 153, 154, 183) were investigated in the current study. All the analytes were analyzed using an Agilent GC 6890N with a DB-5 MS fused silica capillary column (30 m × 0.25 mm i.d., 0.25 μm film thickness, J&W Scientific) equipped with a Quattro micro-GC tandem MS (Waters) as detailed in Khairy et al. (2016).
Samples (1 μL) were auto injected in splitless mode with the injection port at 250°C. Column flow was maintained at 1.0 mL/min. The instrument operated in the multiple reaction monitoring mode with the following temperature program: initial oven temperature of 100°C (1 min), ramping at a rate of 11.0°C/min to 180°C, then to 260°C (0.00 min) at 3.0°C/min, and finally at 20.0°C/min to a final temperature of 300°C with a final holding time of 6.00 min. PBDEs were analyzed using the same system with the same column in the splitless mode with the injection port at 260°C. Column flow was maintained at 2.0 mL/min. The instrument operated in the multiple reaction monitoring mode with the following temperature program: initial temperature 140°C, hold 2 min, 10°C/min to 180°C, 3°C/min to 220°C, 10°C/min to 310, and hold for 5 min. PBDEs were identified based on the comparison of the retention time and mass spectrum of selected ions with the calibration standards.
Biomarkers Sampling and Analysis
Details on the sampling and analysis of gene transcription of selected immune- and pollutant- related molecular biomarkers can be found in Lehnert et al. (2017). Briefly, blood samples were taken from the epidural intravertebral vein of the individuals. Full blood was collected in ethylenediaminetetraacetic acid (EDTA) tubes pre-prepared with RNAlater and stored at −20°C until RNA isolation. Hair samples for Hg analysis were always taken on the lower back part of the seal.
Quality Assurance
Procedural blanks and matrix spikes were included with each sample batch throughout the entire analytical procedure in a manner identical to the real samples. Recoveries of the surrogate standards ranged from 71 to 96% for OCPs, PCBs and PBDEs, respectively. Matrix spikes recoveries ranged from 93 to 103% for all the target analytes and relative standard deviation was < 20%. Method detection limits were calculated as a signal to noise ratio of 3.0 (0.3 – 1.2 ng/g lipids for all POPs). Few analytes (PCB 18 and HCB) were detected in the blanks at levels < 8.0% of what was detected in the samples; samples were not blank-corrected. BDE- 47 was detected in the blanks at concentrations < 17% of the sample concentrations and samples were corrected for the blank concentration.
Results and Discussion
Geometric means (GM) and ranges of OCPs, PCBs and PBDEs in the blubber samples of seals and killer whales are given in Table 1. Concentrations < LOD were replaced by 1/2 LOD for data processing. In general, ΣDDTs displayed the highest concentrations, of up to 4,600 ng/g lipid (GM: 1,148 ng/g lipid) in orcas and 650 ng/g lipid (GM: 115 ng/g lipid) in seals, followed by sum Σ29PCBs (GM: 596 ng/g lipid in orcas, 65 ng/g lipid in seals) and chlordanes (GM: 477 ng/g lipid in orcas, 36 ng/g lipid in seals). ΣDDTs dominated in Weddell and Ross seals, and killer whales, while PCBs were most abundant in crabeater seals. Concentrations of OCPs, PCBs and PBDEs in all the samples are detailed in Supplementary Tables 2–13, respectively.
In all the sex and age classes of Weddell, ross, and in killer whales, the following descending order (based on GM) was observed: DDTs (sum of o,p′-DDE, o,p′-DDD, o,p′-DDT, p,p′-DDE, p,p′-DDD and p,p′-DDT; 90 – 244 ng/g lipid, 154 – 205 and 1,148 ng/g lipid, respectively) > PCBs (36 – 73 ng/g lipid, 35 – 54 ng/g lipid and 600 ng/g lipid) > chlordanes (sum of trans- and cis-chlordane, trans- and cis-nonachlor and oxychlordane; 33–85 ng/g lipid, 20–34 ng/g lipid and 477 ng/g lipid) > hexachlorobenzene (HCB; 1.0 – 9.0 ng/g lipid, 4.0 ng/g lipid and 455 ng/g lipid) > PBDEs (4.0 – 8.0 ng/g lipid, 3.6 – 4.1 ng/g lipid and 17 ng/g lipid). A different order was observed in crabeater, where PCBs displayed highest concentrations (52 – 162 ng/g lipid) followed by DDTs (50 – 79 ng/g lipid), chlordanes (<LOD – 37 ng/g lipid), PBDEs (7.0 – 20 ng/g lipid) and HCB (3.4 – 24 ng/g lipid).
In the current study, higher concentrations of chlordanes were observed in crabeaters (<LOD – 82 ng/g lipid) compared to previously detected concentrations (23 ng/g lipid) (Cipro et al., 2012) although the mean concentration was comparable (arithmetic mean: 24 ng/g lipid). Chlordane concentrations in Weddells (18–177 ng/g lipid) were higher than concentrations reported during 2004–2006 [10 ng/g lipid, n = 1 (Cipro et al., 2012)]. Additionally, reported chlordane concentrations in adult males (28–177 ng/g lipid) and adult females (18–98 ng/g lipid) were higher than concentrations previously observed for the same age and sex groups in 2006 [38 and 17 ng/g lipid (Trumble et al., 2012)] but lower than concentrations observed in subadult males [39–69 ng/g lipid in the current study compared to 73 ng/g lipid in 2006 (Trumble et al., 2012)]. Differences in the measured isomers (cis- and trans-nonachlor were not included in Cipro et al. (2012), total number of investigated samples [1–3 samples (Cipro et al., 2012) and 21 in Trumble et al. (2012) compared to 30 in the current study] and sampling locations should be considered in this comparison.
DDT concentrations were much higher than concentrations reported by Cipro et al. (2012), and lower than all the other performed studies from Antarctica (George and Frear, 1966; Sladen et al., 1966; Corsolini, 2009; Trumble et al., 2012). OCP concentrations were also within the range or lower than concentrations previously reported for Antarctic fur seal blubber (Schiavone et al., 2009). Detected OCP concentrations in the killer whale were within the range previously observed for killer whales from the Antarctic (Krahn et al., 2008) but much lower than concentrations previously observed for killer whales from the southern Indian Ocean (Noël et al., 2009), Alaska, West California and Eastern Pacific (Krahn et al., 2008).
Detected concentrations of PCBs in Weddells (36 – 73 ng/g lipid) were lower than concentrations previously reported in 1981 [345 ng/g lipid (Siegfried et al., 2013)], 1994/1995 [395 ng/g lipid (Corsolini et al., 2002)], 2004–2006 [300 ng/g lipid (Cipro et al., 2012)] but within the range observed in 2006 [17 – 73 ng/g lipid (Trumble et al., 2012)] although different PCB congeners were investigated in the above mentioned studies. PCB concentrations in the killer whale (247 – 1,598 ng/g lipid) were within the range previously observed in 2006 [93 – 5,300 ng/g lipid) (Krahn et al., 2008)] and lower than concentrations observed in other places worldwide (Krahn et al., 2008). Median concentrations of PCBs, chlordanes and DDTs reported for seals in the current study are ∼ 2 folds higher than median concentrations reported for Gentoo and Chinstrap penguins collected during 2013 – 2014 from King George Island, Antarctica (Kim et al., 2021).
Few studies investigated the levels of PBDEs in the blubber of seals and killer whales in Antarctica. In studies performed on Weddell seals during 2004–2006 (Cipro et al., 2012) and 2006 (Trumble et al., 2012), only BDE-99 was detected in the first study in one sample with a concentration (2.0 ng/g lipid) close to the arithmetic mean observed in the current study (2.7 ng/g lipid), whereas in the second study, BDE-47 was the only reported congener with similar concentrations (<LOD – 1.8 ng/g lipids) to our findings (1.2 – 2.3 ng/g lipid) for only adult males, females and subadult males. However, we detected several tri-through hexa-brominated BDE congeners in the seal blubber samples (1.0–88 ng/g lipid), with an average concentration (arithmetic mean: 13 ng/g lipid) that was comparable to that observed for fur seal blubber samples collected from the Antarctic in 2004 [11 ng/g lipid (Schiavone et al., 2009)] despite the different number of investigated congeners (6 congeners compared to 12 in our study). Detected concentrations in the killer whale blubber samples (10 – 32 ng/g lipid) were within the range previously observed in 2005 [ < LOD – 74 ng/g lipid (Krahn et al., 2008)].
Organochlorine Pesticides
Cis-chlordane was only detected in the subadult males and females of Weddell seals and killer whales comprising on average 16 and 20%, respective to the total chlordane concentrations (Supplementary Figure 3A), which could possibly be attributed to the higher degradation rate of the trans-isomer (Eitzer et al., 2001). Trans-chlordane (TC) was only detected in the killer whale blubber (6.0–8.0 ng/g lipid) with a concentration lower than the cis-isomer (CC; 52 – 249 ng/g lipid). Ratios of TC/CC for the killer whale samples ranged from 0.02 to 0.23, which is much lower than the value calculated for technical chlordane (1,56), indicating an aged chlordane source (Bidleman et al., 2002).
Trans-nonachlor (TN) was more frequently detected (n = 56) than the cis-isomer (n = 40). In the adult crabeater and Weddell seals, trans-nonachlor was the dominant isomer comprising 28–100% of the total chlordane concentrations, whereas comparable contributions of the two nonachlor isomers (28 – 33% each) were observed in the crabeater and Weddell subadults, adult Ross seal seals and killer whale (Supplementary Figure 3A). The ratio of TN/TC in the current study ranged from 2 to 276 for the samples where TC was detected. These values are much higher than values previously reported for the technical chlordane [0.42, (Jantunen et al., 2000) and 0.76, (Mattina et al., 1999)]. TN was also enriched in air above soil containing weathered technical chlordane residues (Eitzer et al., 2001) indicating again the aged chlordane source in the current study.
ΣDDTs were the most abundant and the most frequently detected (94% of the total samples) OCPs in the blubber samples of the seals and killer whales. p,p′-DDE was the most abundant isomer comprising > 61% of the total DDT concentrations in all the species (Supplementary Figure 3B) followed by the parent compound p,p′-DDT (13 – 32%) and p,p′-DDD (1.0 – 13%). o,p-isomers of the parent compound and metabolites were < LOD in all the seal blubber samples. The ratio of p,p′-DDE/p,p′-DDT in the current study ranged from 1.4 to 23 indicating an aged DDT source (Sarkar et al., 2008).
Hexachlorobenzene was detected in 83% of the total analyzed seal blubber samples and in all the killer whale samples. However, it displayed lower concentrations compared to DDTs and chlordanes (Table 1). This pattern was already previously observed for marine mammals in the Antarctic (Corsolini, 2009).
Although HCHs and α-endosulfan were not detected in any of the samples in the current study, they were previously detected in the blubber samples of crabeater (HCHs: 0.22 ng/g lipid; α-endosulfan: 2.1 ng/g lipid) and Weddell seals (2.6 ng/g lipid; 14 ng/g lipid) collected from the Antarctic in the austral summers of 2004/2005 and 2005/2006 (Cipro et al., 2012). This could be attributed to the ban of HCHs and their short half-lives compared to DDTs.
Polychlorinated Biphenyls
Polychlorinated biphenyls were detected in 100% of the samples with concentrations ranging from 20 to 700 ng/g lipid and 250 – 1,600 ng/g lipid in the seal and killer whale blubber samples, respectively. Concentrations of PCBs in the killer whale (250 – 1,600 ng/g lipid) were much higher than concentrations reported for the seal samples most likely owing to its higher trophic position in the food web.
Octa- through deca-chlorinated congeners were < LOD in all the seal samples. As previously observed for seals (Trumble et al., 2012) and in the scat of killer whales (Lundin et al., 2016), PCB 153 and 138 were the most abundant PCB congeners followed by PCB 101, 52, 118, and 180, as is typically observed in higher trophic level biota.
As for the PCB patterns, all animals, regardless of their sex, life stage or species, were dominated by the hexa-, hepta- and penta-chlorinated congeners (Supplementary Figure 3C). This pattern was previously observed for killer whales in the southern Indian Ocean (Noël et al., 2009) and higher chlorinated PCB congeners dominated in the blubber samples of killer whales collected from the Antarctic (Krahn et al., 2008). However, a slightly different pattern, characterized by the dominance of the tetra-, penta-, hexa- and hepta- congeners (Cipro et al., 2012), was previously observed in crabeater and Weddell seal blubber. Organisms located at higher trophic levels accumulate POPs from their diet, and higher chlorinated (more lipophilic and resistant to metabolic transformation) PCB congeners (hexa- and hepta-) are expected to dominate as previously observed (Focardi et al., 1995).
Polybrominated Diphenyl Ethers
Geometric mean concentrations of PBDEs in all the samples were lower than those reported for the legacy POPs (Table 1) and ranged from 7.0 to 20 ng/g lipid (crabeater), 4.0 – 8.0 ng/g lipid (Weddell) and 4.0 ng/g lipid (Ross) in the seal samples. The geometric mean PBDE concentrations in the killer whale samples (17 ng/g lipid) were comparable to those of the male Crabeater seals (both adults and subadults; 20 and 14 ng/g lipid, respectively) and ∼ 2–4 folds higher than in the Weddell, Ross and female Weddell blubber samples (4.0 – 10 ng/g lipid), which was different from our findings for OCPs and PCBs, implying that either the uptake sources of PBDEs are different and/or a difference in the metabolic rates across the marine mammals exists. Age differences between the killer whales and seals could also play a role.
All the investigated blubber samples in the three species of the seals were dominated by BDE-47 and BDE-99 comprising 52 – 71% of the total PBDE concentrations (Supplementary Figure 3D) due to the wide global use of the penta-technical formulation (Jia and Gan, 2014). Snowmelt samples collected from the same region were also dominated by the same congeners, which were mainly emitted from local sources (research stations) (Khairy et al., 2016). BDE-47 was also the highest detected congener in the blubber samples of Antarctic fur seal (>90%) (Schiavone et al., 2009). In the killer whale samples, BDE-47 was still the dominant congener (48%) followed by BDE-49 (28%) and all the other congeners showed comparable contributions (<4% each). In all the samples, BDE-2, BDE-8 and BDE-15 were < LOD and BDE-183 was only detected in the adult males of Ross seals.
Persistent Organic Pollutant Trends Across Pinnipeds and Killer Whales
Several interesting trends were observed in the investigated animals though only few were statistically significant. OCP and PCB concentrations in the killer whales displayed much greater concentrations than the seals, in-line with their trophic position (see biomagnification section below). OCPs displayed the most diverse variations between and within seals (e.g., male versus female, subadult versus adult) (Figure 1A). Comparable PBDE concentrations in the seals and killer whales were observed (Table 1), possibly related to the exposure to the same sources (local sources as mentioned earlier) and possibly similar metabolic rates. This observed pattern for PBDEs was different compared to PCBs and OCPs indicating differences in the uptake sources and elimination patterns.
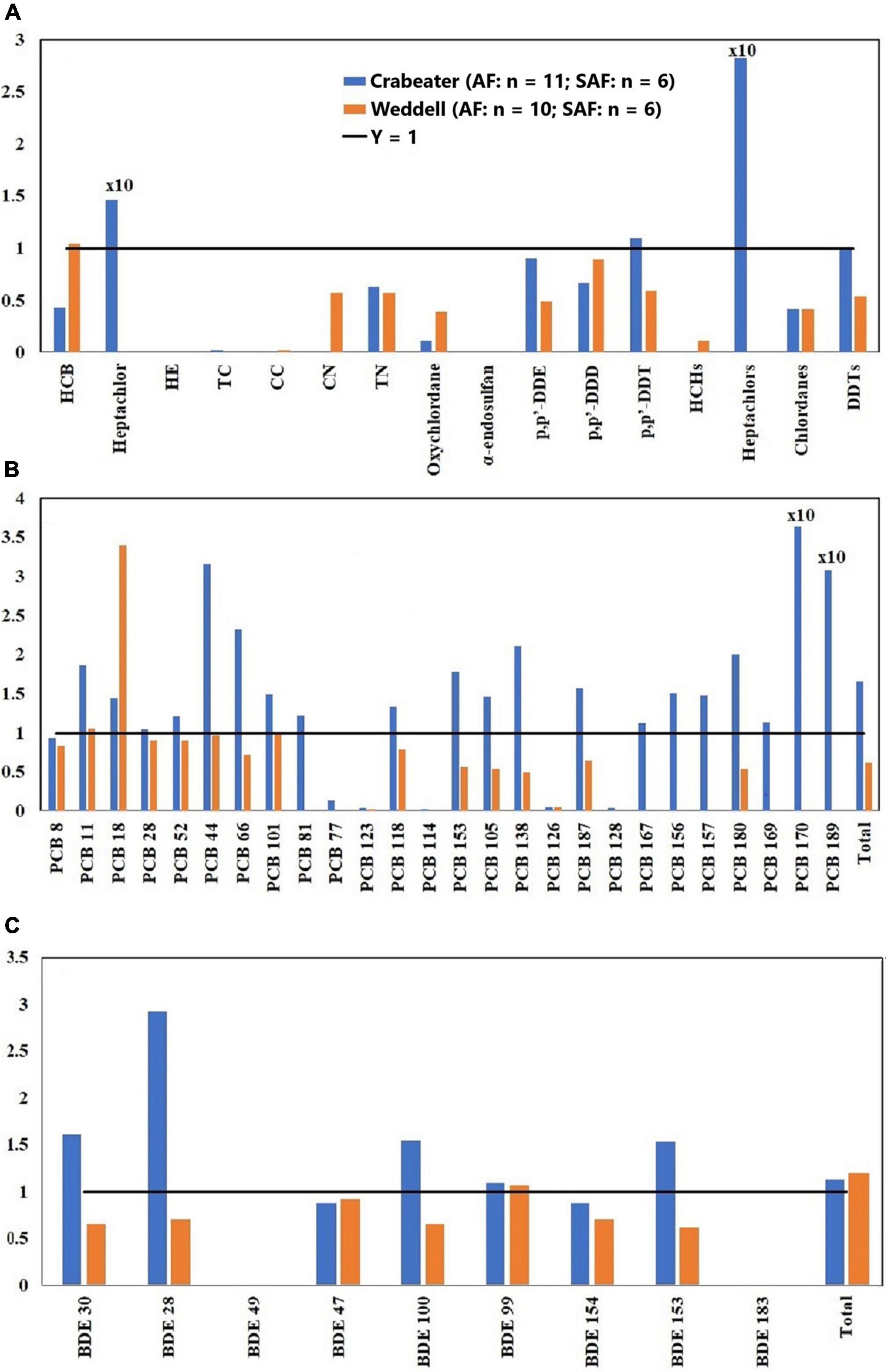
Figure 1. Adult female (AF)/subadult female (SAF) concentration ratios of OCPs (A), PCBs (B) and PBDEs (C). HE, heptachlor-epoxide; TC, trans-chlordane; CC, cis-chlordane; CN, cis-nonachlor; TN: trans-nonachlor. See Table 1 for sample size in each group.
Adults vs. Subadults
Previous studies on marine mammals have indicated that concentrations of POPs were generally higher in adults compared to subadults due to the longer exposure periods (age) and the reliance on prey items from different tropic levels. However, metabolic transformation rates in adults are higher compared to subadults, which could result in higher accumulation in the subadults (Aguilar et al., 1999). Additionally, the transfer of POPs from adult females to the newly born offspring via lactation could result in a reduced body burden of POPs in the adult females (Aguilar et al., 1999).
In the current study, no specific pattern was observed when OCP concentrations in the adult and subadult crabeater females were compared (Figure 1A). In contrast, the majority of OCPs showed higher concentrations (1.3 – 5.0 factor difference) in subadult females of Weddell seals, which was statistically significant (Kruskal-Wallis ANOVA on ranks, p < 0.05) for cis-nonachlor. This finding was similar to the pattern observed for the lower chlorinated PCBs in the Northern Pacific elephant seals (Mirounga angustirostris) (Peterson et al., 2014). For both seal species, OCP concentrations in the adult males were higher than the subadults (1.3 – 6.0 factor difference; Figure 2A) and was statistically significant (Kruskal-Wallis ANOVA on ranks, p < 0.05) for p,p′-DDE and ΣDDTs in the crabeater, and p,p′-DDE and p,p′-DDT in the Weddell seals.
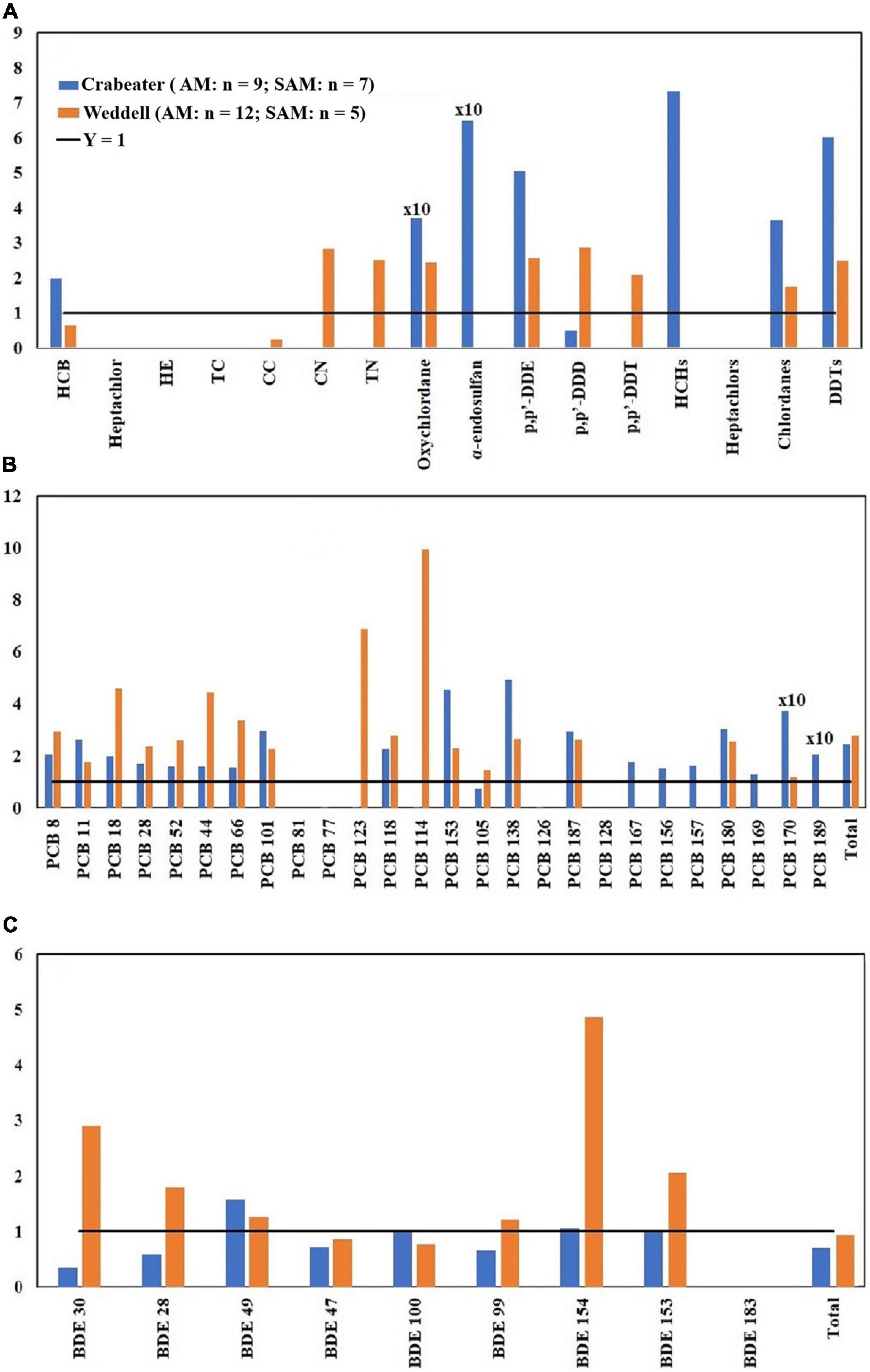
Figure 2. Adult male (AM)/subadult male (SAM) concentration ratios of OCPs (A), PCBs (B) and PBDEs (C). HE, heptachlor-epoxide; TC, trans-chlordane; CC, cis-chlordane; CN, cis-nonachlor; TN, trans-nonachlor. See Table 1 for sample size in each group.
Concentrations of PCBs and PBDEs in the crabeater seals were generally higher in adult females than in subadults (Figures 1B,C), whereas PCBs and PBDEs in the Weddell seals showed a similar pattern to OCPs (higher in subadult females). In the males of both species, adults displayed higher concentrations of PCBs and PBDEs (Figures 2B,C), as was observed for OCPs. Except for PBDEs and PCBs in the crabeater, our observed findings were comparable to what was previously found for marine mammals (Aguilar et al., 1999), and that the pattern observed for PCBs and PBDEs in the crabeater (higher in adult females than subadults) could be attributed to differences in metabolic transformations (higher in adults) and different diet, which was found to substantially differ with age and sex in a study of cetaceans (Aguilar et al., 1999).
Males vs. Females
In marine mammals, females generally showed lower detoxification ability for POPs compared to males, which could result in higher concentrations in females (Aguilar et al., 1999). However, other factors such as lactation (for females), their respective life stage (age), and dietary differences can influence the pattern. In the current study, concentrations of most OCPs in the adult females of crabeater and Ross seals were generally higher than concentrations in adult males, whereas the reverse was observed for Weddell seals (Figure 3A). Concentrations of HCB, heptachlor, trans-nonachlor and chlordanes in adult and subadult females of crabeater, and p,p′-DDE in adult female Ross seals were significantly higher (Kruskal-Wallis ANOVA on ranks, p < 0.05) than in male seals (Figures 1A, 2A, 3A). In contrast, concentrations of trans-nonachlor, p,p′-DDE, p,p’-DDT and DDTs in adult males of Weddells were significantly higher (Kruskal-Wallis ANOVA on ranks, p < 0.05) than adult females.
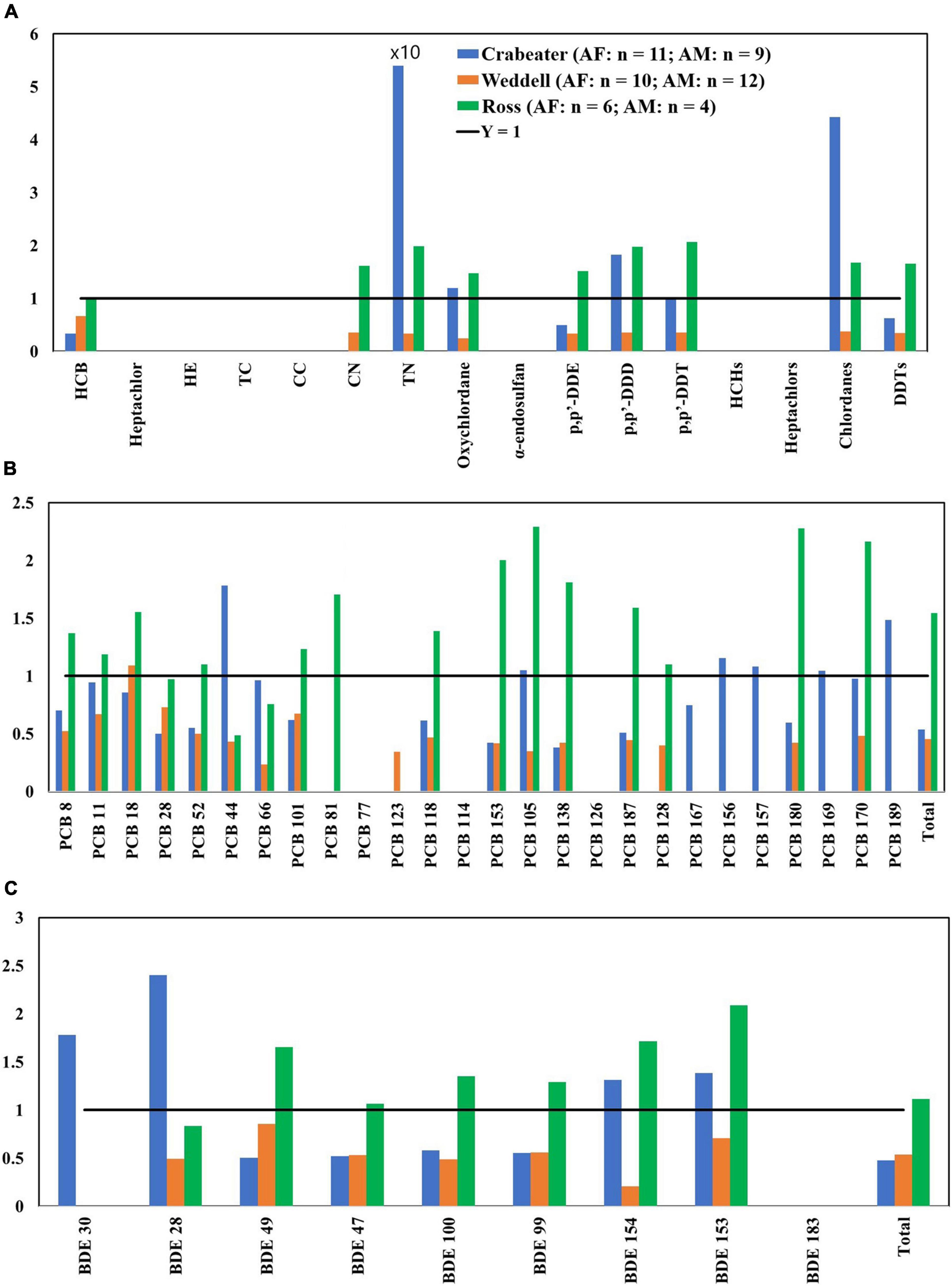
Figure 3. Adult female (AF)/adult male (AM) concentration ratios of OCPs (A), PCBs (B) and PBDEs (C). HE, heptachlor-epoxide; TC, trans-chlordane; CC, cis-chlordane; CN, cis-nonachlor; TN, trans-nonachlor. See Table 1 for sample size in each group.
Concentrations of the majority of PCB and PBDE congeners (Figures 3B,C) in crabeater and Weddell seals were higher in adult males than adult females. In contrast, concentrations of PBDEs and PCBs were higher in the adult female Ross seals (by 1.5 – 6.0 times). In our study, although higher concentrations of PCBs and PBDEs were observed in males, this was not consistent for all the seal species (Ross seals were different from the other two species) and not for all the classes of POPs (OCPs showed different pattern that PCBs and PBDEs in the crabeater). Accordingly, metabolic transformation alone could not explain the observed patterns and that other factors such as differences in food items, foraging areas, nutritive conditions, habitat differences [as was observed for other species (Krahn et al., 2007; Dickhut et al., 2009), and blubber thickness should be considered (Aguilar et al., 1999)].
Ross vs. Crabeater and Weddell Seals
Focusing on the general patterns of POPs concentrations in seals and killer whales implied that whales, Weddell and Ross seals shared common uptake pathways for POPs, whereas crabeater seals were distinctly different. In the former two species, concentrations of POPs generally followed ΣDDTs > chlordanes > PCBs > HCBs and PBDEs, while for crabeater seals, the ranking was PCBs > ΣDDTs > PBDEs, HCB and chlordanes. This difference in POPs ranking could be explained by the fact that Weddell and Ross seals both feed on higher trophic levels (squid, fish), while crabeaters mostly feed on krill (Rau et al., 1992; Burns et al., 1998).
There is also the influence of feeding regions on POPs accumulations: crabeater and Weddell seals are both residents of the Antarctic coastal waters, whereas Ross seals may migrate northwards 1,300 kilometers out in the open ocean (Arcalís-Planas et al., 2015) and some vagrants have been sighted as far north as South Georgia, the Falkland Island and the South Sandwich Islands (Stewart, 2007) thus reflecting a possible sub-Antarctic pollution signature. Detected concentrations of POPs in Ross seals were comparable to concentrations observed in the other two seal species. However, concentrations of PCBs and PBDEs in the females were almost always higher than the males, which in most cases contradicts what was observed for the other seal species (Figure 3). Accordingly, we conclude that this observed sex difference could be attributed to difference in the diet, foraging areas, and metabolic activities with no strong influence of the migratory behavior of the Ross seals.
Gene Transcripts and Persistent Organic Pollutants
mRNA transcripts of target genes in blood samples of the seal species are shown in Supplementary Table 14. The correlation analysis between POPs and gene transcripts in the three seal species revealed significant relationships only in crabeater seals (Supplementary Table 15). Gene transcription of aryl hydrocarbon receptor nuclear translocator (ARNT) and aryl hydrocarbon receptor (AHR) and peroxisome proliferator-activated receptor alpha (PPARα) showed significant relationships with HCB, chlordanes, DDTs, Σ29PCBs, and PCB homologs groups, but not with PBDEs and 3-Cl biphenyls. AHR and ARNT are induced by environmental contaminants and mediate immunotoxic compounds (Gaspar-Ramírez et al., 2015). AHR, ARNT and PPARα transcription were previously found to be associated with the exposure to organic pollutants in Baikal seals (Kim et al., 2005; Ishibashi et al., 2008) and in harbor seals (Lehnert et al., 2016).
In a study performed by Lehnert et al. (2017), no correlation was found between xenobiotic biomarker transcripts and Hg concentrations in fur of the same seal species. Nevertheless, the highest transcription levels of pollutant-related markers were found in crabeaters despite lower detected Hg concentrations compared to Ross and Weddell seals. Based on the correlations observed in the current study, and the higher detected PCB concentrations in the crabeaters (Table 1), it seems that PCB concentrations may be responsible for the high ARNT and AHR gene transcription observed in crabeater seals. The strong correlation of DDTs and HCB with ARNT, however, may be due to species-specific metabolic processes and mechanisms of bioaccumulation and biomagnification in crabeater seals.
Pro-inflammatory cytokines interleukin-2 (IL-2) transcription was significantly correlated (p < 0.05, Supplementary Table 16) to HCB (r = 0.75), PCBs (r = 0.58) and trichloro-, tetrachloro- and pentachloro-biphenyls (r > 0.65) in the crabeater seals, as was previously observed in harbor seals (Lehnert et al., 2016). IL-2 is a cell mediator of inflammatory disease in marine mammals (Boyman and Sprent, 2012). The significant correlation between IL-2 transcription and some POP compounds in the current study may indicate immune-modulatory effects caused by pollutant exposure.
Heat-shock-protein 70 (HSP70) transcription was significantly correlated with trichloro- (r = 0.64) and pentachloro- (r = 0.58) biphenyl concentrations in the crabeater seals (Supplementary Table 15) indicating a possible effect on their stress response. Similarly, some heavy metals showed significant correlations with AHR, IL-2 and HSP70 transcription in harbor seals, indicating effects of environmental contaminants on the xenobiotic metabolism, stress- and immune response in pinnipeds (Lehnert et al., 2016).
It should be noted that POPs were analyzed in blubber, while biomarker transcripts were measured in blood samples. The different matrices used may in part account for differences observed as pollutant concentrations in blood and blubber may vary due to metabolic processes and biomobilization characteristics of pollutant compounds.
Bioaccumulation of Persistent Organic Pollutants
In the current study, the bioaccumulation factors (BAF) for POPs were calculated based on the detected concentrations of POPs in the blubber samples (Clip, ng/kg lipid) and dissolved water concentrations, obtained from Galbán-Malagón et al. (2013) (PCBs and OCPs for samples collected in 2009) and Bigot et al. (2016) (OCPs only for samples collected in 2014). Unfortunately, no data were available for PBDEs; accordingly, they were excluded from this discussion. Calculated BAFs for OCPs and PCBs are given in Supplementary Table 16 and shown in Figure 4. BAFs were the highest for PCBs and ranged from 1.3 × 106 – 1.1 × 1010 and 1.5 × 107 – 1.8 × 1010 in the seals and killer whales, respectively, followed by OCPs (2.1 × 104 – 2.6 × 109 and 9.4 × 104 – 4.4 × 109). Calculated BAFs for PCBs and OCPs in the killer whales were significantly higher (One Way Repeated Measures ANOVA, p < 0.001) than calculated BAFs for the seals. Additionally, calculated BAFs for all OCPs (except cis-nonachlor) and PCBs were 1 – 4 orders of magnitude higher than Klip–w in the seal and killer whale samples (Figure 4). At the same time, the log BAF-Klip–w linear relationships for PCBs and OCPs were statistically insignificant (p > 0.05). All the above findings suggest that the uptake of POPs is mainly occurring through diet, and the absence of equilibrium partitioning between lipids and the water. Calculated BAFs for oxychlordane, p,p′-DDE, p,p′-DDD and hexachlorobenzene (average: 108) were 1–2 order of magnitude higher than the other OCPs (average: 106–107) (Supplementary Table 16). Similarly, calculated BAFs for the hepta-chlorinated congeners (average: 109 for PCBs 180 and 187) were 1–2 order of magnitude higher than the other congeners (107–108). This could possibly be attributed to either more uptake from the food items and/or less metabolic transformation rates (persistence) for the higher chlorinated PCB congeners, p,p′-DDE, p,p′-DDD and oxychlordane.
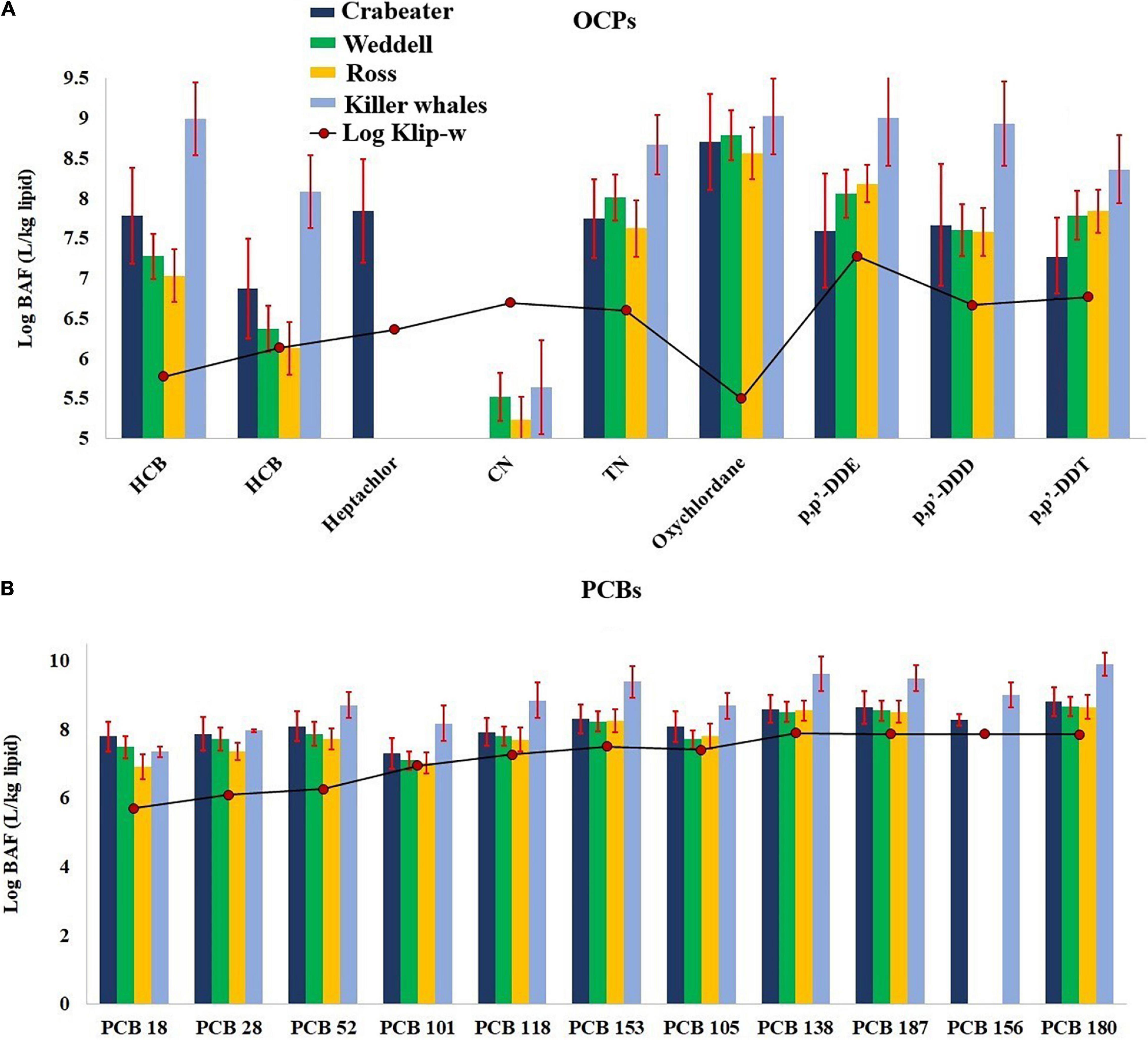
Figure 4. Log BAF-log Klip–w relationship for OCPs (A) and PCBs (B) in the seal and killer whale samples collected from western Antarctic. Error bars represent the standard deviation. See Table 1 for sample size in each group.
Trophic Magnification of Persistent Organic Pollutants
To investigate the biomagnification of POPs, the trophic level of each of the seal species and killer whales was calculated based the stable nitrogen isotope values, δ15N, using the phytoplankton as the base trophic level according to Post (2002). Concentrations of several OCPs and PCBs (trans-chlordane, cis-chlordane, trans-nonachlor, cis-nonachlor, PCB 101, 105, 118, 138, and 153) from the higher trophic level predators reported here were compared to those in Antarctic krill, phytoplankton and silverfish together with stable nitrogen isotope values (δ15N) for the same species (Brault, 2012). The lower trophic level samples were collected during the same expeditions as the seal samples of the current study. δ15N were not estimated for the seal and killer whale samples collected in this study, but rather obtained from Zhao et al. (2004) and Krahn et al. (2008). We note that this approach relies on assuming a consistent baseline value of δ15N in primary producers (Graham et al., 2010).
Except for phytoplankton, an increasing trend of PCB and OCP concentrations with increasing trophic level was observed, from krill to the killer whale (Figure 5). As shown in Supplementary Table 17, cis-chlordane, nonachlors, PCB 118, 138, and 153 showed trophic magnification [trophic magnification factors (TMF) > 1]. However, TMFs were only significantly greater than one (p < 0.05) for trans- and cis-nonachlor (TMFs = 2.52 – 5.48). In contrast, TMFs for trans-chlordane, PCB 101 and 105 were less than one indicating their biodilution in the food web, although statistically insignificant (p > 0.05).
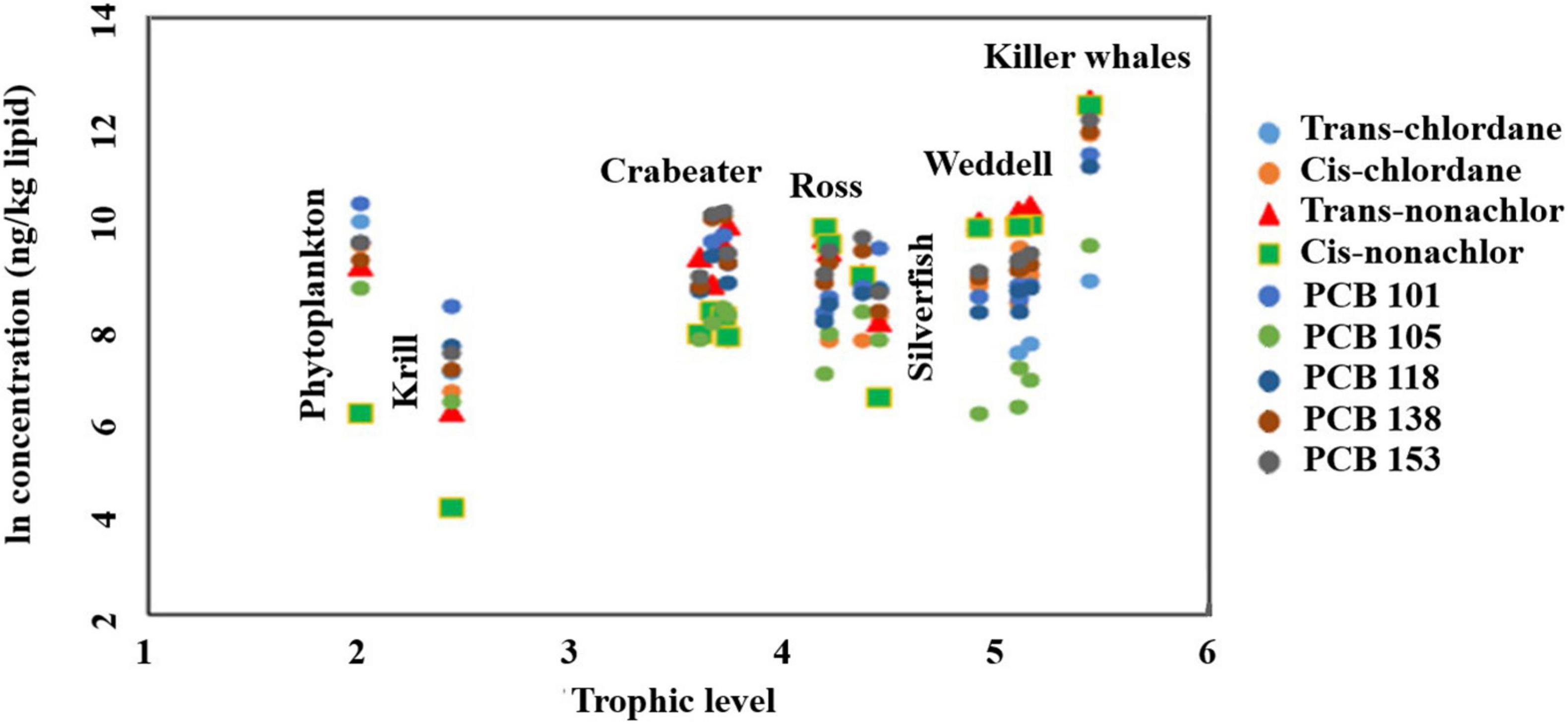
Figure 5. Ln concentration-trophic level relationship for average values of chlordanes and PCB congeners in the trophic food web of western Antarctica (Amundsen and Ross Sea).
To investigate whether the obtained relations and TMF values are foodweb-dependent (depends on what species were included in the TMF calculations), TMFs were recalculated after excluding phytoplanktons (Supplementary Table 17). When phytoplanktons were excluded, all the investigated pollutants showed trophic magnification (TMFs > 1). Additionally, calculated TMFs were statistically significant for trans- and cis-nonachlor, cis-chlordane, PCB 138 and 153. This implies that TMF calculations and their significance are partially influenced by the included species. Calculated TMFs for the nonachlor isomers were generally higher than values calculated for the PCBs, and the highest values was observed for cis-nonachlor, which was 2–5 times higher than all the other values (Supplementary Table 17). It must be pointed out that calculated TMFs in the current study are influenced by many factors. First, not all the dietary items for seals and the killer whales were included in the current study (e.g., large predatory fish and crabeater seal pups). Additionally, based on the measured concentrations of POPs, we assumed that even for the same seal species, different foraging areas and food items could be expected in addition to the physiological differences that could affect the uptake and excretion of POPs and hence TMFs. Second, the trophic levels for the investigated species were calculated based on δ15N values, and there is a difference in the turnover time between nitrogen isotopes (within weeks) compared to POPs (months to years) (Post, 2002). Finally, migration patterns especially for killer whales could also be a factor affecting the calculated TMFs in the current study, all of which indicates the complex nature of this unique food web.
Conclusion
Detected concentrations of OCPs and PCBs in seals were generally lower than concentrations previously detected in the period from 1981 to 2006, whereas in the killer whale, no decline in PCB concentrations were observed. In both seals and killer whales, PBDE concentrations were much lower than the legacy pollutants, but within the range observed in previous studies. Similar PCB/OCP profiles in orcas, Weddell and Ross seals imply a common regional POPs concentration across the wider foraging region. This in turn may highlight the continuous role played by regional sources (research stations, deep sea currents and ice melting) in polluting this unique aquatic environment (Goerke et al., 2004). Accordingly, blubber samples collected over time should be considered in future research to highlight any differences arising from temporal changes in POPs dynamics in top predators (glacier and snow melting, temperature variations, and variations in the thicknesses of the blubber layer). The current work also highlighted that sex, age and species type only played a minor role in explaining the variability in the detected concentrations of POPs in the blubber samples and that additional factors linked to habitat and diet are needed to better explain observed differences between species and various POPs concentrations. The highest gene transcription in xenobiotic biomarkers were observed in crabeater seals, probably due to their greater PCB concentrations compared to the other investigated seal species, despite their lower trophic levels. The observed correlations between POP concentrations and other biomarkers such as HSP70 in the crabeaters may indicate the possibility of occurrence of immunotoxic and deleterious health effects in the crabeater population.
Capsule
The investigation of POPs in Antarctic marine mammals indicated that sex, age and species type only played a minor role in explaining the variability in the detected concentrations and that additional factors linked to habitat and diet are needed to better explain observed differences. Results indicated the possibility of occurrence of immunotoxic and deleterious health effects in one species from the exposure to PCBs.
Data Availability Statement
The original contributions presented in the study are included in the article/Supplementary Material, further inquiries can be directed to the corresponding author.
Author Contributions
RD conceived the original study. EB performed the field work. KH, TH, OK, KL, and JT shared samples and contributed to editing the manuscript. MK analyzed the samples and wrote the manuscript. RL took over the samples after RD’s untimely death and supported the analysis and manuscript drafting. All authors contributed to the article and approved the submitted version.
Funding
RL acknowledges funding from the National Science Foundation (ANT 1332492) to understand emerging pollutant dynamics in the Antarctic. The Swedish Polar Research Secretariat provided logistic support through the two Oden Southern Ocean expeditions (OSO 2008/2009 and OSO 2010/11) and the Swedish Research Council granted financial support to TH and KH (Grant Numbers: 2008-6464 and 2008-6239). The Centre for Evolutionary Marine Biology (CeMeb) at Gothenburg University also contributed financial support through a grant to KH (Grant Number: 217-2008-1719).
Conflict of Interest
The authors declare that the research was conducted in the absence of any commercial or financial relationships that could be construed as a potential conflict of interest.
Publisher’s Note
All claims expressed in this article are solely those of the authors and do not necessarily represent those of their affiliated organizations, or those of the publisher, the editors and the reviewers. Any product that may be evaluated in this article, or claim that may be made by its manufacturer, is not guaranteed or endorsed by the publisher.
Acknowledgments
We thank RD (Virginia Institute of Marine Science) for her prior leadership on this grant. We thank the skilled and dedicated crew of the Swedish icebreaker Oden and especially the helicopter crew Sven Stenvall and Nils Eriksson. Under the permit issued by the Swedish Polar Research Secretariat (Dnr 2010–112) all animal handling protocols were authorized.
Supplementary Material
The Supplementary Material for this article can be found online at: https://www.frontiersin.org/articles/10.3389/fmars.2021.768715/full#supplementary-material
References
Aguilar, A., Borrell, A., and Pastor, T. (1999). Biological factors affecting variability of persistent pollutant levels in cetaceans. J. Cetacean Res. Manag. 1, 83–116.
Arcalís-Planas, A., Sveegaard, S., Karlsson, O., Harding, K. C., Wåhlin, A., Harkonen, T., et al. (2015). Limited use of sea ice by the Ross seal (Ommatophoca rossii), in Amundsen Sea, Antarctica, using telemetry and remote sensing data. Polar Biol. 38, 445–461.
Aubail, A., Teilmann, J., Dietz, R., Rigét, F., Harkonen, T., Karlsson, O., et al. (2011). Investigation of mercury concentrations in fur of phocid seals using stable isotopes as tracers of trophic levels and geographical regions. Polar Biol. 34, 1411–1420.
Bargagli, R. (2008). Environmental contamination in Antarctic ecosystems. Sci. Total Environ. 400, 212–226. doi: 10.1016/j.scitotenv.2008.06.062
Bidleman, T. F., Jantunen, L. M. M., Helm, P. A., Brorström-Lundén, E., and Juntto, S. (2002). Chlordane enantiomers and temporal trends of chlordane isomers in Arctic Air. Environ. Sci. Technol. 36, 539–544. doi: 10.1021/es011142b
Bigot, M., Muir, D. C. G., Hawker, D. W., Cropp, R., Dachs, J., Teixeira, C. F., et al. (2016). Air–Seawater exchange of organochlorine pesticides in the Southern ocean between Australia and Antarctica. Environ. Sci. Technol. 50, 8001–8009. doi: 10.1021/acs.est.6b01970
Boyman, O., and Sprent, J. (2012). The role of interleukin-2 during homeostasis and activation of the immune system. Nat. Rev. Immunol. 12, 180–190.
Brault, E. (2012). Evaluating Persistent Organic Pollutants (POPs) and Mercury in the West Antarctic Peninsula (WAP) Food Web, with a Focus on Antarctic Fur Seals (Arctocephalus gazella). M.Sc. thesis. Gloucester Point, VA: Virginia Institute of Marine Sciences. doi: 10.25773/v5-bzse-sc35
Brault, E. K., Goebel, M. E., Geisz, H. N., Canuel, E. A., and Dickhut, R. M. (2013). Inter-annual variation of persistent organic pollutants (POPS) in an Antarctic top predator Arctocephalus gazella. Environ. Sci. Technol. 47, 12744–12752. doi: 10.1021/es403577f
Burns, J. M., Trumble, S. J., Castellini, M. A., and Testa, J. W. (1998). The diet of Weddell seals in McMurdo Sound, Antarctica as determined from scat collections and stable isotope analysis. Polar Biol. 19, 272–282. doi: 10.1007/s003000050245
Cipro, C. V. Z., Bustamante, P., Taniguchi, S., and Montone, R. C. (2012). Persistent organic pollutants and stable isotopes in pinnipeds from King George Island, Antarctica. Mar. Pollut. Bull. 64, 2650–2655. doi: 10.1016/j.marpolbul.2012.10.012
Corsolini, S. (2009). Industrial contaminants in Antarctic biota. J. Chromatogr. A 1216, 598–612. doi: 10.1016/j.chroma.2008.08.012
Corsolini, S., Borghesi, N., Schiamone, A., and Focardi, S. (2007). Polybrominated diphenyl ethers, polychlorinated dibenzo-dioxins,-furans, and-biphenyls in three species of antarctic penguins. Environ. Sci. Pollut. Res. Int. 14, 421–429. doi: 10.1065/espr2006.01.017
Corsolini, S., Kannan, K., Imagawa, T., Focardi, S., and Giesy, J. P. (2002). Polychloronaphthalenes and other dioxin-like compounds in Arctic and Antarctic marine food webs. Environ. Sci. Technol. 36, 3490–3496.
Dickhut, R. M., Cincinelli, A., Cochran, M., and Ducklow, H. W. (2005). Atmospheric Concentrations and air-water flux of organochlorine pesticides along the Western Antarctic Peninsula. Environ. Sci. Technol. 39, 465–470. doi: 10.1021/es048648p
Dickhut, R. M., Deshpande, A. D., Cincinelli, A., Cochran, M. A., Corsolini, S., Brill, R. W., et al. (2009). Atlantic bluefin tuna (Thunnus thynnus) population dynamics delineated by organochlorine tracers. Environ. Sci. Technol. 43, 8522–8527.
Dorneles, P. R., Lailson-Brito, J., Secchi, E. R., Dirtu, A. C., Weijs, L., Dalla Rosa, L., et al. (2015). Levels and profiles of chlorinated and brominated contaminants in Southern Hemisphere humpback whales, Megaptera novaeangliae. Environ. Res. 138, 49–57. doi: 10.1016/j.envres.2015.02.007
Eitzer, B. D., Mattina, M. I., and Iannucci-Berger, W. (2001). Compositional and chiral profiles of weathered chlordane residues in soil. Environ. Toxicol. Chem. 20, 2198–2204. doi: 10.1002/etc.5620201011
Focardi, S., Bargagli, R., and Corsolini, S. (1995). Isomer-specific analysis and toxic potential evaluation of polychlorinated biphenyls in Antarctic fish, seabirds and Weddell seals from Terra Nova Bay (Ross Sea). Antarct. Sci. 7, 31–35.
Galbán-Malagón, C. J., Del Vento, S., Berrojalbiz, N., Ojeda, M.-J., and Dachs, J. (2013). Polychlorinated biphenyls, hexachlorocyclohexanes and hexachlorobenzene in seawater and phytoplankton from the Southern Ocean (Weddell, South Scotia, and Bellingshausen Seas). Environ. Sci. Technol. 47, 5578–5587. doi: 10.1021/es400030q
Gaspar-Ramírez, O., Pérez-Vázquez, F. J., Salgado-Bustamante, M., González-Amaro, R., Hernandez-Castro, B., and Pérez-Maldonado, I. N. (2015). DDE and PCB 153 independently induce aryl hydrocarbon receptor (AhR) expression in peripheral blood mononuclear cells. J. Immunotoxicol. 12, 266–272. doi: 10.3109/1547691X.2014.960108
Goerke, H., Weber, K., Bornemann, H., Ramdohr, S., and Plötz, J. (2004). Increasing levels and biomagnification of persistent organic pollutants (POPs) in Antarctic biota. Mar. Pollut. Bull. 48, 295–302. doi: 10.1016/j.marpolbul.2003.08.004
Graham, B. S., Koch, P. L., Newsome, S. D., McMahon, K. W., and Aurioles, D. (2010). “Using isoscapes to trace the movements and foraging behavior of top predators in oceanic ecosystems,” in Isoscapes, eds J. West, G. Bowen, T. Dawson, and K. Tu (Dordrecht: Springer), 299–318.
Hale, R. C., Kim, S. L., Harvey, E., La Guardia, M. J., Mainor, T. M., Bush, E. O., et al. (2008). Antarctic research bases: local sources of Polybrominated Diphenyl Ether (PBDE) flame retardants. Environ. Sci. Technol. 42, 1452–1457. doi: 10.1021/es702547a
Hinke, J., Watters, G., Trivelpiece, W., and Goebel, M. (2012). Synoposis of Data From Satellite Telemetry of Foraging Trips and Migration Routes of Penguins and Pinnipeds From the South Shetland Islands, 1997/98 to present. Document no. WG-EMM-12/37. Santa Cruz de Tenerife: CCAMLR Working Group on Ecosystem Monitoring and Management.
Ishibashi, H., Iwata, H., Kim, E.-Y., Tao, L., Kannan, K., Amano, M., et al. (2008). Contamination and effects of perfluorochemicals in baikal seal (Pusa sibirica). 1. residue level, tissue distribution, and temporal trend. Environ. Sci. Technol. 42, 2295–2301. doi: 10.1021/es072054f
Jantunen, L. M. M., Bidleman, T. F., Harner, T., and Parkhurst, W. J. (2000). Toxaphene, chlordane, and other organochlorine pesticides in Alabama Air. Environ. Sci. Technol. 34, 5097–5105. doi: 10.1021/es001197y
Jia, F., and Gan, J. (2014). Comparing black carbon types in sequestering polybrominated diphenyl ethers (PBDEs) in sediments. Environ. Pollut. 184, 131–137. doi: 10.1016/j.envpol.2013.08.009
Karolewski, M. A., Lukowski, A. B., and Halba, R. (1987). Residues of chlorinated hydrocarbons in the adipose tissue of the Antarctic pinnipeds. Polish Polar Res. 8, 189–197.
Kawano, M., Inoue, T., Hidaka, H., and Tatsukawa, R. (1984). Chlordane compounds residues in Weddell seals () from the Antarctic. Chemosphere 13, 95–100. doi: 10.1016/0045-6535(84)90011-0
Khairy, M. A., Luek, J. L., Dickhut, R., and Lohmann, R. (2016). Levels, sources and chemical fate of persistent organic pollutants in the atmosphere and snow along the western Antarctic Peninsula. Environ. Pollut. 216, 304–313. doi: 10.1016/j.envpol.2016.05.092
Kim, E.-Y., Iwata, H., Suda, T., Tanabe, S., Amano, M., Miyazaki, N., et al. (2005). Aryl hydrocarbon receptor (AHR) and AHR nuclear translocator (ARNT) expression in Baikal seal (Pusa sibirica) and association with 2,3,7,8-TCDD toxic equivalents and CYP1 expression levels. Comp. Biochem. Physiol. Part C Toxicol. Pharmacol. 141, 281–291. doi: 10.1016/j.cca.2005.07.007
Kim, J.-T., Choi, Y.-J., Barghi, M., Kim, J.-H., Jung, J.-W., Kim, K., et al. (2021). Occurrence, distribution, and bioaccumulation of new and legacy persistent organic pollutants in an ecosystem on King George Island, maritime Antarctica. J. Hazard. Mater. 405:124141. doi: 10.1016/j.jhazmat.2020.124141
Krahn, M. M., Hanson, M. B., Baird, R. W., Boyer, R. H., Burrows, D. G., Emmons, C. K., et al. (2007). Persistent organic pollutants and stable isotopes in biopsy samples (2004/2006) from Southern resident killer whales. Mar. Pollut. Bull. 54, 1903–1911.
Krahn, M. M., Pitman, R. L., Burrows, D. G., Herman, D. P., and Pearce, R. W. (2008). Use of chemical tracers to assess diet and persistent organic pollutants in Antarctic Type C killer whales. Mar. Mammal Sci. 24, 643–663. doi: 10.1111/j.1748-7692.2008.00213.x
Larsson, P., Järnmark, C., and Södergren, A. (1992). PCBs and chlorinated pesticides in the atmosphere and aquatic organisms of Ross Island, Antarctica. Mar. Pollut. Bull. 25, 281–287. doi: 10.1016/0025-326X(92)90683-W
Laws, R. M. (1977). Seals and whales of the Southern Ocean. Philos. Trans. R. Soc. London B Biol. Sci. 279, 81–96.
Lehnert, K., Ronnenberg, K., Weijs, L., Covaci, A., Das, K., Hellwig, V., et al. (2016). Xenobiotic and Immune-relevant molecular biomarkers in harbor seals as proxies for pollutant burden and effects. Arch. Environ. Contam. Toxicol. 70, 106–120. doi: 10.1007/s00244-015-0202-3
Lehnert, K., Weirup, L., Harding, K. C., Härkönen, T., Karlsson, O., and Teilmann, J. (2017). Antarctic seals: molecular biomarkers as indicators for pollutant exposure, health effects and diet. Sci. Total Environ. 59, 1693–1704. doi: 10.1016/j.scitotenv.2017.04.224
Loganathan, B. G., and Kannan, K. (1991). Time perspectives of organochlorine contamination in the global environment. Mar. Pollut. Bull. 22, 582–584. doi: 10.1016/0025-326X(91)90244-M
Lundin, J. I., Ylitalo, G. M., Booth, R. K., Anulacion, B., Hempelmann, J. A., Parsons, K. M., et al. (2016). Modulation in persistent organic pollutant concentration and profile by prey availability and reproductive status in southern resident killer whale scat samples. Environ. Sci. Technol. 50, 6506–6516. doi: 10.1021/acs.est.6b00825
Mattina, M. J. I., Iannucci-Berger, W., Dykas, L., and Pardus, J. (1999). Impact of long-term weathering, mobility, and land use on chlordane residues in soil. Environ. Sci. Technol. 33, 2425–2431. doi: 10.1021/es990012o
Montone, R. C., Taniguchi, S., and Weber, R. R. (2003). PCBs in the atmosphere of King George Island, Antarctica. Sci. Total Environ. 308, 167–173. doi: 10.1016/S0048-9697(02)00649-6
Noël, M., Barrett-Lennard, L., Guinet, C., Dangerfield, N., and Ross, P. S. (2009). Persistent organic pollutants (POPs) in killer whales (Orcinus orca) from the Crozet Archipelago, southern Indian Ocean. Mar. Environ. Res. 68, 196–202. doi: 10.1016/j.marenvres.2009.06.009
Peterson, S. H., Hassrick, J. L., Lafontaine, A., Thomé, J.-P., Crocker, D. E., Debier, C., et al. (2014). Effects of age, adipose percent, and reproduction on PCB concentrations and profiles in an extreme fasting North Pacific marine mammal. PLoS One 9:e96191. doi: 10.1371/journal.pone.0096191
Post, D. (2002). Using stable isotopes to estimate trophic position: models, methods, and assumptions. Ecology 83, 703–718.
Rau, G. H., Ainley, D. G., Bengston, J. L., Torres, J. J., and Hopkins, T. L. (1992). N-15/N-14 and C-13/C-12 in Weddell sea birds, seals, and fish: implications for diet and trophic structure. Mar. Ecol. Ser. 84, 1–8.
Risebrough, R. W., and Rieche, P. (1968). Polychlorinated biphenyls in the global ecosystem. Nature 220, 1098–1102.
Sarkar, S. K., Bhattacharya, B. D., Bhattacharya, A., Chatterjee, M., Alam, A., Satpathy, K. K., et al. (2008). Occurrence, distribution and possible sources of organochlorine pesticide residues in tropical coastal environment of India: an overview. Environ. Int. 34, 1062–1071. doi: 10.1016/j.envint.2008.02.010
Schiavone, A., Kannan, K., Horii, Y., Focardi, S., and Corsolini, S. (2009). Occurrence of brominated flame retardants, polycyclic musks, and chlorinated naphthalenes in seal blubber from Antarctica: comparison to organochlorines. Mar. Pollut. Bull. 58, 1415–1419.
Siegfried, W. R., Condy, P. R., and Laws, R. M. (2013). Antarctic Nutrient Cycles and Food Webs. Berlin: Springer.
Sladen, W. J. L., Menzie, C. M., and Reichel, W. L. (1966). DDT residues in Adelie penguins and a crabeater seal from Antarctica: ecological implications. Nature 210, 670–673.
Staniland, I. J., Robinson, S. L., Silk, J. R. D., Warren, N., and Trathan, P. N. (2012). Winter distribution and haul-out behaviour of female Antarctic fur seals from South Georgia. Mar. Biol. 159, 291–301.
Stewart, B. S. (2007). A summary of Status of Knowledge of the Biology, Distribution, and Abundance of the Ross Seal, Ommatophoca rossii. in XXX Antartic Treaty Consultative Meeting. Cambridge: Scientific Committee on Antarctic Research.
Tanabe, S., Hidaka, H., and Tatsukawa, R. (1983). PCBs and chlorinated hydrocarbon pesticides in Antarctic atmosphere and hydrosphere. Chemosphere 12, 277–288. doi: 10.1016/0045-6535(83)90171-6
Trumble, S. J., Robinson, E. M., Noren, S. R., Usenko, S., Davis, J., and Kanatous, S. B. (2012). Assessment of legacy and emerging persistent organic pollutants in Weddell seal tissue (Leptonychotes weddellii) near McMurdo Sound, Antarctica. Sci. Total Environ. 439, 275–283. doi: 10.1016/j.scitotenv.2012.09.018
Vecchiato, M., Argiriadis, E., Zambon, S., Barbante, C., Toscano, G., Gambaro, A., et al. (2015). Persistent Organic Pollutants (POPs) in Antarctica: occurrence in continental and coastal surface snow. Microchem. J. 119, 75–82. doi: 10.1016/j.microc.2014.10.010
Vetter, W., Weichbrodt, M., and Stoll, E. (2003). Indication of geographic variations of organochlorine concentrations in the blubber of antarctic weddell seals (Leptonychotes weddelli). Environ. Sci. Technol. 37, 840–844.
Wild, S., McLagan, D., Schlabach, M., Bossi, R., Hawker, D., Cropp, R., et al. (2014). An Antarctic research station as a source of brominated and perfluorinated persistent organic pollutants to the local environment. Environ. Sci. Technol. 49, 103–112.
Keywords: Ross seal, Weddell seal, crabeater seal, killer whale, POPs, DDT, trophic magnification, gene transcription
Citation: Khairy M, Brault E, Dickhut R, Harding KC, Harkonen T, Karlsson O, Lehnert K, Teilmann J and Lohmann R (2021) Bioaccumulation of PCBs, OCPs and PBDEs in Marine Mammals From West Antarctica. Front. Mar. Sci. 8:768715. doi: 10.3389/fmars.2021.768715
Received: 01 September 2021; Accepted: 29 October 2021;
Published: 06 December 2021.
Edited by:
Begoña Jiménez, Consejo Superior de Investigaciones Científicas (CSIC), SpainReviewed by:
Tania Martellini, University of Florence, ItalyMandana Barghi, Korea University, South Korea
Copyright © 2021 Khairy, Brault, Dickhut, Harding, Harkonen, Karlsson, Lehnert, Teilmann and Lohmann. This is an open-access article distributed under the terms of the Creative Commons Attribution License (CC BY). The use, distribution or reproduction in other forums is permitted, provided the original author(s) and the copyright owner(s) are credited and that the original publication in this journal is cited, in accordance with accepted academic practice. No use, distribution or reproduction is permitted which does not comply with these terms.
*Correspondence: Rainer Lohmann, rlohmann@uri.edu
†Deceased