Recent Changes in Peatland Testate Amoeba Functional Traits and Hydrology Within a Replicated Site Network in Northwestern Québec, Canada
- 1ECRU, Ecosystems and Environment Research Programme, Faculty of Biological and Environmental Sciences, University of Helsinki, Helsinki, Finland
- 2Helsinki Institute of Sustainability Science (HELSUS), Helsinki, Finland
- 3Institute for Atmospheric and Earth System Research (INAR)/Physics, University of Helsinki, Helsinki, Finland
- 4Geography, College of Life and Environmental Sciences, University of Exeter, Exeter, United Kingdom
- 5Department of Geography, Université du Québec à Montréal, Montréal, QC, Canada
Northern peatlands, which are highly heterogeneous ecosystems, are a globally important carbon (C) store. Understanding the drivers and predicting the future trajectory of the peatland C store requires upscaling from cores and sites to regions and continents, alongside a detailed understanding of the mechanisms governing their C sequestration. Studies incorporating replication are therefore important to quantify how peatland heterogeneity may affect upscaling from local-scale dynamics to models. In addition, we need to better understand the processes driving observed variability, but the interplay between plants, microbes and C cycling in peatlands remains poorly understood. One approach to address both issues is to examine replicated microbiological functional traits within a multi-proxy framework to provide an ecosystem-level perspective on ecological and biogeochemical processes. Peatland testate amoebae are a functionally important group of protists that are well suited to such an approach. Analysing testate amoeba functional traits provides an opportunity to examine processes that may affect key peatland ecosystem services, such as C sequestration. Here, we compared four key testate amoeba functional traits (mixotrophy, biovolume, aperture size and aperture position) to C accumulation, hydrological and vegetation changes in 12 post-Little Ice Age peat records. Samples were collected from high-boreal and low-subarctic regions in northwestern Québec, Canada in an experimental design that includes internal and external replication at both site and regional scales. Our results showed that correspondence between C accumulation, hydrology and testate amoeba functional traits varied, but recent changes in mixotrophy and aperture size, which may affect peatland C sequestration potential and microbial food web structure, respectively, showed tentative links to recent C accumulation increases. Vegetation, especially Sphagnum abundance was important in promoting mixotrophy and small aperture size in testate amoeba communities. Future impacts of climate change on peatland vegetation will further influence the functional role of testate amoebae on C sequestration through changing mixotrophic testate amoeba abundance.
Introduction
Peatlands, as an important terrestrial carbon (C) store (Yu, 2012; Loisel et al., 2014; Nichols and Peteet, 2019), are sensitive to climate change due to consequent changes in hydrology, vegetation and microbial communities. Understanding the response of peatland C cycling to climate change requires comprehensive interpretation of ecosystem-climate feedbacks. Several studies focus on the interactions between peatland hydrology and vegetation (Magnan et al., 2018; Van Bellen et al., 2018; Zhang et al., 2020), revealing spatial and temporal variations in their response to climate changes. Testate amoebae, a group of single-celled protists that are abundant and diverse in Sphagnum-dominated peatlands, are key constituents of peatland microbial communities, representing a considerable portion of microbial biomass (Gilbert et al., 1998). They are essential parts of peatland microbial food webs and play critical roles in nutrient and C cycling (Jassey et al., 2015). However, studies on such peatland microbial communities and their interactions with climate change, and ultimate influences on peatland C accumulation, are limited, except via their use as hydrological proxies.
Testate amoeba functional traits have been shown to strongly relate to ecosystem processes (Fournier et al., 2015; Jassey et al., 2015; Marcisz et al., 2016). Traditionally, two categories of functional traits can be defined: (1) response traits (i.e., shell biovolume, shell compression and aperture position) that reflect species’ response to environmental change and (2) effect traits (i.e., aperture size and mixotrophy) that reveal species’ impact on ecosystem processes (Violle et al., 2007). However, some traits can also act as both response and effect traits. For example, mixotrophy responds both to moisture and landscape openness while it also meaningfully contributes to C sequestration in peatlands (Jassey et al., 2015; Herbert et al., 2019; Lamentowicz et al., 2020). Previous studies have shown links between these traits and warming (Jassey et al., 2015), peatland water table (Lamentowicz et al., 2020), fire and dust deposition (Marcisz et al., 2016, 2019) and light penetration (Herbert et al., 2019). However, the derived patterns also suggest a high degree of spatial and temporal variation in these traits (Fournier et al., 2015). Particularly, in terms of understanding peatland C cycling, more studies are needed to understand how mixotrophic testate amoebae vary temporally and spatially in response to external environmental drivers, as they contribute to photosynthetic C fixation (Gilbert et al., 1998). Peatland C fixation is generally estimated by taking into account only Sphagnum growth, without considering photosynthetic microorganisms, despite the fact that C intake during photosynthesis by mixotrophic testate amoebae may be significant at the ecosystem level (Jassey et al., 2015; Zhang et al., 2020).
Future climate change, defined by increasing temperatures, spatially variable precipitation trends and more extreme events (Collins et al., 2013) may cause environmental stress and disturbance, leading to shifts in peatland ecosystem structure and function, for example permafrost thawing (Swindles et al., 2015a), increased fire activity (De Groot et al., 2013) and vegetation turn over (Kokkonen et al., 2019). All these might cause changes in microbial communities and functional trait assemblages. Due to the high degree of observed spatial and temporal variability in derived patterns between testate amoeba functional traits and environmental changes, the consequences to microbial communities of these climate change- (or potentially autogenically driven-, see Gałka et al., 2017b) induced switches in peatland vegetation and hydrology are largely unknown, but may have important implications for C and nutrient cycling. The upscaling or modeling of global peatland C cycling under climate change is based on the patterns derived from local studies. In order to constrain the changes that we see in our data and provide better information for global estimates, there is a need to understand the local- and regional-scale impacts of these drivers and how they might vary between site types (e.g., permafrost thawing).
In this study, we examined testate amoeba functional traits, i.e., mixotrophy, biovolume, aperture size and aperture position, and two key environmental factors in peatlands, i.e., hydrology and vegetation, in 12 peat records collected from four peatlands of two regions in northern Québec, Canada. We aim to investigate (1) the spatial and temporal variations of peatland testate amoeba functional traits using replicated peat records at the site and regional scale, (2) the external drivers of variations in functional traits, and (3) the potential impacts of functional trait-environment interactions on peatland C cycling.
Materials and Methods
Study sites
The studied regions, Kuujjuarapik and Radisson, are located within the discontinuous and sporadic permafrost zones in northwestern Quebéc, Canada (Figure 1). Kuujjuarapik (peatland sites K1P and K2) represents the subarctic, forested tundra ecoregion at the southernmost limit of the discontinuous permafrost zone. K1P peatland is characterized by palsa mounds, whereas K2 is a small fen with peat thickness of ca. 1–2 meters. Radisson (peatland sites LG2 and Rad) represents the northernmost boreal ecoregion. LG2 and Rad are ombrotrophic with peat thickness of ca. 3–4 meters. For more details of the studied peatlands, please refer to Piilo et al. (2019). In both study regions, mean annual temperatures are below 0°C (Table 1). In Kuujjuarapik, mean summer temperature has increased 1°C since 1961, while mean winter temperature has increased 0.5°C since 1971, and the increase in growing degree-days (GDD0) (between 1971–2000 and 1981–2010) is ca. 6%. In Radisson, since 1971, the biggest seasonal increase of 0.5°C is in mean autumn temperatures, and the increase in GDD0 is ca. 4%. In both regions, autumn rainfall especially has increased, and 35–40% of the precipitation falls as snow (Environment Canada, 2019).
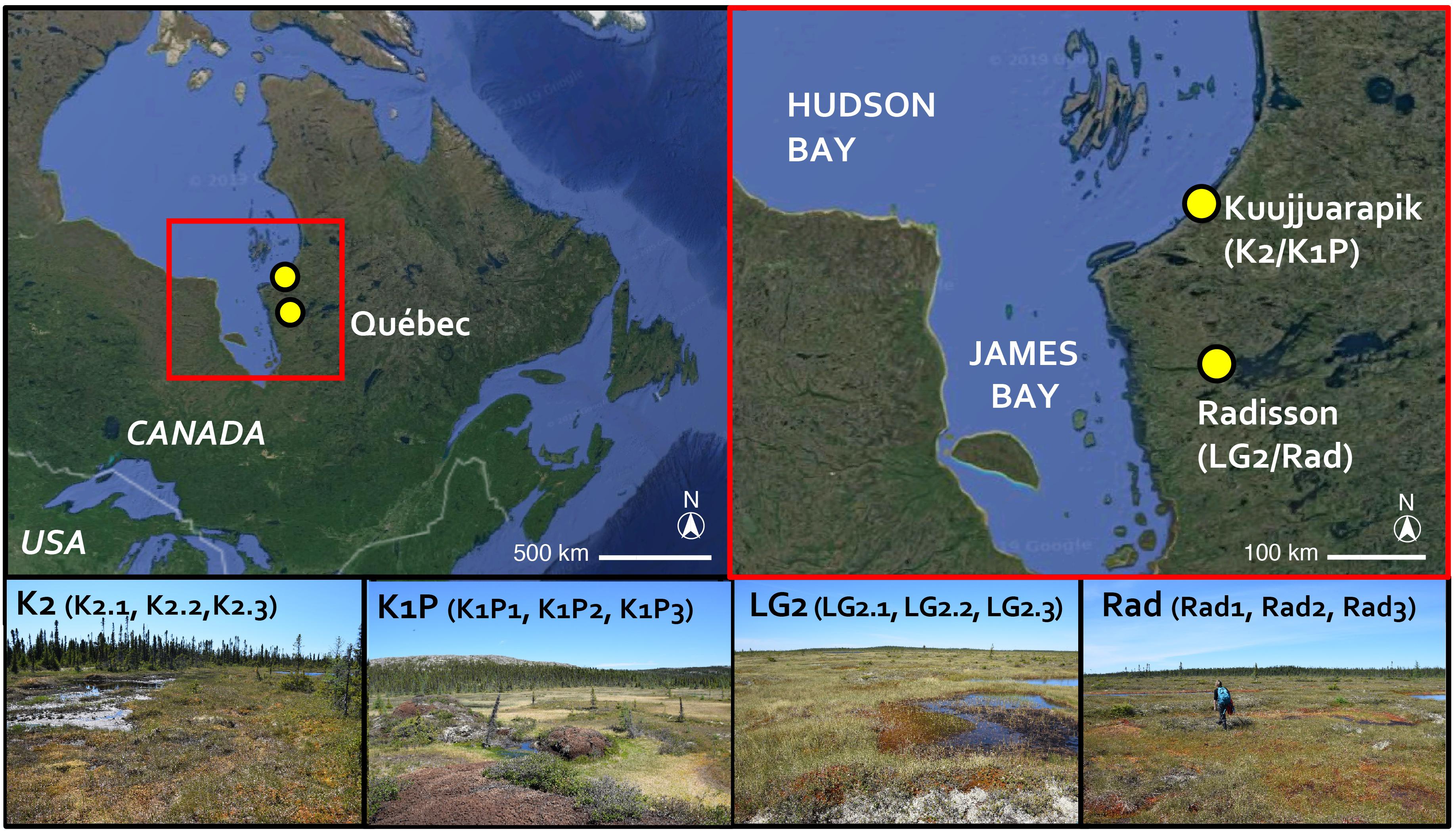
Figure 1. Study site locations marked with yellow dots (base map © 2020 Google). Peatlands K2 and K1P are in Kuujjuarapik; LG2 and Rad are in Radisson. Pictures showing the coring sites (© SP) with the replicated three cores in each site indicated.
Sampling
The studied 12 peat cores, three from each four peatlands (K2, K1P, LG2, and Rad), were collected in early July 2017 (Figures 1, 2 and Table 1). The core lengths ranged between 32 and 39 cm. Peat sections were sampled by hand, sawing the layer overlying seasonal frost or permafrost from intermediate lawn microforms (water tables ranged from 13 to 23 cm) inhabited mainly by Sphagnum fuscum. Once in the laboratory, the peat cores were cut into contiguous 1 cm slices for further analyses. Plant macrofossil, peat property and carbon accumulation results of these cores were previously published in Piilo et al. (2019).
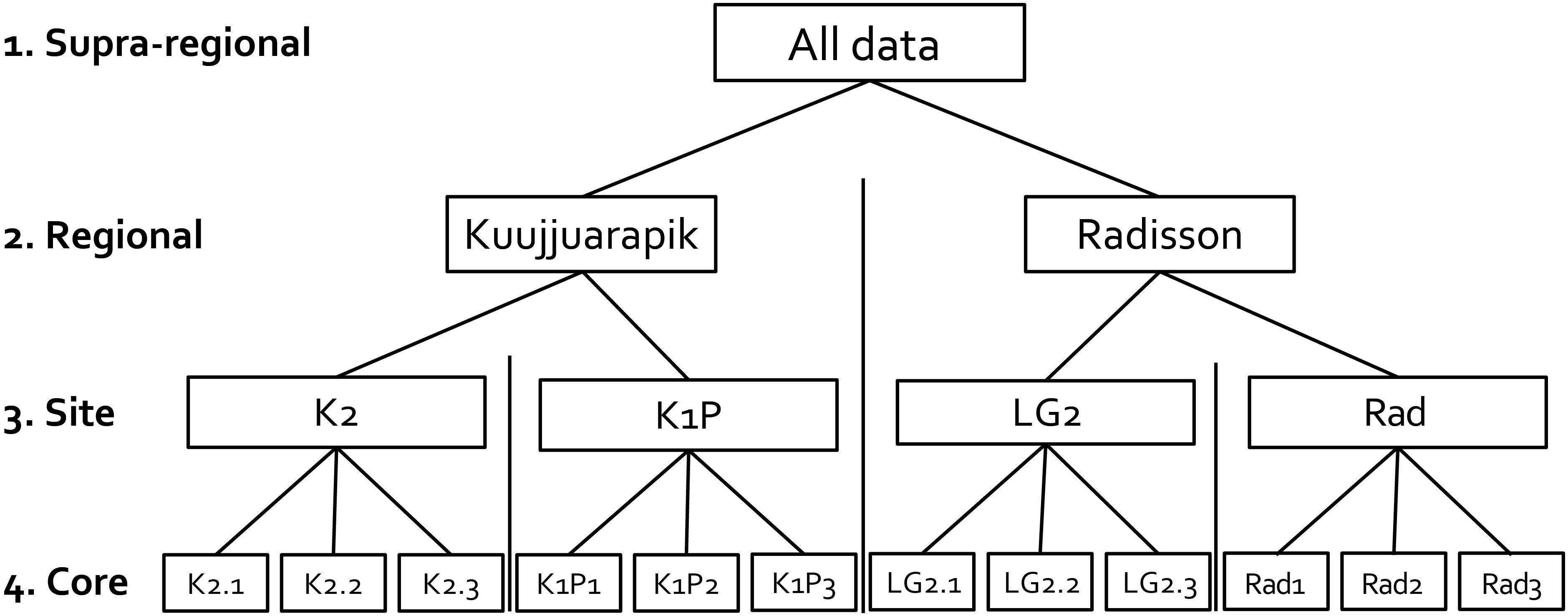
Figure 2. Illustration showing the replicated site network design in this study. Numbered terms represent different spatial scales.
Testate Amoeba Analysis
Testate amoeba analysis was performed at 2 cm resolution. Processing of testate amoeba samples followed a modified version of the standard method (Booth et al., 2010). Samples were simmered in distilled water for 15 min and stirred occasionally. The samples were then sieved with a 300-μm mesh and back sieved with a 15-μm mesh. Materials retained on the 15-μm sieve were centrifuged at 3000 rpm for 5 min. At least 100 individual shells for each sample were counted and identified to species or “type” level under a light microscope at the magnification of 200–400. Taxonomy followed Charman et al. (2000), supplemented with online sources (Siemensma, 2019).
Testate Amoeba Functional Trait Calculation and Data Analysis
We calculated the community weighted mean values of each standardized trait (Table 1; mixotrophy, biovolume, aperture size and aperture position), which is an index of functional composition expressed as the mean trait value of species present in the community, weighted by their relative abundances (Fournier et al., 2015). In addition, we calculated species richness and Shannon-Wiener diversity index (SWDI) for each sample. Values for test dimensions used to calculate all functional traits with the exception of mixotrophy were those published in the supplementary information of Fournier et al. (2015) except for species that were not included in that study. In those cases, we used average dimensions as described by Siemensma (2019) and in the case of one taxon (Pyxidicula type), an average of multiple replicates of our own light microscopic measurements. Mixotrophy was defined as a binary 0 (non-mixotroph) or 1 (mixotroph), also based on the definitions in Fournier et al. (2015).
Testate amoeba-based water-table depth (WTD) reconstructions were performed using the transfer function developed by Amesbury et al. (2018). The absolute WTD values were normalised to z scores over the length of cores from each region (Swindles et al., 2015b; Amesbury et al., 2016). Z > 0 indicates drier conditions than the region’s average, z < 0 indicates wetter than average. The analysis was conducted using “rioja” package in R version of 3.6.0 (R Core Team, 2019).
The chronologies for each peat record, developed using the “Bacon” package in R and based on 14C and 210Pb dating, were previously published in Piilo et al. (2019). A locally estimated scatterplot smoothing (LOESS) function with a span-value (degree of smoothing) setting of 0.5 was applied to the supra-regional, regional, site and core level data of C accumulation rate, reconstructed WTD and testate amoeba functional trait to explore the temporal trends and spatial variations. The analysis was conducted using the function loess () in R. Linear regression analysis (95% confidence intervals displayed) was applied to C accumulation and testate amoeba functional trait, and reconstructed WTD data using the function lm() in R.
A non-metric multidimensional scaling (NMDS) ordination analysis was applied to testate amoeba functional traits and reconstructed WTD, vegetation types (Piilo et al., 2019) and testate amoeba richness and diversity data to investigate the spatial variations and drivers of the recorded functional trait features. The analysis was performed using “vegan” package in R.
Results
The age-depth models show that peat accumulation rates varied, and individual core basal ages varied from ca. cal. −550 AD to ca. cal. 1960 AD. In general, peat accumulation rates increased toward the present, starting from the 1950s, even though peatland-specific variations in accumulation rates existed. However, this recent increase of peat accumulation rates might be partly due to the incomplete decomposition process and lower compaction of the surface peat. In spite of this uncertainty, by using specified focus periods, the data nevertheless enable spatial-temporal comparisons (Piilo et al., 2019). The focused time-period in this study is the past ca. 200 years, which covers the post-Little Ice Age warming and recent warming since 1850 AD. The plant macrofossil (Piilo et al., 2019), testate amoeba community, testate amoeba functional trait, and carbon accumulation (Piilo et al., 2019) data show some consistent features, but also clear core-, site- and region-specific variations (Figures 3–5).
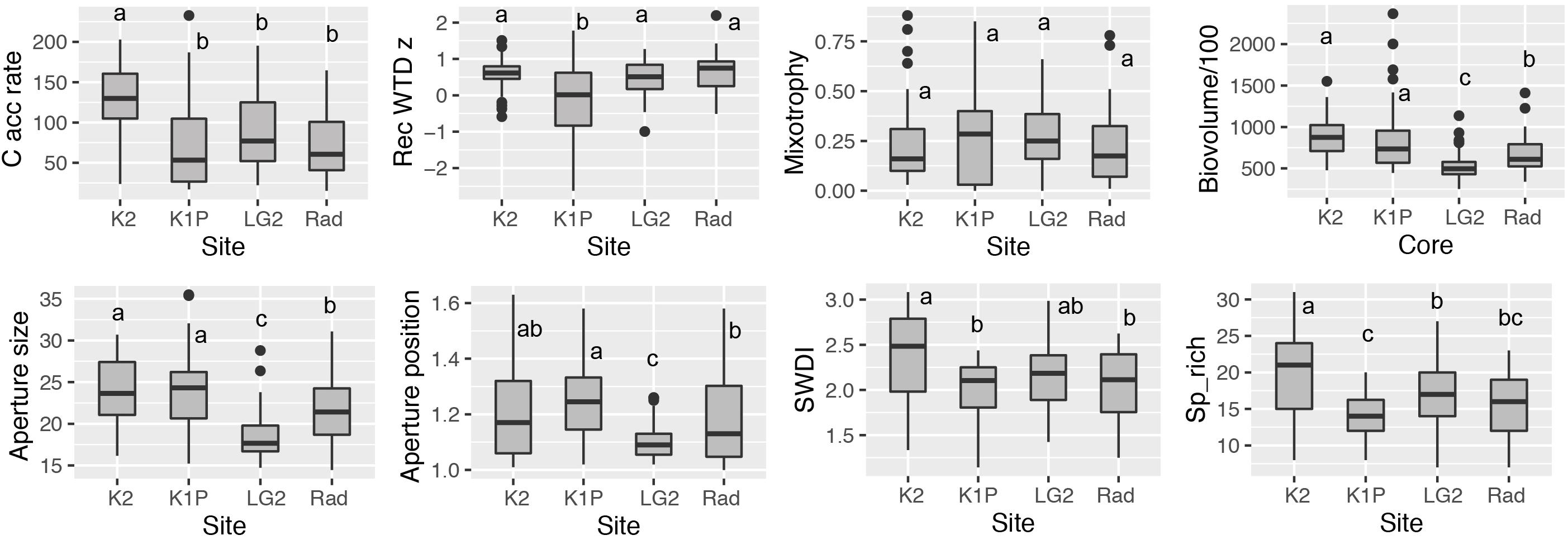
Figure 3. Box plots of carbon accumulation rate (C acc rate; g C m–2 yr–1), reconstructed water-table depth z score (Rec WTD z), mixotrophy, biovolume (μm3), aperture size (μm), aperture position, Shannon-Wiener diversity index (SWDI), species richness (Sp_rich) and at each site. Different letters indicate significant differences (p < 0.05) among sites calculated using the Turkey’s Honest Significant Difference method. Note the time span of each record included for each site varied and can be found in Figure 4.
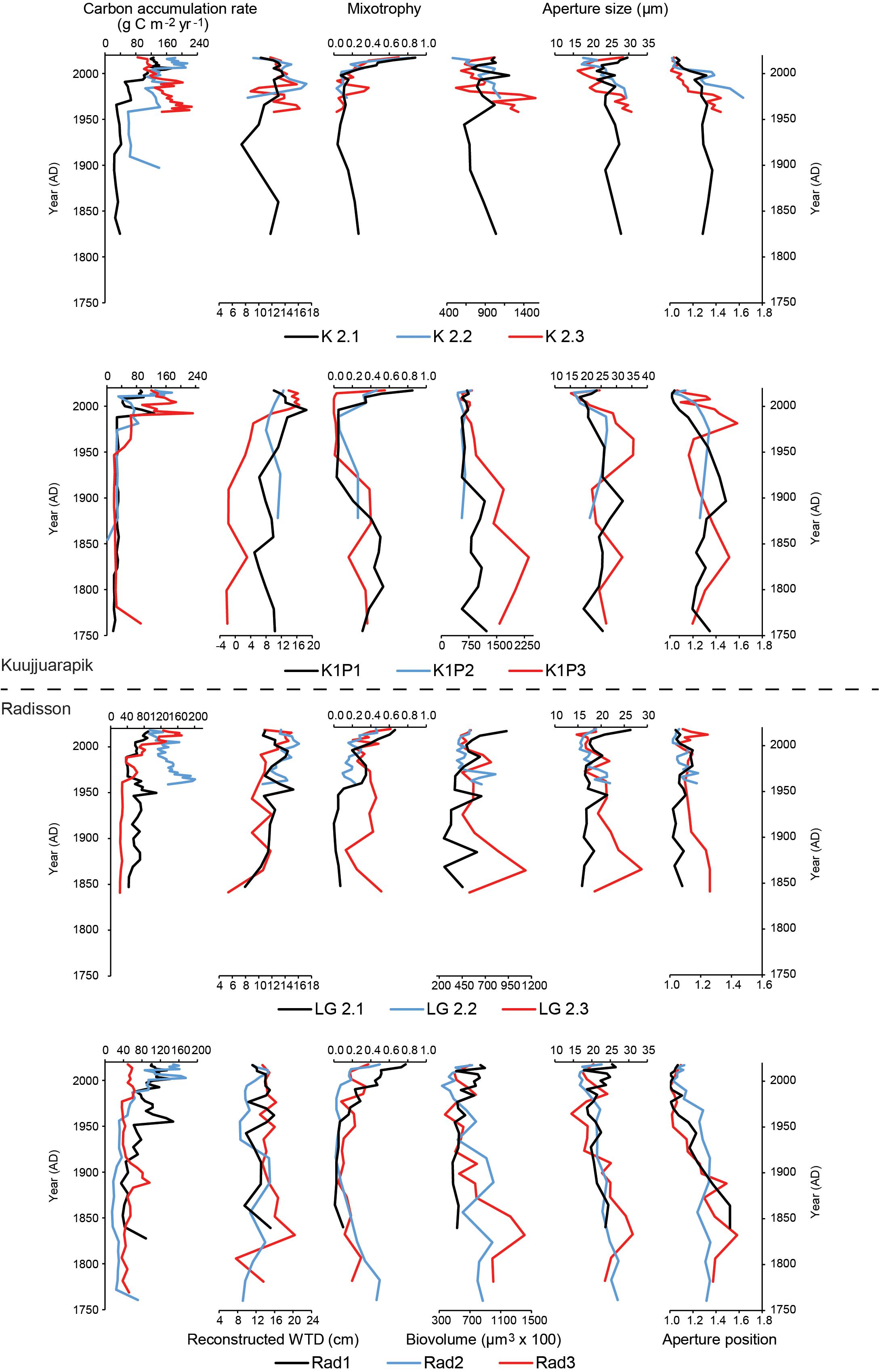
Figure 4. Diagrams of carbon accumulation rates, reconstructed water-table depth (WTD), mixotrophy, biovolume, aperture size and position of testate amoebae for the studied peat records.
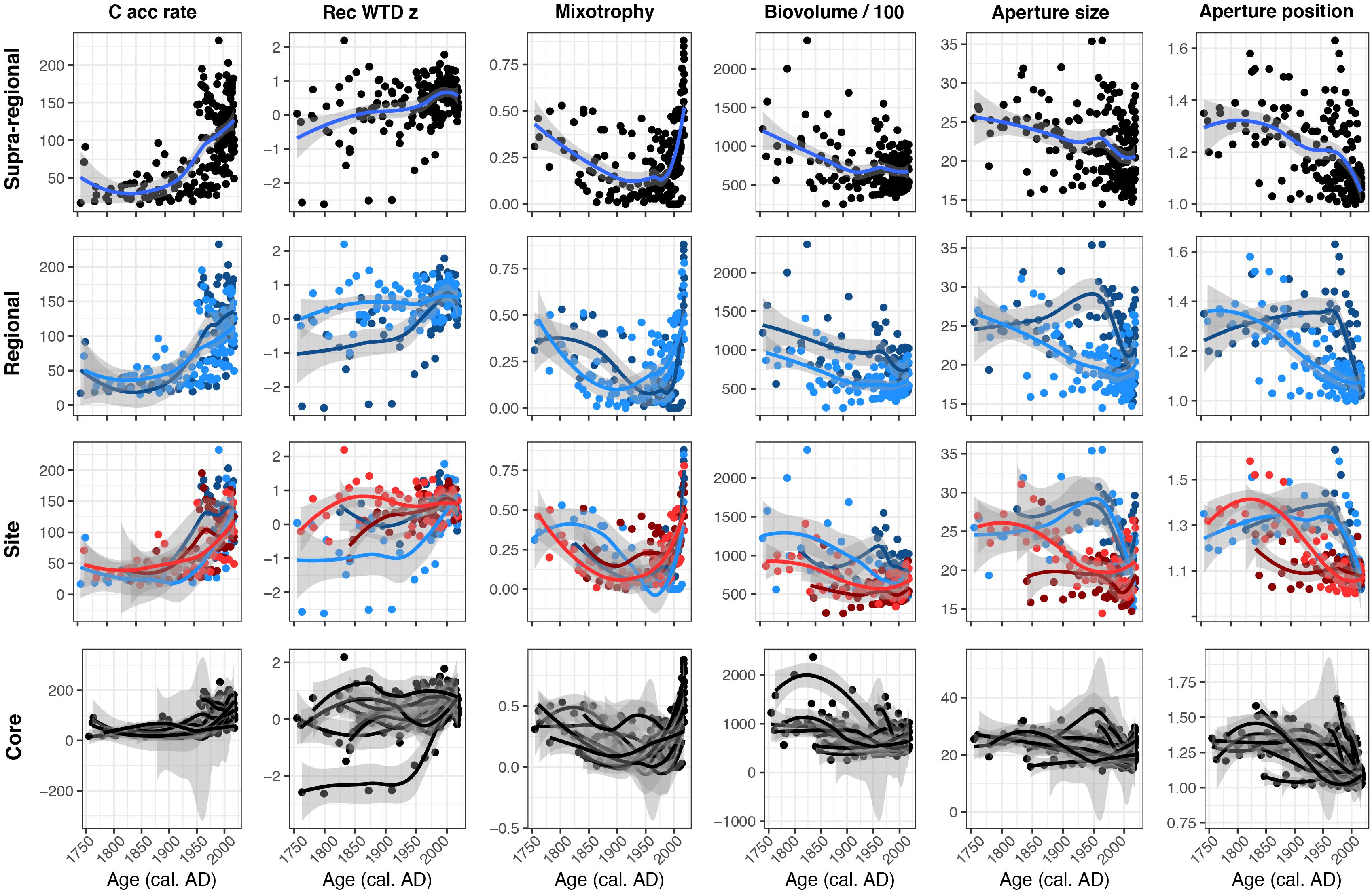
Figure 5. Locally estimated scatterplot smoothing plots showing supra-regional, regional, site and core level variations of carbon accumulation rates (C acc rate; g C m–2 yr–1), reconstructed water-table depth z score (Rec WTD z), mixotrophy, biovolume/100 (μm3), aperture size (μm) and aperture position. In the Regional panels, dark blue represents region Kuujjuarapik and light blue represents Radisson. In the Site panels, dark blue represents site K2 and light blue represents K1P, while dark red represents site LG2 and light red represents Rad.
Testate Amoeba Community and Reconstructed Water-Table Depth
In total, 64 taxa were found in the studied 12 peat records (Supplementary Figure S1). In general, the most commonly recorded taxa in all peat cores were Alabasta militaris, Archerella flavum, Hyalosphenia elegans, and H. papilio. Also, Difflugia pulex was dominant in Radisson cores. In addition, the Pyxidicula type (Supplementary Figure S2), currently unidentifiable to the species level, was found in all the peat records, and in some cases the proportion reached 50%. Site K2 showed higher species richness (average 21) than the other three sites (average around 15) (Figure 3). Sample-specific values for the Shannon-Wiener diversity index (SWDI) were higher at site K2 (average 2.5) than other sites (average 2.1). Temporally, both richness and diversity presented a remarkable decrease starting from ca. 1980 AD at 11 out of 12 records (Supplementary Figure S1). The compositional change of testate amoebae clearly indicated a consistent pattern in all the 12 records, i.e., mixotrophic taxa Hyalosphenia elegans, H. papilio and for some cores Heleopera sphagni became dominant (peak percentage around 50%) in the surface peat sections, even though the timing varied for different cores, ranging from early 1900 to late 1900 AD. Detailed testate amoeba community composition for each peat record can be found in Supplementary Figure S1.
Reconstructed water-table depth showed variations within each site and each region. The moisture conditions for the studied records mainly remained lawn features for the last few centuries. K1P site (especially core K1P3) recorded the largest variations and the wettest conditions (Figures 3, 4). Kuujjuarapik region was wetter than Radisson, especially for the period before 1950 AD (Figure 5), and Kuujjuarapik showed a clear drying starting from early 1900s, while the moisture conditions at Radisson remained relatively stable.
Testate Amoeba Functional Traits
Mixotrophy did not show significant differences between different sites, but a consistent increasing trend toward recent decades (Figures 3–5). The lowest mixotrophy occurred around 1950–2000 and 1900 at regions Kuujjuarapik and Radisson, respectively. Biovolume and aperture size were significantly higher at sites LG2 and Rad than K2 and K1P. Biovolume showed a general decreasing trend toward the present, even though some records showed an increasing trend (LG2.1 and Rad1). Aperture position at site LG2 was lower than other three sites. Regionally, aperture size and position at Radisson showed a decreasing trend toward the present, while at Kuujjuarapik first increased until 1950 AD and then started to decrease. In addition, aperture size and position were much higher at Radisson than Kuujjuarapik starting from 1900 to 2000 AD.
Functional trait, C accumulation and reconstructed WTD results at different spatial scales showed differing degrees of variability (Figure 5). C accumulation and mixotrophy results were relatively consistent across all spatial scales, showing recent increases. Reconstructed WTD values tended to show a relatively consistent trend toward drier conditions, but the regional level data showed this was a more recent and sharper trend in the Kuujjuarapik region, compared to a more gradual trend in the Radisson region. A similar pattern is evident in the aperture size and aperture position data: both showed consistent decreasing trends using all data, but regional level sub-division showed that the trends were more severe and recent in the Kuujjuarapik region and more gradual in the Radisson region. Biovolume tended to decrease over the study period, but regional level data showed that biovolume was generally higher in the Kuujjuarapik compared to Radisson region and core level data showed that the trend toward lower biovolume was much stronger in some cores, whereas others displayed relatively little change.
There were significant correlations between C accumulation and testate amoeba functional trait data, but the patterns were not consistent (Figure 6). For example, despite qualitatively similar recent increases in both C accumulation and mixotrophy across many cores (Figure 5), correlations between these factors were significant in both positive (K2.1, Rad1) and negative (K2.3, LG2.2) directions, even at the same site (K2) (Figure 6). Correlations between aperture size and aperture position were generally more consistent, with 3 of 4 and 6 of 7 significant correlations, respectively, between C accumulation and these functional traits across all sites being negative (Figure 6).
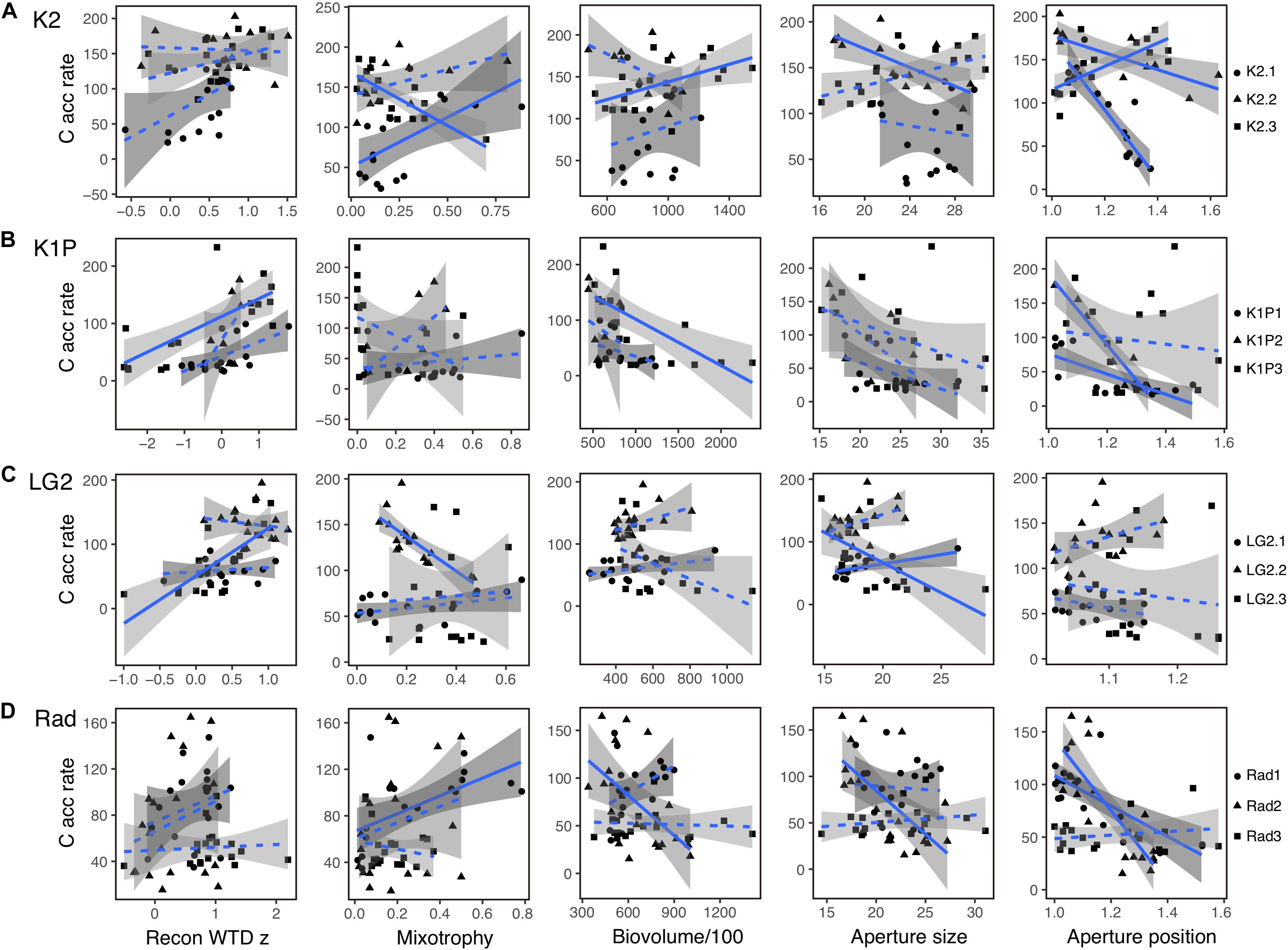
Figure 6. Linear regression analysis of carbon accumulation rate (C acc rate; g C m–2 y–1) and reconstructed water-table depth z score (Rec WTD z), mixotrophy, biovolume/100 (μm3), aperture size (μm) and aperture position for each peat record at sites K2 (A), K1P (B), LG2 (C) and Rad (D). The gray shading areas represent the 95% confidence intervals. Correlations with p < 0.05 were indicated using solid lines and correlations with p > 0.05 using dashed lines.
General Patterns of Testate Amoeba Functional Traits and Peatland Conditions
The NMDS results, i.e., no clear clusters of samples from a certain site, showed that the testate amoeba functional traits in each peatland were relatively homogeneous (Figure 7). Mixotrophy was highly linked to the first axis and clearly preferred habitats with Sphagnum mosses. Aperture size located on the other side of the first axis showed a shorter length, i.e., smaller aperture in Sphagnum-dominated habitats. The second axis can be explained by water table conditions, with the upper part indicating dry conditions with abundant woody species and the lower part indicating wetter conditions with sedges and non-Sphagnum mosses and higher testate amoeba richness and diversity. Biovolume was closely linked to the second axis, with larger values occurring in wetter conditions. Aperture position with hidden apertures showed a preference to drier conditions with abundant woody plants.
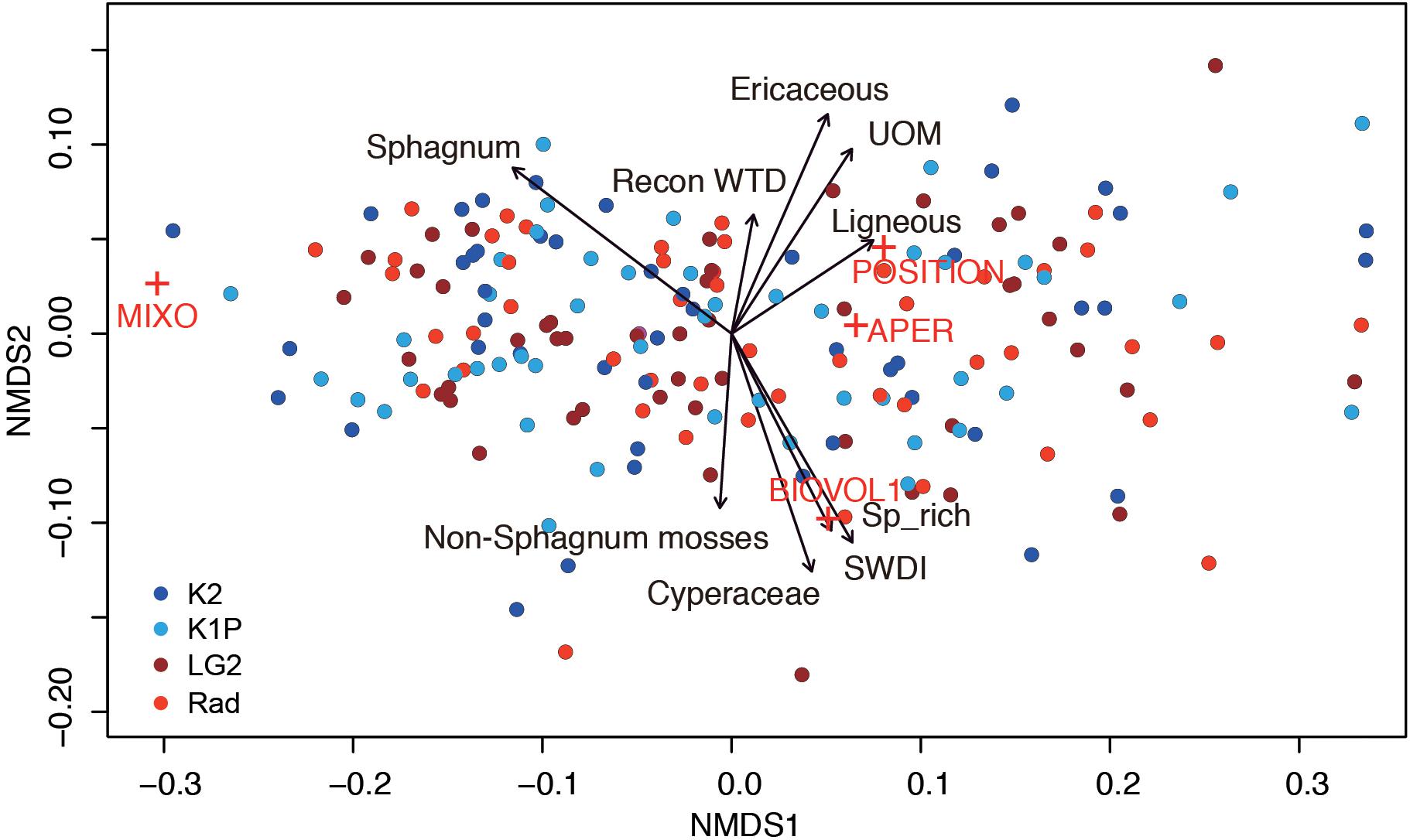
Figure 7. Non-metric multidimensional scaling of testate amoeba functional traits (stress: 0.04; MIXO: mixotrophy; BIOVOL: biovolume; APER: aperture size; POSITION: aperture position) with reconstructed water-table depth (WTD), testate amoeba richness (Sp_rich), diversity (SWDI), and vegetation types as environmental factors (all factors are significant with p < 0.01). UOM: Unidentifiable organic matter. Red crosses indicate testate amoeba functional traits and circles with the same color indicate samples from the same site.
Discussion
The studied peat cores, peatlands and regions differed in the patterns of inferred hydrology, vegetation, testate amoeba functional traits and carbon (C) accumulation. These differences further indicated that variations of peatland conditions affected the testate amoeba communities through their response traits and in the effect of these community changes for functioning of the microbial food web through their effect traits (Fournier et al., 2015 and references therein). In general, temporal trends of C accumulation, hydrology and testate amoeba functional traits observed in southern region Radisson were smoother compared with those in northern region Kuujjuarapik that were typically more recent and distinct. This could be linked to changes in thawing permafrost dynamics in the northern Kuujjuarapik region (Piilo et al., 2019).
Responses and Effects of Testate Amoeba Functional Traits on Peatland Environmental Change and Functioning
Changes in response traits at the community scale are inferred as being driven by changes in environmental conditions, while changes in effect traits may indicate shifts in process at the ecosystem scale. Previous studies have shown that correlations between response traits and effect traits can be used to determine how environmental change will influence ecosystem functioning (Lavorel and Garnier, 2002; Suding et al., 2008; Hillebrand and Matthiessen, 2009).
Biovolume and aperture position are suggested to be correlated with moisture, with low water level promoting smaller biovolume and hidden and protected apertures (Fournier et al., 2015; Marcisz et al., 2016). Our data strongly supported such links, especially for biovolume; that higher biovolume highly correlated with wetter conditions. Unlike biovolume, in addition to water table, aperture position might be influenced by other factors like the abundance of woody plants; for example, Rad1 had protected aperture (higher values of aperture position) in response to drier conditions, while for more records, e.g., LG2.2, Rad2, Rad3, K1P1, K1P2, K2.2, and K2.3, less protected aperture increased under wetter conditions. This suggests that variations in water level may not be a decisive factor for changing aperture position. Also, it may be explained by our field sampling design, i.e., all the peat cores were collected from lawn microforms with relatively good water availability, so the detected variations in water table were not strong enough to drive aperture position composition changes. Previous studies have also shown that larger disturbances, like flooding, fire, very low water tables and deforestation might have stronger impacts on such trait compositions (Marcisz et al., 2016, 2019). While dry conditions that supported woody plant growth was not a driver for aperture position, the altered vegetation composition (i.e., more woody plants) might nevertheless be challenging for testate amoebae to adapt to (Marcisz et al., 2016 and references therein).
Mixotrophy, seem to show relatively consistent increasing trends over recent decades, which are in line with the increased carbon accumulation rates, even though these increases of C accumulation rates might partly due to less decomposition of recently accumulated peats (Piilo et al., 2019). Mixotrophic testate amoebae that acquire most of their C by photosynthesis of their symbionts, essentially functioning as autotrophs (Lara and Gomaa, 2017) are suggested to play an important role in driving peatland C accumulation, with higher abundances promoting C accumulation (Marcisz et al., 2016; Zhang et al., 2020), but the opposite pattern can also be expected depending on the specific condtions. For example, in this study both positive and negative linear links between mixotrophic testate amoebae and C accumulation were derived (Figure 6), which might depend on the prey availability, i.e., mixotrophic testate amoebae either favour photosynthesis and form a positive link between C accumulation and their abundance, or predation and constitute a negative link between C accumulation and their abundance (Jassey et al., 2012, 2013; Mieczan et al., 2015). Furthermore, in most of our cases the larger the aperture size, the lower the C accumulated, which supports the latter mechanism. Although it has been shown before that lowering of water table leads to a decrease in the abundance of mixotrophs (Marcisz et al., 2016), this is not visible in our records. This might also be due to the sample collecting habitats, i.e., lawns that supply sufficient water and did not reach the threshold for changes of mixotrophy (Jassey et al., 2018; Koenig et al., 2018; Lamentowicz et al., 2019; Zhang et al., 2020). Instead, we found that increases in mixotrophy were linked to increased Sphagnum (mainly S. fuscum) abundance (Van Bellen et al., 2018). This suggests that mixotrophs appear to thrive under increasingly acidic and ombrotrophic conditions (Fournier et al., 2015). In addition, higher light intensity at the surface could be another potential reason for increased near surface mixotrophy (Marcisz et al., 2014; Payne et al., 2016; Creevy et al., 2018), even though increase of mixotrophy might also occur under low light intensity conditions (Herbert et al., 2019). Aperture size that indicates the trophic position of testate amoebae in the microbial food web (Jassey et al., 2013) was found to mainly present a negative correlation to C accumulation, however, an adverse pattern was also captured (Figure 6). Similar to mixotrophy, aperture size also showed no links to water table, but a negative response to increase of Sphagnum, with smaller size observed in Sphagnum-dominated habitats.
Implications of Peatland Testate Amoeba Functional Traits on Peatland Carbon Dynamics
As discussed above, mixotrophic testate amoebae might increase or decrease peatland C accumulation depending on different biotic conditions. Therefore, changes in their abundance will have impacts on peatland C dynamics. Our results show that the lowest abundance (close to zero) of mixotrophic testate amoebae occurred when Sphagnum dominance remarkably decreased. Post-LIA warming seems to play an important role in driving such vegetation change, followed by increasing of Sphagnum (Piilo et al., 2019) and correspondent mixotrophic testate amoebae, thus testate amoebae-peatland functioning. Particularly, the palsa site (K1P) showed synchronous vegetation changes, characterised by decreased Sphagnum abundance, for all records starting from ca. cal. AD 1950s and lasting until mid-1990s, which could be linked to changes in thawing permafrost dynamics in the site (Piilo et al., 2019). Increased snowfall since the 1950s and warm temperatures in the 1990s triggered rapid permafrost thawing on the eastern coast of Hudson Bay. Hence, permafrost is predicted to disappear from subarctic Québec in the coming decade (Payette et al., 2004), likely causing drying or wetting of the peat surface (Swindles et al., 2015a; Zhang et al., 2018b) and altering the vegetation (Zhang et al., 2018b; Piilo et al., 2019) and mixotrophic testate amoeba abundance, and ultimately changing peatland carbon dynamics.
In addition, boreal peatlands in, for example, western Canada are highly sensitive to fire because of the dry climate and high tree density, which sustains the spread of fire. Enhanced warming and drying during the 21st century will result in more intense regional fire regimes (De Groot et al., 2013). Fires were captured in 11 out of 12 studied records, especially in peatland Rad (Piilo et al., 2019). Atmospheric deposition of mineral dusts, whether they are supplied by natural or anthropogenic sources, may affect testate amoeba communities and their functional traits (Fialkiewicz-Koziel et al., 2015; Lamentowicz et al., 2015). For example, enhanced inputs of atmospheric dust are likely to limit mixotrophic testate amoeba abundance and therefore C uptake. However, the effects of drivers such as dust deposition are likely to have less effects on changes of testate amoeba taxa when compared with vegetation change, which partly depends on the volume of dust (Van Bellen et al., 2018), which seems to be supported also by our records that fires did not trigger clear shifts on testate amoeba composition.
Warming could enhance peatland C uptake due to increased growing season length (Charman et al., 2013; Gallego-Sala et al., 2018), more specifically increased plant photosynthesis. However, in terms of photosynthetic microorganisms, warming and subsequent changes in hydrology and vegetation all have impacts on mixotrophic testate amoebae. Studies have shown a reduction in mixotrophic testate amoebae with rising temperature (Wilken et al., 2013; Jassey et al., 2015) and lowering water tables (Marcisz et al., 2016; Basińska et al., 2020). However, one hypothesis is that global warming will lead into water level drawdown in peatlands, which favours new establishment of Sphagnum (Tahvanainen, 2011; Magnan et al., 2018). This Sphagnum-related change would likely to promote mixotrophic testate amoeba presence and mitigate the decrease of mixotrophic testate amoebae caused by warming and lowering of water table. On the other hand, drying might also cause shrub expansion (Gałka et al., 2017a; Zhang et al., 2018b) and decrease the openness of the peatland, thus hinder peatland C uptake through decreases in mixotrophic testate amoebae (Payne et al., 2016; Lamentowicz et al., 2020). Nevertheless, future climate change might influence peatland C sequestration through not only directly altering the hydrology, but also vegetation and consequent changes in mixotrophic testate amoebae, even though the magnitude and direction of such changes are not well constrained and might vary from one ecoclimatic region to another.
Future Perspective on Detecting Reliable Peatland-Climate Feedback Signal
The spatial and temporal variations detected within both peatlands and regions highlight the importance of studying multiple peat sections from one study site and preferably the approach should be extended to regional-scales (Loisel and Garneau, 2010; Lamarre et al., 2012; Mathijssen et al., 2017; Zhang et al., 2018a, b; Piilo et al., 2019). To enable a spatial comparison of our records, we collected peat cores consistently from lawn habitats, however, even in a particular site large differences between the three replicated cores were captured. It has been previously reported that different peatland habitats may also undergo different peatland-climate feedback pathways (Zhang et al., 2020). Any one site-based discussion of regional feedbacks is likely to be biased because different peatland types can experience specific successions under similar climate conditions, such as the differences observed between palsa K1P and fen K2 in region Kuujjuarapik in this study, let alone different regions that have different climate (Sim et al., 2019). This problem is exacerbated when within-site data, even taken from a consistent microform, is also variable. As one main aim of small-scale studies is to provide information for global modeling of peatland-climate feedbacks, we need to keep it in mind that any single core is likely not represent the overall site or regional response. Consistent signals detected from replicated records are definitely more convincing in terms of interpretations on peatland-climate feedbacks. However, due to practical issues for particular studies, we do not provide any specific suggestions here about, for example, the number of records that are needed for a reliable signal, but emphasize the need for multiple cores in peatland-climate feedback studies.
Data Availability Statement
The datasets presented in this study can be found in online repositories. The names of the repository/repositories and accession number(s) can be found in the article/Supplementary Material.
Author Contributions
MV, SP, AG-S, and MG collected the samples. MA analyzed the testate amoeba samples. HZ and MA performed the data analyses. HZ wrote the manuscript with MA. All other authors contributed with discussions and comments on the text.
Funding
This study was supported by the Academy of Finland (No. 296519) and Natural Sciences and Engineering Research Council of Canada discovery grant (NSERC; No. 250287).
Conflict of Interest
The authors declare that the research was conducted in the absence of any commercial or financial relationships that could be construed as a potential conflict of interest.
Acknowledgments
We thank N. Chaumont for fieldwork assistance. We thank the INQUA Congress 2019 for publishing an early version of the abstract in their conference proceedings. We thank the editor and reviewers for their constructive comments.
Supplementary Material
The Supplementary Material for this article can be found online at: https://www.frontiersin.org/articles/10.3389/fevo.2020.00228/full#supplementary-material
References
Amesbury, M. J., Booth, R. K., Roland, T. P., Bunbury, J., Clifford, M. J., Charman, D. J., et al. (2018). Towards a Holarctic synthesis of peatland testate amoeba ecology: development of a new continental-scale palaeohydrological transfer function for North America and comparison to European data. Q. Sci. Rev. 201, 483–500. doi: 10.1016/j.quascirev.2018.10.034
Amesbury, M. J., Swindles, G. T., Bobrov, A., Charman, D. J., Holden, J., Lamentowicz, M., et al. (2016). Development of a new pan-European testate amoeba transfer function for reconstructing peatland palaeohydrology. Q. Sci. Rev. 152, 132–151. doi: 10.1016/j.quascirev.2016.09.024
Basińska, A. M., Reczuga, M. K., Ga̧bka, M., Stróżecki, M., Łuców, D., Samson, M., et al. (2020). Experimental warming and precipitation reduction affect the biomass of microbial communities in a Sphagnum peatland. Ecolo. Indicat. 112:106059. doi: 10.1016/j.ecolind.2019.106059
Booth, R. K., Lamentowicz, M., and Charman, D. J. (2010). Preparation and analysis of testate amoebae in peatland palaeoenvironmental studies. Mires Peat 7, 1–7.
Charman, D. J., Beilman, D. W., Blaauw, M., Booth, R. K., Brewer, S., Chambers, F. M., et al. (2013). Climate-related changes in peatland carbon accumulation during the last millennium. Biogeosciences 10, 929–944. doi: 10.5194/bg-10-929-2013
Charman, D. J., Hendon, D., and Woodland, W. A. (2000). The Identification of Testate Amoebae (Protozoa: Rhizopoda) in Peats. Oxford: Quaternary Research Association.
Collins, M., Knutti, R., Arblaster, J., Dufresne, J.-L., Fichefet, T., Gao, P. F. X., et al. (2013). “Long-term climate change: projections, com- mitments and irreversibility,” in Climate Change 2013: The Physical Science Basis. Contribution of Working Group I to the Fifth Assessment Report of the Intergovernmental Panel on Climate Change, eds T. F. Stocker, D. Qin, G.-K. Plattner, M. Tignor, S. K. Allen, J. Boschung, et al. (Cambridge: Cambridge University Press).
Creevy, A. L., Andersen, R., Rowson, J. G., and Payne, R. J. (2018). Testate amoebae as functionally significant bioindicators in forest-to-bog restoration. Ecolo. Indicat. 84, 274–282. doi: 10.1016/j.ecolind.2017.08.062
De Groot, W. J., Flannigan, M. D., and Cantin, A. S. (2013). Climate change impacts on future boreal fire regimes. Forest Ecol. Manag. 294, 35–44. doi: 10.1016/j.foreco.2012.09.027
Environment Canada (2019). 1981-2010, 1961-1990 and 1971-2000 Climate Normals and Averages. Available online at: http://climate.weather.gc.ca/climate_normals/ (accessed December 4, 2019).
Fialkiewicz-Koziel, B., Smieja-Krol, B., Ostrovnaya, T. M., Frontasyeva, M., Sieminska, A., and Lamentowicz, M. (2015). Peatland microbial communities as indicators of the extreme atmospheric dust deposition. Water Air Soil Pollut. 226:97. doi: 10.1007/s11270-015-2338-1
Fournier, B., Lara, E., Jassey, V. E. J., and Mitchell, E. A. D. (2015). Functional traits as a new approach for interpreting testate amoeba palaeo-records in peatlands and assessing the causes and consequences of past changes in species composition. Holocene 25, 1375–1383. doi: 10.1177/0959683615585842
Gałka, M., Szal, M., Watson, E. J., Gallego-Sala, A., Amesbury, M. J., Charman, D. J., et al. (2017a). Vegetation succession, carbon accumulation and hydrological change in subarctic Peatlands, Abisko, Northern Sweden. Permafr. Perigl. Proces. 28, 589–604. doi: 10.1002/ppp.1945
Gałka, M., Tobolski, K., Lamentowicz, Ł, Ersek, V., Jassey, V. E. J., van der Knaap, W. O., et al. (2017b). Unveiling exceptional Baltic bog ecohydrology, autogenic succession and climate change during the last 2000 years in CE Europe using replicate cores, multi-proxy data and functional traits of testate amoebae. Q. Sci. Rev. 156, 90–106. doi: 10.1016/j.quascirev.2016.11.034
Gallego-Sala, A. V., Charman, D. J., Brewer, S., Page, S. E., Prentice, I. C., Friedlingstein, P., et al. (2018). Latitudinal limits to the predicted increase of the peatland carbon sink with warming. Nat. Clim. Change 8, 907–913. doi: 10.1038/s41558-018-0271-1
Gilbert, D., Amblard, C., Bourdier, G., and Francez, A. J. (1998). The microbial loop at the surface of a peatland: structure, function, and impact of nutrient input. Microb. Ecol. 35, 83–93. doi: 10.1007/s002489900062
Herbert, R. P., Peters, S. C., Nelson, D. M., and Booth, R. K. (2019). Light variability and mixotrophy: responses of testate amoeba communities and shell delta(13)C values to a peatland shading experiment. Eur. J. Protistol. 67, 15–26. doi: 10.1016/j.ejop.2018.10.005
Hillebrand, H., and Matthiessen, B. (2009). Biodiversity in a complex world: consolidation and progress in functional biodiversity research. Ecol. Lett. 12, 1405–1419. doi: 10.1111/j.1461-0248.2009.01388.x
Jassey, V. E., Signarbieux, C., Hattenschwiler, S., Bragazza, L., Buttler, A., Delarue, F., et al. (2015). An unexpected role for mixotrophs in the response of peatland carbon cycling to climate warming. Sci. Rep. 5:16931. doi: 10.1038/srep16931
Jassey, V. E. J., Meyer, C., Dupuy, C., Bernard, N., Mitchell, E. A. D., Toussaint, M. L., et al. (2013). To what extent do food preferences explain the trophic position of heterotrophic and mixotrophic microbial consumers in a sphagnum peatland? Microb. Ecol. 66, 571–580. doi: 10.1007/s00248-013-0262-8
Jassey, V. E. J., Reczuga, M. K., Zielińska, M., Słowińska, S., Robroek, B. J. M., Mariotte, P., et al. (2018). Tipping point in plant–fungal interactions under severe drought causes abrupt rise in peatland ecosystem respiration. Glob. Change Biol. 24, 972–986. doi: 10.1111/gcb.13928
Jassey, V. E. J., Shimano, S., Dupuy, C., Toussaint, M.-L., and Gilbert, D. (2012). Characterizing the feeding habitats of the testate amoebae Hyalosphenia Papilio and Nebela Tincta along a narrow “fen-bog” gradient using digestive vacuole content and 13C and 15N isotope analyses. Protist 163, 451–464. doi: 10.1016/j.protis.2011.07.006
Koenig, I., Mulot, M., and Mitchell, E. A. D. (2018). Taxonomic and functional traits responses of Sphagnum peatland testate amoebae to experimentally manipulated water table. Ecol. Indicat. 85, 342–351. doi: 10.1016/j.ecolind.2017.10.017
Kokkonen, N., Laine, A., Laine, J., Vasander, H., Kurki, K., Gong, J., et al. (2019). Responses of peatland vegetation to 15-year water level drawdown as mediated by fertility level. J. Veget. Sci. 30, 1206–1216. doi: 10.1111/jvs.12794
Lamarre, A., Garneau, M., and Asnong, H. (2012). Holocene paleohydrological reconstruction and carbon accumulation of a permafrost peatland using testate amoeba and macrofossil analyses, Kuujjuarapik, subarctic Québec, Canada. Rev. Palaeobot. Palynol. 186, 131–141. doi: 10.1016/j.revpalbo.2012.04.009
Lamentowicz, M., Galka, M., Lamentowicz, L., Obremska, M., Kuhl, N., Lucke, A., et al. (2015). Reconstructing climate change and ombrotrophic bog development during the last 4000 years in northern Poland using biotic proxies, stable isotopes and trait-based approach. Palaeogeogr. Palaeoclimatol. Palaeoecol. 418, 261–277. doi: 10.1016/j.palaeo.2014.11.015
Lamentowicz, M., Gałka, M., Marcisz, K., Słowiński, M., Kajukało-Drygalska, K., Dayras, M. D., et al. (2019). Unveiling tipping points in long-term ecological records from Sphagnum-dominated peatlands. Biol. Lett. 15:20190043. doi: 10.1098/rsbl.2019.0043
Lamentowicz, M., Kajukało-Drygalska, K., Kołaczek, P., Jassey, V. E. J., Ga̧bka, M., and Karpińska-Kołaczek, M. (2020). Testate amoebae taxonomy and trait diversity are coupled along an openness and wetness gradient in pine-dominated Baltic bogs. Eur. J. Protistol. 73, 125674. doi: 10.1016/j.ejop.2020.125674
Lara, E., and Gomaa, F. (2017). Symbiosis between testate amoebae and photosynthetic organisms. Algal Cyanobact. Symbio. 17, 399–419. doi: 10.1142/9781786340580_0013
Lavorel, S., and Garnier, E. (2002). Predicting changes in community composition and ecosystem functioning from plant traits: revisiting the Holy Grail. Funct. Ecol. 16, 545–556. doi: 10.1046/j.1365-2435.2002.00664.x
Loisel, J., and Garneau, M. (2010). Late Holocene paleoecohydrology and carbon accumulation estimates from two boreal peat bogs in eastern Canada: Potential and limits of multi-proxy archives. Palaeogeogr. Palaeoclimatol. Palaeoecol. 291, 493–533. doi: 10.1016/j.palaeo.2010.03.020
Loisel, J., Yu, Z. C., Beilman, D. W., Camill, P., Alm, J., Amesbury, M. J., et al. (2014). A database and synthesis of northern peatland soil properties and holocene carbon and nitrogen accumulation. Holocene 24, 1028–1042. doi: 10.1177/0959683614538073
Magnan, G., van Bellen, S., Davies, L., Froese, D., Garneau, M., Mullan-Boudreau, G., et al. (2018). Impact of the Little Ice Age cooling and 20th century climate change on peatland vegetation dynamics in central and northern Alberta using a multi-proxy approach and high-resolution peat chronologies. Q. Sci. Rev. 185, 230–243. doi: 10.1016/j.quascirev.2018.01.015
Marcisz, K., Colombaroli, D., Jassey, V. E. J., Tinner, W., Kolaczek, P., Galka, M., et al. (2016). A novel testate amoebae trait-based approach to infer environmental disturbance in Sphagnum peatlands. Sci. Rep. 6:33907. doi: 10.1038/srep33907
Marcisz, K., Lamentowicz, Ł, Słowińska, S., Słowiński, M., Muszak, W., and Lamentowicz, M. (2014). Seasonal changes in Sphagnum peatland testate amoeba communities along a hydrological gradient. Eur. J. Protistol. 50, 445–455. doi: 10.1016/j.ejop.2014.07.001
Marcisz, K., Lamentowicz, M., Gałka, M., Colombaroli, D., Adolf, C., and Tinner, W. (2019). Responses of vegetation and testate amoeba trait composition to fire disturbances in and around a bog in central European lowlands (northern Poland). Q. Sci. Rev. 208, 129–139. doi: 10.1016/j.quascirev.2019.02.003
Mathijssen, P. J. H., Kähkölä, N., Tuovinen, J.-P., Lohila, A., Minkkinen, K., Laurila, T., et al. (2017). Lateral expansion and carbon exchange of a boreal peatland in Finland resulting in 7000 years of positive radiative forcing. J. Geophys. Res. 122:3749. doi: 10.1002/2016JG003749
Mieczan, T., Michał, N., Adamczuk, M., and Bielańska-Grajner, I. (2015). Stable isotope analyses revealed high seasonal dynamics in the food web structure of a peatbog. Int. Rev. Hydrobiol. 100, 141–150. doi: 10.1002/iroh.201501788
Nichols, J. E., and Peteet, D. M. (2019). Rapid expansion of northern peatlands and doubled estimate of carbon storage. Nat. Geosci. 12, 917–921. doi: 10.1038/s41561-019-0454-z
Payette, S., Delwaide, A., Caccianiga, M., and Beauchemin, M. (2004). Accelerated thawing of subarctic peatland permafrost over the last 50 years. Geophys. Res. Lett. 31:L18208. doi: 10.1029/2004gl020358
Payne, R. J., Creevy, A., Malysheva, E., Ratcliffe, J., Andersen, R., Tsyganov, A. N., et al. (2016). Tree encroachment may lead to functionally-significant changes in peatland testate amoeba communities. Soil Biol. Biochem. 98, 18–21. doi: 10.1016/j.soilbio.2016.04.002
Piilo, S. R., Zhang, H., Garneau, M., Gallego-Sala, A., Amesbury, M., and Väliranta, M. (2019). Recent peat and carbon accumulation following the Little Ice Age in northwestern Québec, Canada. Environ. Res. Lett. 14:075002. doi: 10.1088/1748-9326/ab11ec
R Core Team (2019). R: A Language and Environment for Statistical Computing. Vienna: R Foundation for Statistical Computing.
Siemensma, F. J. (2019). Microworld, world of Amoeboid Organisms. World-Wide Electronic Publication, Kortenhoef, the Netherlands. Available online at: https://www.arcella.nl (accessed March 31, 2019).
Sim, T. G., Swindles, G. T., Morris, P. J., Galka, M., Mullan, D., and Galloway, J. M. (2019). Pathways for ecological change in canadian high arctic wetlands under rapid twentieth century warming. Geophys. Res. Lett. 46, 4726–4737. doi: 10.1029/2019gl082611
Suding, K. N., Lavorel, S., Chapin, F. S., Cornelissen, J. H. C., Diaz, S., Garnier, E., et al. (2008). Scaling environmental change through the community-level: a trait-based response-and-effect framework for plants. Glob. Change Biol. 14, 1125–1140. doi: 10.1111/j.1365-2486.2008.01557.x
Swindles, G. T., Morris, P. J., Mullan, D., Watson, E. J., Turner, T. E., Roland, T. P., et al. (2015a). The long-term fate of permafrost peatlands under rapid climate warming. Sci. Rep. 5:17951. doi: 10.1038/srep17951
Swindles, G. T., Holden, J., Raby, C. L., Turner, T. E., Blundell, A., Charman, D. J., et al. (2015b). Testing peatland water-table depth transfer functions using high-resolution hydrological monitoring data. Q. Sci. Rev. 120, 107–117. doi: 10.1016/j.quascirev.2015.04.019
Tahvanainen, T. (2011). Abrupt ombrotrophication of a boreal aapa mire triggered by hydrological disturbance in the catchment. J. Ecol. 99, 404–415. doi: 10.1111/j.1365-2745.2010.01778.x
Van Bellen, S., Magnan, G., Davies, L., Froese, D., Mullan-Boudreau, G., Zaccone, C., et al. (2018). Testate amoeba records indicate regional 20th-century lowering of water tables in ombrotrophic peatlands in central-northern Alberta, Canada. Glob. Change Biol. 24, 2758–2774. doi: 10.1111/gcb.14143
Violle, C., Navas, M.-L., Vile, D., Kazakou, E., Fortunel, C., Hummel, I., et al. (2007). Let the concept of trait be functional! Oikos 116, 882–892. doi: 10.1111/j.0030-1299.2007.15559.x
Wilken, S., Huisman, J., Naus-Wiezer, S., and Van Donk, E. (2013). Mixotrophic organisms become more heterotrophic with rising temperature. Ecol. Lett. 16, 225–233. doi: 10.1111/ele.12033
Yu, Z. (2012). Northern peatland carbon stocks and dynamics: a review. Biogeosciences 9, 4071–4085. doi: 10.5194/bg-9-4071-2012
Zhang, H., Gallego-Sala, A. V., Amesbury, M. J., Charman, D. J., Piilo, S. R., and Väliranta, M. M. (2018a). Inconsistent response of arctic permafrost peatland carbon accumulation to warm climate phases. Glob. Biogeochem. Cycles 32, 1605–1620. doi: 10.1029/2018GB005980
Zhang, H., Piilo, S. R., Amesbury, M. J., Charman, D. J., Gallego-Sala, A. V., and Väliranta, M. M. (2018b). The role of climate change in regulating Arctic permafrost peatland hydrological and vegetation change over the last millennium. Q. Sci. Rev. 182, 121–130. doi: 10.1016/j.quascirev.2018.01.003
Keywords: testate amoebae, functional traits, palaeohydrology, vegetation, carbon accumulation, replicated sites, peatlands, climate change
Citation: Zhang H, Amesbury MJ, Piilo SR, Garneau M, Gallego-Sala A and Väliranta MM (2020) Recent Changes in Peatland Testate Amoeba Functional Traits and Hydrology Within a Replicated Site Network in Northwestern Québec, Canada. Front. Ecol. Evol. 8:228. doi: 10.3389/fevo.2020.00228
Received: 27 April 2020; Accepted: 23 June 2020;
Published: 10 July 2020.
Edited by:
Vincent Jassey, UMR 5245 Laboratoire Ecologie Fonctionnelle et Environnement (ECOLAB), FranceReviewed by:
Valentyna Krashevska, University of Göttingen, GermanyKatarzyna Marcisz, Adam Mickiewicz University, Poland
Copyright © 2020 Zhang, Amesbury, Piilo, Garneau, Gallego-Sala and Väliranta. This is an open-access article distributed under the terms of the Creative Commons Attribution License (CC BY). The use, distribution or reproduction in other forums is permitted, provided the original author(s) and the copyright owner(s) are credited and that the original publication in this journal is cited, in accordance with accepted academic practice. No use, distribution or reproduction is permitted which does not comply with these terms.
*Correspondence: Hui Zhang, hui.zhang@helsinki.fi