Recycling of Biogas Digestates in Crop Production—Soil and Plant Trace Metal Content and Variability
- Faculty of Environmental Sciences and Natural Resource Management, Norwegian University of Life Sciences, Akershus, Norway
Production of biogas and utilization of the resulting digestates as fertilizers has been increasing in Europe in the last few decades. Depending on the feedstock used for the anaerobic digestion process, these organic fertilizers may be a source of different pollutants, such as trace metals. When applied to soils, digestates may influence the natural metal content and enhance the release of metals to the environment since they can be rich in different trace metals and are usually rich in dissolved organic matter. This study focused on investigating metal presence, seasonal variability of their soluble forms and crop uptake in a 2-year field experiment, using two different biogas digestates as fertilizers. The use of digestates as fertilizers was compared to cattle manure, mineral fertilizer and a control without fertilizer addition, with respect to the presence and distribution of the trace metals Cd, Cu, Zn, Ni, Cr, Mn, and Mo, as well as Al in the soil and plant. The results of the study showed that both biogas digestates have caused a 10–20% increase in the total soil concentration of Ni, Cd and Cr compared to control plots without fertilizer addition. Application of biogas digestates had only a minor effect on metal uptake in plants. Overall, the selected application rate of 100 kg/ha of plant available nitrogen has had little effect on plant metal uptake and crop quality and the use of biogas digestates was comparable to the use of animal manure.
Introduction
Over the last few decades, the use of different organic residues as fertilizers has increased. For instance, anaerobic digestion for biogas production results in large amounts of liquid digestate, which contains high amounts of nutrients, such as nitrogen, potassium and phosphorus, and micronutrients in plant-available forms. The fertilization benefit of digestates are well documented (Möller and Müller, 2012; Nkoa, 2014) and long-term application of digestates has been seen to improve soil quality, stimulate crop yields, and even influence positively on the soil bacteria growth (Abubaker et al., 2012).
The most common substrates for biogas production are animal manures (Fantozzi and Buratti, 2009), food waste collected from the municipal households and sewage sludge (Maragkaki et al., 2018) or co-digestion of several substrates. Most of the studies have focused on the use of manure or sewage-sludge based digestates with an emphasis on their nutrient and fertilizer value (Ni et al., 2017; Yun et al., 2018). There are few studies with digestates based on source-separated food waste focused on the optimization of digestate quality as fertilizer through co-digestion with other materials, such as sewage sludge (Borowski et al., 2018). Recent findings have shown that food-waste based digestates can be used for growing vegetables and mushrooms, especially when anaerobic digestion is combined with hydroponics (Stoknes et al., 2016).
Modern crop production is focused on both good yield and grain quality, but also on preservation of the environment. There are studies showing that both animal manure (Nicholson et al., 1999; Yang et al., 2017) and sewage sludge (Ashworth and Alloway, 2004; Chen et al., 2017) contain trace metals in variable concentrations. Still, sewage sludge application to agricultural land is strictly regulated because of the trace elements build up in soil, in particular elements such as cadmium, mercury, lead, and chromium. Further, soil leaching studies for trace metals have showed high ecological risk factors with the application of organic wastes such as compost or manure (Cambier et al., 2014). Pollutants may be applied to soil through inorganic or organic fertilizers and may bioaccumulate in soil organisms and plants (Antoniadis et al., 2017a). The organic matter quality and structure in biogas digestates is dependent on the biogas production feedstock and the applied technology, which means that it can have an effect on the trace metal availability. Soil quality is an important factor for crop quality and yield, and will be affected negatively by accumulation of trace metals or other pollutants. One challenge with the field application of biogas digestates is their chemical safety (Törnwall et al., 2017). Naturally, the content of potential contaminants in substrates used for the biogas process determines the content of these contaminants in the digestates. Metal content in biogas digestates and its potential implications for the use as fertilizer has been a subject of increasing interest in recent years (Kupper et al., 2014; Parraga-Aguado et al., 2017). Environmental effects of added trace metals, such as uptake into plants and soil organisms or leaching to water recipients, depends on their mobility in the soil, which in turn is strongly affected by pH and dissolved organic carbon (DOC) content (Antoniadis and Alloway, 2002; Welikala et al., 2018). For instance, Cu, Zn, Cd, and Ni, are generally mobile in soil under common conditions for crop production in a Nordic climate, i.e., in a pH range between ~6 and 7. On the other hand, As, Sb, and Hg may precipitate in insoluble forms under these conditions. Applying organic fertilizers represents an addition of DOC and may influence the pH in the soil and may thus increase the risk of plant uptake or leaching to water recipients.
In the field experiment described in this study, two digestates based on food waste were used, one digested alone and one co-digested with sewage sludge. The study has focused on the presence and availability/water extractability of the trace metals Cd, Cu, Zn, Ni, Cr, Mn, and Mo, as well as Al in soil after applying biogas digestates and animal manure in a field experiment conducted over two growing seasons. Aluminum was included because one of the digestates studied contains large amounts of Al from phosphate removal in sewage sludge with Al salts. The main hypothesis of this study was that the application of biogas digestates as organic fertilizers would increase the mobility and thus plant availability of trace metals in soil. Results of the study were used to calculate a contamination factor for each metal based on the concentrations from the soil top layer.
Materials and Methods
Organic Fertilizers
For the field experiment, two commercially produced biogas digestates and cattle manure were used as organic fertilizers. The digestates (DIG) were produced at two municipal biogas plants in the southeastern part of Norway. The respective companies use different feedstock mixtures and different technologies for biogas production. The digestate marked as DIG1 is derived from pretreated (steam-exploded) source-separated household and industry food waste as substrate for the anaerobic digestion process. The second digestate, DIG2, was produced using a mixture of source-separated food waste and sewage sludge (ratio 50:50) as substrate.
The manure was a cattle slurry from the farm at the Norwegian University of Life Sciences in Ås (Norway). Before chemical analysis, all the samples were stored at 4°C, in the case of NH4 and NO3 analysis no longer than 48 h. Selected characteristics of digestates and manure used in both growing seasons are given in Table 1.
Field Experiment
The field experiment was located at Ås, southeastern Norway (59°39′52″N 10°47′40″E) on a loam soil (13% sand, 57% silt, and 30% clay, as determined by the pipette method; Elonen, 1971). The soil was an epistagnic Retisol according to the World Reference Base for Soil Resources (IUSS working group WRB, 2015). It had a pH value of approximately 6.0, a total organic matter content of 4.2% and total nitrogen content of 0.21% prior to the experiment. The field experiment was started in 2014.
The experiment was organized as a randomized complete block design with three replicates, growing barley (Hordeum vulgare L.) in 2015 and oats (Avena sativa L.) in 2016 as test crops. The field was spring-plowed and harrowed before adding the different fertilizers in April 2015 and mid-May 2016, respectively. The fertilizers were incorporated into the soil by harrowing, and fields were sown within 48 h after application of fertilizers. The size of the experimental area was 39 m × 24 m (0.094 ha), and the size of each treatment plot was 3 × 7 m (0.0021 ha).
Treatments consisted of a control without fertilizer addition (NN), mineral fertilizer (MF), animal manure (AM), and the two digestates. The mineral fertilizer used was an NPK fertilizer with an NPK ratio of 22:3:10. The applied rate per hectare was 100 kg N, 14 kg P, and 45 kg K. The amounts of digestates and manure were determined based on plant-available N NO3-N and NH4-N, Table 1) measured in the samples and corresponded to 100 kg N ha−1. In a recent study it was found that the content of inorganic nitrogen in digestates was a good estimation of the fertilizer value (Sogn et al., 2018). In Norway, farmers are currently obliged to register N amounts but not P addition from organic fertilizers such as manure.
Table 2 shows total amounts and the main nutrients N, P, and K applied with organic fertilizers in kg ha−1.
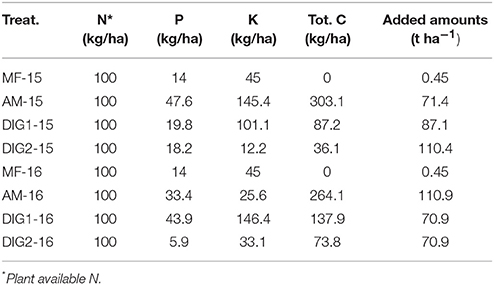
Table 2. Amounts of nitrogen (N), phosphorus (P), potassium (K), and total carbon (Tot. C) added in kg per hectare for both growing seasons.
The average yields for the 2015 seasons were 1.87 t ha−1 NN (control plot), 5.31 t ha−1 MF, 4.96 t ha−1 AM, 6.18 t ha−1 DIG1 and 5.64 t ha−1 DIG2. For 2016 season, the yields were 1.2 t ha−1 for NN (control plot), 4.0 t ha−1 for MF, 2.2 t ha−1 for AM, 3.9 t ha−1 for DIG1 and 4.0 t ha−1 for DIG2 plots. The data from 2016 was used for calculating total metal content in oat grains.
Sample Preparation and Chemical Analysis
In general, the metal content in digestates depends on substrate and applied technology for biogas production. The trace metals investigated in this study are either under Norwegian or EU regulation for application of organic fertilizers, or selected based on previous research findings. Aluminum was included in the analysis because of its high content in the sewage sludge used for the production of DIG2, which in turn is due to removal of phosphorus with Al-salts before the digestion process.
The soil pH was determined by using dried soil with a soil to water ratio of 1:2.5. Total carbon content in dried soil, digestate, and manure samples was determined by a dry combustion method using a Leco Carbon Determinator EC12 (Nelson and Sommers, 1982). Water-soluble concentrations of metals were determined by extracting 5 g of soil/fresh digestates/fresh manure with 25 mL deionized water (Ashworth and Alloway, 2004). Soil water extractions are considered to be good in assessing the plant nutrient availability (van der Paauw, 1971; Sonneveld, 1990). Water-soluble concentrations of metals were analyzed within 48 h after preparing and filtering (Milipore, 0.45 μm) the extracts. Water extracts of manure and digestates were also analyzed for DOC by using a Shimadzu TOC analyzer. The concentration of NO3-N and NH4-N in the organic fertilizers was determined in fresh samples by extraction with 2 M KCl and flow injection analysis using FIAstar 5000, FOSS.
For trace metal analysis, soil was sampled from the plow layer (0–20 cm) of each plot once a month from June to August. In 2015, plant material was collected at the same time, whereas in 2016, only grain samples were taken at harvest. The plant samples were dried at 55°C for 5 days and then stored before sample preparation and analysis. Prior to total metal analysis, the soil, digestate, and manure samples were dried (24 h, 105°C), sieved and ground. A sample of 0.2–0.3 g was digested in concentrated ultrapure nitric acid (HNO3) prior to ICP-MS analysis by stepwise (90 min) heating up to 250°C using a Milestone Ultraclave. For the analysis of metal concentrations in soils, digestates, plants, and extracts, an Agilent ICP-MS 8800 TripleQ was used. The ICP-MS analysis has followed minor method validation (depending on the sample matrix) while certified reference materials and reference materials were used for quality control of the applied analytical methods. The filtered samples (extracts of soils and fertilizers) were prepared in 10 % ultrapure nitric acid prior to analysis.
Statistics and Calculations
Analysis of variance (one-way and two-way ANOVA) was carried out to determine the effect of treatment on total metal concentrations in soil and grains. Two-way ANOVA was applied to the water extract concentrations after digestate application with treatment and sampling time as factors. Different means were separated by t-test. Pearson correlation was carried out to determine the pH and DOC correlation to the water-soluble metal concentration in soil. The statistical programs used were R Commander 3.2.3 and Sigma Plot 14.0. The confidence limit was 95% (p < 0.05).
Principal component analysis (PCA) is a dimension reduction technique, which may be used to demonstrate potential source and spatial distribution of metals in field experiments (Zhang et al., 2018). In the present study, the principal components (PC) were identified by the analysis of the correlation matrix for the data set regarding the water-extracted soil concentrations prepared from soils collected from the field experiment. The PCA analysis was used to identify factors underlying our set of variables (fertilizer treatments, sampling time) in order to determine relationships among them. Results were also used to investigated clustering of samples into groups based on their correlations.
A soil contamination factor (Antoniadis et al., 2017b; Shaheen et al., 2017) was calculated in order to evaluate the potential of long-term accumulation of investigated metals by applying the selected rates of fertilizers. The contamination factor, CF, was determined by the following formula (1):
Where, ci represents the mean total concentration of the metal in soil of the organic fertilizer treatment, and cn that of the unfertilized control.
Results
Total Metal Concentration in Digestates and Manure
The total concentrations of trace metals and Al in digestates and manure used in 2015 and 2016 are given in Table 3. With the exception of Cr, Ni, and Cd (DIG1 2016), trace metal concentrations were below limits set for use in agriculture (Class I). Norwegian regulation in regards to the use of organic fertilizers categorizes all organic wastes in four classes (class 0, 1, 2, and 3). For comparison we have used class 1, since only class 0 and 1 are allowing the use of organic wastes with no or minimum limitations (Authority, 2006). Nickel concentrations in both digestates were higher in 2016 than in 2015. The Cr concentrations, although high, were not increased between 2015 and 2016. Due to the technology used, Al concentration were high in DIG2 in both years. Lead (Pb) concentrations ranged from 0.5 to 6.0 mg kg−1 (data not shown), which was well below regulation values (Class I fertilizers, 60 mg kg−1).
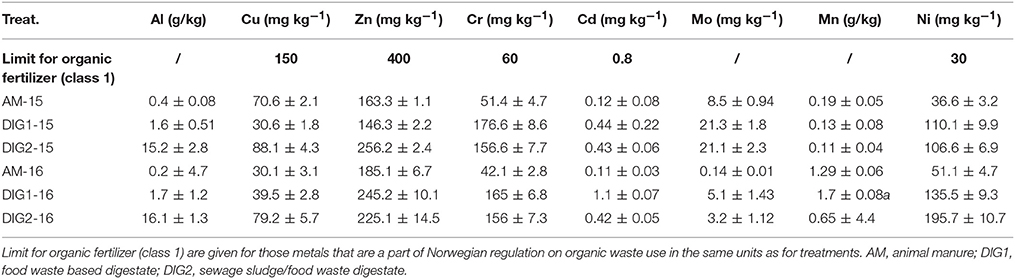
Table 3. Concentration of investigated metals (± standard deviation) for biogas digestates 241 and animal manure from the years 2015 and 2016.
Total Concentration of Metals in Soil
Total metal concentrations in the MF treatment were almost identical to those in the control plots (NN), and results from both NN and MF are presented. Total soil trace metal concentrations are given in Figure A1.
When compared to control plots, total soil concentrations of Ni, Cr, Cd, Cu, Zn were significantly increased in the digestate treatments. For Cr, there was a significant decrease from June to August in both seasons. For Ni, the same significant decrease can be seen. For Zn, results from 2016 showed significant increase in total Zn concentration with biogas digestates used as treatments.
Soil pH and DOC
Generally, pH was higher in NN and AM plots than in the digestate plots at all samplings, with the largest differences in the beginning of the season. The results from MF plots are not included in Figures 1, 2 since the values were not significantly different from the NN plots. For both growing seasons, the soil pH from control plots (NN) was in the range 5.8–6.0. Soil pH varied over the course of both growing seasons and depended on the treatment type (Figure 1). The pH decreased in both growing seasons from levels around 6.0 to the lowest 5.1. The lowest values for 2015 was DIG1 and for 2016 DIG2 in respect to August samples.
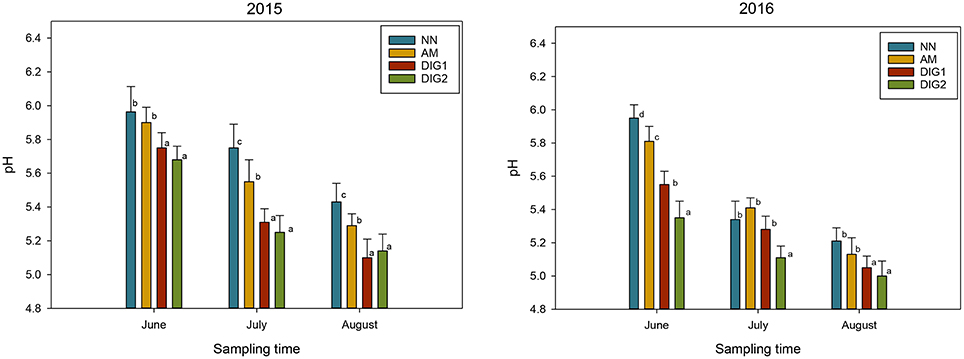
Figure 1. Soil pH in from plots treated with different fertilizer treatments in 2015 and 2016. Letters show significant difference between each of the treatments (p < 0.05).
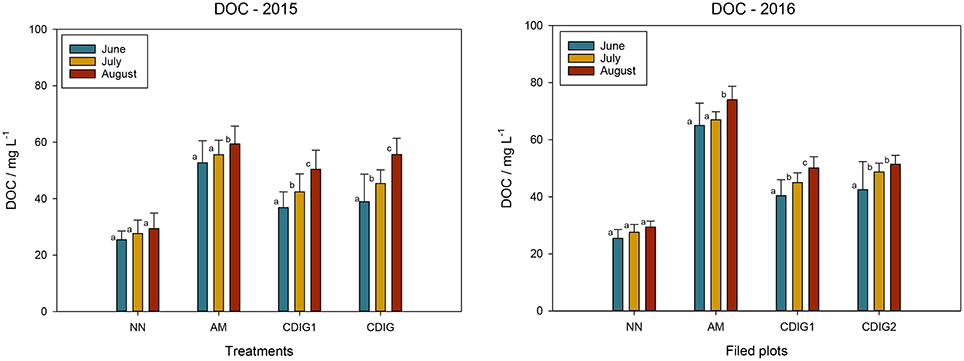
Figure 2. Dissolved organic carbon in soil extracts from soil samples taken from the different plots with fertilizer treatments in 2015 and 2016. Different letters indicate significant differences between treatments at one sampling date (p < 0.05). The extracts were prepared by adding 5 g of soil in 25 mL of deionized water.
In 2016, a significantly lower pH was found for DIG2 compared to the other treatments at all sampling points.
The addition of the organic fertilizers significantly increased DOC concentrations in the soil for the duration of the growing season. In both growing seasons, the effect was most pronounced in the AM treatment (Figure 2).
Water-Soluble Metal Concentrations in Soil
In general, water-soluble concentrations of the trace metals increased in all treatments over the course of the growing season, both in 2015 and 2016 (Figure 3). Concentrations were similar in both years with the exception of Cd, where concentrations were higher in all treatments and at all sampling dates in 2016 compared to 2015. As no significant differences in water-soluble concentrations were observed between treatments for Mn and Mo, the data is not included in Figure 3, but concentrations followed the same overall pattern with increasing values over the growing season.
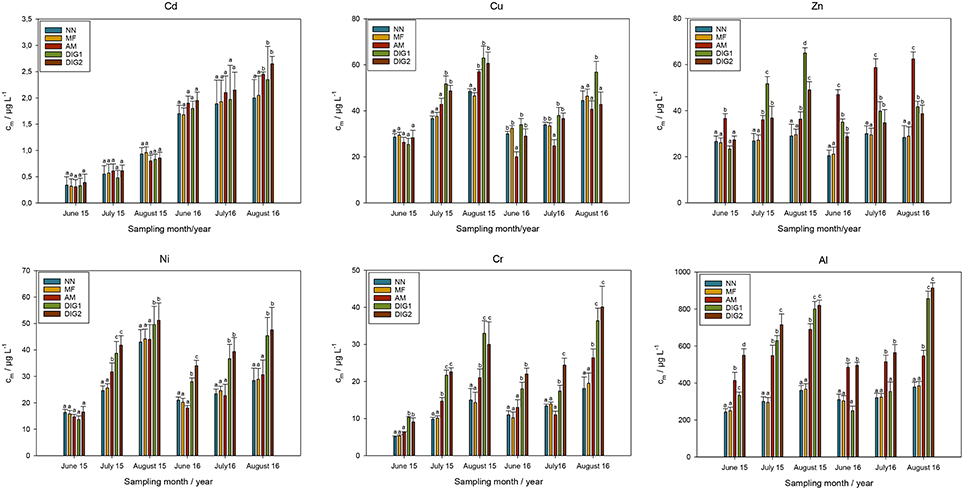
Figure 3. Selected water-soluble metal concentrations in soil extracts (growing seasons 2015 and 2016). The results are presented as means ± SD. Different letters indicate significant differences between treatments at each sampling time (p < 0.05). The extracts were prepared by adding 5 g of soil in 25 mL of deionized water.
There were no differences in Cd, Cu, and Ni concentrations between treatments at the first sampling date in 2015 and in the case of Cd, differences between treatments remained low throughout the season. In 2016, concentrations at the first sampling were generally higher for Cd than in 2016, and concentrations increased more with time in the organic treatments than in the control. For Cu, higher concentrations were found in the organic and especially in the digestate treatments at the 2nd and 3rd sampling date in 2015. In 2016, however, the manure treatments had lower concentrations even than the control at the first two sampling dates and only DIG1 addition resulted in higher concentrations than the control. The pattern for Ni was similar in 2015 and 2016 with highest concentrations in both digestate treatments at the last two sampling dates, whereas the manure treatment was on a similar level to the control.
Zn, Cr, and Al already showed significant differences in concentrations at the first sampling date. In the case of Zn, the manure treatment showed higher concentrations than the other treatments both at the first sampling date in 2015 and 2016. For most dates, the concentrations in the organic treatments were significantly increased compared to the control. In July and August 2015, highest concentrations were found in the DIG1 treatment, whereas in 2016, the manure treatment remained the one with highest Zn concentrations throughout the season. Cr concentrations were highest in the two digestate treatments at all sampling dates but also generally also enhanced in the manure treatment compared to the control. Addition of the digestate with the high Al content (DIG2) resulted in higher concentrations of water-soluble Al at all sampling dates compared to the control, but the other two organic treatments showed similar levels either early in the growing season (manure) or at the later sampling dates (DIG1). In the latter case, Al concentrations were at the same low level as in the control early in June but increased strongly until August.
Concentrations of the investigated metals from June to August sampling were in general negatively correlated with pH, with the exception of Zn, and positively correlated with DOC, with R-values above 0.75.
Additional statistical consideration of the results from the section of water-soluble metal concentration was used to assess the influence of the addition of different treatments, pH and DOC. The results of two-way ANOVA analysis are given in Table 4.

Table 4. Results of two-way ANOVA analysis with defined factor of significance for the concentration of selected metals measured in soil extracts using treatments and sampling time as factors and their interactions.
Water-soluble concentrations of Cu, Cr, and Ni were significantly affected by the factor treatment. Between the factors treatment and sampling time, the F-value is indicating that the sampling time had a more significant role especially for Cd, Cu, and Ni.
The PCA analysis was performed on the entire data set of measured concentrations from June to August for the 2016 season. In Table 5, the results for component loadings and eigenvalues of each PC are presented. PC1 had an eigenvalue >2 whereas PC2 had a value below 1 (0.8). Since only PCs with eigenvalues equal to or above 1 can be taken into consideration, only PC1 can be used to explain the variability.
The data structure and component loadings of the first two components, PC1 and PC2 are shown by use of a biplot (Figure 4). The first two components describe approximately 97% of the variance (PC1 85%, PC2 12%).
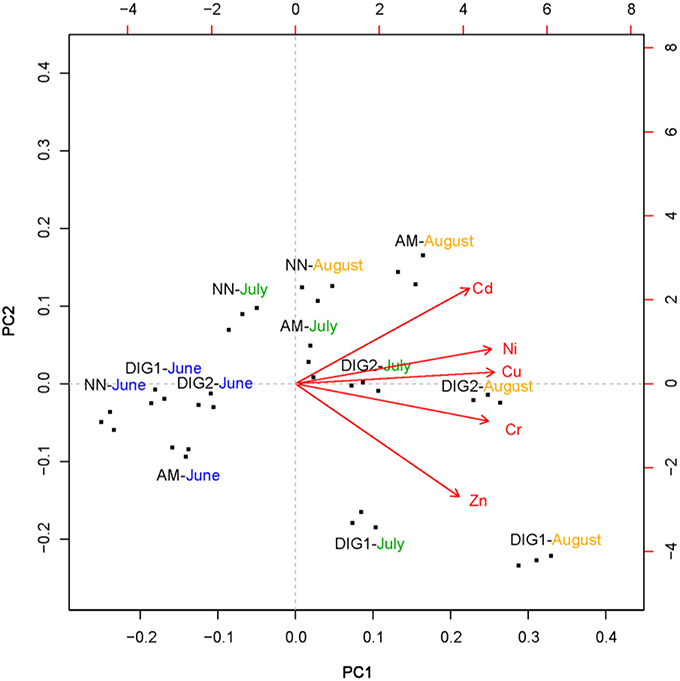
Figure 4. Biplot PC2 vs. PC1 illustrating the loading of each component and clustering (association) of treatments for different sampling periods (growing season 2016).
For all the metals, PC1 values were positive. Loading values were above 0.4 for all metals except for Zn. Most of the treatments formed a cluster, except for the DIG1 in July and August (Figure 4). The clustering of treatments is strongest for June and July sampling while for August the clustering was more dispersed. Clustering in this type of experimental setup usually implies that used treatments have a similar effect on the metal concentration variability.
Total Metal Concentrations in Plant Material
In 2015, plants metal content was determined at three times during the growing season at the same time as the soil samples were taken. In 2016, grain metal content was analyzed at harvest. Total concentrations of metals in plant material are given in Figure 5. For Cd, during the growing season there was no significant difference between the treatments, where a concentration dilution effect is seen from June to August. Grain concentrations from 2016 were below 0.1 mg kg−1 for all treatments. A different uptake scenario was seen for the plant nutrients Cu and Zn, where total plant concentrations are significantly affected only by the digestates treatments (DIG1 and DIG2). Grain concentrations for Cu were around 0.2 mg kg−1 while for Zn they were around 25 mg kg−1.
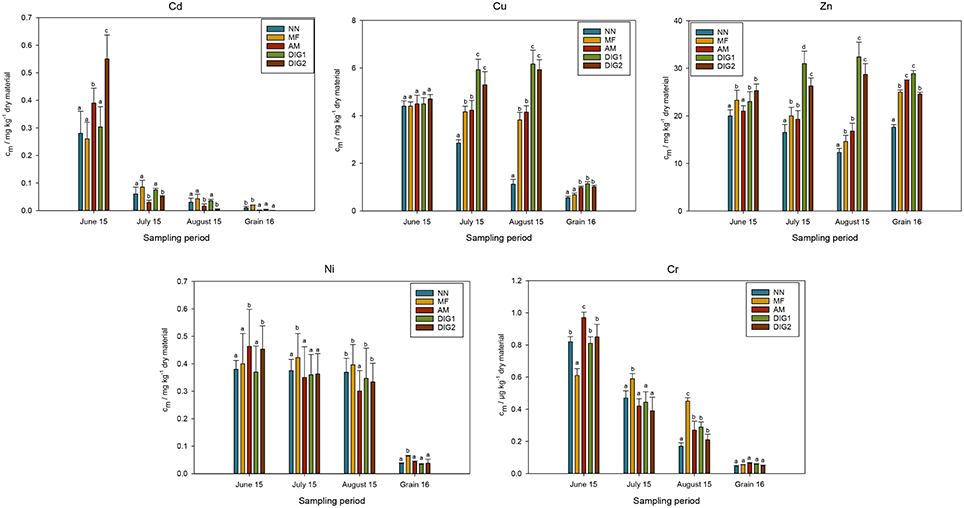
Figure 5. Total concentration of selected metals in aboveground plant parts sampled during growing season 2015, and in barley grains harvested in 2016. Letters are showing significance of differences between the treatments (p < 0.05).
For treatments in season 2015, there was no significant difference in Ni concentrations during the growing season. There were no significant differences in Cr concentration for the used treatments in the sampled plant material for each of the sampling points (season 2015). The same dilution effect as seen for Cd can be also seen for Cr. In general, Cr concentration was lower than concentration of other metals detected in the plant material. The highest concentration of Cr in plant material was found in the plots treated with AM. Still, the grain concentrations in 2016 were very low (below 0.2 μg kg−1 of dry plant material).
Calculated amounts of trace metals taken up per hectare combine the concentrations in grains given in Figure 5 with the yield (section Field Experiment, year 2016) of the different treatments. Thus, it can be seen (on a per hectare basis) what proportion of the trace metals added with the fertilizers is removed by harvesting.
The highest concentration was seen for Zn, while the lowest was seen for Cd, Ni, and Cr. After Zn, Cu was taken up and transported to the grains in highest amounts.
Contamination Factors for Soil Treated With Biogas Digestates
The potential metal contamination effect was assessed by using data from the total soil concentration from both growing seasons for the calculation of the contamination factor. Calculated contamination factors (CF) are given in Figure 7, using the formula from section Statistics and Calculations.
The general classification of the level of contamination available from the literature (Antoniadis et al., 2017a) usually divides CF into three classes, CF < 1, low degree, 1 ≤ CF < 3, moderate contamination and 3 ≤ CF < 6 with high degree of contamination. For 2015 within the experimental sampling the increase can be seen for Ni (CF = 1.05), Cr (CF = 1.2), and Al (CF = 1.3) for DIG2 treatment (Figure 6). In the calculation of CF value from 2016 there is increase for Cd (CF = 1.4) and Cr (CF = 1.2) for both DIG treatments. Figure 6 shows that the rest of the investigated trace metals have a low or moderate level of contamination.
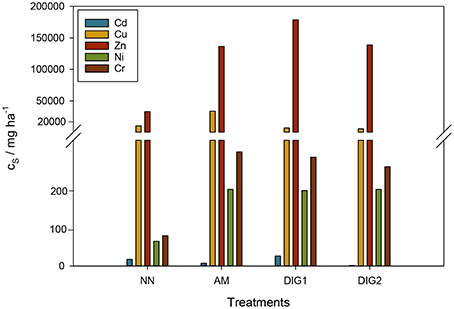
Figure 6. Selected trace metals content (Cs) as mg of trace metals in grain per hectare. Figure break is set to 350 mg ha−1.
Discussion
The trace metals in focus in this study were both plant micronutrients (i.e., Cu, Zn, Ni, Mn, and Mo), and those that are in general not considered to be important or even harmful for plant growth (Cd, Cr, and Al). However, both groups of metals can negatively influence both plant development and soil quality (including runoff water) if present in excessive amounts. The addition of digestate has resulted in a significant increase in the total soil metal concentration, especially in the case of Ni and Zn, but also Cr and Cd. The water-extractable concentrations in the soil have significantly increased for Ni, Zn, and Cr with addition of digestate or manure in both growing seasons. A decrease in pH had an important role in the general increase of the water-soluble concentration of cationic forms of investigated metals. Still, the significant change in the pH and the addition of metals with biogas digestates did not influence metal uptake in plant material from 2015 or in the grain samples from 2016. The PCA has shown that the water-extractable concentrations of trace metals from plots treated digestate are similar to the ones measured for animal manure. In addition, clustering of specific sample points during one growing season as presented in PCA results (biplot) also contributes to the claim that digestates exhibit similar effects when compared to AM.
Influence of Biogas Digestates Fertilization on Trace Metals as Plant Micronutrients
Application of biogas digestates as fertilizers for both growing seasons has significantly increased Zn and Ni total soil content, while Cu, Mn, and Mo were not significantly increased (Figure A1).
Measured levels of total soil Zn are a result of the digestates/manure Zn content, which was in a range 150–250 mg kg−1 (Table 3). Under Mediterranean conditions, suggested adequate Zn content is in a range of 100–300 mg kg−1 soil for cereal production (Brunetti et al., 2012). Still, for the north of Europe the values are usually in range of 47–61 mg/kg, which means that the measured concentrations are within the suitable levels for growing cereal crops (Kabata-Pendias, 2011). A similar effect of Zn addition was seen in a published 2-year field study with the use of organic waste based fertilizer (composted municipal solid waste) where measured values of total Zn concentration in soils were also under 300 mg kg−1 and comparable to our experimental results (Yuksel, 2015). The addition of DIG1 treatment significantly increased the total Zn content especially in growing season 2016. Still, measured values for AM treatment were comparable to the values of DIG1. The concentration of Ni in digestates was high. This resulted in significantly higher total Ni soil concentrations (Figure A1) when compared to the NN or MF plots. Suitable levels for total Ni concentration in soil is around 50 mg kg−1 for a normal agricultural activity. The levels in our study were below a toxicity threshold (above 100 mg kg−1 in soil) and well within the suitable levels (35–55 mg kg−1) for crop production in general (Kabata-Pendias, 2011; Brunetti et al., 2012).
The observed changes for Zn and Ni water-soluble concentrations for DIG treatments were comparable to the levels measured for the AM treatment, especially in the case of Zn. Zn water-extractable concentration in the soil were not significantly higher than the ones measured for AM in season 2016 (Figure 3). The mentioned comparison clearly shows that the Zn added through digestates shows similar behavior as the Zn added through AM. Water-soluble Ni concentrations have significantly increased during both growing seasons in the digestate treatments. This may be due to the decrease in pH, as Ni mobility is enhanced under acidic conditions (Zhu et al., 2011). Kim et al. (2015) also confirmed that low pH can increase the release of naturally present Ni from soil.
The Zn concentration in the plant material collected during the 2015 season was significantly higher in the digestate treatments than in all other treatments (Figure 5). This reflects the higher availability of Zn as indicated by increased water-soluble concentrations. There were no significant differences in Zn concentration in the grain samples between the digestate and MF or AM treatments. Added digestate treatments had no significant influence on grain Ni concentration and the values were comparable to AM or MF treatments. Despite differences for Ni concentrations in soil-water extracts between treatments, no significant effect of the organic fertilizer treatments on plant Ni concentrations was found (Figure 5). The level of Ni concentrations in grains was comparable to that found in other studies with organic fertilizers conducted in Northern Europe (Hamner et al., 2013). Still, the long-term effect of the Zn or Ni accumulation in soil should not be disregarded. Currently, Zn is marked as one of the three metals mostly contributing to the global environmental pollution through the use of animal manure fertilizing practices (Leclerc and Laurent, 2017). The soil contamination factors for Zn and Ni were below or at the moderate levels for the 2015 season and at moderate contamination level for the 2016 season, which implies a moderate increase in the risk of trace element accumulation. The maximum water-extractable concentration of Ni from soil was around 50 μg L−1, which is below the 70 μg L−1 defined by the World Health Organization (WHO) drinkingwater regulation (World Health Organization, 2006).
Addition of digestates has not increased significantly the total Cu, Mn, and Mo concentration. The Cu, Mn, and Mo are important micronutrients needed for normal plant growth and development, and change in soil concentration may have a positive effect on plant growth. The recommended values of Cu total soil concentration for cereal growth is ranging between 20 and 40 mg kg−1, while toxic effects are dependent on the soil type and are usually seen between 60 and 125 mg kg−1 (Kabata-Pendias, 2011). Values measured in both growing seasons are approximately 10 times lower than the upper toxic limit for Cu in soil for all used treatments, which implies a lower concentration of Cu in soil then recommended. The reference values of Mn for soils used I agriculture in Norway is ~6 mg kg−1, where values measured in our studies was around 2 mg kg−1, showing that the addition of biogas digestates has no significant effect on the total Mn concentration in soil. For total Mo concentration in soil the reference value in soils is ~2 mg kg−1, which is not different than the values measured in our 2-year experiment (1.2–1.4 mg kg−1). Based on the total concentrations it is clear that the addition of both biogas digestates had no significant influence on the reference values of Mo in soil used for plant production (Kabata-Pendias, 2011; Brunetti et al., 2012).
Copper mobility, measured as water-extractable Cu, generally increased from June to August for all organic fertilizers. Copper mobility is mostly influenced by the changes in soil pH and DOC concentration due to the different treatments. Dissolved organic carbon, i.e., the soluble phase of organically bound carbon is known to affect metal mobility both in soil solution and thus in the soil (Pérez-Esteban et al., 2014). Addition of biogas digestate has increased the amounts of DOC in soil water extracts. In 2016, a significant increase in DOC was seen in the AM treatment in June. This follows the total amounts added with manure that year. In 2016, a higher volume of manure was added (233 L per plot) than in 2015 because of a lower N concentration. An increase in DOC concentration over the growing season with the use of organic fertilizers (composts) has also been confirmed in a recent study (Manninen et al., 2018). The rather low water-soluble concentration of Mo in our study may be explained by the fact that the strongest adsorption of Mo to the soil occurs at around pH 5 (Smith et al., 1997), which is not different from the pH values measured in the samples from our field experiment in August. Despite a pH change from 6.2 to 5.1 from June to August, no significant changes in water-extractable Mo concentrations were observed.
The total Cu concentrations in plants were significantly increased in the digestates treatments especially at the sampling in August 2015 (Figure 5) when compared to control plots, but grain Cu concentrations at harvest (year 2016) were not significantly increased. The contamination factor was below 1 for Cu, Mn, and Mo for all treatments (Figure 7), which implies a low risk of soil contamination.
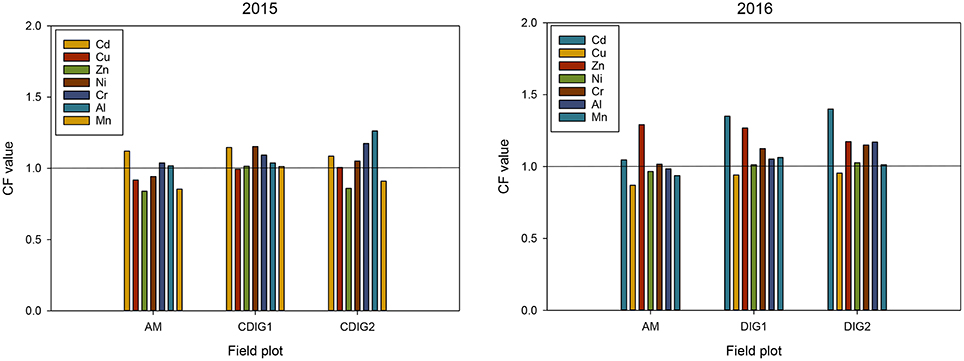
Figure 7. Calculated contamination factors for Cd, Cu, Zn, Ni, Cr, Al, and Mn for both growing seasons. The straight line in the figure presents the CF = 1.
Influence of Biogas Digestates Fertilization on Trace Metals Not Essential to Plants
For this group of tracemetals (Cr, Cd, and Al), the total soil concentration was significantly higher in the plots treated with biogas digestates (Figure A1) when compared to NN and MF plots. Use of both digestates significantly increased total Cr and Cd concentrations in soil (season 2016), while only DIG2 increased the total Al content of the soil. This is due to the high concentration of Cr and Al, but also Cd (2016) in the digestates. Cr concentration in the digestates was above 150 mg Cr/kg DM (Table 3), which was higher than the limits set by the Norwegian regulation on organic fertilizers used in agriculture (60 mg/kg dry fertilizer, class I). Slightly higher values were also seen for Cd (Table 3), while Al content is not regulated for organic fertilizers in Norway. The Cd content in organic wastes vs. that in mineral fertilizers is an important issue of discussion The maximum allowed total concentration of Cd in soils treated with organic waste such as sludge was 3 mg/kg (Brunetti et al., 2012), and this limit was not been exceeded in the two growing seasons. Toxic values for total Cr concentration in agricultural soils may vary between 150 and 400 mg/kg depending on the soil type (Kabata-Pendias and Pendias, 2001; Kabata-Pendias, 2011). In both seasons, total Cr concentration did not exceed 100 mg kg−1.
The water-soluble Cd concentrations were low in all treatments in the first season, as Cd additions with the organic fertilizers were low and little Cd was present in the soil initially. It is important to mention that the field experiment was started with the same treatments already in 2014, while the samples were taken and analyzed in the next 2 years (2015 and 2016). This may be important in evaluating potential Cd accumulation in the soils treated with digestates. Research studies with similar levels of Cd in organic fertilizers have also shown that the addition of organic fertilizers (manure) does not cause a significant change in the soil Cd content (Xu et al., 2015). In both growing seasons, Cd was strongly correlated to the pH then to DOC, which is expected and also confirmed in a recent study (van der Sloot et al., 2017), where lower pH was seen to increase the Cd soil water-extracted concentrations. (Van Zwieten et al., 2013) reported that application of organic fertilizers (poultry litter) with pH above 7 has resulted in soil pH decrease during the growing season (Van Zwieten et al., 2013), which has also seen for our experimental set up. There is a significant increase of Cr water-soluble concentrations during both growing seasons (Figure 3) for digestate treatments when compared to NN and MF. Still, measured concentrations were below the limit of 50 μg L−1 of total Cr, which is a recommended value for fresh waters (World Health Organization, 2006). The values measured in both seasons were comparable to those found in a similar field experiment reported by Wierzbowska et al. where values were also below 50 μg L−1 (Wierzbowska et al., 2016). In the pH range measured for both growing seasons, Cr(III) is expected to be the predominant form of Cr present in the soil solution (Bradl, 2004; Choppala et al., 2018). The same authors are also suggest that lower pH values (below 6.5) considered as a major factor that is increasing the level of Cr(III) sorption to soil particles. This can be used to explain the low water-extractable Cr concentrations found in our study (Figure 3).
Aluminum availability in soil and soil pore water is generally influenced by soil pH and DOC. In our study, both pH and DOC showed good correlations to water-extractable Al concentrations. The high total Al concentration in DIG2 was due to the sewage treatment process where Al salts were used for precipitation of P from sewage water prior to anaerobic digestion. Aluminum phosphates are poorly soluble, and this is clearly reflected in similar levels of water-extractable Al in DIG2 and even though no Al salts were added in the process for DIG1. In general, Al does not occur in toxic forms in the soil at pH values suitable for cereal production (Wang et al., 2006).
Low concentrations in both total and water-soluble forms have resulted in low Cd levels in the plant material (Figure 5), with a significant increase in Cd grain concentration only in the AM and DIG2 treatments. The amounts of Cd taken up by grains calculated per hectare were very low for all treatments (Figure 6). Cd originating from sewage sludge and similar sources is controlled more strictly than that from mineral fertilizers, although the latter can have a high Cd content (Pizzol et al., 2014). Digestate treatments had no significant effect on plant Cd or Cr concentrations and measured concentrations were comparable with AM or MF. The contamination factor for Cd, Cr, and Al though moderate, were highest from all the CFs measured in this study. The contamination factor for Al is of little relevance unless pH levels decrease below the adequate range (below pH 4) for cereal production, which could firstly affect the barely production.
In our field experiment with cereals, biogas digestates were applied at the same fertilization rates (100 kg /ha available N). At these rates, there was little difference in the effects of the applied fertilizers on soil or crop metal concentrations. Concentrations measured in the cereal grains were well below maximum allowed values found in human exposure studies with cereal crops (Huang et al., 2008). Still, research on accumulation rates over a longer period of application may give valuable insight into soil processes that the use of organic fertilizers can induce in soil. Our field experiment was only conducted for 3 years, and measurements only exist for the last 2 years. Longer time series will be necessary for assessing the plant uptake of trace metals after repeated additions of digestates.
Conclusions
In our study, we have reported for the first time results from field experiment conducted through two growing seasons to investigate the application of two different commercial biogas digestates based on food waste and food waste/sewage sludge mixture. The main hypothesis that the application of biogas digestates as organic fertilizers would increase the mobility and thus plant availability of trace metals in soil was not entirely confirmed. While total concentrations of Ni, Cd, Zn, and Cr were increased in soil upon application of digestates, their water-extracted concentrations were below the defined limits established by WHO. In addition, total Cu, Mn, and Mo concentrations were not significantly increased in the soil. Both pH and DOC were important factors in determining the mobility of the trace elements, in most cases increasing the water-soluble concentrations of trace elements from June to August. Plant concentrations did not indicate an increased uptake of trace metals into the cereal crops due to digestate application. Based on these results, the use of digestates can be compared to the use of animal manure or mineral fertilizers with respect to trace metal accumulation in soil and grain uptake. Still, a period of 2 years is too short to conclude on potential trace metal accumulation in soils due to the use of digestates as fertilizers.
Author Contributions
In regards to the work done regarding this manuscript, ID main tasks were sampling during the field experiment, sample analytical lab work, statistical considerations, manuscript draft, and final version preparation. SE-G main tasks were planning and organizing the field experiment, experiment yield measurements, help with the sampling and work on the draft and manuscript final version, while TS main tasks were planning the field experiment and work on the draft and final version of manuscript.
Funding
This research was partly funded by the Norwegian Research Council (Project no. 228747/E20, BiogasFuel).
Conflict of Interest Statement
The authors declare that the research was conducted in the absence of any commercial or financial relationships that could be construed as a potential conflict of interest.
References
Abubaker, J., Risberg, K., and Pell, M. (2012). Biogas residues as fertilisers – Effects on wheat growth and soil microbial activities. Appl. Energy 99, 126–134. doi: 10.1016/j.apenergy.2y.2012.04.050
Antoniadis, V., and Alloway, B. J. (2002). The role of dissolved organic carbon in the mobility of Cd, Ni and Zn in sewage sludge-amended soils. Environ. Pollut. 117, 515–521. doi: 10.1016/S0269-7491(01)00172-5
Antoniadis, V., Levizou, E., Shaheen, S. M., Ok, Y. S., Sebastian, A., Baum, C., et al. (2017a). Trace elements in the soil-plant interface: phytoavailability, translocation, and phytoremediation-A review. Earth Sci. Rev. 171, 621–645. doi: 10.1016/j.earscirev.2v.2017.06.005
Antoniadis, V., Shaheen, S. M., Boersch, J., Frohne, T., Du Laing, G., and Rinklebe, J. (2017b). Bioavailability and risk assessment of potentially toxic elements in garden edible vegetables and soils around a highly contaminated former mining area in Germany. J. Environ. Manage. 186, 192–200. doi: 10.1016/j.jenvman.2n.2016.04.036
Ashworth, D. J., and Alloway, B. J. (2004). Soil mobility of sewage sludge-derived dissolved organic matter, copper, nickel and zinc. Environ. Pollut. 127, 137–144. doi: 10.1016/S0269-7491(03)00237-9
Authority, M. N. F. S. (2006). Regulation on the Use of Organic Fertilizers/Forskrift om Gjødselvarer mv. av Organisk Opphav.
Borowski, S., Boniecki, P., Kubacki, P., and Czyzowska, A. (2018). Food waste co-digestion with slaughterhouse waste and sewage sludge: digestate conditioning and supernatant quality. Waste Manage. 74, 158–167. doi: 10.1016/j.wasman.2n.2017.12.010
Bradl, H. B. (2004). Adsorption of heavy metal ions on soils and soils constituents. J. Colloid Interface Sci. 277, 1–18. doi: 10.1016/j.jcis.2s.2004.04.005
Brunetti, G., Farrag, K., Soler-Rovira, P., Ferrara, M., Nigro, F., and Senesi, N. (2012). Heavy metals accumulation and distribution in durum wheat and barley grown in contaminated soils under Mediterranean field conditions. J. Plant Interact. 7, 160–174. doi: 10.1080/17429145.2011.603438
Cambier, P., Pot, V., Mercier, V., Michaud, A., Benoit, P., Revallier, A., et al. (2014). Impact of long-term organic residue recycling in agriculture on soil solution composition and trace metal leaching in soils. Sci. Total Environ. 499, 560–573. doi: 10.1016/j.scitotenv.2v.2014.06.105
Chen, Y. H., Wen, X. W., Wanga, B., and Nie, P. Y. (2017). Agricultural pollution and regulation: H: How to subsidize agriculture? J. Clean. Prod. 164, 258–264. doi: 10.1016/j.jclepro.2o.2017.06.216
Choppala, G., Kunhikrishnan, A., Seshadri, B., Park, J. H., Bush, R., and Bolan, N. (2018). Comparative sorption of chromium species as influenced by pH, surface charge and organic matter content in contaminated soils. J. Geochem. Explor. 184, 255–260. doi: 10.1016/j.gexplo.2o.2016.07.012
Fantozzi, F., and Buratti, C. (2009). Biogas production from different substrates in an experimental Continuously Stirred Tank Reactor anaerobic digester. Bioresour. Technol. 100, 5783–5789. doi: 10.1016/j.biortech.2h.2009.06.013
Hamner, K., Eriksson, J., and Kirchmann, H. (2013). Nickel in Swedish soils and cereal grain in relation to soil properties, fertilization and seed quality. Acta Agric. Scand. Sect. B Soil Plant Sci. 63, 712–722. doi: 10.1080/09064710.2013.861507
Huang, M., Zhou, S., Sun, B., and Zhao, Q. (2008). Heavy metals in wheat grain: A: Assessment of potential health risk for inhabitants in Kunshan, China. Sci. Total Environ. 405, 54–61. doi: 10.1016/j.scitotenv.2v.2008.07.004
IUSS working group WRB (2015). World Reference Base for Soil Resources 2015. World Soil Resources Report, 106.
Kabata-Pendias, A., and Pendias, H. (2001). Trace Elements in Soils and Plants, 3rd Edn. Boca Raton, FL: CRC Press.
Kim, R. Y., Yoon, J. K., Kim, T. S., Yang, J. E., Owens, G., and Kim, K. R. (2015). Bioavailability of heavy metals in soils: definitions and practical implementation-a critical review. Environ. Geochem. Health 37, 1041–1061. doi: 10.1007/s10653-015-9695-y
Kupper, T., Bürge, D., Bachmann, H. J., Gusewell, S., and Mayer, J. (2014). Heavy metals in source-separated compost and digestates. Waste Manage. 34, 867–874. doi: 10.1016/j.wasman.2n.2014.02.007
Leclerc, A., and Laurent, A. (2017). Framework for estimating toxic releases from the application of manure on agricultural soil: national release inventories for heavy metals in 2000-2014. Sci. Total Environ. 590, 452–460. doi: 10.1016/j.scitotenv.2v.2017.01.117
Manninen, N., Soinne, H., Lemola, R., Hoikkala, L., and Turtola, E. (2018). Effects of agricultural land use on dissolved organic carbon and nitrogen in surface runoff and subsurface drainage. Sci. Total Environ. 618, 1519–1528. doi: 10.1016/j.scitotenv.2v.2017.09.319
Maragkaki, A. E., Fountoulakis, M., Kyriakou, A., Lasaridi, K., and Manios, T. (2018). Boosting biogas production from sewage sludge by adding small amount of agro-industrial by-products and food waste residues. Waste Manage. 71, 605–611. doi: 10.1016/j.wasman.2n.2017.04.024
Möller, K., and Müller, T. (2012). Effects of anaerobic digestion on digestate nutrient availability and crop growth: a review. Eng. Life Sci. 12, 242–257. doi: 10.1002/elsc.2c.201100085
Nelson, D. W., and Sommers, L. E. (1982). Methods of Soil Analysis. Part 2 Chemical Microbiological Properties. Madison, WI: American Society of Agronomy, Inc.; Soil Science Society of America, Inc. 9, 539–579.
Ni, P., Lyu, T., Sun, H., Dong, R. J., and Wu, S. B. (2017). Liquid digestate recycled utilization in anaerobic digestion of pig manure: effect on methane production, system stability and heavy metal mobilization. Energy 141, 1695–1704. doi: 10.1016/j.energy.2y.2017.11.107
Nicholson, F. A., Chambers, B. J., Williams, J. R., and Unwin, R. J. (1999). Heavy metal contents of livestock feeds and animal manures in England and Wales. Bioresour. Technol. 70, 23–31.
Nkoa, R. (2014). Agricultural benefits and environmental risks of soil fertilization with anaerobic digestates: a review. Agron. Sus. Dev. 34, 473–492. doi: 10.1007/s13593-013-0196-z
Parraga-Aguado, I., Alvarez-Rogel, J., Gonzalez-Alcaraz, M. N., and Conesa, H. M. (2017). Metal mobility assessment for the application of an urban organic waste amendment in two degraded semiarid soils. J. Geochem. Explor. 173, 92–98. doi: 10.1016/j.gexplo.2o.2016.11.022
Pérez-Esteban, J., Escolástico, C., Masaguer, A., Vargas, C., and Moliner, A. (2014). Soluble organic carbon and pH of organic amendments affect metal mobility and chemical speciation in mine soils. Chemosphere 103, 164–171. doi: 10.1016/j.chemosphere.2e.2013.11.055
Pizzol, M., Smart, J. C. R., and Thomsen, M. (2014). External costs of cadmium emissions to soil: a drawback of phosphorus fertilizers. J. Clean. Prod. 84, 475–483. doi: 10.1016/j.jclepro.2o.2013.12.080
Shaheen, S. M., Shams, M. S., Khalifa, M. R., El-Dali, M. A., and Rinklebe, J. (2017). Various soil amendments and environmental wastes affect the (im) mobilization and phytoavailability of potentially toxic elements in a sewage effluent irrigated sandy soil. Ecotoxicol. Environ. Saf. 142, 375–387. doi: 10.1016/j.ecoenv.2v.2017.04.026
Smith, K. S., Balistrier, L. S., Smit, S. M., and Severso, R. C. (1997). “Distribution and mobility of molybdenum in the terrestrial environment,” in Molybdenum in Agriculture, ed U. C. Gupta (CambridgeCambridge: Cambridge University Press), 23–46.
Sogn, T. A., Dragicevic, I., Linjordet, R., Krogstad, T., Eijsink, V. G. H., and Eich-Greatorex, S. (2018). Recycling of biogas digestates in plant production: NPK fertilizer value and risk of leaching. Intl. J. Recycl. Organ. Waste Agric. 7, 49–58. doi: 10.1007/s40093-017-0188-0
Sonneveld, C. (1990). Estimating quantities of water-soluble nutrients in soils using a specific 1:2 by volume extract. Commun. Soil Sci. Plant Anal. 21, 1257–1265.
Stoknes, K., Scholwin, F., Krzesinski, W., Wojciechowska, E., and Jasinska, A. (2016). Efficiency of a novel “Food to waste to food” system including anaerobic digestion of food waste and cultivation of vegetables on digestate in a bubble-insulated greenhouse. Waste Manage. 56, 466–476. doi: 10.1016/j.wasman.2n.2016.06.027
Törnwall, E., Pettersson, H., Thorin, E., and Schwede, S. (2017). Post-treatment of biogas digestate – An evaluation of ammonium recovery, energy use and sanitation. Energy Procedia 142, 957–963. doi: 10.1016/j.egypro.2o.2017.12.153
van der Paauw, F. (1971). An effective water extraction method for the determination of plant-available soil phosphorus. Plant Soil 34, 467–481.
van der Sloot, H. A., Kosson, D. S., and van Zorneren, A. (2017). Leaching, geochemical modelling and field verification of a municipal solid waste and a predominantly non-degradable waste landfill. Waste Manage. 63, 74–95. doi: 10.1016/j.wasman.2n.2016.07.032
Van Zwieten, L., Kimber, S. W., Morris, S. G., Singh, B. P., Grace, P. R., Scheer, C., et al. (2013). Pyrolysing poultry litter reduces N2O2O and CO2 fluxes. Sci. Total Environ. 465, 279–287. doi: 10.1016/j.scitotenv.2v.2013.02.054
Wang, J. P., Raman, H., Zhang, G. P., Mendham, N., and Zhou, M. X. (2006). Aluminium tolerance in barley (Hordeum vulgare L.): physiological mechanisms, genetics and screening methods. J. Zhejiang Univ. Sci. B 7, 769–787. doi: 10.1631/jzus.2s.2006.B0769
Welikala, D., Hucker, C., Hartland, A., Robinson, B. H., and Lehto, N. J. (2018). Trace metal mobilization by organic soil amendments: insights gained from analyses of solid and solution phase complexation of cadmium, nickel and zinc. Chemosphere 199, 684–693. doi: 10.1016/j.chemosphere.2e.2018.02.069
Wierzbowska, J., Sienkiewicz, S., Krzebietke, S., and Bowszys, T. (2016). Heavy metals in water percolating through soil fertilized with biodegradable waste materials. Water Air Soil Pollut. 227:456. doi: 10.1007/s11270-016-3147-x
Xu, Y. G., Yu, W. T., Ma, Q., and Zhou, H. (2015). Potential risk of cadmium in a soil-plant system as a result of long-term (10 years) pig manure application. Plant Soil Environ. 61, 352–357. doi: 10.17221/100/2015-PSE
Yang, X., Li, Q., Tang, Z., Zhang, W., Yu, G., Shen, Q., et al. (2017). Heavy metal concentrations and arsenic speciation in animal manure composts in China. Waste Manage. 64, 333–339. doi: 10.1016/j.wasman.2n.2017.03.015
Yuksel, O. (2015). Influence of municipal solid waste compost application on heavy metal content in soil. Environ. Monit. Assess. 187:313. doi: 10.1007/s10661-015-4562-y
Yun, L. H. L., Guoxue, L., Wenhai, L., and Ying, S. (2018). Manure digestate storage under different conditions: c: Chemical characteristics and contaminant residuals. Sci. Total Environ. 639, 19–25. doi: 10.1016/j.scitotenv.2v.2018.05.128
Zhang, X., Wei, S., Sun, Q., Wadood, S. A., and Guo, B. (2018). Source identification and spatial distribution of arsenic and heavy metals in agricultural soil around Hunan industrial estate by positive matrix factorization model, principle components analysis and geo statistical analysis. Ecotoxicol. Environ. Saf. 159, 354–362. doi: 10.1016/j.ecoenv.2v.2018.04.072
Zhu, R., Wu, M., and Yang, J. (2011). Mobilities and leachabilities of heavy metals in sludge with humus soil. J. Environ. Sci. 23, 247–254. doi: 10.1016/S1001-0742(10)60399-3
Appendix
Keywords: organic waste, fertilizer, cadmium, chromium, nickel, zinc
Citation: Dragicevic I, Sogn TA and Eich-Greatorex S (2018) Recycling of Biogas Digestates in Crop Production—Soil and Plant Trace Metal Content and Variability. Front. Sustain. Food Syst. 2:45. doi: 10.3389/fsufs.2018.00045
Received: 16 February 2018; Accepted: 11 July 2018;
Published: 07 August 2018.
Edited by:
Tom Misselbrook, Rothamsted Research (BBSRC), United KingdomReviewed by:
Fiona Nicholson, Agricultural Development Advisory Service, United KingdomMarianne Thomsen, Aarhus University, Denmark
Raul Zornoza, Universidad Politécnica de Cartagena, Spain
Copyright © 2018 Dragicevic, Sogn and Eich-Greatorex. This is an open-access article distributed under the terms of the Creative Commons Attribution License (CC BY). The use, distribution or reproduction in other forums is permitted, provided the original author(s) and the copyright owner(s) are credited and that the original publication in this journal is cited, in accordance with accepted academic practice. No use, distribution or reproduction is permitted which does not comply with these terms.
*Correspondence: Ivan Dragicevic, ivan.dragicevic@nmbu.no