Erratum: Nitrogen Supply Affects Yield and Grain Filling of Maize by Regulating Starch Metabolizing Enzyme Activities and Endogenous Hormone Contents
- 1State Key Laboratory of Aridland Crop Science, Gansu Agricultural University, Lanzhou, China
- 2College of Agronomy, Gansu Agricultural University, Lanzhou, China
- 3Institute of Crop Science, Xinjiang Academy of Agri-Reclamation Sciences, Shihezi, China
- 4Institute of Molecular Biology and Biotechnology, The University of Lahore, Lahore, Pakistan
This study aimed to examine the effect of nitrogen (N) application rate and time on yield, grain filling, starch metabolizing enzymes, and hormones of maize based on a long-term field experiment initiated in 2012. The total N fertilizer dose [(0 (N0), 100 (N1), 200 (N2), and 300 (N3) kg N ha–1] was split into two (T1, one-third at sowing and two-thirds at the six-leaf stage) or three (T2, one-third each at sowing, six-leaf, and eleven-leaf stage) times application. The results showed that the highest yield was obtained under N3T2, N2T1, and N3T2 in 2018, 2019, and 2020, which was 222.49, 185.31, and 194.00% than that of N0 in each year, respectively. N2 and N3 significantly increased the yield through enhancing ears ha–1, grains per plant, and 100-grain weight; however, N2 and N3 did not show a significant difference in yield and above-yield components. In addition, N application time did not significantly change yield under the same N rate. N0 limited the activities of starch metabolizing enzymes, resulting in insufficient accumulation of sucrose and starch. The contents of indole-3-acetic acid, cytokinin, abscisic acid, and gibberellin were decreased under N0 during grain filling. The average grain-filling rate and maximum grain-filling rate (Gmax) and grain weight increment achieving Gmax increased under N2 and N3, and the grain-filling parameters were positively correlated with 100-grain weight. In conclusion, 200 kg N ha–1 with one-third application at sowing and two-thirds application at the six-leaf stage is a suitable N supply way to improve starch metabolizing enzymes, regulate hormone content, and enhance grain-filling rates, and thus increasing the maize yield in the semiarid Loess Plateau of China.
Introduction
Nitrogen (N) is a major nutrient for maize (Zea mays L.) production and also takes part in the plant physiological process as a significant component, such as enzymes, hormones, and amino acids (Thind et al., 2011; Ciampitti and Vyn, 2012). Therefore, to get a higher yield, high N application is the most common practice for farmers in the semiarid Loess Plateau of China. However, excessive N leads to abundant losses of N fertilizer as well as restraining antioxidant enzymes of cereal leaf and decreasing grain yields (Kong et al., 2017). In addition, the suitable time of N applied could increase maize yield, but the variation of the yield is tightly associated with the N rate and external environment (Ning et al., 2017; Banger et al., 2018). These studies suggest that excessive high N rate and inappropriate N application time could decrease crop yield. However, the influence of N rate and time on maize yield is vague in the semiarid Loess Plateau of China. Grain weight, ears ha–1, and grains per ear are the decisive factor of yield formation (Lu et al., 2017). Previous studies showed that N fertilization significantly increased grain yield by enhancing the grain weight, ears ha–1, and grains per ear (Srivastava et al., 2018; Wei et al., 2019). The appropriate N enhances grain weight as a result of increasing effective grain-filling duration and rate (Wei et al., 2019). However, the heavy use of N results in slow grain filling and unfavorably delayed senescence which prolongs grain-filling duration, leading to low grain weight (Yang and Zhang, 2006). So, it is vital to clarify the physiological mechanisms of grain filling for the increase of maize yield under different N application rates and times in the semiarid Loess Plateau of China.
The grain-filling process could be divided into gradual increase period (GIP), rapid increase period (RIP), and slight increase period (SIP) through the generative parameters (Wei et al., 2019). Cao et al. (2015) found that appropriate N application increased active grain-filling period (AGP) and maximum grain-filling rate (Gmax), which parameters calculated by logistic equation, and the variation of yield may due to AGP and Gmax, which were positively correlated with yield. Wang et al. (2018) found that Gmax and AGP increased significantly under controlled-release urea, so N application time also affects grain yield by affecting grain filling. For some large ear crops, insufficient grain filling of grains is one of the main factors limiting yield potential (Yang and Zhang, 2010). Thus, a key approach to increasing grain weight is to maintain a good grain-filling process. However, there has been little agreement on what N rate and time affect grain filling in maize in the semiarid Loess Plateau of China.
As momentous substances regulating grain filling, starch-metabolizing enzymes and endogenous hormones contribute significantly to grain development (Fahy et al., 2018; Wang and Zhang, 2020). The concerted activities of enzymes such as ADP-glucose pyrophosphorylase (AGPase) and starch synthases (SSs) lead to the synthesis of the starch (Thitisaksakul et al., 2012). Starch is the principal component of maize grain that determines grain weight (Cazetta et al., 1999), and N-limitation decreases grain starch accumulation by affecting carbohydrate biosynthetic enzyme activity (Seebauer et al., 2010). Furthermore, sucrose synthase (SuSy) and sucrose phosphate synthase (SPS) can regulate starch accumulation by affecting sucrose synthesis (Ning et al., 2018). Studies reported that AGPase and SSs activities increased with the increase of N application, which indicated these two enzymes might play a more critical role in the biosynthesis and regulation of the accumulation rate of corn starch (Liu et al., 2021). In addition, AGPase and SSs activities in grains could be improved by proper N application time, and different enzymes act differently at different grain-filling periods (Li et al., 2005). The endogenous hormones in grains such as indole-3-acetic acid (IAA), cytokinin (CTK), abscisic acid (ABA), and gibberellin (GA) are involved in the regulation of grain-filling rate and duration (Liu et al., 2013; Xu et al., 2013). The reciprocal regulation between hormones and enzymes and the balance of hormones play a crucial role in the final grain weight (Fu et al., 2013). ABA content regulates the signaling pathway of sugar synthesis, and several studies have documented an increase in ABA content by N application compared to non-N-fertilized (Rook et al., 2001; Qin et al., 2013). The proper N application time is conducive to increasing CTK content in grains, promoting the accumulation of dry matter into the grains, and increasing grain weight (Luo et al., 2018). Jiang et al. (2009) reported that high GA content contributes to maize grain-filling rate in the grain-filling stage.
It has been proved that starch-metabolizing enzymes and endogenous hormones regulate starch accumulation and grain filling. However, the effects of the N rate and time on yield and grain filling via regulating enzymes and hormones in grain filling were unclear, especially in the Loess Plateau under field conditions. Therefore, we analyze the variation in maize yield, yield components, grain-filling parameters, starch synthase activities, and endogenous hormone content under different N rates and times in this study. The objective of this study was to: (i) identify the contribution of grain filling and yield components to yield under different N applications, (ii) investigate the effect of N fertilizer on starch accumulation through regulating starch-metabolizing activities, and (iii) confirm the relationship between hormone contents and grain-filling characteristics, which determined by logistic and standardized analytical modeling. The research may provide a theoretical basis for optimizing N application for maize in the semiarid Loess Plateau of China.
Materials and Methods
Site Description
The field study was conducted at the Dingxi Experimental Station (35°28′N, 104°44′E) of the Gansu Agricultural University, Gansu Province, Northwest China. The area is a semiarid Loess Plateau with an average altitude of 2,000 m. The experimental data for 2018, 2019, and 2020 have been presented in this study. The aeolian soil of the site is prone to erosion and classified as a Calcaric Cambisol (Food and Agriculture Organization [FAO], 1990). The soil at 0–20 cm depth has a sandy loam texture with 50% sand, moderate to low fertility, slightly alkaline pH (8.3), soil organic carbon of 7.65 g kg–1, available potassium of 220 mg kg–1, and Olsen’s phosphorus of 13 mg kg–1, and the soil mineral N status before the sowing as shown in Table 1. Annual frost-free period of 140 days, and annual radiation is 5,930 MJ m–2. The average annual growing season precipitation, mean temperature, cumulative radiation, and evapotranspiration at the site during 2018, 2019, and 2020 are shown in Figure 1. In the crop-growing season, precipitation (May–September) was 377.7, 405.3, and 481.0 mm in 2018, 2019, and 2020, respectively. Total sunny days in the main grain-filling period (August and September) were 13, 25, and 21 days in 2018, 2019, and 2020, respectively, according to meet record 2018 was a year with the lowest precipitation and the least sunny days during grain filling.
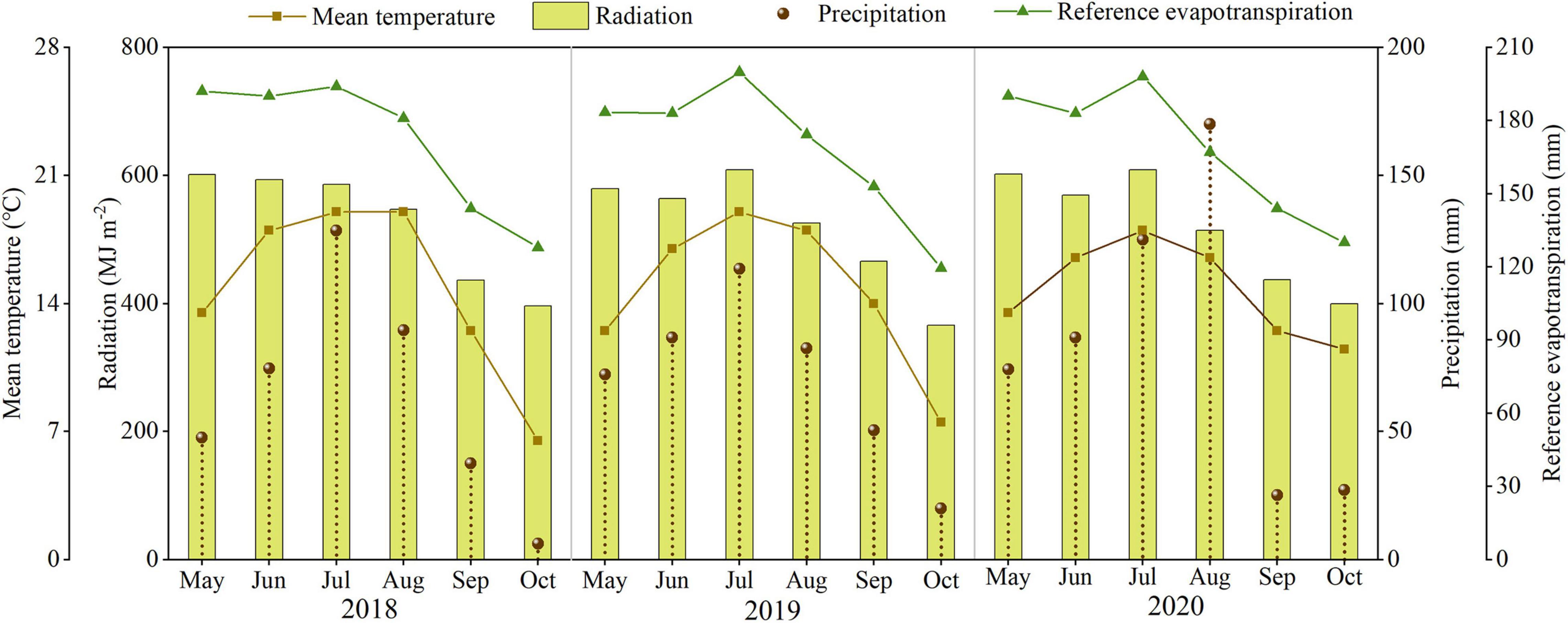
Figure 1. The precipitation, mean temperature, cumulative radiation, and evapotranspiration during the maize growth period in 2018, 2019, and 2020.
Experiment Design
The four N fertilization levels were divided into two and three application times in a completely randomized block design and three replications per treatment. The four N fertilization levels were: N0 (no N fertilizer), N1 (100 kg N ha–1), N2 (200 kg N ha–1), and N3 (300 kg N ha–1). For the first N application time (T1), one-third of the total N rate was applied at sowing while the remaining two-thirds were at the six-leaf collar stage (V6). In the second N application time (T2), one-third of the total N rate was applied at sowing and V6 and eleven-leaf collar (V11) stages. N fertilizer was applied using urea (46% N) and phosphorus (150 kg ha–1) as P2O5. No potassium fertilizer was applied as available potassium (220 mg kg–1) in the soil is enough for maize production. Completely film mulched alternate narrow and wide ridges with furrow planting, which is a new planting technology, can increase soil temperature and reduce direct evaporative losses. The area of each plot was 18.7 m2 (4.4 m × 4.25 m). The alternate wide (0.7 m) and narrow (0.4 m) ridges were mulched with transparent plastic films (1.1 m wide) before sowing maize. The maize, cv. Xianyu 335, which is the most popular variety in this area, were sown in furrows at a target final stand of 52,500 plants ha–1 in late April, which is 98 plants per plot. Maize plants were harvested in early October of each year.
Measurements and Calculations for Grain Filling, Enzymes, and Endogenous Hormones Measurements
In each plot, 24 plants were labeled before pollination. Three ears were selected from 24 labeled plants each week after pollination, that is, at 7, 14, 21, 28, 35, 42, 49, and 56 days after pollination (DAP), respectively. A total of 200 grains were then randomly sampled from the middle part of the three ears (total grains in each ear is approximately 350 grains), counting from the bottom to the top from the tenth to the twentieth row (Fang et al., 2020), and divided into two portions. The first portion of grains (7, 14, 21, 28, and 35 DAP) was frozen in liquid N at once, carried back to the laboratory, and stored in −80°C fridge for starch synthetic enzymes and endogenous hormones measurements. The second portion of grains (7, 14, 21, 28, 35, 42, 49, and 56 DAP) was dried in an oven for 30 min at 105°C and then at 80°C to constant weight, the grains were then weighed, dried grains (7, 14, 21, 28 and 35 DAP) were also sampled to determine the sucrose, starch, and protein content.
Grain-Filling Traits
The grain-filling process was modeled using the following logistic equation (Wei et al., 2019):
where W is the grain weight, A, B, and C are the equation coefficients, t is the days after pollination.
The grain–filling parameters were calculated as Wei et al. (2019):
The duration, weight, and average grain-filling rate of GIP, RIP, and SIP, we set as T1, W1, G1, T2, W2, G2, T3, W3, and G3, the parameters were calculated as Wei et al. (2019).
Where W is the 100-grain weight at 56 DAP.
Starch and Sugar Analysis
The grain starch content (%) was determined using the anthrone-sulfuric acid method (Zou, 1995). First, 0.2 g of grain powder was weighed, 7 ml of 80% ethanol was added into 80°C water bath for 30 min, centrifuged at 4,000 rpm for 5 min, the precipitate is retained, and the extraction is performed three times. Then, 2 ml of deionized water was added to the precipitate and the ethanol was evaporated at 80°C and gelatinized in a boiling water bath for 15 min. After cooling, 2 ml of 9.2 M HClO4 was added and hydrolyzed for 15 min, and then 4 ml of deionized water was added and centrifuged for 10 min. Subsequently, the supernatant was poured into a volumetric flask. Then, 2 ml of 4.6 M HClO4 was added to the precipitate and 6 ml of deionized water was added after 15 min of extraction, centrifuged for 10 min, and diluted to 50 ml with deionized water. Furthermore, 0.5 ml of 2% anthrone and 5 ml of concentrated sulfuric acid were added to the supernatant. The starch content was calculated according to the absorbance measured at 620 nm.
The grain sucrose content (mg g–1) was determined using the resorcinol method (Zhang and Qu, 2003). First, 0.2 g sample was weighed, 4 ml of 80% ethanol extract was added into 80°C water bath for 40 min and centrifuged, the supernatant was retained, the precipitate was extracted two times with 2 ml of 80% ethanol and diluted to 10 ml. Then, 0.4 ml of the supernatant was taken, added to 200 μl of 2 M NaOH, mixed thoroughly, and subsequently boiled in a water bath for 10 min. Then, 2.8 ml of 30% HCl was added and mixed well and kept at 80°C for 10 min. At last, 1 ml 1% resorcinol was added to the mixture, mixed thoroughly, and kept at 80°C for 10 min again. After cooling, the absorbance at 480 nm was measured to calculate sucrose content.
Sucrose Synthase and Sucrose Phosphate Synthase
The grains samples (0.5 g) were homogenized with the extraction buffer containing 5 ml of 50 mM HEPES-NaOH (pH 7.5) in ice-chilled (0°C∼4°C) mortar. The mixture was centrifuged at 10,000 × g for 10 min, and the supernatant was used for enzyme determination (Yang et al., 2018).
Sucrose synthase (SuSy) assay was performed by using the proposed method with modifications (Yang et al., 2018). About 50 μl HEPES-NaOH (pH 7.5), 20 μl 100 mM UDPG, 20 μl 100 mM fructose, and 20 μl 50 mM MgCl2 were added to 50 μl enzyme solution, and then the reaction was performed at a temperature of 30°C for 30 min. Later, 0.2 ml of 2 M NaOH was added, the reaction was terminated, and then boiling water was used to heat the solution for 10 min. After that, 2 ml 30% HCl was poured into it and remained at a temperature of 80°C for 10 min. Then, 1 ml 1% resorcinol was poured into it and remained for about 10 min. Finally, it was cooled down, and 3.64 ml of deionized water was added. Later, at 480 nm, optical density was obtained, and the amount of sucrose produced was determined by the unit activity. It could be concluded that the SPS determination process was equal to SuSy, except the fructose has been replaced by fructose-6-phosphate (Yang et al., 2018).
Assay of Starch Synthesis Enzyme
Starch synthases (SSS) and AGPase were determined using the ELISA kit that was supplied by Jiangsu Meibiao Biotechnology Co., Ltd., (Yancheng, China).
Endogenous Hormone
According to previous studies (Yang et al., 2001; Liang et al., 2017), the endogenous ABA, IAA, GA, and CTK were determined by enzyme-linked immunosorbent assay (ELISA), the ELISA kits were supplied by Jiangsu Meibiao Biotechnology Co., Ltd., (Yancheng, China).
Yield and Yield Components
At the physiological maturity stage, 10 plants were randomly selected to determine ears ha–1, grains per plant, and 100-grain weight. As for grain yield, the sampling area was excluded, then yield per hectare was calculated based on the actual harvest yield from each plot.
Statistical Analysis
We used ANOVA to analyze data with SPSS 25.0 (SPSS Institute Incorporation, Armonk, NY, United States). Treatments were compared using Duncan’s test at the 0.05 probability level. Pearson correlation analysis and canonical correlation analysis were also performed with the help of SPSS 25.0. Figures were made with Origin 2019.
Results
Grain Yield and Yield Components
N rate significantly altered the yield and yield components; however, N application time and N rate and time interaction did not significantly affect the yield and yield components (Table 2). There was a huge difference between years, the annual average yield among all the treatments was 6,578, 9,188, and 9,265 kg ha–1 in 2018, 2019, and 2020, respectively. The highest yield was obtained under N3T2, N2T1, and N3T2 in 2018, 2019, and 2020, which was 222.49, 185.31, and 194.00% than that of N0 in each year, respectively. Furthermore, the difference in yield is not significant under N2 and N3 (except for N2T2 in 2018). Under the N2 and N3, ears ha–1, grains per plant, and 100-grain weight were enhanced compared to N0, but no significant differences were shown between N2 and N3. Under N0 treatment, there were 52,500 ears ha–1 (except 53,375 ears ha–1 in 2018), however, it cumulated up to 87,500–99,750 ears ha–1 under N2 and N3. Simultaneously, compared with N0, grains per plant of N2 and N3 increased by 240.21 and 280.47% in 2019, and 163.63 and 176.03%. No significant difference was found between N1, N2, and N3 in the 100-grain weight, N2 and N3 significantly increased by 19.19% and 18.88% in 2019, and 19.41% and 28.87%. In addition, the yield of maize was positively correlated with 100-grain weight, grains per plant, and ears ha–1 (Figure 2). The most relevant is the grains per plant, followed by ears ha–1, and 100-grain weight.
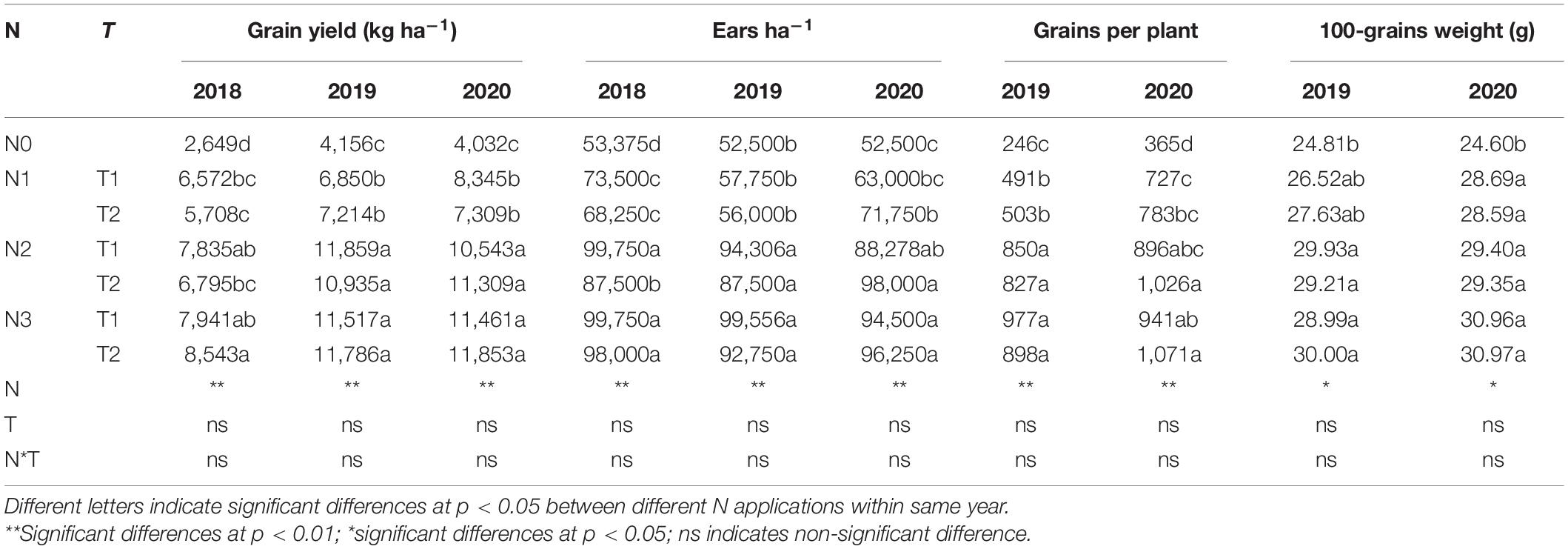
Table 2. Effects of different N rates and application times on grain yield and yield components of maize.
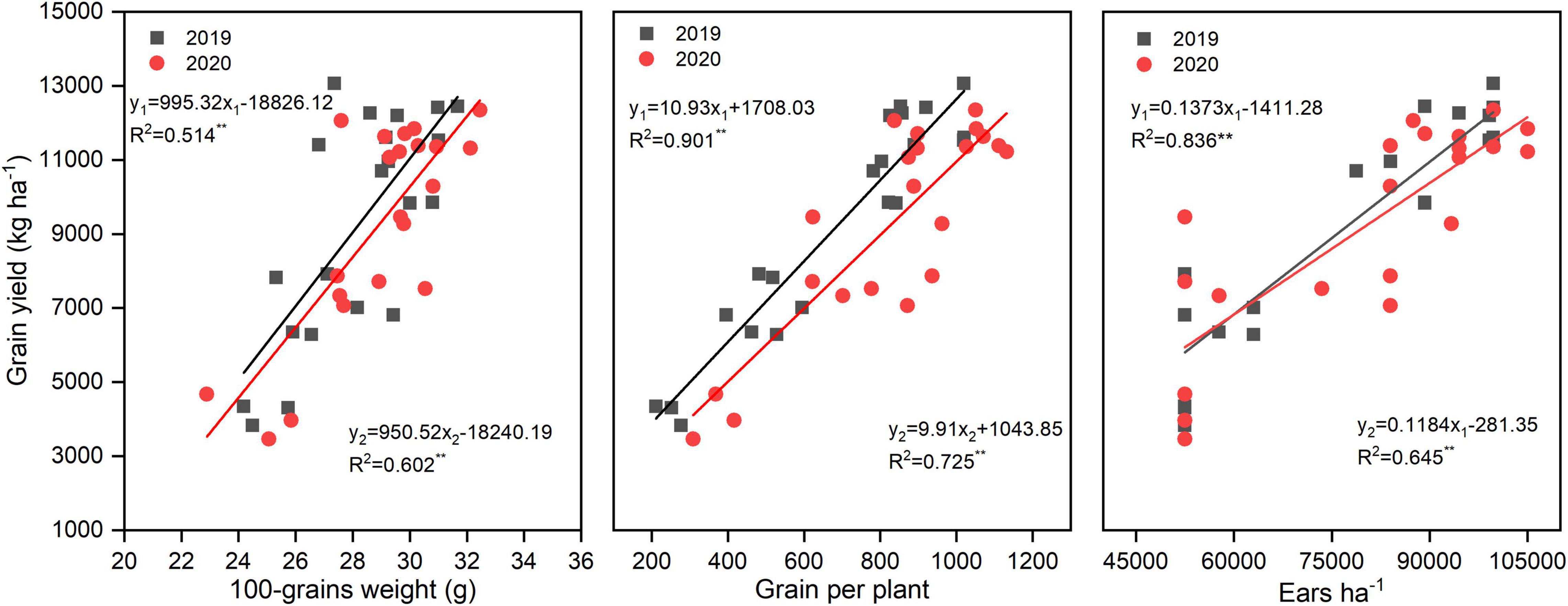
Figure 2. Correlations between yield, 100-grains weight, grains per plant, and ears ha–1. y1 and y2 represent and 2020 simulation equation, respectively.
Sucrose and Starch Contents
Within 7–35 DAP, the sucrose content in grains was affected by the N rate (Figures 3A,B). The highest sucrose content was recorded under N2 and N3 treatments at the 14 DAP except for N2T1 in 2020. Under N0 and N1, the maximum sucrose content appeared at 21 DAP and then decreased with the growth stage. The sucrose content for N2 and N3 was dramatically higher than that for N0 at 14 DAP, and no significance was observed between N2 and N3 for sucrose content. Under the same N rate, N application time did not affect sucrose content significantly.
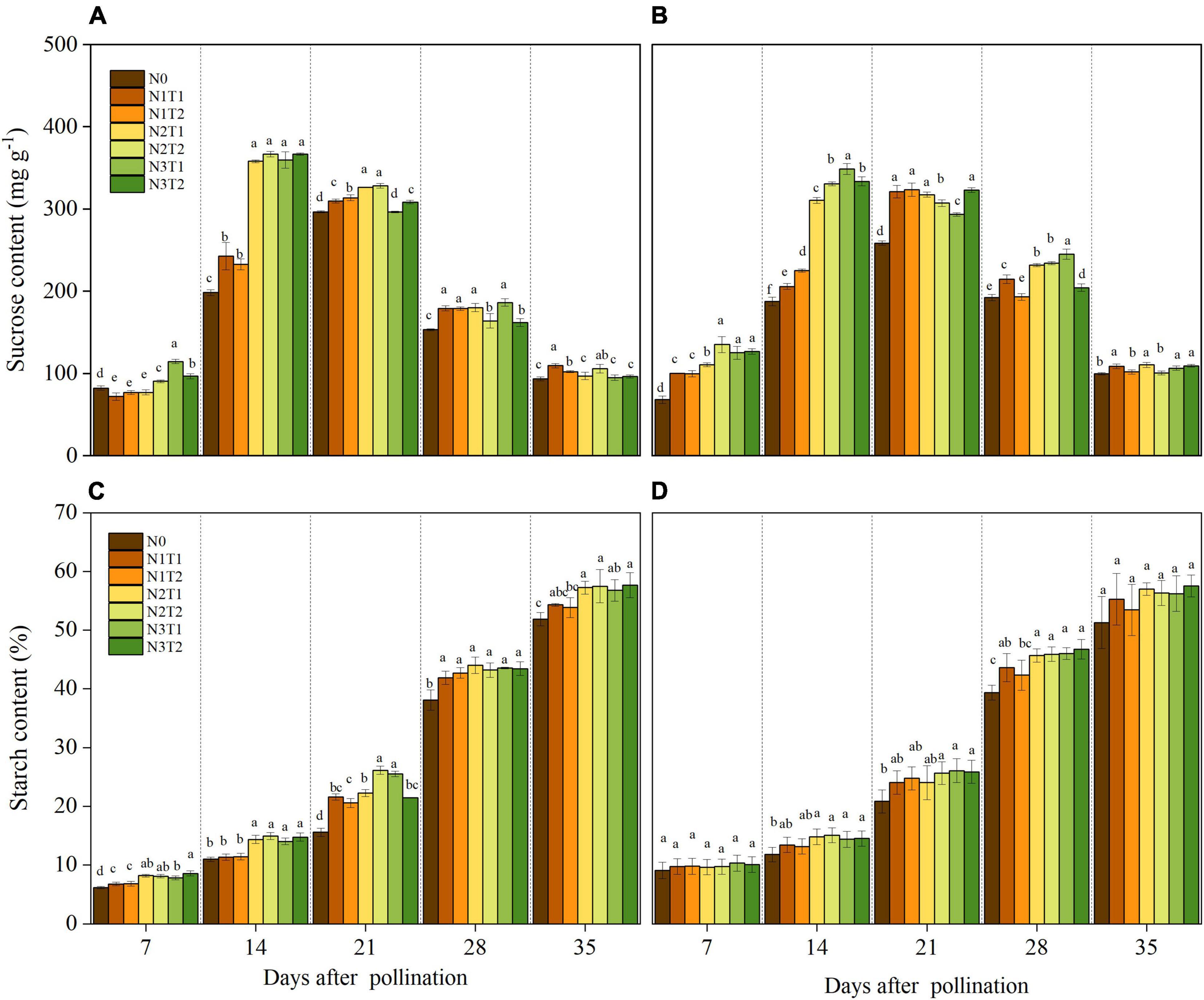
Figure 3. Effects of N rate and time on the content of sucrose and starch in 2019 (A,C) and 2020 (B,D). N rate, N0, no N fertilizer; N1, 100 kg N ha–1; N2, 200 kg N ha–1; N3, 300 kg N ha–1. N application time, T1, one-third of the full N rate corresponding to the treatment at sowing and the remaining two-thirds at V6; T2, one-third of the full N rate corresponding to the treatment at sowing, V6, and V11. Different letters indicate significant differences at p < 0.05.
The starch content increased consistently with time after pollination (Figures 3C,D). After 21 DAP, the starch content under N2 and N3 was increased as compared to N0. There was no significant difference in starch content between T1 and T2 under the same N rate. On average, compared with N0, N2, and N3 increased by 10.62, 10.35, and 10.44, and 10.86% at 35 DAP in 2019 and 2020, respectively.
Sucrose Synthase and Sucrose Phosphate Synthase Activities
There was no significant difference in N2 and N3 of SuSy activities, and the variation of SuSy activities was not significant in T1 and T2 under the same N rate (Figures 4A,B). During grain filling, N2 and N3 increased SuSy activity significantly, and the SuSy activities reached a peak at 14 DAP. On average, N2 increased the SuSy activity at 14 DAP by 48.6% and 42.5% compared with N0 in 2019 and 2020, respectively.
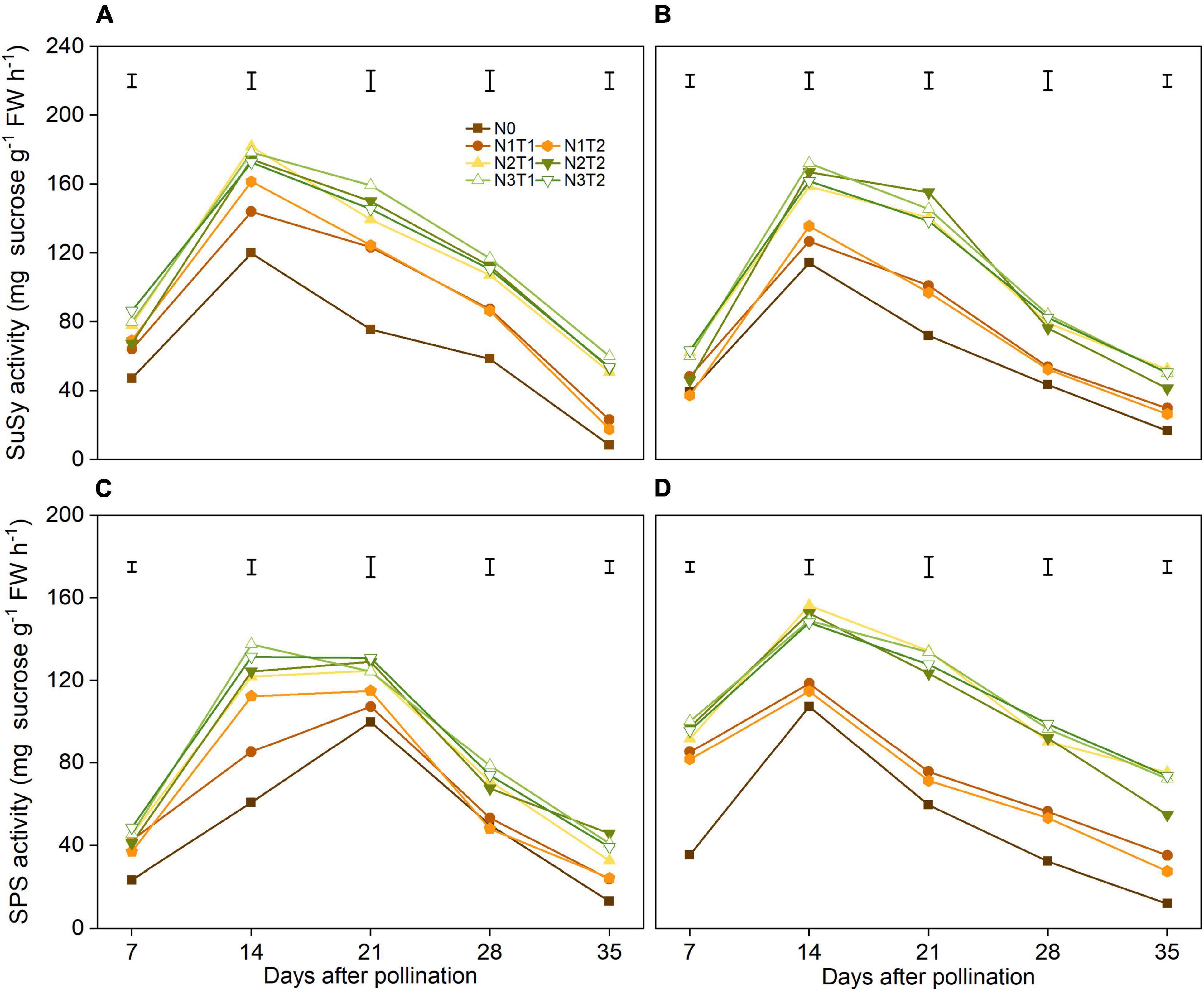
Figure 4. Effects of N rate and time on the activities of SuSy and SPS in 2019 (A,C) and 2020 (B,D). SuSy, sucrose synthase; SPS, sucrose phosphate synthase. The vertical bars represent the LSD (n = 3).
Nitrogen affected the SPS activity, however, the peak of SPS was different in 2 years (Figures 4C,D). The N2 and N3 had significantly increased the SPS activities from 7 DAP and 35 DAP compared with N0. In general, N application time did not alter SPS activities significantly under the same N rate.
ADP-Glucose Pyrophosphorylase and Starch Synthases Activities
The activities of AGPase and SSS showed variation with the N supply and peaked at 28 DAP (Figure 5). In the grain-filling period, AGPase activity first increased and then decreased, and N2 and N3 increased AGPase activity compared to N0. No significant differences were obtained between N2 and N3 at 28 DAP, and N2 increased the AGPase by 8.37 and 6.39% in 2019 and 2020 compared with N0, respectively. The AGPase activities for different N application times were not significantly different under different N rates. Similarly, N application time also did not alter SSS activities significantly. Within 7–35 DAP, N2 and N3 increased SSS activities compared to N0, and N2 and N3 did not show a significant difference. At the 28 DAP, N2 significantly increased the SSS activities by 31 and 42.8% compared with N0 in 2019 and 2020.
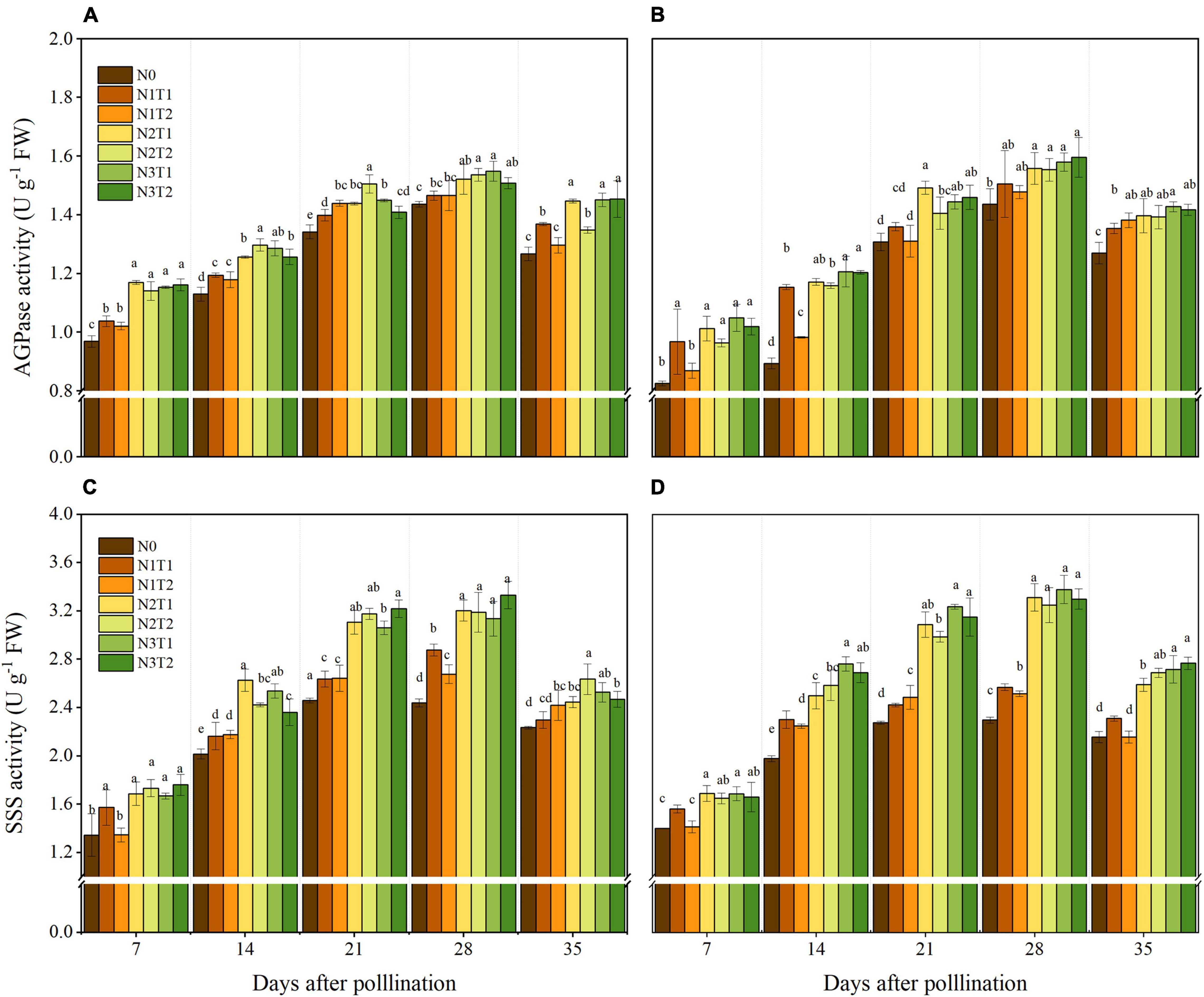
Figure 5. Effects of N rate and time on the activities of AGPase and SSS in 2019 (A,C) and 2020 (B,D). AGPase, ADP-glucose pyrophosphorylase, starch synthases. Different letters indicate significant differences at p < 0.05.
Grain-Filling Characteristic Parameters
Progress in grain filling was well-simulated by the logistic model under different N rates and time applications (R2 ≥ 0.988) (Table 3). The Gmax, Wmax, Gmax, and the AGP increased from N0 to N2, but N2 and N3 do not have a noticeable difference. Different N times do not show significant effects on maize grain-filling characteristics parameters. N0 and N1T2 prolonged Tmax but reduced Gmax, Gmax, Gmax, and AGP. On average, the Gmax was increased by N2 and N3 in both years.
N application time did not significantly affect the duration, weight, and grain-filling rate during GIP, RIP, and SIP under the same N rate (Table 4). Compared with other treatments, N0 and N1 prolonged the duration of GIP but shortened the duration of RIP and SIP. The duration of RIP under N0, N2, and N3 accounted for 27.65, 29.50, and 30.31% in 2019 and 29.16, 31.20, and 31.75% in 2020 of the whole grain-filling duration, respectively. The average grain-filling rate of N2 was 59.6% and 53.8% higher during GIP and 31.4% and 24.6% higher during RIP than N0 in 2019 and 2020, respectively. Similarly, the grain-filling rate of N3 was 65.6 and 61.5% higher during GIP and 25.6 and 23% higher during RIP than N0 in 2019 and 2020, respectively. Correlation analysis showed that 100-grain weight was significantly positively correlated with Wmax, Gmax, Gmax, G1, T2, G2, T3, and G3, and negatively correlated with T1 (Table 5).
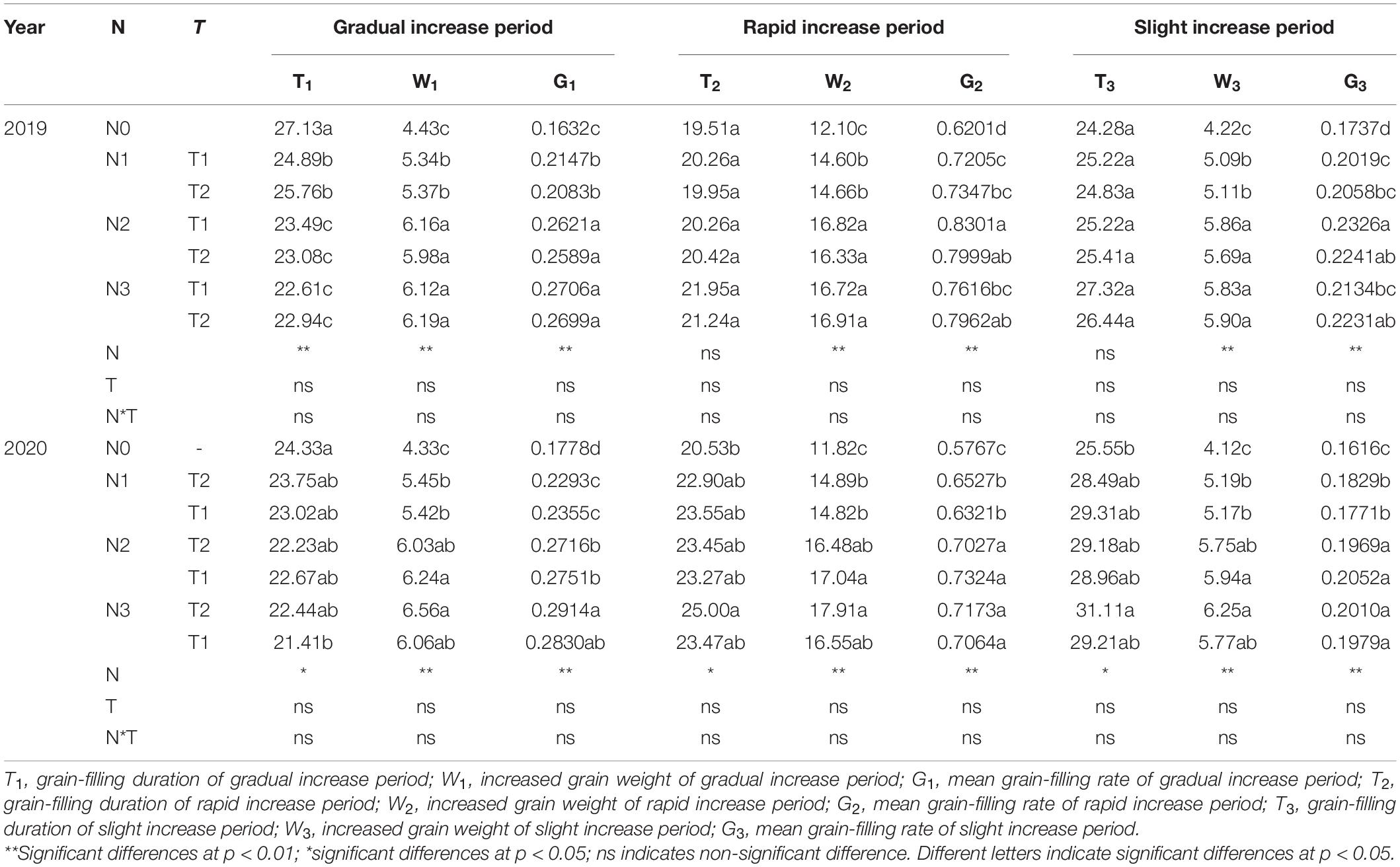
Table 4. Effects of N rate and time on characteristics parameters of the three grain-filling phases of maize grains.
Hormone Content
The IAA, CTK, and ABA content increased first and then declined; however, GA content declined gradually during the grain-filling process (Figure 6). IAA, ABA, and CTK significantly increased under N2 and N3 compared with N0 and reached a maximum value at 21 DAP. Furthermore, there were no significant differences between N2 and N3, T2 and T1 under the same N rate for most hormones. On average, the peak of the IAA, ABA, and CTK was higher under the N2 than N0 treatments which were increased by 26.2, 8.7, and 19.1% in 2019 and 35.9, 25.6, and 40.7% in 2020. The N2 and N3 treatments significantly improved GA content as compared with N0, while there was no significant difference in GA content between N1 and N0 from 7 to 21 DAP.
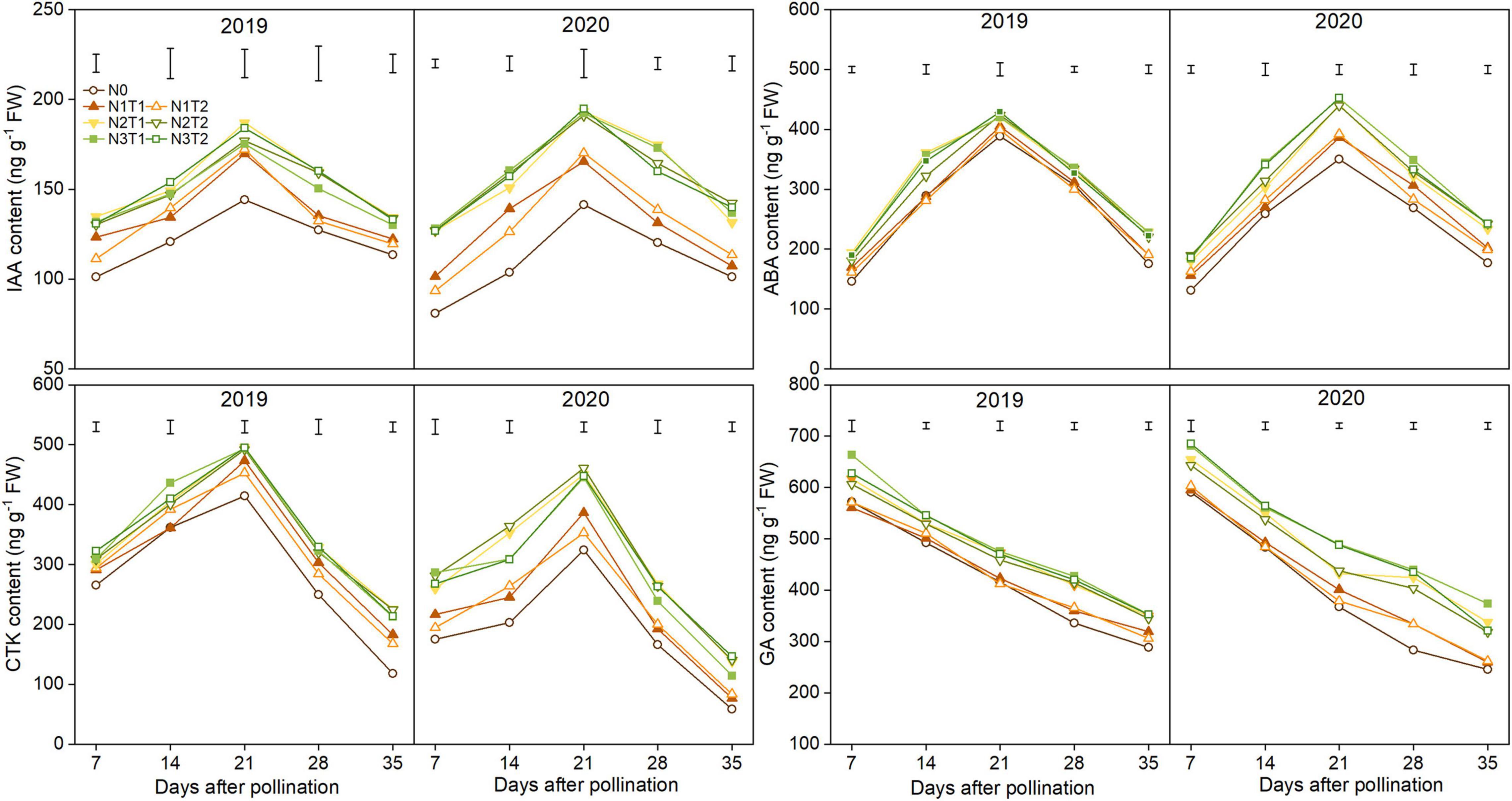
Figure 6. Effects of N rate and time on the content of indole-3-acetic acid (IAA), cytokinin (CTK), abscisic acid (ABA), and gibberellic acid (GA) in 2019 and 2020. The vertical bars represent the LSD (n = 3).
Correlation Between Grain-Filling Characteristic Parameters and Hormone Content
Hormone contents at 7, 14, 28, 35, and 42 DAP were set as variables a1, a2, a3, a4, and a5, while, Wmax, Gmax, Gmax, W2, and G2 were set as b1, b2, b3, b4, and b5, respectively (Table 6). As Table 6, the variation of grain-filling parameters depended on the content of ABA at 35 DAP and GA at 28 DAP. There were two significant standardized analytical models between IAA content and grain-filling parameters in 2019, and as the first and second typical variables showed that IAA at 35 DAP and IAA at 14 DAP had a greater impact on grain-filling parameters. However, IAA at 35 DAP had an advantage in affecting grain-filling parameters in 2020. CTK affected grain-filling parameters at the late grain-filling stage (28 DAP in 2019 and 35 DAP in 2020).
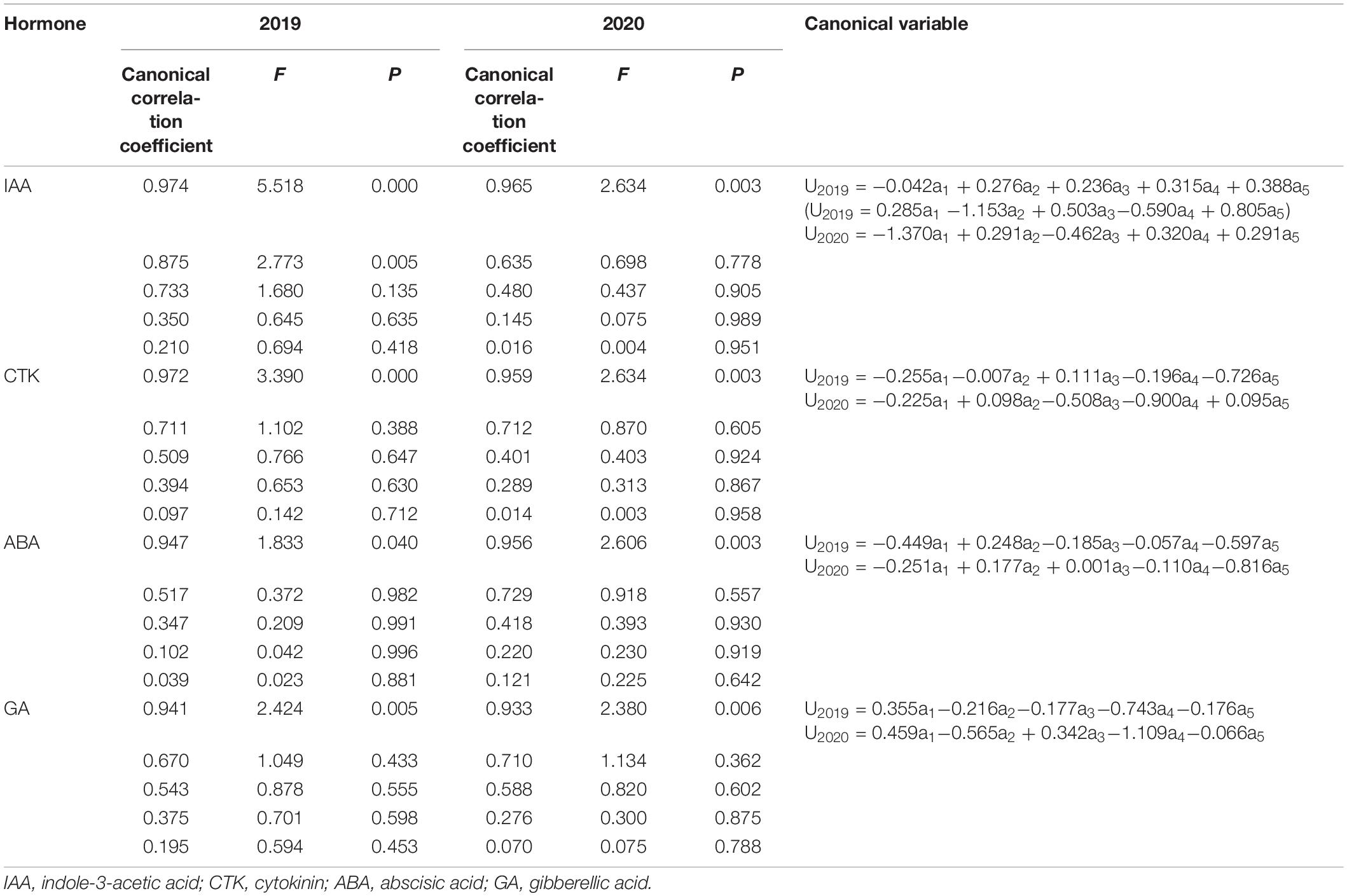
Table 6. Canonical correlation coefficient between grain-filling parameters and hormone content and standardized analytical model.
Discussion
Contribution of Yield Components to Yield Under Different Nitrogen Applications
N2 and N3 significantly increased about 200% yield compared to N0, this may N enhance ears ha–1, 100-grain weight, and grains per plant, which were the momentous factor influencing maize yield (Wei et al., 2017; Srivastava et al., 2018). Qian et al. (2016) showed that N increased grains per ear and 1,000-grain weight, which subsequently led to an increase in the grain yield. Our result also confirms that yield increased mainly caused by greater ears ha–1, associated with an increase in grains per plant, and greater 100-grain weight. The correlations between yield and 100-grain weight (R2 = 0.514 in 2019, R2 = 0.602 in 2020) were less than and that yield and ears ha–1 (R2 = 0.836 in 2019, R2 = 0.645 in 2020), which provided further evidence that grain weight is more conservative than the grains per plant in response to varying environmental conditions (Tsimba et al., 2013). However, once the density and variety are determined, it is difficult to change the ears ha–1 unless there is insufficient nutrition (Zhang et al., 2014; Li et al., 2017). As mentioned above, this may also be the reason why there is no significant difference between N2 and N3 on ears ha–1, and N0 significantly reduces ears ha–1. It is feasible that N optimized maize grain filling increased 100-grain weight since maximum grain size was defined primarily during the lag phase of grain filling (Tsimba et al., 2013). The yield of N3 and N2 did not have a significant difference; this might be because N2 can basically meet fundamental conditions for growing maize in this area, furthermore, N3 promoted the development of vegetative organs but possibly inhibited the transport of nutrients (Li et al., 2017). As reported by Chen et al. (2015), the yield increased obviously with an increase in N application (0–240 kg N ha–1); however, enhancing N application has little effect on yield when N application reaches a certain limit. Wazir and Akmal (2019) found that three-split N application maintained ample nutrients for maize grain development, which might profit for higher grain yield. In our study, under the same N rate, N application time could not alter yield significantly. The reason may be due to the fact that plastic film mulch enhanced mineralization of soil N and improves the availability of N, thus weakening the effect of N application time on yield. The yield in T2 was higher than T1 under N3 in 3 years, there may be a more favorable N combination between N2 and N3; however, the specific N application needs further study. The results illustrated that N rate rather than N application time was the central factor affecting the yield when N application rate is less than 200 kg N ha–1 (Li and Li, 2015). The yield in 2018, 2019, and 2020 varied wildly. According to Lu et al. (2017), less sunshine inhibits pollination and dry matter accumulation, resulting in lower yield. Precipitation is also a determining factor of yield in rainfed agriculture. Lower yield in 2018 may be due to the lowest in crop rainfall and fewer sunny days during grain filling in 2018.
Nitrogen Affect Grains Weight by Regulating Grain Filling
Grain filling is an essential physiological process that determines grain formation. Appropriate N rate and time contribute to the rate and duration of grain filling, determining grain weight (Zhou et al., 2017; Wei et al., 2019). In our study, Gmax, Gmax, G1, G2, and G3 were positively correlated with 100-grain weight, but the T1 was negatively correlated with 100-grain weight. So, in this area, the filling rate determined the formation of grains under different N rates and time combinations. However, Bolaños (1995) showed that variation in grains weight due to the duration of grain filling but not the grain-filling rate. Similar studies have found that the change in grain weight of the same variety is mainly affected by the filling rate, but the difference of different varieties depends on the duration of grain filling (Yang et al., 2008; Wei et al., 2019). Wmax, Gmax, Gmax, and the duration and average grain-filling rate of GIP, RIP, and SIP improved with the N increase, but N2 and N3 had no significant differences, indicating that N application is not the critical factor to control the filling rate when it reaches 200 kg ha–1 in this region. The grain-filling process of maize show an “S”-shaped curve, and the grain-filling rate show the characteristics of slow-fast-slow. In our research, the duration of RIP under different treatments accounted for 27.65∼31.75% of the whole grain-filling duration. Miao et al. (2018) found that the duration of RIP is up to 36.9% of the whole grain-filling duration, so we should pay attention to the duration of RIP to obtain a higher grain weight. There is a mechanism that shortens the duration of GIP while prolonging the period of RIP, increasing 100-grain weight (Wei et al., 2019). Our result also showed that compared to N0, N2, and N3 shorten the duration of the GIP and prolonged the period of RIP, thus increasing grains weight. Under the same N rate, N application time could not alter grain-filling parameters significantly, which determined that the change of grain weight at N application time was not significant.
Nitrogen Affect Grain Filling Through Regulating Starch Accumulation
Sucrose, the main form of carbon transport, is produced by plant photosynthesis, and the breakdown of sucrose provides intermediates for the synthesis of starch (Farrar et al., 2000). The sucrose content of N2 and N3 was higher than N0 and N1 and reached a maximum value at 14 DAP (except for N2T1 in 2020). These results indicated that N2 and N3 were conducive to sucrose accumulation at the early grain filling, which laid the foundation for starch metabolizing. The reason for sucrose content accumulation is that N increased SPS and SuSy activities, which are the key enzyme in sucrose synthesis (Yang et al., 2008). At 35 DAP, the sucrose content did not show significant changes among N0, N1, N2, and N3, this may be N deficiency preventing the transformation from sucrose to starch (Rufty et al., 1983; Zhao et al., 2013). AGPase and SSS were sensitive to N, indicating that N could regulate the biosynthesis rate of maize starch by affecting AGPase and SSS (Liu et al., 2021). Our result also showed that N2 and N3 could significantly increase AGPase and SSS activities compared to N0. Higher AGPase and SSS activities promoted the accumulation of starch, which is beneficial to the final grain weight (Liang et al., 2017). However, Fahy et al. (2018) found that final grain weight was not determined by AGPase or starch synthase activities during grain filling, and the improvement of grain weight was mainly due to developmental processes before grain filling. Under the same N rate, the starch content and starch metabolizing enzymes showed no significant difference between T1 and T2. This may be one of the reasons why there is no significant change in 100-grain weight between T1 and T2.
Relationship Between Hormone Content and Grain-Filling Characteristics
Phytohormones, namely, CTK, IAA, ABA, and GA, are instrumental in regulating the development of maize grains by affecting grain cell division and expansion (Yang et al., 2006; Liu et al., 2013). At the grain-filling stage, N application significantly altered hormone contents; however, the change trends (IAA, ABA, and CTK contents first increased and then decreased, GA content declined gradually) were not affected. The proper amount of auxin content can facilitate grain filling by regulating endosperm development, while low IAA constrained the grain weight (Wang et al., 2006). In this study, CTK increased in the early stage of the maize grain-filling period. The high levels of CTKs at the early stage of grains development promote cell division and grain filling (Yang et al., 2000). Our data showed that N deficiency limits the synthesis of CTK, thus reducing grain weight. Liang et al. (2017) found that CTK contents were significantly and positively correlated with the SSS and AGPase activities in grain, higher CTK increased SSS and AGPase activities, thus promoting grain filling. In addition, IAA and CTK content was positively correlated with the Gmax and Gmax, and the reason for this may be high IAA levels induced by CTK level (Liu et al., 2016). In our study, the IAA and CTK transiently increased, reached a maximum value at 21 DAP, and then decreased. The canonical correlation between grain-filling parameters and the content of IAA and CTK showed that N application altered the grain-filling process mainly by altering IAA and CTK content. So, the lower 100-grain weight by N deficiency could be attributed to the low CTK and IAA content. ABA has a role in the transport and accumulation of photosynthetic products that regulate the starch-metabolizing enzyme activities in starch biosynthesis (Travaglia et al., 2007; Zhang et al., 2017). The N2 and N3 had significantly increased the ABA content. Previous research showed that the high ABA in the late grain-filling stage was not conducive to the grain filling, and eventually affected grain weight (Wei et al., 2019), and the findings were confirmed as identified by the standardized analytical model in our study. GA promotes endosperm cell proliferation at the early grain-filling progress, but GA increased α-amylase or other hydrolytic enzymes at the late grain filling, which accelerate starch decomposition (Yang et al., 2001; Xu et al., 2015). In this study, GA content reached the maximum value at 7 DAP and increased with increasing N rate, which heightened grain elongation rapidly, and the results are similar with Li et al. (2019). Furthermore, the result also was proved by the standardized analytical model in our study.
Conclusion
Yield, yield components, grain-filling parameters, starch metabolizing enzymes, and endogenous hormones of maize grain were significantly affected by N rate (200 and 300 kg N ha–1 did not have significant difference), but N application time did not show significant difference under the same N rate. As N increased to 200 kg N ha–1, the IAA, CTK, and GA content and starch-metabolizing enzymes such as SuSy, SPS, AGPase, and SSS, which regulated grain filling, were significantly improved as compared with 0 kg N ha–1. Furthermore, all hormones had strong canonical correlations with the grain-filling parameters (Wmax, Gmax, Gmax, W2, and G2). The grain-filling parameters were significantly positively correlated with 100-grain weight. N increased ears ha–1, 100-grain weight, and the number of grains per plant, which enhanced maize production compared with 0 kg N ha–1. Therefore, we concluded that 200 and 300 kg N ha–1 optimized grain-filling parameters by affecting hormones and enzymes, thus improving yield. Considering inputs and environmental factors comprehensively, 200 kg N ha–1 with one-third applied at sowing and two-thirds at the six-leaf stage, may be prioritized to choose in the semiarid Loess Plateau of China.
Data Availability Statement
The raw data supporting the conclusions of this article will be made available by the authors, without undue reservation.
Author Contributions
KY and LL: conceptualization. KY and JuX: methodology. SF: software. KY, LL, and YL: validation. KY: formal analysis, investigation, and writing—original draft preparation. JuX: resources. KY and JiX: data curation. KY, LL, SA, and SF: writing—review and editing. All authors have read and agreed to the published version of the manuscript.
Funding
This study was supported by the Education Science and Technology Innovation Project of Gansu Province (GSSYLXM-02), the National Natural Science Foundation of China (31761143004), the Department of Science and Technology of Gansu Province (20JR5RA033), and the Fund for Young Postgraduate Supervisors of Gansu Agricultural University (GAU-QDFC-2020-03).
Conflict of Interest
The authors declare that the research was conducted in the absence of any commercial or financial relationships that could be construed as a potential conflict of interest.
Publisher’s Note
All claims expressed in this article are solely those of the authors and do not necessarily represent those of their affiliated organizations, or those of the publisher, the editors and the reviewers. Any product that may be evaluated in this article, or claim that may be made by its manufacturer, is not guaranteed or endorsed by the publisher.
Acknowledgments
We would like to appreciate assistance in the field and laboratory by students of the Rainfed Agricultural Experimental Station of the Gansu Agricultural University.
References
Banger, K., Nafziger, E. D., Wang, J., Muhammad, U., and Pittelkow, C. M. (2018). Simulating nitrogen management impacts on maize production in the US Midwest. PLoS One 13:e0201825. doi: 10.1371/journal.pone.0201825
Bolaños, J. (1995). Physiological bases for yield differences in selected maize cultivars from Central America. Field Crops Res. 42, 69–80. doi: 10.1016/0378-4290(95)00022-I
Cao, Y., Dou, J., Gao, Y., Wei, W., Lv, Y., Yao, F., et al. (2015). Effect of nitrogen application on yield and grain filling Characteristics under Different Densities of Maize. J. Maize Sci. 23, 136–141. doi: 10.13597/j.cnki.maize.science.20150624
Cazetta, J. O., Seebauer, J. R., and Below, F. E. (1999). Sucrose and nitrogen supplies regulate growth of maize kernels. Ann. Bot. 84, 747–754. doi: 10.1006/anbo.1999.0976
Chen, Y., Xiao, C., Wu, D., Xia, T., Chen, Q., Chen, F., et al. (2015). Effects of nitrogen application rate on grain yield and grain nitrogen concentration in two maize hybrids with contrasting nitrogen remobilization efficiency. Eur. J. Agron. 62, 79–89. doi: 10.1016/j.eja.2014.09.008
Ciampitti, I. A., and Vyn, T. J. (2012). Physiological perspectives of changes over time in maize yield dependency on nitrogen uptake and associated nitrogen efficiencies: a review. Field Crops Res. 133, 48–67. doi: 10.1016/j.fcr.2012.03.008
Fahy, B., Siddiqui, H., David, L. C., Powers, S. J., Borrill, P., Uauy, C., et al. (2018). Final grain weight is not limited by the activity of key starch-synthesising enzymes during grain filling in wheat. J. Exp. Bot. 69, 5461–5475. doi: 10.1093/jxb/ery314
Fang, H., Gu, X., Jiang, T., Yang, J., Li, Y., Huang, P., et al. (2020). An optimized model for simulating grain-filling of maize and regulating nitrogen application rates under different film mulching and nitrogen fertilizer regimes on the Loess Plateau, China. Soil Tillage Res. 199:104546. doi: 10.1016/j.still.2019.104546
Farrar, J., Pollock, C., and Gallaghet, J. (2000). Sucrose and the integration of in metabolism in vascular Plants. Plant Sci. 154, 1–11. doi: 10.1016/S0168-9452(99)00260-5
Food and Agriculture Organization [FAO] (1990). Soil Map of the World, Revised Legend. World Soil Resource Report, Vol. 60. Rome: FAO.
Fu, J., Xu, Y., Chen, L., Yuan, L., Wang, Z. Q., and Yang, J. C. (2013). Changes in enzyme activities involved in starch synthesis and hormone concentrations in superior and inferior spikelets and their association with grain filling of super rice. Rice sci. 20, 120–128. doi: 10.1016/S1672-6308(13)60116-X
Jiang, X. L., Miao, Y. L., Yang, F. T., and Li, G. (2009). Formation of grain sink of maize (Zea mays L.) and it’s hormonal regulation. J. Jilin Agric. Sci. 2, 14–16. doi: 10.16423/j.cnki.1003-8701.2009.02.009
Kong, L., Xie, Y., Hu, L., Si, J., and Wang, Z. (2017). Excessive nitrogen application dampens antioxidant capacity and grain filling in wheat as revealed by metabolic and physiological analyses. Sci. Rep. 7:43363. doi: 10.1038/srep43363
Li, C. Y., Feng, C. N., Zhang, Y., Guo, W. S., Zhu, X. K., and Peng, Y. X. (2005). Effects of the ratio between basal N and topdressing N on grain starch formation in weak gluten wheat variety Ningmai 9 and it’s enzymes activities. Sci. Agric. Sin. 38, 1120–1125.
Li, G., Liu, J., Dong, S., Liu, P., Zhang, J., Zhao, B., et al. (2017). Effects of close planting and nitrogen application rates on grain yield and nitrogen utilization efficiency of different density-tolerance maize hybrids. Sci. Agric. Sin. 50, 2247–2258. doi: 10.3864/j.issn.0578-1752.2017.12.006
Li, X. G., and Li, F. M. (2015). Soil organic carbon balance and nitrogen cycling in plastic film mulched croplands in rainfed farming systems. Sci. Agric. Sin. 48, 4630–4638. doi: 10.3864/j.issn.0578-1752.2015.23.004
Li, Y., Yang, L., Wang, H., Xu, R., Chang, S., Hou, F., et al. (2019). Nutrient and planting modes strategies improves water use efficiency, grain filling and hormonal changes of maize in semi-arid regions of China. Agric. Water Manag. 2019:105723. doi: 10.1016/j.agwat.2019.105723
Liang, W., Zhang, Z., Wen, X., Liao, Y., and Liu, Y. (2017). Effect of non-structural carbohydrate accumulation in the stem pre-anthesis on grain filling of wheat inferior grain. Field Crops Res. 211, 66–76. doi: 10.1016/j.fcr.2017.06.016
Liu, X. M., Gu, W. R., Li, C. F., Li, J., and Wei, S. (2021). Effects of nitrogen fertilizer and chemical regulation on spring maize lodging characteristics, grain filling and yield formation under high planting density in Heilongjiang Province, China. J. Integr. Agric. 20, 511–526. doi: 10.1016/S2095-3119(20)63403-7
Liu, Y., Liang, H., Lv, X., Liu, D., Wen, X., and Liao, Y. (2016). Effect of polyamines on the grain filling of wheat under drought stress. Plant Physiol. Biochem. 100, 113–129. doi: 10.1016/j.plaphy.2016.01.003
Liu, Y., Sui, Y., Gu, D., Wen, X., Chen, Y., Li, C., et al. (2013). Effects of conservation tillage on grain filling and hormonal changes in wheat under simulated rainfall conditions. Field Crops Res. 144, 43–51. doi: 10.1016/j.fcr.2013.01.009
Lu, H. D., Xue, J. Q., and Guo, D. W. (2017). Efficacy of planting date adjustment as a cultivation strategy to cope with drought stress and increase rainfed maize yield and water-use efficiency. Agric. Water Manag. 179, 227–235. doi: 10.1016/j.agwat.2016.09.001
Luo, Y., Tang, Y., Zhang, X., Li, W., Chang, Y., Pang, D., et al. (2018). Interactions between cytokinin and nitrogen contribute to grain mass in wheat cultivars by regulating the flag leaf senescence process. Crop J. 6, 538–551. doi: 10.1016/j.cj.2018.05.008
Miao, Y., Yan, J., Zhao, D., Tian, Y., Yan, J., Xian-Chun, X., et al. (2018). Relationship between grain filling parameters and grain weight in leading wheat cultivars in the yellow and Huai Rivers Valley. Acta Agronomica Sin. 44, 260–267. doi: 10.3724/SP.J.1006.2018.00260
Ning, P., Fritschi, F. B., and Li, C. (2017). Temporal dynamics of post-silking nitrogen fluxes and their effects on grain yield in maize under low to high nitrogen inputs. Field Crops Res. 204, 249–259. doi: 10.1016/j.fcr.2017.01.022
Ning, P., Yang, L., Li, C., and Fritschi, F. B. (2018). Post-silking carbon partitioning under nitrogen deficiency revealed sink limitation of grain yield in maize. J. Exp. Bot. 69, 1707–1719. doi: 10.1093/jxb/erx496
Qian, C., Yu, Y., Gong, X., Jiang, Y., Zhao, Y., Yang, Z., et al. (2016). Response of grain yield to plant density and nitrogen rate in spring maize hybrids released from 1970 to 2010 in Northeast China. Crop J. 4, 459–467.
Qin, S., Zhang, Z., Ning, T., Ren, S., Su, L., and Li, Z. (2013). Abscisic acid and aldehyde oxidase activity in maize ear leaf and grain relative to post-flowering photosynthetic capacity and grain filling rate under different water/nitrogen treatments. Plant Physiol. Biochem. 70, 69–80. doi: 10.1016/j.plaphy.2013.04.024
Rook, F., Corke, F., Card, R., Munz, G., Smith, C., and Bevan, M. W. (2001). Impaired sucrose-induction mutants reveal the modulation of sugar-induced starch biosynthetic gene expression by abscisic acid signaling. Plant J. 26, 421–433. doi: 10.1046/j.1365-313X.2001.2641043.x
Rufty, T. W., Kerr, P. S., and Huber, S. C. (1983). Characterization of diurnal changes in activities of enzymes in sucrose biosynthesis. Plant Physiol. 73, 428–433. doi: 10.1104/pp.73.2.428
Seebauer, J. R., Singletary, G. W., Krumpelman, P. M., Ruffo, M. L., and Below, F. E. (2010). Relationship of source and sink in determining kernel composition of maize. J. Exp. Bot. 61, 511–519. doi: 10.1093/jxb/erp324
Srivastava, R. K., Panda, R. K., Chakraborty, A., and Halder, D. (2018). Enhancing grain yield, biomass and nitrogen use efficiency of maize by varying sowing dates and nitrogen rate under rainfed and irrigated conditions. Field Crops Res. 221, 339–349. doi: 10.1016/j.fcr.2017.06.019
Thind, H. S., Kumar, A., and Vashistha, M. (2011). Calibrating the leaf colour chart for need based fertilizer nitrogen management in different maize (Zea mays L.) genotypes. Field Crops Res. 120, 276–282. doi: 10.1016/j.fcr.2010.10.014
Thitisaksakul, M., Jiménez, R. C., Arias, M. C., and Beckles, D. M. (2012). Effects of environmental factors on cereal starch biosynthesis and composition. J. Cereal Sci. 56, 67–80. doi: 10.1016/j.jcs.2012.04.002
Travaglia, C., Cohen, A. C., Reinoso, H., Castillo, C., and Bottini, R. (2007). Exogenous abscisic acid increases carbohydrate accumulation and redistribution to the grains in wheat grown under field conditions of soil water restriction. J. Plant Growth Regul. 26, 285–289. doi: 10.1007/s00344-007-9018-3
Tsimba, R., Edmeades, G. O., Millner, J. P., and Kemp, P. D. (2013). The effect of planting date on maize grain yields and yield components. Field Crops Res. 150, 135–144. doi: 10.1016/j.fcr.2013.05.028
Wang, F., Cheng, F., and Zhang, G. (2006). The relationship between grain filling and hormone content as affected by genotype and source-sink relation. Plant Growth Regul. 49, 1–8. doi: 10.1007/s10725-006-0017-3
Wang, G., and Zhang, J. (2020). Carbohydrate, hormone and enzyme regulations of rice grain filling under post-anthesis soil drying. Environ. Exp. Bot. 178:104165. doi: 10.1016/j.envexpbot.2020.104165
Wang, L., Kang, J., Lang, Y., Feng, P., and Xu, C. (2018). Effect of controlled release and conventional urea fertilizer application on grain filling characteristics and yield of spring maize. J. Nuclear Agric. Sci. 32, 2054–2061.
Wazir, M. S., and Akmal, M. (2019). Nitrogen split application timing effect on wheat intercrop with selected species for sustainable production. Sarhad J. Agric. 35, 70–79. doi: 10.17582/journal.sja/2019/35.1.70.79
Wei, S., Wang, X., Li, G., Qin, Y., Jiang, D., and Dong, S. T. (2019). Plant density and nitrogen supply affect the grain filling parameters of maize kernels located in different ear positions. Front. Plant Sci. 10:180. doi: 10.3389/fpls.2019.00180
Wei, T., Hu, F., Zhao, C., Feng, F., Yu, A., Liu, C., et al. (2017). Response of dry matter accumulation and yield components of maize under N-fertilizer postponing application in oasis irrigation areas. Sci. Agric. Sin. 50, 2916–2927. doi: 10.3864/j.issn.0578-1752.2017.15.006
Xu, Y., Gu, D., Qin, H., Zhang, H., Wang, Z. Q., and Yang, J. C. (2015). Changes in carbohydrate accumulation and activities of enzymes involved in starch synthesis in maize kernels at different positions on an ear during grain filling. Acta Agronomica Sin. 41, 297–307. doi: 10.3724/SP.J.1006.2015.00297
Xu, Y., Gu, D., Zhang, B., Zhang, H., Wang, Z., and Yang, J. (2013). Hormone contents in kernels at different positions on an ear and their relationship with endosperm development and kernel filling in maize. Acta Agronomica Sin. 39, 1452–1461. doi: 10.3724/SP.J.1006.2013.01452
Yang, H., Gu, X., Ding, M., Lu, W., and Lu, D. (2018). Heat stress during grain filling affects activities of enzymes involved in grain protein and starch synthesis in waxy maize. Sci. Rep. 8:15665. doi: 10.1038/s41598-018-33644-z
Yang, J., and Zhang, J. (2006). Grain filling of cereals under soil drying. New Phytol. 169, 223–236. doi: 10.1111/j.1469-8137.2005.01597.x
Yang, J., and Zhang, J. (2010). Grain filling problem in ‘super’ rice. J. Exp. Bot. 61, 1–5. doi: 10.1093/jxb/erp348
Yang, J., Zhang, J., Wang, Z., Zhu, Q., and Wang, W. (2001). Hormonal changes in the grains of rice subjected to water stress during grain filling. Plant Physiol. 127, 315–323. doi: 10.1104/pp.127.1.315
Yang, J. C., Peng, S. B., Visperas, R. M., Sanico, A. L., Zhu, Q. S., and Gu, S. L. (2000). Grain filling pattern and cytokinin content in the grains and roots of rice plants. Plant Growth Regul. 30, 261–270. doi: 10.1023/A:1006356125418
Yang, J. C., Zhang, J. H., Liu, K., Wang, Z. Q., and Liu, L. J. (2006). Abscisic acid and ethylene interact in wheat grains in response to soil drying during grain filling. New Phytol. 171, 293–303. doi: 10.1111/j.1469-8137.2006.01753.x
Yang, W., Peng, S., Dionisio-Sese, M. L., Laza, R. C., and Visperas, R. M. (2008). Grain filling duration, a crucial determinant of genotypic variation of grain yield in field-grown tropical irrigated rice. Field Crops Res. 105, 221–227. doi: 10.1016/j.fcr.2007.10.006
Zhang, L., Li, X. H., Gao, Z., Shen, S., Liang, X. G., Zhao, X., et al. (2017). Regulation of maize kernel weight and carbohydrate metabolism by abscisic acid applied at the early and middle post-pollination stages in vitro. J. Plant Physiol. 216, 1–10. doi: 10.1016/j.jplph.2017.05.005
Zhang, Q., Zhang, L., Evers, J., van der Werf, W., Zhang, W., and Duan, L. (2014). Maize yield and quality in response to plant density and application of a novel plant growth regulator. Field Crops Res. 164, 82–89. doi: 10.1016/j.fcr.2014.06.006
Zhang, Z. L., and Qu, W. J. (2003). Guidance of Plant Physiology Experiment. Beijing: Higher Education Press, 128–129.
Zhao, F. C., Jing, L. Q., Yan, F. B., Lu, D. L., Wang, G. Y., and Lu, W. P. (2013). Effect of nitrogen fertilization on yield, quality and enzyme activity associated with sucrose metabolism of sweet corn. Plant Nutr. Fertilizer Sci. 19, 45–53.
Zhou, B., Yue, Y., Sun, X., Ding, Z., Ma, W., and Zhao, M. (2017). Maize kernel weight responses to sowing date-associated variation in weather conditions. Crop J. 5, 43–51. doi: 10.1016/j.cj.2016.07.002
Keywords: nitrogen application, maize yield, hormone, starch metabolizing enzymes, grain filling
Citation: Yue K, Li L, Xie J, Liu Y, Xie J, Anwar S and Fudjoe SK (2022) Nitrogen Supply Affects Yield and Grain Filling of Maize by Regulating Starch Metabolizing Enzyme Activities and Endogenous Hormone Contents. Front. Plant Sci. 12:798119. doi: 10.3389/fpls.2021.798119
Received: 27 October 2021; Accepted: 31 December 2021;
Published: 02 February 2022.
Edited by:
Jose M. Garcia-Mina, University of Navarra, SpainReviewed by:
Muhammad Kamran, Lanzhou University, ChinaAdalberto Benavides-Mendoza, Universidad Autónoma Agraria Antonio Narro, Mexico
Copyright © 2022 Yue, Li, Xie, Liu, Xie, Anwar and Fudjoe. This is an open-access article distributed under the terms of the Creative Commons Attribution License (CC BY). The use, distribution or reproduction in other forums is permitted, provided the original author(s) and the copyright owner(s) are credited and that the original publication in this journal is cited, in accordance with accepted academic practice. No use, distribution or reproduction is permitted which does not comply with these terms.
*Correspondence: Lingling Li, lill@gsau.edu.cn