- 1Institute of Ecology and Biodiversity, School of Life Sciences, Shandong University, Qingdao, China
- 2Shandong Provincial Engineering and Technology Research Center for Vegetation Ecology, Shandong University, Qingdao, China
- 3Qingdao Forest Ecology Research Station of National Forestry and Grassland Administration, Shandong University, Qingdao, China
- 4Qingdao Forestry Station, Qingdao, China
Changing precipitation patterns have aggravated the existing uneven water distribution, leading to the alternation of drought and rewatering. Based on this variation, we studied species, namely, Robinia pseudoacacia and Quercus acutissima, with different root forms and water regulation strategy to determine physiological responses to repeated drought-rewatering under different planting methods. Growth, physiological, and hydraulic traits were measured using pure and mixed planting seedlings that were subjected to drought, repeated drought-rewatering (i.e., treatments), and well-irrigated seedlings (i.e., control). Drought had negative effects on plant functional traits, such as significantly decreased xylem water potential (Ψmd), net photosynthetic rate (AP), and then height and basal diameter growth were slowed down, while plant species could form stress imprint and adopt compensatory mechanism after repeated drought-rewatering. Mixed planting of the two tree species prolonged the desiccation time during drought, slowed down Ψmd and AP decreasing, and after rewatering, plant functional traits could recover faster than pure planting. Our results demonstrate that repeated drought-rewatering could make plant species form stress imprint and adopt compensatory mechanism, while mixed planting could weaken the inhibition of drought and finally improve the overall drought resistance; this mechanism may provide a theoretical basis for afforestation and vegetation restoration in the warm temperate zone under rising uneven spatiotemporal water distribution.
Introduction
Precipitation patterns have been substantially altered as a consequence of global climate change, specifically, the frequency and intensity of precipitation increase, but the total amount decreases (Easterling et al., 2000; Högy et al., 2013; Gimbel et al., 2015; Ge et al., 2017). This phenomenon has aggravated the existing spatiotemporal uneven water distribution in the warm temperate zone (Luan et al., 2011; Corlett, 2016), leading to the alternation of drought and rewatering. Severe drought may lead to plant hydraulic failure, which has been invoked as the most direct and critical mechanism causing tree dieback and forest mortality (Martinez-Vilalta and Pinol, 2002; Nardini et al., 2013; O’Grady et al., 2013; Liu et al., 2020). After drought, the pace of recovery differs among physiological processes; leaf water potential typically recovers within few days even few hours upon rewatering, while leaf gas exchange-related variables lag (Ruehr et al., 2019), leading to plant growth traits slow recovery (Li et al., 2019, 2020).
Drought and rewatering will be likely repeated (Tomasella et al., 2019). There are two main strategies for plants to respond to repeated drought-rewatering. One is stress imprint, which means that the history of exposure to stresses will change the subsequent responses of plant species, make them more resistant, and respond faster to future stresses (Walter et al., 2013; Forner et al., 2018; Tomasella et al., 2019). Main mechanisms of stress imprint are storage of stress-resistant substances through physiological and biochemical responses, accumulation of signal proteins or transcription factors, and epigenetic changes caused by DNA methylation (Bruce et al., 2007; Liu and He, 2020). The other is pressure fatigue, which refers to the phenomenon that under cyclic stress, plant functional traits cannot be completely recovered, gradually weaken, or even lose (Umebayashi et al., 2019), such as under repeated drought, hydraulic conductivity could not recover to the origin level after rewatering (Moran et al., 2017). In that way, in the warm temperate zone, which is the main strategy for plant species to respond to the repeated drought-rewatering?
Ecological theories suggest that multigroup composite structures of community often show large differences in ecological strategies for coping with environmental stress (Richards et al., 2010; Forrester and Bauhus, 2016). Some previous studies have reported contradictory findings about the relationship between plant diversity and drought. Lebourgeois et al. (2013) and Pretzsch et al. (2013) show that the mixed-species forests are divided by hydrological niches, which could reduce the competition for limited water resources and strengthened regional drought resistance. Such as in mixed planting, the differences in the distribution and structure of plant roots of different species lead to less overall competition for water than that in pure planting (Schwendenmann et al., 2015), which may prolong the desiccation time of drought (Blackman et al., 2019). However, if the interacting species have similar functional characteristics (i.e., functional redundancy), ecological niche overlap may lead to increasing water competition, which in turn increases the degree of drought. Therefore, it is important to understand how mixed-species forests of specific species in a limited space to cope with drought together. However, due to the multiple interrelated processes and complex feedback mechanisms between plant diversity and drought stress, it is still unknown whether mixed-species forests can attenuate regional drought stress.
Robinia pseudoacacia L., shallow-rooted species with wide root crown width, and Quercus acutissima Carr., deep-rooted species with narrow root crown width, are dominant species in the tree layer of temperate deciduous broadleaved forests in Northern China. They are widely dispersed sympatric tree species in Northern China (Wang and Zhou, 2000; Wang et al., 2020), and in recent research, they are renowned for their high drought tolerance and fast recovery capability in the warm temperate zone (Xu et al., 2009; Du et al., 2017; Li et al., 2019; Wang et al., 2020). Moreover, on the “isohydric-anisohydric” continuous spectrum, R. pseudoacacia is an anisohydric species, while Q. acutissima is an isohydric one (Wang and Zhou, 2000; Li et al., 2020). Besides, there are many seedlings in the regeneration layer under forests (Wang et al., 2021). The regeneration of woody plant seedlings is an important component of maintaining the vegetation diversity of forest ecosystem (Fukushima et al., 2008), playing a central role in the process of community assembly and forest dynamic changes (Nyland et al., 2006). Therefore, we set up a repeated drought-rewatering experiment to find out how plant functional traits of R. pseudoacacia and Q. acutissima respond to repeated drought and rewatering under different planting methods. We hypothesized that (1) the first drought may make the two plant species form stress imprint, resulting in fast recovery of plant functional traits after repeated drought-rewatering and (2) mixed planting may weaken the negative effects of drought.
Materials and Methods
Plant Materials
This study was conducted at the Fanggan Research Station at Shandong University in Jinan, Shandong Province, China (36°26′ N, 117°27′ E). Seeds from R. pseudoacacia L. were collected from the trees in our common garden, while seeds from Q. acutissima Carr. were collected from the trees on a mountain nearby, and they were stored at 4°C in a refrigerator. These seeds were germinated in a growth chamber filled with humus soil in early April 2018. When most seedlings reached 10 cm, healthy and uniform germinants were sown in plastic pots (32 × 29 cm, height × diameter) with an 8 kg mixed sandy loam and humus soil, whose holding capacity of soil water at full saturation was ∼2 kg. Seedlings in the pots were allowed to grow for 3 months. The average greenhouse conditions for the entire duration after treatments were as follows: air temperature 30.2°C (26.7–33.5°C) in the daytime (06:00–18:00) and 24.8°C (20.2–28.6°C) at night (18:00–06:00) and relative humidity 79.3% (55.2–100%) in the daytime and 93.6% (67.3–100%) at night.
Experimental Design
Two planting methods were used in our research, namely, pure planting, two R. pseudoacacia in one pot (RR) or two Q. acutissima in one pot (QQ), and mixed planting, one R. pseudoacacia (RQ) and one Q. acutissima (QR) in the same pot. In both planting methods, two seedlings were planted at one-third and two-thirds of the pot diameter, respectively. Each planting method had 72 well-irrigated pots of seedlings before treatments. In control group (CK), 28 pots had been well irrigated to the end of the experiment; in drought treatment (D), when treatments began, first, 44 pots were withheld from watering on July 1 for 10 days; second, 20 out of the 44 drought treatment pots underwent the first rewatering treatment (R1) on July 11 for 20 days; and then, 12 out of the 20 that were given first rewatering treatment pots underwent repeated drought treatment on July 31 for 10 days; finally, 8 out of the 12 pots underwent repeated rewatering treatment (R2) on August 10 for 20 days. Both R1 and R2 belonged to repeated drought-rewatering treatment (R). We drew a table to summarize our experimental design (Table 1). In our experiment, 10 days after drought resulted in soil water content of all pots reaching c. 20% of the field capacity (2 kg). We had seven harvest times, namely, July 1 (Day 0), July 11 (Day 10), July 21 (Day 20), July 31 (Day 30), August 10 (Day 40), August 20 (Day 50), and August 30 (Day 60). On July 1, we only harvested the control group; on July 11, we harvested both control group and drought treatment; on other harvest times, we harvested all the groups. Four pots of each planting method and treatment were randomly selected for measurement.

Table 1. Table for experimental design. Data stand for remaining pots at harvest times of each treatment before harvesting.
Growth Traits
Height (H, m), basal diameter (BD, cm), and total biomass (TBM, g) were recorded at each harvest time. Four repetitions in each treatment were harvested and separated into roots, stems, and leaves. Then, the samples were oven-dried at 80°C for 48 h and weighed. TBM was calculated as the sum of leaf biomass (LBM, g), stem biomass (SBM, g), and root biomass (RBM, g). The allometric growth was calculated as Šrutek (1997):
Leaf Physiological Traits
Fully expanded and healthy leaves of four repetitions in each treatment of both species were chosen for a net photosynthetic rate (AP, μmol m–2 s–1) measurement on each harvest time, and it was measured in situ with an infrared gas analysis system (Li-6800, Li-Cor, Lincoln, NE, United States). The measurements were conducted at 1,000 μmol m–2 s–1 photosynthetic photo flux density, which was supplied by an external light emitting diode light. Gas-exchange characteristics were measured between 9:00 and 11:00 on sunny days. During the measurement, temperature, relative humidity, and CO2 concentration inside the chamber were controlled at 28°C, 50%, and 400 ppm, respectively (Wang et al., 2020; Liu et al., 2021).
Stem Hydraulic Traits
Xylem midday water potential (Ψmd, MPa) of pure planting was measured using one of the seedlings in each plot; Ψmd of mixed planting was estimated by measuring leaf water potential in a pressure chamber (1505D-EXP, PMS Instrument Company, Albany, OR, United States); before measurement, leaves were covered with aluminum foil for 1 h to allow leaf water potential to equilibrate with xylem water potential. Four repetitions of each treatment were measured one by one as soon as they were cut from the seedlings at harvest times after AP measurement.
The stem-specific hydraulic conductivity (Ks, kg m–1 s–1 MPa–1) was measured at the same time as xylem midday water potential measurement. Four repetitions of each treatment were cut and immersed into degassed water. Subsequently, the samples were transported promptly to the laboratory with the crowns covered with black plastic bags. All leaves and bark were removed, and each segment of R. pseudoacacia was 30 cm long, while that of Q. acutissima was 20 cm long, to ensure there would never be open vessels (Liu et al., 2020). The segments were connected to a hydraulic conductivity measurement system that contained degassed, filtered 20.0 mmol L–1 KCl solution. A 30 cm hydraulic head generated hydrostatic pressure to impel water through the segments. The Ks was calculated as follows:
where L is the length of the segment (m), Qm is the mass of the water per unit of time through a segment (kg s–1), S is the average cross-sectional area for both ends of the stem (m2), and p is the intensity of the water pressure across the segment (MPa) (Liu et al., 2021).
Statistical Analysis
Data were checked for normality (Shapiro–Wilk test) and homogeneity (Levene test). Four-way analysis of variance (ANOVA) was applied to detect the main effect and interaction of species, planting methods, treatments, and harvest time on plant functional traits. One-way ANOVA was used to test the differences of plant functional traits among different treatments or harvest time within the same species. All ANOVAs were followed by Duncan’s multiple comparison tests at α = 0.05 when significant differences were found. The relationships between height and harvest time, between basal diameter and harvest time, were analyzed by linear regression and quadratic polynomial regression, respectively. The results with significance and larger adjusted coefficient of determination (R2adj) were retained. Data of height and basal diameter were log-transformed, and then, linear regression was performed to find out the allometric growth patterns. All statistical analyses were performed using the SPSS 26 software package (SPSS Inc., Chicago, IL, United States), and all figures were drawn using Origin 2019b (Originlab Co., Northampton, MA, United States).
Results
Effects of Species, Planting Methods, Treatments, and Harvest Time
The results of four-way ANOVA show that the main effects of species, planting methods, treatments, and harvest time on plant functional traits are significant (P < 0.05). In addition, there were interaction effects of the four factors with plant functional traits (Table 2). In other words, different planting methods, treatments, and harvest time had significant effects on plant hydraulic traits of the two tree species. Then, we compared the differences of plant functional traits of R. pseudoacacia and Q. acutissima in different planting methods, treatments, and harvest time.
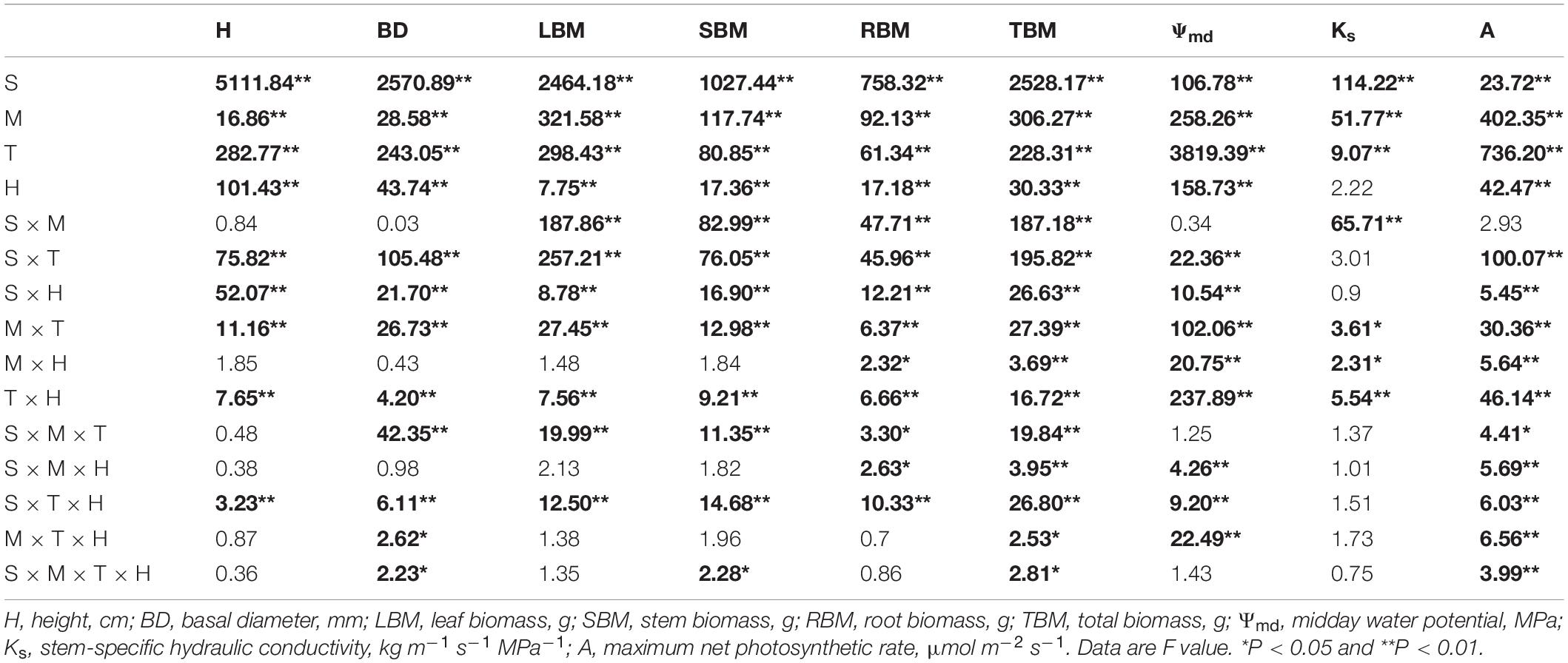
Table 2. Four-way ANOVA of plant functional traits concerning species (S), planting methods (M), treatments (T), and harvest time (H).
Plant Hydraulic Traits
Ψmd was significantly reduced by drought, 10 days after drought, Ψmd reached nearly to –10 MPa, and could recover to the control level (c. −0.01 MPa) after rewatering. For R. pseudoacacia, Ψmd of RR reached the lowest level (–10 MPa) at Day 30, while that of RQ reached the lowest level at Day 40. For Q. acutissima, Ψmd of QQ reached the lowest level at Day 40, while that of QR reached the lowest level at Day 50. In terms of the two drought-rewatering stages, the water potential decreased by the second drought, which was less than that decreased by the first drought; however, recovery of Ψmd in repeated rewatering treatment of two species was faster than that in the first rewatering treatment, and it was shown that Ψmd at Day 40 was higher than that at Day 10 (P < 0.05); it was shorter for repeated rewatering treatment to recover to the control level after rewatering than the first rewatering treatment (Figure 1).
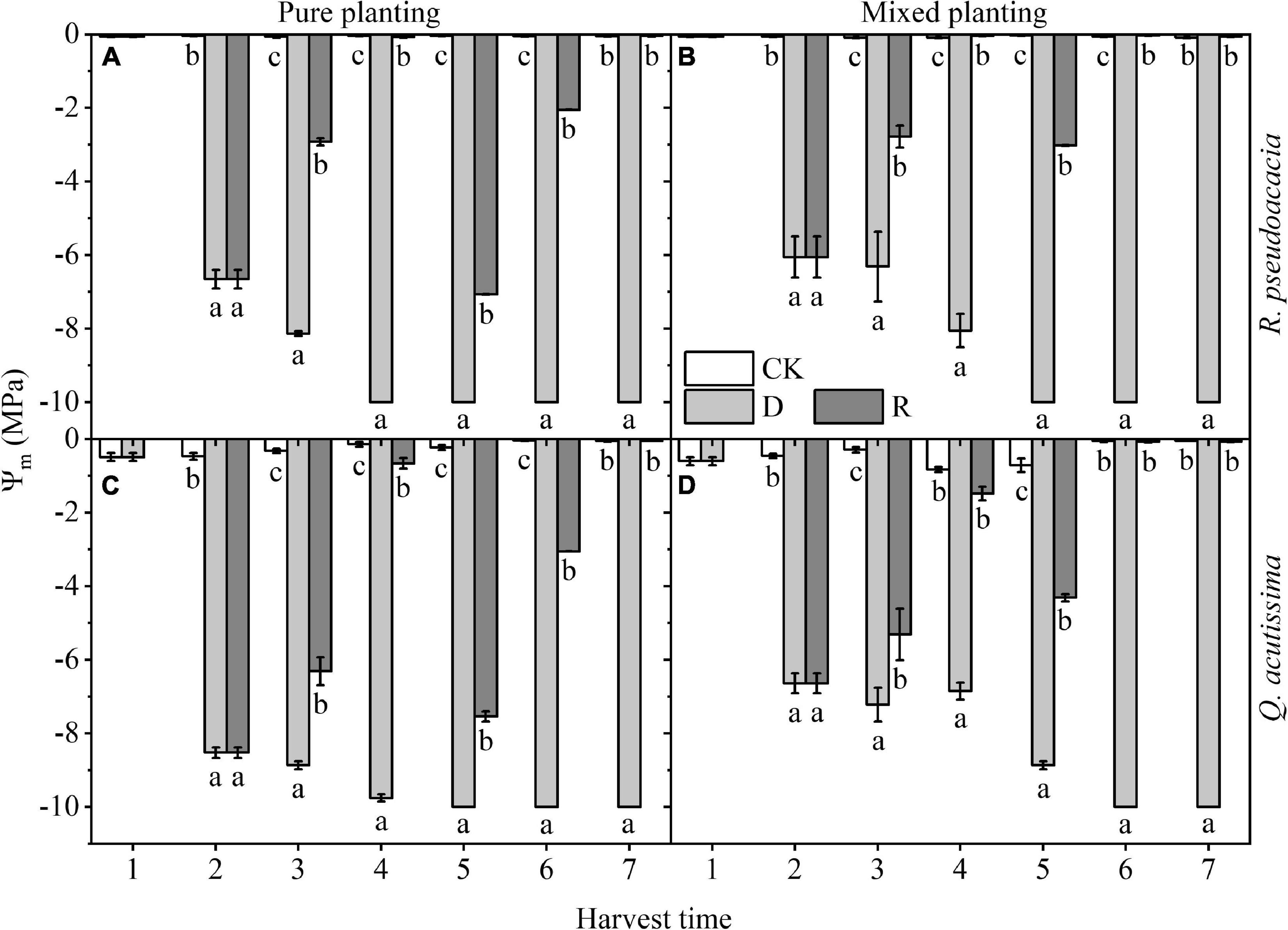
Figure 1. Changes of xylem midday water potential (Ψmd, MPa) with harvest time under different planting methods and treatments in R. pseudoacacia and Q. acutissima. White columns stand for control group (CK), light gray columns stand for drought treatment (D), and gray columns stand for rewatering treatment (R). The first drought-rewatering treatment, 2–4 harvest times; repeated drought-rewatering treatment, 5–7 harvest times. (A) R. pseudoacacia in pure planting; (B) R. pseudoacacia in mixed planting; (C) Q. acutissima in pure planting; and (D) Q. acutissima in mixed planting. One-way ANOVA followed by Duncan multiple comparison was used to test the differences among treatments; different letters stand for significant difference, P < 0.05.
Ks of RR reached 0, where plant may die, at Day 30; however, Ks of RQ reached 0 at Day 40 in R. pseudoacacia. Meanwhile, it was higher in rewatering treatment than that in the other two treatments. Compared with pure planting, mixed planting reduced Ks (P < 0.05). For Q. acutissima in QQ, the Ks reached 0 at Day 40, while that of QR never reached 0. In pure planting, Ks was significantly higher than that of the other two treatments, but there was no significant difference in mixed planting group (Figure 2).
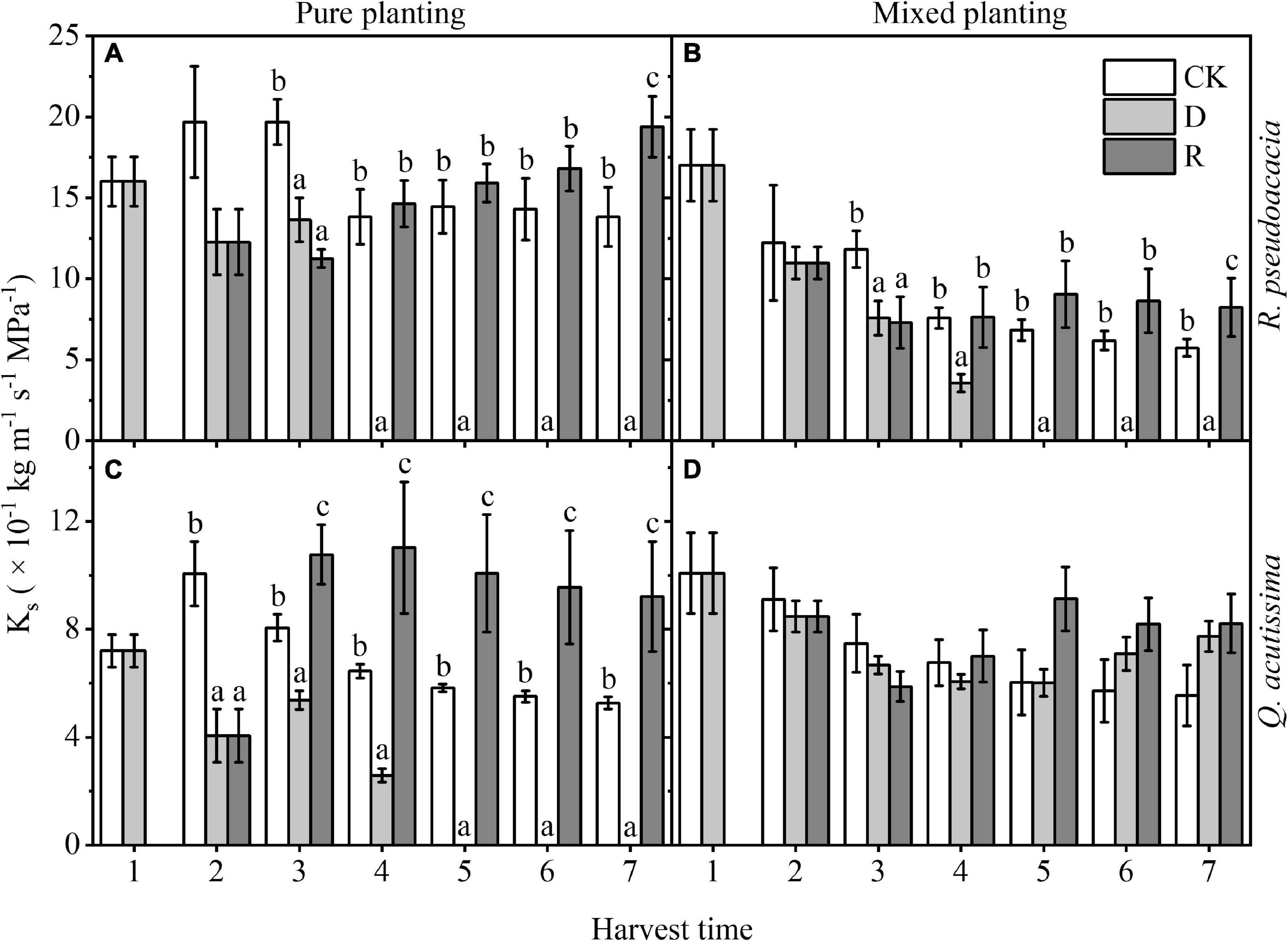
Figure 2. Changes of stem-specific hydraulic conductivity (Ks, kg m–1 s–1 MPa–1) with harvest time under different planting methods and treatments in R. pseudoacacia and Q. acutissima. White columns stand for control group (CK), light gray columns stand for drought treatment (D), and gray columns stand for rewatering treatment (R). The first drought-rewater treatment, 2–4 harvest times; repeated drought-rewatering treatment, 5–7 harvest times. (A) R. pseudoacacia in pure planting; (B) R. pseudoacacia in mixed planting; (C) Q. acutissima in pure planting; and (D) Q. acutissima in mixed planting. One-way ANOVA followed by Duncan multiple comparison was used to test the differences among treatments; different letters stand for significant difference, P < 0.05.
Leaf Photosynthetic Traits
For R. pseudoacacia, drought reduced AP at first, even less than 0, which recovered rapidly after rewatering, but still significantly less than the control group; AP of RQ was higher than that of RR within the same treatment (P < 0.05). For Q. acutissima, AP was reduced by drought, but it did not fall below 0. After rewatering, AP recovered rapidly, and AP of QQ could reach the control level, while that of QR could recover even significantly higher than the control group after rewatering (Figure 3).
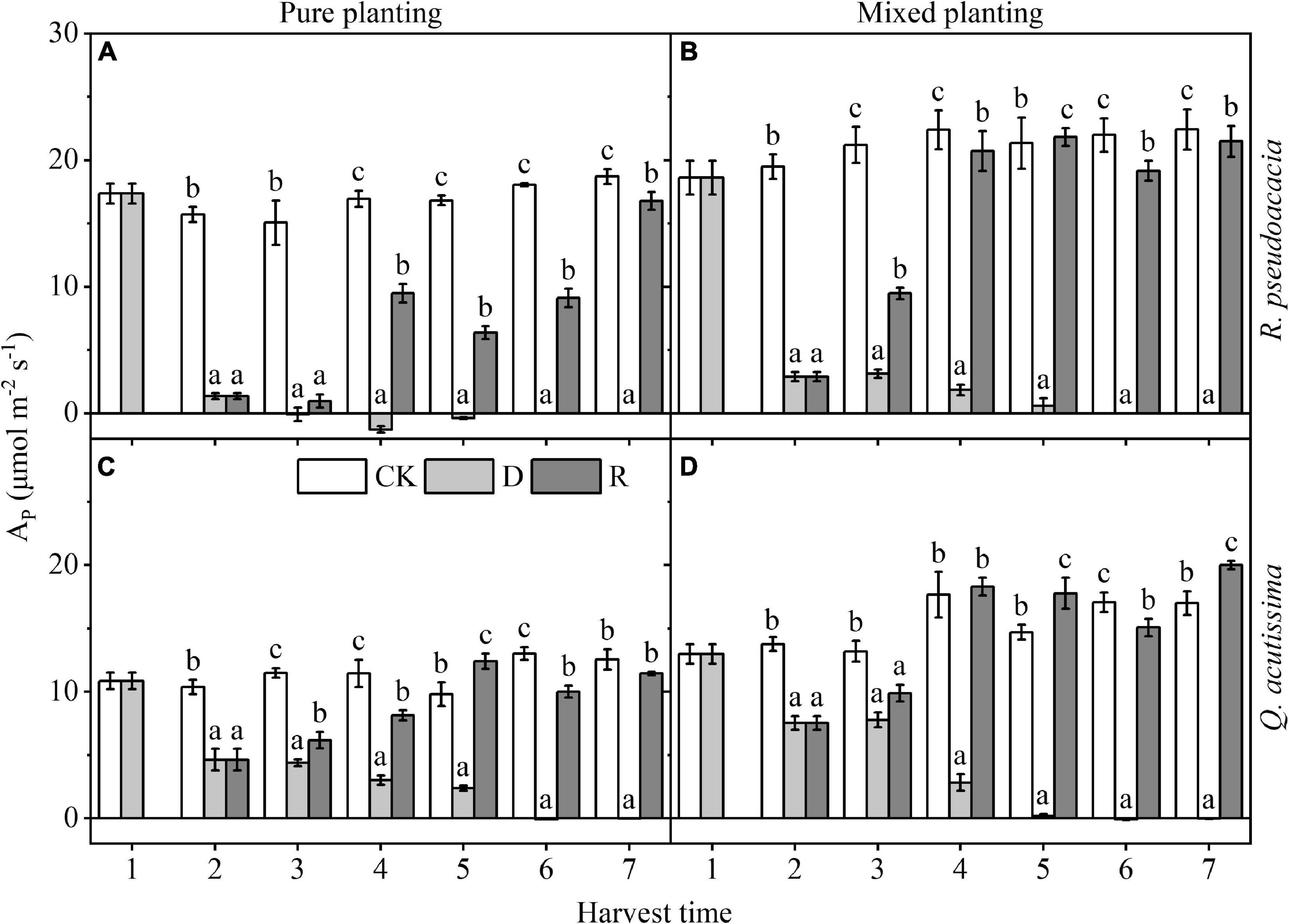
Figure 3. Changes of maximum net photosynthetic rate (AP, μmol m–2 s–1) with harvest time under different planting methods and treatments in R. pseudoacacia and Q. acutissima. White columns stand for control group (CK), light gray columns stand for drought treatment (D), and gray columns stand for rewatering treatment (R). The first drought-rewater treatment, 2–4 harvest times; repeated drought-rewatering treatment, 5–7 harvest times. (A) R. pseudoacacia in pure planting; (B) R. pseudoacacia in mixed planting; (C) Q. acutissima in pure planting; and (D) Q. acutissima in mixed planting. One-way ANOVA followed by Duncan multiple comparison was used to test the differences among treatments; different letters stand for significant difference, P < 0.05.
Plant Growth Traits
For R. pseudoacacia, first, in drought treatment, H increased first and then decreased; the highest H of RR appeared on Day 20, and that of RQ appeared at Day 30. Then, in repeated drought-rewatering treatment, H continued to increase after repeated drought-rewatering, and the growth rate was close to or even higher than the control group especially in repeated rewatering treatment. For Q. acutissima, first, the growth rate of H in QR was higher than that of QQ in control group. In drought treatment, H of QQ began to decrease after drought treatment, while that of QR first increased after drought treatment, and the highest H appeared at Day 20 and then gradually decreased. Finally, in rewatering treatment, the increase of H in the first rewatering treatment was not obvious, but H in repeated rewatering treatment increased significantly. However, the growth rates were lower than the control group (Figure 4 and Supplementary Table 1).
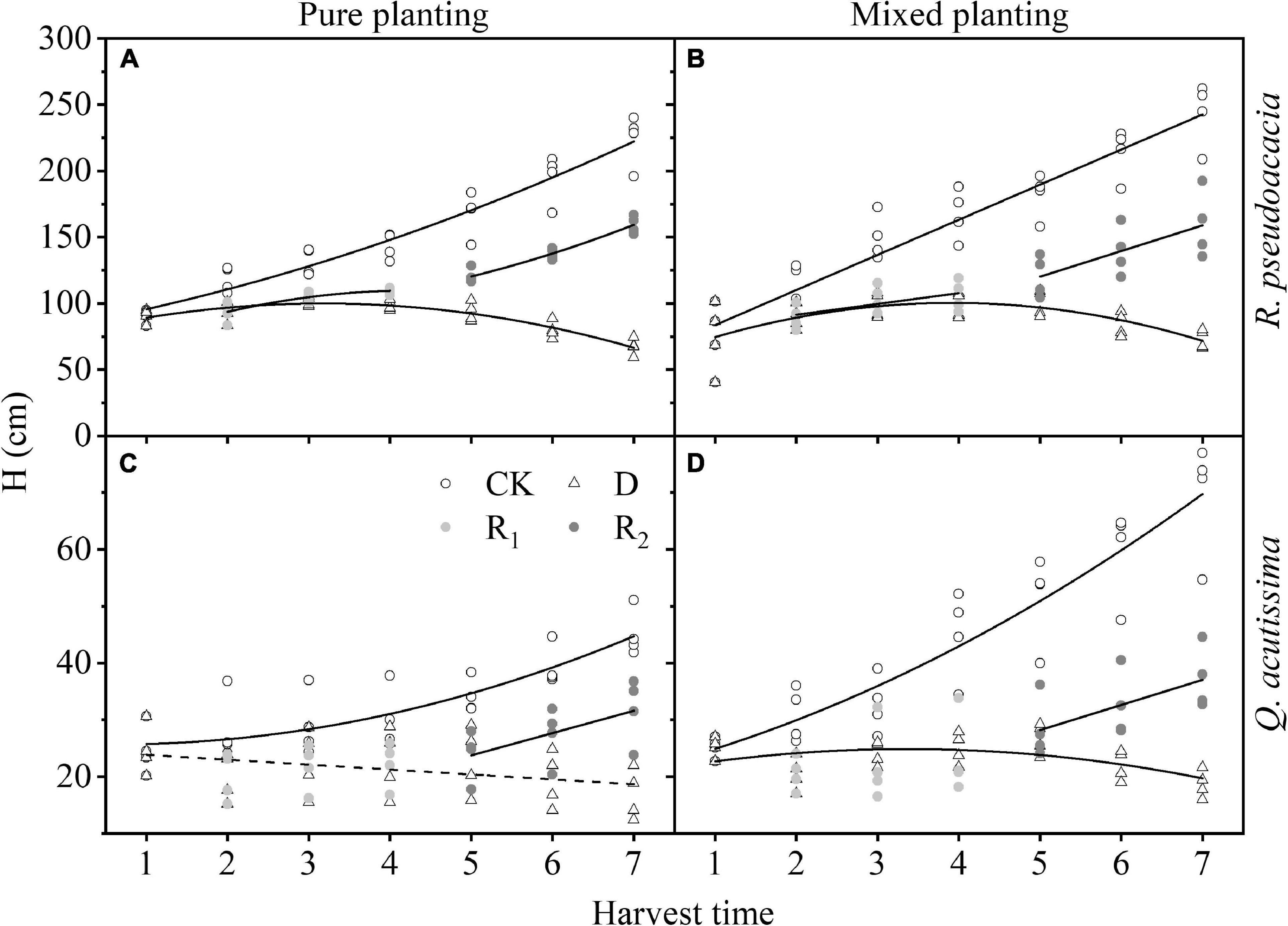
Figure 4. Changes of height (H, cm) with harvest time under different planting methods and treatments in R. pseudoacacia and Q. acutissima. Hollow circles stand for control group (CK), hollow triangles stand for drought treatment (D), light gray circles stand for rewatering treatment (R1), and gray circle stands for repeated rewatering treatment (R2). The first drought-rewater treatment, 2–4 harvest times; repeated drought-rewatering treatment, 5–7 harvest times. (A) R. pseudoacacia in pure planting; (B) R. pseudoacacia in mixed planting; (C) Q. acutissima in pure planting; (D) and Q. acutissima in mixed planting. Lines represent the regression models; solid lines, P < 0.05; dash line, P < 0.1.
For R. pseudoacacia, first in CK, BD and its growth rate were significantly increased by mixed planting. Then, in drought treatment, BD increased and then decreased, and the maximum BD of RR appeared at Day 40; however, the maximum BD of RQ appeared at Day 30. Finally, in rewatering treatment, BD of RR increased rapidly during the first rewatering treatment, and the increase rate was even higher than that of the control group. For Q. acutissima, first in drought treatment, BD decreased gradually, and the decreasing rate of QQ was fast and then slowed down, while that of QR was slow and then became faster. Then, in rewatering treatment, BD increased rapidly during the first rewatering treatment, and the increasing rate was close to that of control group, but there was no significant change in BD during repeated rewatering treatment (Figure 5 and Supplementary Table 2).
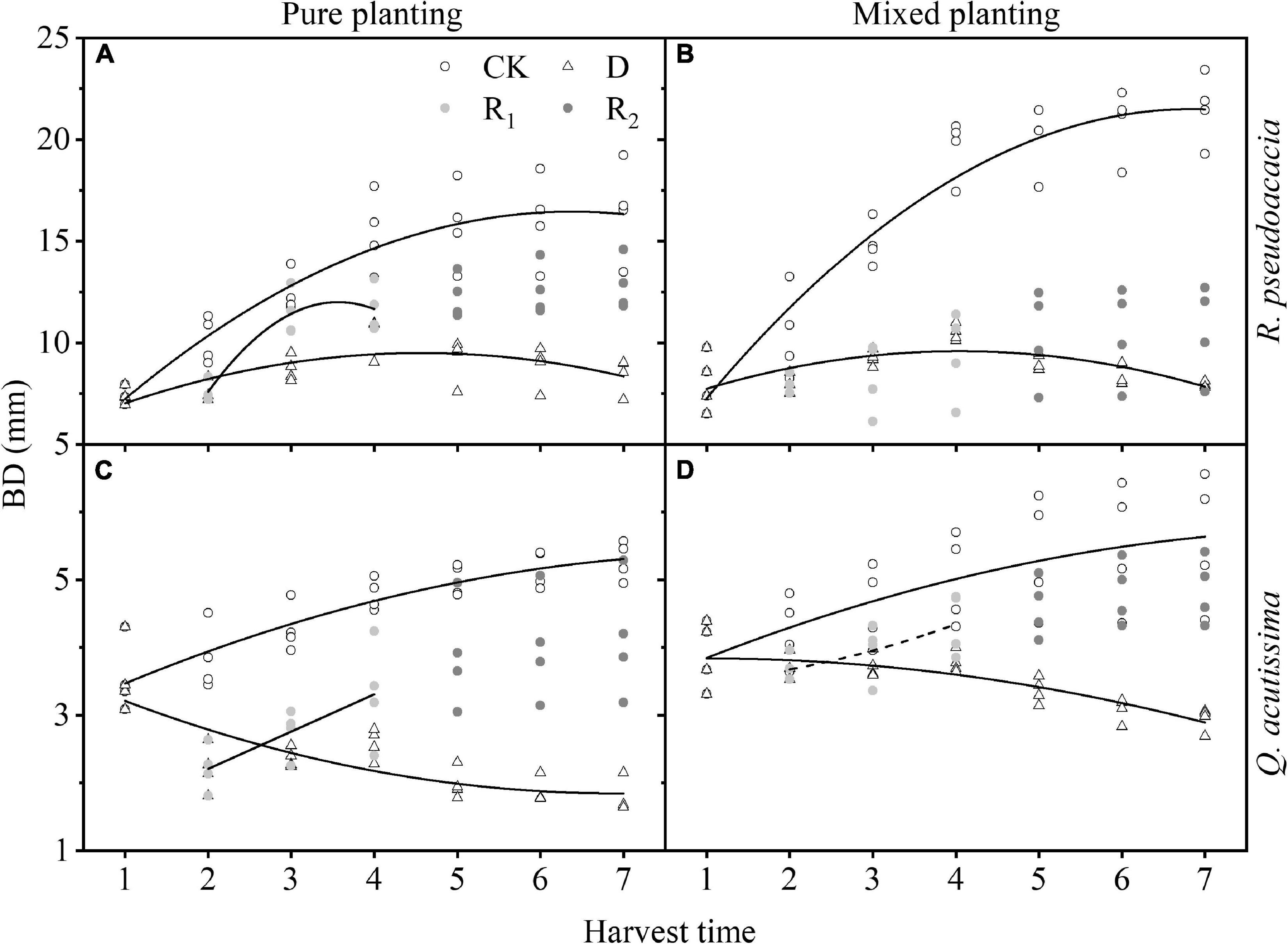
Figure 5. Changes of basal diameter (BD, cm) with harvest time under different planting methods and treatments in R. pseudoacacia and Q. acutissima. Hollow circles stand for control group (CK), hollow triangles stand for drought treatment (D), light gray circles stand for rewatering treatment (R1), and gray circle stands for repeated rewatering treatment (R2). The first drought-rewater treatment, 2–4 harvest times; repeated drought-rewatering treatment, 5–7 harvest times. (A) R. pseudoacacia in pure planting; (B) R. pseudoacacia in mixed planting; (C) Q. acutissima in pure planting; (D) and Q. acutissima in mixed planting. Lines represent the regression models; solid lines, P < 0.05; dash line, P < 0.1.
For R. pseudoacacia, the first rewatering treatment made the allometric growth pattern of RR more inclined to the thickening pattern of basal diameter than control group. However, drought made RQ more inclined to the increase of H; in other treatments, the growth pattern had no obvious trend, and the overall growth rate of H was larger than that of BD. For Q. acutissima, QR was more inclined to the higher H pattern than QQ; in other treatments, the growth pattern had no obvious trend, and the overall growth rate of H was larger than that of BD (Figure 6 and Supplementary Table 3).
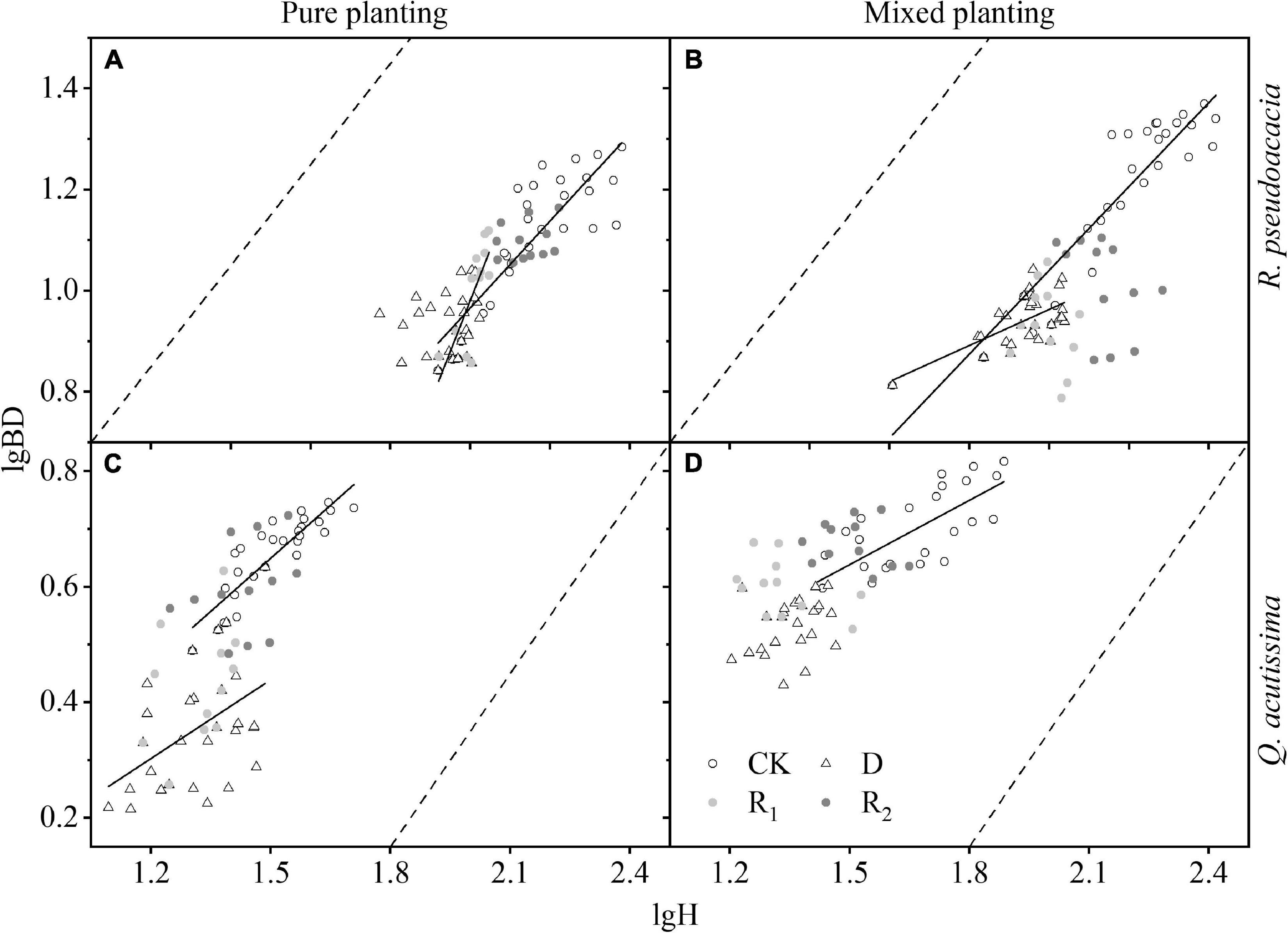
Figure 6. Allometric growth under different planting methods and treatments in R. pseudoacacia and Q. acutissima. Hollow circles stand for control group (CK), hollow triangles stand for drought treatment (D), light gray circles stand for rewatering treatment (R1), and gray circle stands for repeated rewatering treatment (R2). (A), R. pseudoacacia in pure planting; (B) R. pseudoacacia in mixed planting; (C) Q. acutissima in pure planting; (D) and Q. acutissima in mixed planting. Solid lines represent the regression models, P < 0.05; dash lines are lines with slope 1.
For R. pseudoacacia, drought made the biomass significantly lower than that of control group. No matter what kind of planting methods and treatments, compared with drought treatment, the biomass of rewatering treatment had no significant change; under the same treatment, the biomass of RQ was higher than that of RR (P < 0.05). For Q. acutissima, the biomass of drought treatment was little, but it could return to control group after rewatering; under the same treatment, the biomass of QR was higher than that of QQ (P < 0.05) (Figure 7).
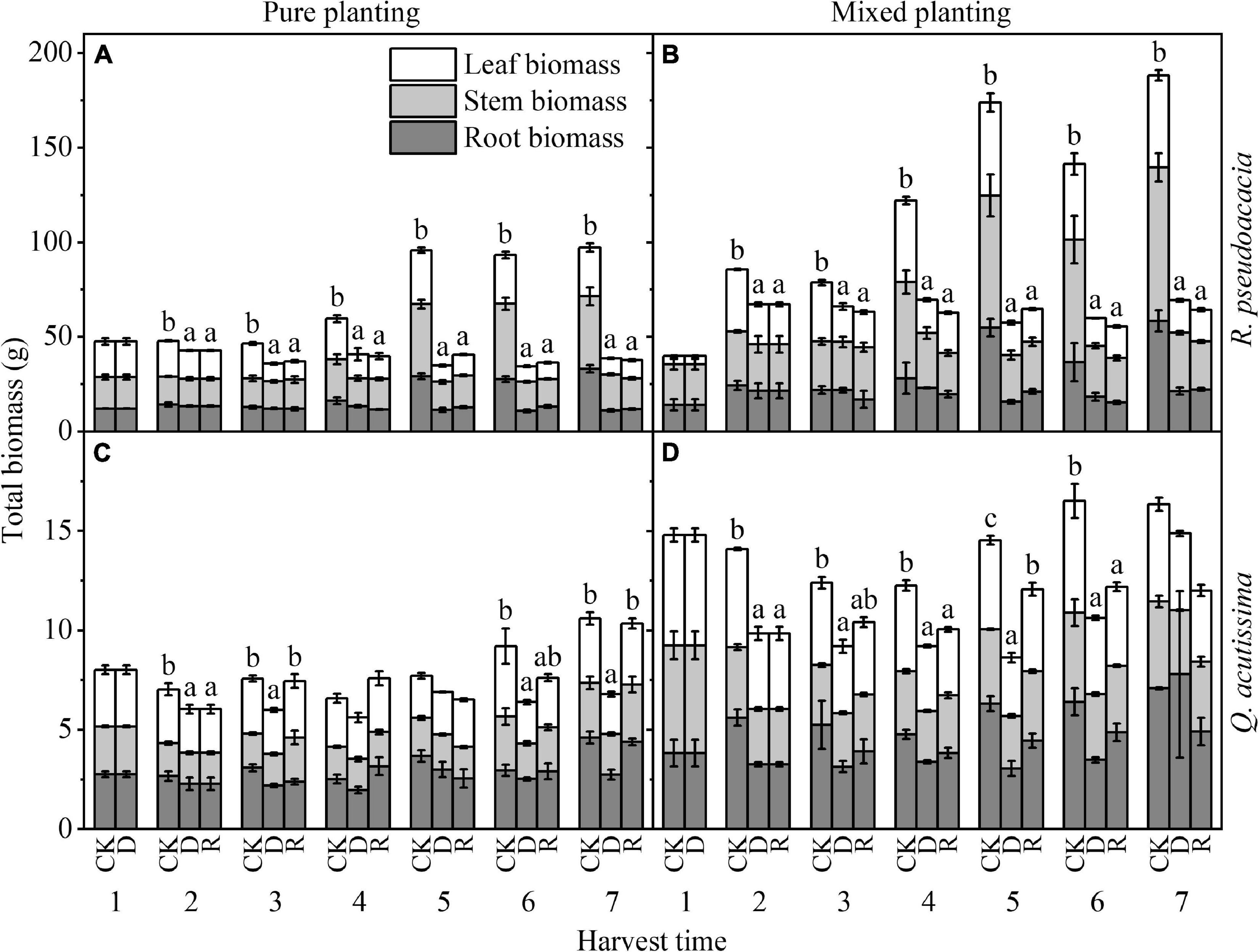
Figure 7. Changes of biomass (g) with harvest time under different planting methods and treatments in R. pseudoacacia and Q. acutissima. White columns stand for leaf biomass, light gray columns stand for stem biomass, and gray columns stand for root biomass. The first drought-rewater treatment, 2–4 harvest times; repeated drought-rewatering treatment, 5–7 harvest times. (A) R. pseudoacacia in pure planting; (B) R. pseudoacacia in mixed planting; (C) Q. acutissima in pure planting; and (D) Q. acutissima in mixed planting. One-way ANOVA followed by Duncan multiple comparison was used to test the differences among treatments; different letters stand for significant difference, P < 0.05.
Discussion
Plant Hydraulic Traits
Different water availability had significant effects on plant hydraulic traits (Figure 1 and Table 2); this may result from the different relative positions of the two tree species on the “isohydric-anisohydric” continuous spectrum, that is, R. pseudoacacia is closer to anisohydric plant, and its hydraulic traits respond quickly with the change of water availability, while Q. acutissima is closer to the isohydric plant, and its hydraulic traits respond slowly with the change of water availability (Moser et al., 2016; Li et al., 2019, 2020; Liu et al., 2020). The decrease of water potential caused by the second drought was less than that caused by the first drought, and there was no significant difference in Ψmd of the two species between repeated rewatering treatment and control group at the end of repeated rewatering treatment. It may be due to that the first drought made the two species produce stress imprint, so that they could better cope with the repeated drought and recover quickly after rewatering (Munné-Bosch et al., 2003; Jakab et al., 2005; Douma et al., 2017). For the two species, Ks of drought treatment decreased gradually, while it maintained at a high level after rewatering. Ks of rewatering treatment was almost higher than that of drought treatment, which may be due to the formation of stress imprint after drought stress, so that the two tree species could recover quickly after rewatering and provide enough water for other plant functional traits. Moreover, repeated drought-rewatering did not cause pressure fatigue according to our results. Our results do not find the negative effect of repeated drought-rewatering on plant hydraulic traits of the two tree species. On the contrary, the stress imprint induced by repeated drought-rewatering was conducive to the rapid recovery of the seedlings of the two tree species in the changing environment.
Planting methods had significant effects on plant hydraulic traits (Figures 1, 2 and Table 2). In terms of the relationship between drought and planting methods, the differences in root distribution and structure between R. pseudoacacia and Q. acutissima may overall reduce the competition for water resource and enhance the drought resistance (Wang and Zhou, 2000). In addition, mixed planting may reduce Ks to make the limited water be retained, and it is helpful for the mixed planting R. pseudoacacia and Q. acutissima to survive under drought stress. However, species in pure planting have similar functional characteristics, resulting in functional redundancy and niche overlap, which may lead to intensified water competition and increased drought stress (Lebourgeois et al., 2013; Pretzsch et al., 2013; Forrester and Pretzsch, 2015; Schwendenmann et al., 2015). The results show that species composition may have a certain effect on drought resistance, and mixed planting could weaken the ecological effect of drought.
Leaf Photosynthetic Traits
Treatments had significant effects on leaf photosynthetic traits (Table 2 and Figure 3). Combined with the changes of Ψmd (Figure 1) and Ks (Figure 2), our results illustrate that both two species formed a stress imprint after the first drought, and when facing the repeated drought, they could better respond to it (Munné-Bosch et al., 2003; Jakab et al., 2005; Douma et al., 2017). In contrast, the two tree species grew rapidly by increasing photosynthesis after rewatering, that is, they adopted the compensatory mechanism. R. pseudoacacia adopted an under compensatory mechanism or equal compensatory mechanism (Figures 3A,B), and Q. acutissima adopted an equal compensatory mechanism or even over compensatory mechanism (Figures 3C,D; Trlica and Rittenhouse, 1993; Pinkard et al., 2007; Quentin et al., 2012; Wang et al., 2020), to minimize the impact of drought.
Planting methods had significant effects on AP (Table 2). Mixed planting delayed the decrease of AP in drought treatment and increased AP in the control group and rewatering treatment. This is basically consistent with the change of Ψmd. Mixed planting prolonged the time for the two species to reach the lowest Ψmd in drought treatment (Figure 1), resulting in the fact that the decrease of AP in drought treatment was delayed. This may come from that repeated drought-rewatering, and different planting methods did not break the water-carbon coupling mechanism of plants (Li et al., 2019, 2020). AP increased as soon as water availability was high. For R. pseudoacacia, AP was significantly increased by mixed planting (Figures 3A,B). For Q. acutissima, after rewatering, AP of QQ showed an equal compensatory mechanism (Figure 3C), while that of RQ showed an over compensatory mechanism (Figure 3D). Therefore, mixed planting of R. pseudoacacia and Q. acutissima was not only conducive to drought resistance, but it was also conducive to the recovery and improvement of photosynthetic capacity after rewatering.
Plant Growth Traits
Treatments had significant effects on H and BD (Table 2). Drought significantly inhibited the growth of H (Figure 4) and BD (Figure 5) of the two tree species, which made the allometric growth pattern different (Figure 6). The allometric growth pattern of R. pseudoacacia was not changed by different planting methods, but drought made pure planting and mixed planting present the opposite pattern. In other words, RR presented the BD growth pattern (Figure 6A), while RQ presented the H growth pattern (Figure 6B). It indicated that under drought conditions, RR adopted the survival strategy in the tradeoff between growth-survival strategy. During the fierce intraspecific competition for water resources, RR could increase BD to store more water and nutrients in response to drought stress, while RQ had less competition for water resources (Schwendenmann et al., 2015), and less drought stress was better for R. pseudoacacia to get more water by accelerating growth in response to drought. Repeated drought-rewatering increased the growth rate of H of the two tree species (Figure 4) but decreased the growth rate of BD (Figure 5). In terms of plant growth traits, repeated drought did not cause pressure fatigue, while the stress imprint formed by the first drought did not make the two tree species adopt the survival strategy of BD growth mode but take the growth strategy of fast growth.
Planting methods had significant effects on H, BD, and the allometric growth pattern. Our results may be due to the difference in root structure of the two species, resulting in less overall competition for water resource (Schwendenmann et al., 2015). Therefore, under the condition of limited water resources, compared with pure planting, mixed planting reduced the competition for water resources, enhanced drought resistance, and delayed the wilting rate of plant growth (Lebourgeois et al., 2013; Pretzsch et al., 2013). However, pure planting of the two tree species had the same functional characteristics, that is, functional redundancy. The overlap of niches led to the intensification of water competition of R. pseudoacacia or Q. acutissima, increased the degree of drought, and led to the wilting of plant growth under drought stress. Although the response of mixed species to water use and drought stress is very complex (Forrester and Pretzsch, 2015; De Cáceres et al., 2021), our results show that mixed planting could effectively reduce the competition of water resources and enhance drought resistance in terms of plant growth traits.
Drought had a significant inhibitory effect on BM. After rewatering, BM of R. pseudoacacia could not return to the control level (Figures 7A,B), while that of Q. acutissima could return to the control level (Figures 7C,D). This may result from that R. pseudoacacia is close to the anisohydric plant on “isohydric-anisohydric” continuous spectrum (Moser et al., 2016; Li et al., 2019; Liu et al., 2020). Faced with drought, the biomass accumulation stopped, due to the rapid decline of plant hydraulic traits (Figures 1A,B) and the rapid decline of AP (Figures 3A,B), while BM increased slowly after rewatering due to the compensatory mechanism of photosynthesis, especially in RR. However, Q. acutissima was close to the anisohydric plant on “isohydric-anisohydric” continuous spectrum (Moser et al., 2016; Li et al., 2019; Liu et al., 2020). Faced with drought, plant hydraulic traits decreased slowly (Figures 1C,D), leading to a slow decrease in photosynthesis (Figures 3C,D). After rewatering, BM increased rapidly due to the over compensatory mechanism of photosynthesis, especially in QR. Planting methods have significant effects on BM (Table 2). Mixed planting increased the BM of the two species in each treatment (Figures 7B,D), which may result from the more reasonable allocation of resources, reducing the competition between the two species and conserving water and soil (Schwendenmann et al., 2015).
Response of R. pseudoacacia and Q. acutissima
Drought had a significant effect on the plant functional traits of the two tree species under different planting methods. It decreased Ψmd, Ks, and AP of the two tree species, and ultimately reduced H, BD, and BM. Mixed planting could reduce the competition of light, water, space, and other resources, making the limited resources more reasonable allocation, so as to weaken the inhibition of drought on the two tree species to a certain extent. After rewatering, plant functional traits were recovered. Repeated drought-rewatering did not make the two tree species produce pressure fatigue. On the contrary, it made the two tree species form stress imprint, that is, the storage of stress-resistant substances through physiological and biochemical responses. The stress imprint could better respond to drought when faced with drought stress again. After rewatering, Ψmd quickly recovered, and photosynthesis of R. pseudoacacia adopted compensatory strategies. Therefore, the BM was accumulated. Photosynthesis of Q. acutissima was overcompensated to make the BM close to or even reach the level without drought. Therefore, the combination of the two species and repeated drought-rewatering can improve the overall drought resistance ability to a certain extent.
Through the experiment of repeated drought-rewatering of R. pseudoacacia and Q. acutissima under pure and mixed planting, it is found that mixed planting of the two tree species could weaken the inhibition of drought to a certain extent; repeated drought-rewatering did not cause pressure fatigue to the two species but made them form stress imprint, which can quickly respond to repeated drought and recover quickly after rewatering. Our research suggested that the mixed planting of the two tree species could better adapt to repeated drought-rewatering under global climate change.
Data Availability Statement
The original contributions presented in the study are included in the article/Supplementary Material, further inquiries can be directed to the corresponding author/s.
Author Contributions
XL, HW, PZ, PF, ND, and RW proposed the study. XL conducted the field and laboratory measurements and analyzed the data. HW, PZ, and RW designed the experiment and secured the funding. QZ, MS, and NW helped in the laboratory measurements and data analysis. PW and KC helped in the data analysis. XL wrote the manuscript that was intensively edited by all authors. All authors contributed to the article and approved the submitted version.
Funding
This work was financially supported by the Special Foundation for National Science and Technology Basic Resources Investigation of China (2019FY202300), the Research Foundation of Qingdao Forest Ecosystem, and the Fundamental Research Funds of Shandong University.
Conflict of Interest
The authors declare that the research was conducted in the absence of any commercial or financial relationships that could be construed as a potential conflict of interest.
Publisher’s Note
All claims expressed in this article are solely those of the authors and do not necessarily represent those of their affiliated organizations, or those of the publisher, the editors and the reviewers. Any product that may be evaluated in this article, or claim that may be made by its manufacturer, is not guaranteed or endorsed by the publisher.
Acknowledgments
We would like to thank Feng Wang, Xiaohan Sun, Rong Cui, and Yang Zhang for laboratory measurements.
Supplementary Material
The Supplementary Material for this article can be found online at: https://www.frontiersin.org/articles/10.3389/fpls.2021.760510/full#supplementary-material
References
Blackman, C. J., Li, X., Choat, B., Rymer, P. D., De Kauwe, M. G., Duursma, R. A., et al. (2019). Desiccation time during drought is highly predictable across species of Eucalyptus from contrasting climates. New Phytol. 224, 632–643. doi: 10.1111/nph.16042
Bruce, T. J. A., Matthes, M. C., Napier, J. A., and Pickett, J. A. (2007). Stressful “memories” of plants: evidence and possible mechanisms. Plant Sci. 173, 603–608. doi: 10.1016/j.plantsci.2007.09.002
Corlett, R. T. (2016). The impacts of droughts in tropical forests. Trends Plant Sci. 21, 584–593. doi: 10.1016/j.tplants.2016.02.003
De Cáceres, M., Mencuccini, M., Martin-StPaul, N., Limousin, J. M., Coll, L., Poyatos, R., et al. (2021). Unravelling the effect of species mixing on water use and drought stress in mediterranean forests: a modelling approach. Agric. For. Meteorol. 296:108233.
Douma, J. C., Vermeulen, P. J., Poelman, E. H., Dicke, M., and Anten, N. P. R. (2017). When does it pay off to prime for defense? A modeling analysis. New Phytol. 216, 782–797. doi: 10.1111/nph.14771
Du, N., Tan, X., Li, Q., Liu, X., Zhang, W., Wang, R., et al. (2017). Dominance of an alien shrub Rhus typhina over a native shrub Vitex negundo var. heterophylla under variable water supply patterns. PLoS One 12:e0176491. doi: 10.1371/journal.pone.0176491
Easterling, D. R., Meehl, G. A., Parmesan, C., Changnon, S. A., Karl, T. R., and Mearns, L. O. (2000). Climate extremes: observations, modeling, and impacts. Science 289, 2068–2074. doi: 10.1126/science.289.5487.2068
Forner, A., Valladares, F., and Aranda, I. (2018). Mediterranean trees coping with severe drought: avoidance might not be safe. Environ. Exp. Bot. 155, 529–540. doi: 10.1016/j.envexpbot.2018.08.006
Forrester, D. I., and Bauhus, J. (2016). A review of processes behind diversity—productivity relationships in forests. Curr. For. Rep. 2, 45–61.
Forrester, D. I., and Pretzsch, H. (2015). Tamm review: on the strength of evidence when comparing ecosystem functions of mixtures with monocultures. For. Ecol. Manag. 356, 41–53. doi: 10.1016/j.foreco.2015.08.016
Fukushima, M., Kanzaki, M., Hara, M., Ohkubo, T., Preechapanya, P., and Choocharoen, C. (2008). Secondary forest succession after the cessation of swidden cultivation in the montane forest area in Northern Thailand. For. Ecol. Manag. 255, 1994–2006. doi: 10.1016/j.foreco.2007.12.022
Ge, C., Yu, X., Kan, M., and Qu, C. (2017). Adaption of Ulva pertusa to multiple-contamination of heavy metals and nutrients: biological mechanism of outbreak of Ulva sp. green tide. Mar. Pollut. Bull. 125, 250–253. doi: 10.1016/j.marpolbul.2017.08.025
Gimbel, K. F., Felsmann, K., Baudis, M., Puhlmann, H., Gessler, A., Bruelheide, H., et al. (2015). Drought in forest understory ecosystems – a novel rainfall reduction experiment. Biogeosciences 12, 961–975.
Högy, P., Poll, C., Marhan, S., Kandeler, E., and Fangmeier, A. J. F. C. (2013). Impacts of temperature increase and change in precipitation pattern on crop yield and yield quality of barley. Food Chem. 136, 1470–1477. doi: 10.1016/j.foodchem.2012.09.056
Jakab, G., Ton, J., Flors, V., Zimmerli, L., Métraux, J.-P., and Mauch-Mani, B. (2005). Enhancing Arabidopsis salt and drought stress tolerance by chemical priming for its abscisic acid responses. Plant Physiol. 139, 267–274. doi: 10.1104/pp.105.065698
Lebourgeois, F., Gomez, N., Pinto, P., and Mérian, P. (2013). Mixed stands reduce Abies alba tree-ring sensitivity to summer drought in the Vosges mountains, western Europe. For. Ecol. Manag. 303, 61–71. doi: 10.1016/j.foreco.2013.04.003
Li, Q., Wang, N., Liu, X., Liu, S., Wang, H., Zhang, W., et al. (2019). Growth and physiological responses to successional water deficit and recovery in four warm-temperate woody species. Physiol. Plant. 167, 645–660. doi: 10.1111/ppl.12922
Li, Q., Zhao, M., Wang, N., Liu, S., Wang, J., Zhang, W., et al. (2020). Water use strategies and drought intensity define the relative contributions of hydraulic failure and carbohydrate depletion during seedling mortality. Plant Physiol. Biochem. 153, 106–118. doi: 10.1016/j.plaphy.2020.05.023
Liu, J., and He, Z. (2020). Small DNA methylation, big player in plant abiotic stress responses and memory. Front. Plant Sci. 11:595603. doi: 10.3389/fpls.2020.595603
Liu, X., Li, Q., Wang, F., Sun, X., Wang, N., Song, H., et al. (2020). Weak tradeoff and strong segmentation among plant hydraulic traits during seasonal variation in four woody species. Front. Plant Sci. 11:585674. doi: 10.3389/fpls.2020.585674
Liu, X., Wang, N., Cui, R., Song, H., Wang, F., Sun, X., et al. (2021). Quantifying key points of hydraulic vulnerability curves from drought-rewatering experiment using differential method. Front. Plant Sci. 12:627403. doi: 10.3389/fpls.2021.627403
Luan, J., Liu, S., Wang, J., Zhu, X., and Shi, Z. (2011). Rhizospheric and heterotrophic respiration of a warm-temperate oak chronosequence in China. Soil Biol. Biochem. 43, 503–512.
Martinez-Vilalta, J., and Pinol, J. (2002). Drought-induced mortality and hydraulic architecture in pine populations of the NE Iberian Peninsula. For. Ecol. Manag. 161, 247–256. doi: 10.1016/s0378-1127(01)00495-9
Moran, E., Lauder, J., Musser, C., Stathos, A., and Shu, M. (2017). The genetics of drought tolerance in conifers. New Phytol. 216, 1034–1048.
Moser, A., Rötzer, T., Pauleit, S., and Pretzsch, H. (2016). The urban environment can modify drought stress of small-leaved lime (Tilia cordata Mill.) and black locust (Robinia pseudoacacia L.). Forests 7:71.
Munné-Bosch, S., Jubany-Marí, T., and Alegre, L. (2003). Enhanced photo- and antioxidative protection, and hydrogen peroxide accumulation in drought-stressed Cistus clusii and Cistus albidus plants. Tree Physiol. 23, 1–12. doi: 10.1093/treephys/23.1.1
Nardini, A., Battistuzzo, M., and Savi, T. (2013). Shoot desiccation and hydraulic failure in temperate woody angiosperms during an extreme summer drought. New Phytol. 200, 322–329. doi: 10.1111/nph.12288
Nyland, R. D., Bashant, A. L., Bohn, K. K., and Verostek, J. M. (2006). Interference to hardwood regeneration in northeastern North America: controlling effects of American beech, striped maple, and hobblebush. Northern J. Appl. For. 23, 122–132. doi: 10.1093/njaf/23.2.122
O’Grady, A. P., Mitchell, P. J. M., Pinkard, E. A., and Tissue, D. T. (2013). Thirsty roots and hungry leaves: unravelling the roles of carbon and water dynamics in tree mortality. New Phytol. 200, 294–297. doi: 10.1111/nph.12451
Pinkard, E. A., Michael, B., and Mohammed, C. L. (2007). Defoliation and nitrogen effects on photosynthesis and growth of Eucalyptus globulus. Tree Physiol. 27, 1053–1063. doi: 10.1093/treephys/27.7.1053
Pretzsch, H., Schütze, G., and Uhl, E. (2013). Resistance of European tree species to drought stress in mixed versus pure forests: evidence of stress release by inter-specific facilitation. Plant Biol. 15, 483–495. doi: 10.1111/j.1438-8677.2012.00670.x
Quentin, A. G., O’Grady, A. P., Beadle, C. L., Mohammed, C., and Pinkard, E. A. (2012). Interactive effects of water supply and defoliation on photosynthesis, plant water status and growth of Eucalyptus globulus Labill. Tree Physiol. 32, 958–967. doi: 10.1093/treephys/tps066
Richards, A. E., Forrester, D. I., Bauhus, J., and Scherer-Lorenzen, M. (2010). The influence of mixed tree plantations on the nutrition of individual species: a review. Tree Physiol. 30, 1192–1208. doi: 10.1093/treephys/tpq035
Ruehr, N. K., Grote, R., Mayr, S., and Arneth, A. (2019). Beyond the extreme: recovery of carbon and water relations in woody plants following heat and drought stress. Tree Physiol. 39, 1285–1299. doi: 10.1093/treephys/tpz032
Schwendenmann, L., Pendall, E., Sanchez-Bragado, R., Kunert, N., and Hölscher, D. (2015). Tree water uptake in a tropical plantation varying in tree diversity: interspecific differences, seasonal shifts and complementarity. Ecohydrology 8, 1–12.
Šrutek, M. (1997). Growth responses of Urtica dioica L. to different water table depth. Plant Ecol. 130, 163–169.
Tomasella, M., Nardini, A., Hesse, B. D., Machlet, A., Matyssek, R., and Häberle, K.-H. (2019). Close to the edge: effects of repeated severe drought on stem hydraulics and non-structural carbohydrates in European beech saplings. Tree Physiol. 39, 717–728. doi: 10.1093/treephys/tpy142
Umebayashi, T., Sperry, J. S., Smith, D. D., and Love, D. M. (2019). ‘Pressure fatigue’: the influence of sap pressure cycles on cavitation vulnerability in Acer negundo. Tree Physiol. 39:740746. doi: 10.1093/treephys/tpy148
Walter, J., Beierkuhnlein, C., Jentsch, A., and Kreyling, J. (2013). Ecological stress memory and cross stress tolerance in plants in the face of climate extremes. Environ. Exp. Bot. 94, 3–8. doi: 10.1016/j.envexpbot.2012.02.009
Wang, N., Li, Q., Liu, X., Yi, S., Zhao, M., Sun, X., et al. (2021). Plant size plays an important role in plant responses to low water availability and defoliation in two woody Leguminosae species. Front. Plant Sci. 12:643143. doi: 10.3389/fpls.2021.643143
Wang, N., Zhao, M., Li, Q., Liu, X., Song, H., Peng, X., et al. (2020). Effects of defoliation modalities on plant growth, leaf traits, and carbohydrate allocation in Amorpha fruticosa L. and Robinia pseudoacacia L. seedlings. Ann. For. Sci. 77:53.
Wang, R., and Zhou, G. (2000). The Vegetation of Shandong Province. Jinan: Shandong Science and Technology.
Keywords: compensatory mechanism, drought resistance ability, mixed planting, plant hydraulic traits, stress imprint, warm temperate zone
Citation: Liu X, Zhang Q, Song M, Wang N, Fan P, Wu P, Cui K, Zheng P, Du N, Wang H and Wang R (2021) Physiological Responses of Robinia pseudoacacia and Quercus acutissima Seedlings to Repeated Drought-Rewatering Under Different Planting Methods. Front. Plant Sci. 12:760510. doi: 10.3389/fpls.2021.760510
Received: 18 August 2021; Accepted: 08 November 2021;
Published: 06 December 2021.
Edited by:
Mario A. Pagnotta, University of Tuscia, ItalyReviewed by:
Benjamin D. Hesse, Technical University of Munich, GermanyQiang Li, Chinese Academy of Geological Sciences, China
Copyright © 2021 Liu, Zhang, Song, Wang, Fan, Wu, Cui, Zheng, Du, Wang and Wang. This is an open-access article distributed under the terms of the Creative Commons Attribution License (CC BY). The use, distribution or reproduction in other forums is permitted, provided the original author(s) and the copyright owner(s) are credited and that the original publication in this journal is cited, in accordance with accepted academic practice. No use, distribution or reproduction is permitted which does not comply with these terms.
*Correspondence: Hui Wang, wanghui1227@sdu.edu.cn