- 1Departamento de Ensino e Pesquisa, Fundação de Medicina Tropical Dr. Heitor Vieira Dourado, Manaus, Amazonas, Brazil
- 2Grupo de Pesquisas em Metabolômica e Espectrometria de Massas, Universidade do Estado do Amazonas, Manaus, Amazonas, Brazil
- 3Instituto de Ciências Biológicas, Universidade Federal do Amazonas, Manaus, Amazonas, Brazil
- 4Departamento de Pós-Graduação, Universidade Nilton Lins, Manaus, Amazonas, Brazil
- 5Curso de Medicina, Universidade Federal de Roraima, Boa Vista, Roraima, Brazil
- 6Departamento de Ensino e Pesquisa, Fundação Alfredo da Matta, Manaus, Amazonas, Brazil
- 7Instituto de Ciências Exatas e Tecnologia, Universidade Federal do Amazonas, Itacoatiara, Amazonas, Brazil
- 8Laboratório de Herpetologia, Campus Floresta, Universidade Federal do Acre, Cruzeiro do Sul, Acre, Brazil
Phyllomedusa bicolor (Phyllomedusidae), popularly known as the kambô in Brazil, is a tree frog that is widely distributed in South American countries and is known for producing a skin secretion that is rich in bioactive peptides, which are often used in indigenous rituals. The biological effects of the skin secretion were observed in the first studies with indigenous communities. Over the last six decades, researchers have been studying the chemical composition in detail, as well as the potential pharmacological applications of its constituents. For this reason, indigenous communities and health agents fear the misuse of the kambô, or the inappropriate use of the species, which can result in health complications or even death of users. This article seeks to provide a transdisciplinary review that integrates knowledge regarding the biology of P. bicolor, ethnoknowledge about the ritual of the kambô, and the chemistry and pharmacology of the skin secretion of this species, in addition to medical aspects of the indiscriminate use of the kambô. Furthermore, this review seeks to shed light on perspectives on the future of research related to the kambô.
1 Natural history of Phyllomedusa bicolor
1.1 The species: General aspects
Phyllomedusa bicolor is popularly known in English as the bicolored treefrog, giant monkey frog, giant leaf frog, two-colored leaf frog, waxy-monkey treefrog, and kambô (and also kampô) in South American countries (Frost, 2021). The word kambô commonly has two meanings in the literature. It can be used for the treefrog P. bicolor and also for the shamanic indigenous ritual (Caramaschi and Cruz, 2002; Frost, 2021). Its skin secretion has been used for centuries by the natives of the Western Amazon in shamanic healing and purification rituals known as kambô (Erspamer-Falconieri et al., 1970) or kapum or the “vacina do sapo” (toad vaccine in English). However, this practice has expanded to urban centers as an alternative to conventional medicine (Silva et al., 2019). It is a pharmacologically important taxon because it is considered a rich source of biologically active peptides, many of which have already been identified or characterized, although it is estimated that there are numerous compounds yet to be discovered (Thompson and Williams, 2022).
Phyllomedusa bicolor was taxonomically described from Suriname by Boddaert (1772) as Rana bicolor and, later, Wagler (1830) proposed the genus Phyllomedusa to house this species. Therefore, P. bicolor is the type species of its genus. The name Phyllomedusa comes from the Greek “phyllo” (leaf or foliage) and “medousa” (queen or protector), therefore meaning “queen of the foliage” or “guardian of the foliage” (Caramaschi and Cruz, 2002). Its specific name “bicolor” refers to the two main colors of this frog (Boddaert, 1772): dorsum dark green and belly white to yellow-white or cream. Monophyly of the genus Phyllomedusa is strongly supported by Duellman et al. (2016), and the genus currently contains 16 taxa (Frost, 2021), with Phyllomedusa chaparroi being the most recently described (Castroviejo-Fisher et al., 2017). Phyllomedusa bicolor and Phyllomedusa vaillantii are frequently recovered as sister species that share the presence of osteoderms in the skin dorsum (Ruibal and Shoemaker, 1984; Faivovich et al., 2005; Wiens et al., 2006; Moen and Wiens, 2009; Faivovich et al., 2010; Duellman et al., 2016).
1.2. Seasonality, reproductive biology, and life cycle
Reproduction occurs mainly during the rainy season, and the length of reproduction varies geographically throughout the species’ distribution, and also because of increasingly frequent extreme climatic events such as El Niño and La Niña (Lima et al., 2012; Rocha et al., 2021). In the Central Amazon, breeding occurs mostly from November to May (Lima et al., 2012), during which constant and heavy rainfall creates suitable lentic environments such as ponds for development of the tadpoles (Venâncio and Melo-Sampaio, 2010; Pinto et al., 2013). This prolonged reproductive season of the species permits male territoriality, asynchronous arrival of females to breeding sites, as well as male-male competition for females with physical combat that includes body displacement of amplectant pairs (Figure 1C; Souza 2009; Venâncio and Melo-Sampaio, 2010; Silva et al., 2020). Reproduction promotes vocalization and movement through the vegetation, possibly increasing detectability of adult individuals, mostly males, by humans.
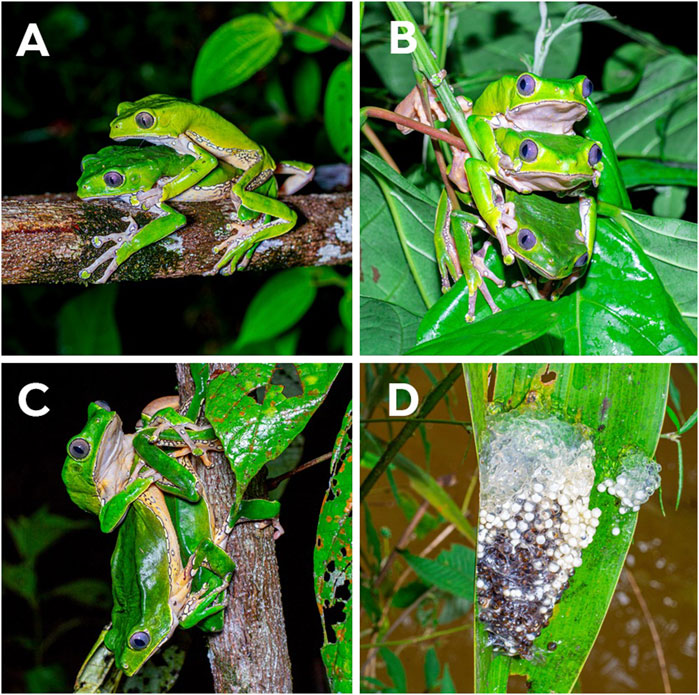
FIGURE 1. Reproductive behavior of Phyllomedusa bicolor in Amapá State, Brazil. (A) An axillary amplexus near riparian vegetation. (B) Multiple amplexus of two males and one female. (C) Physical dispute between two males for a female. (D) The spawn on a totally open leaf above a temporary pond of lentic water where tadpoles develop until metamorphosis. Photos: Wirley Almeida.
Males call alone or in small congregations, on vegetation (branches of bushes and trees), close to streams, temporary or permanent ponds on the inside and edges of the forest, between one and 10 m above the ground (Souza, 2009). The vocalization usually begins at dusk and lasts until near dawn, at which time the males leave the calling sites and move to their upper diurnal shelters (Souza, 2009). The courtship of the species begins at night in upland forests with an active calling male from a high tree until the arrival of the female; after which the pair descends to lower arboreal strata (Venâncio and Melo-Sampaio, 2010; Silva et al., 2020). The advertisement call of P. bicolor males is short, loud, sparse, and low-pitched when compared to most treefrogs (Rodriguez and Duellman, 1994; Lima et al., 2012). As the female comes closer, the male climbs on her dorsum to form the axillary or cephalic amplexus (Figure 1A) in which the pair stays for some minutes before the female moves to a suitable oviposition site (Venâncio and Melo-Sampaio, 2010). The oviposition site is chosen on the vegetation above lentic water surfaces (average 70 cm) or near a slow-flowing water stream (Figure 1D) where the mating pair fold leaves (usually tree leaves) to form a chamber to protect the spawn, which is probably a strategy against predation and dehydration (Venâncio and Melo-Sampaio, 2010; Lima et al., 2012; Pinto et al., 2013). However, it is known that the predation rate upon P. bicolor spawns can reach up to 61% (Neckel-Silveira and Washlewski, 2004).
The spawn of P. bicolor is one of the largest among treefrogs in the Amazon, and corresponds to a gelatinous mass with relatively large (average 2.6 mm) eggs that range between 241 and 1,722 units (Neckel-Oliveira and Wachlevski, 2004; Venâncio and Melo-Sampaio, 2010; Silva et al., 2020) (Figure 2B). After the oviposition, the lecithotrophic eggs develop into tadpoles within the leaf chambers (Pinto et al., 2013). After approximately 2 weeks, tadpoles with external gills emerge from the eggs (Figure 2A) and fall on the water surface under the oviposition site to continue their development with exotrophic feeding until metamorphosis (Figure 2C) (Venâncio and Melo-Sampaio, 2010; Pinto et al., 2013).
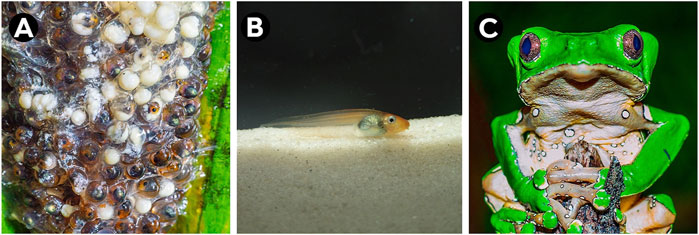
FIGURE 2. Different ontogenetic stages of Phyllomedusa bicolor: (A) eggs, (B) larvae (tadpole) and (C) adult. Photos: A and (C) Wirley Almeida; (B) Domingos Rodrigues.
1.3 Morphology and behavior
Phyllomedusa bicolor are relatively large-sized tree frogs: adult males measure 91–118 mm from snout to vent and females 106–119 mm (Souza, 2009; Lima et al., 2012; Duellman et al., 2016). Adults have a dark green dorsum and a white to yellow-white or cream belly (Lima et al., 2012). There are white spots with dark outlines sparsely distributed on the lower lips, chest, and front legs, though more densely distributed on the flanks and hind legs, dark gray iris, a prominent gland that extends from behind the eyes over the tympanum, and vomerine teeth (Caramaschi and Cruz, 2002; Lima et al., 2012). Fingers are transparent brown with large green adhesive discs although little or no webbing on the feet and none on hands (Faivovich et al., 2010; Duellman et al., 2016). The individuals usually exhibit an elegant slow walk over branches and leaves despite being able to jump (Caramaschi and Cruz, 2002). The species of Phyllomedusiae are regarded as photogenic and charismatic animals, and are frequently called “poster frogs” (Faivovich et al., 2010). Probably because of its large size, wide distribution, and its use in shamanic rituals, P. bicolor frequently figures as a symbol of the Amazonian fauna (Bernarde, 2012). Phyllomedusiae actively produce skin secretions and frequently spread them throughout the body with the hind and front legs (Blaylock et al., 1976).
Most of the characteristics of the tadpoles are similar to other species of Phyllomedusa, i.e., they are exotrophic, lentic, and suspension-raspers (Altig and McDiarmid, 1999; Pinto et al., 2013). The tadpole reaches a total length of up to 50 mm (Souza, 2009). The coloration in life is orange on the dorsum and anterior part of the body, with a silver belly, a pale orange color on the tail musculature and cord, a translucent orange color on the fins, and a silver iris (Figure 2A) (Pinto et al., 2013). This species’ tadpole can be easily distinguished from others (except for those of Phyllomedusa vaillantii) due to their unique cord that is present at all stages, and which corroborates the phylogenetic proximity of these two species (Faivovich et al., 2010). Phyllomedusa bicolor tadpoles show conspicuous colorations in the dorsal area while ventrally they are considered inconspicuous. This is probably for targeting aerial or terrestrial predators while remaining cryptic for underwater animals (Thibaudeau and Altig, 2012; Pinto and Amézquita, 2022). They also prefer to remain on the surface of the water, displaying their coloration: bigger tadpoles present this behavior whereas smaller individuals tend to be distributed along the water column, thus reinforcing the antipredatory strategy (Pinto and Amézquita, 2022). There is evidence of the toxicity of P. bicolor tadpole for vertebrates; it has been shown to significantly affect mice after ingestion, even though Odonata naiads (damselfly larvae) were able to prey on them without difficulties. Therefore, it is likely that the same peptides secreted as adults are present during larval growth (Delfino et al., 1998; Pinto and Amézquita, 2022).
1.4 Geographic distribution and genetic variability
This species has a wide distribution when compared to most amphibian species, since it is found in tropical rainforests in the Amazon Basin in Bolivia, Peru, Colombia, Venezuela, Guyana, French Guiana, Suriname, and possibly Ecuador (Ouboter and Jairam, 2012; Frost, 2021) (Figure 3). However, most of its distributional range lies within Brazil, where the species can be found in rainforests and even in savannah-like environments of all its northern states (Mota et al., 2020; Frost, 2021) (Figure 3).
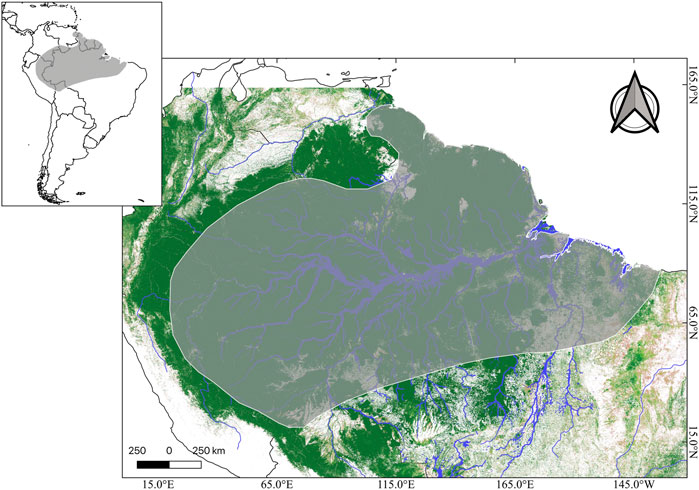
FIGURE 3. Distributional range of Phyllomedusa bicolor (dark gray) in the context of South America (upper panel). Tree coverage is shown in dark green, while lighter green and beige represents less tree coverage. Major Amazonian rivers are depicted in blue. Credits: Shapefile of distributional range from IUCN, and tree cover raster (Landsat 8) from Global Land Analyses & Discovery.
Despite its large body size, wide distribution and medical importance, many of its few published DNA sequences are not assigned to any specific geographic location, which is due to them being obtained from pet trade specimens (Mota et al., 2020). Surprisingly, even phylogenetic studies on the family lack samples of P. bicolor (Wiens et al., 2005; Gomez-Mestre et al., 2008). One study, on the spatial distribution of its genetic variability using both mitochondrial and nuclear markers, detected two well-supported monophyletic groups that diverged approximately 5.16 million years ago in two geographic mega-regions, namely the Eastern and Western Amazon; the former consisting of three highly-structured population groups distributed in the Guiana and Brazilian Shields (Mota et al., 2020). Given that this study was restricted to Brazilian locations and lacked samples from Suriname, the species’ type locality, it is likely that the nominal species is composed of at least two, or possibly more, cryptic species (Mota et al., 2020; Frost, 2021).
1.5 Ecological relationships: Diet, predation and parasitism
There appears to be no records of P. bicolor prey under natural conditions. Other phyllomedusids show generalist diets and are regarded as opportunistic sit-and-wait predators, consuming mainly arachnids (spiders and mites), coleopterans, and lepidopteran larvae (Bertoluci, 2002; Lima et al., 2012).
The risk of predation of anurans was reported to be smaller in species with chemical defense or large-sized bodies (Shine, 1979), which is the case of the adult P. bicolor (Venâncio and Melo-Sampaio, 2010). There are no records of adult P. bicolor being preyed on or found among the stomach contents of any taxa that usually preys on treefrogs, such as snakes, birds, bats, or other mammals. This may be due to its large body size and cryptic coloration that helps camouflage it. However, its skin secretion may play a strong role in predation avoidance because it is unpleasant to predators, possibly causing regurgitation, modifying cardiac function, or producing catalepsy (Sazima, 1974; Negri et al., 1992).
Neckel-Oliveira and Wachlevski (2004) observed the spawns of P. bicolor being predated by flies, beetles, and mammals, and also reported that spawn predation is common in Phyllomedusa. Snakes are known to predate on anuran eggs and may be responsible for some missing clutches (Warkentin, 1995; Martins and Oliveira, 1998; Neckel-Oliveira and Wachlevski, 2004). Neckel-Oliveira and Wachlevski (2004) observed marks of predation of clutches by monkeys. Phyllomedusa bicolor tadpoles were recorded among the stomach contents of the aquatic frog Pipa arrabali, and this species was also observed actively preying on tadpoles as soon as they dropped from the arboreal nest to the pond (Buchacher, 1993).
Only nine species of nematodes have been reported as parasites of Phyllomedusidae, whereas the genus Neocosmocercella was only observed in phyllomedusids, and is likely specific to this family (Campião et al., 2014; Santos et al., 2017). The parasite Neocosmocercella fisherae was the only species found in the large intestine of P. bicolor specimens (Santos et al., 2017). There was also another report of a filarial parasite located near the lungs of P. bicolor, though without further identification (Chai, 2015). Besides the predation of the eggs by flies, there are no records of myiasis for P. bicolor. Observation of endoparasites, both intestinal and pulmonary, are more common in P. bicolor when compared to ectoparasites, which may reflect the protection conferred by its epidermal secretions against external parasitism.
1.6 Conservation and welfare
Amazonian anurans with wide distribution, such as P. bicolor, usually present a cryptic taxonomic diversity, which means that species considered not to be under threat may have an inaccurate, and often underestimated conservation status (Gehara et al., 2014; Mota et al., 2020). Despite the wide currently known geographical distribution of P. bicolor, the species is not abundant in faunal inventories and ecological studies, and is regarded as a less abundant taxon in anuran assemblages (Menin et al., 2008; Jairam, 2019). In spite of this, P. bicolor is not classed as threatened in the Convention on International Trade in Endangered Species of Wild Fauna and Flora (CITES) appendix; an international arrangement that aims to ensure that international trade of wild animals and plants does not threaten the survival of species (https://cites.org/eng). According to the International Union for Conservation of Nature’s (IUCN) Red List of Threatened Species, which indicates the species’ global conservation status (and urgently needs updating), P. bicolor is listed as a species of least concern because of its large distributional range and occurrence in protected areas (IUCN, 2021).
The aforementioned conservation status of P. bicolor might be underestimated due to the following causes: 1) Specific habitat requirements, since it is highly intolerant of anthropized habitats, and is usually found in extensive and well-preserved forests (Tsuji-Nishikido and Menin, 2011; Lima et al., 2012). Their reproductive behavior is elaborate, requiring suitable terrestrial and aquatic (especially lentic) environments for the completion of its reproductive cycle; 2) Possible cryptic diversity with species occurring outside protected areas and/or with small distributional ranges (Mota et al., 2020); 3) Unknown populational effects due to manipulation of individuals for venom extraction, which sometimes involves the use of electric shocks (Long et al., 2017; Liu et al., 2020; Chen et al., 2021), and restraints (Den Brave et al., 2014). In addition, it is unknown how the manipulation and/or removal of adult individuals from nature by humans affects individual behavior, including parental care and, consequently, offspring survival in P. bicolor; 4) Underreporting of trafficked individuals in the pet trade. As any other illegal substance, there is a high risk of identification error, especially due to the morphological similarity among Phyllomedusa species, or possible adulteration of the lyophilized skin extracts (Lima and Labate, 2007; Silva et al., 2019). For example, the metropolitan region of Manaus is known to contain at least four species of Phyllomedusa occurring in sympatry (same location) and syntopy (similar microhabitat) (Lima et al., 2012), which may lead to the misidentification of species and extraction of skin secretions of non-targeted species.
Phyllomedusa bicolor is mostly distributed in Brazil, a country with restrictive legislation against the use and advertisement of kambô for medical purposes without proper clarification of its risks and the lack of evidence for medical treatments (ANVISA, 2004). In addition, there are strong restrictions in regards access to wild animals and their products, even by scientific researchers (IBAMA/ICMBIO). Despite this, the main centers where the secretion is applied are located outside the distribution area of the species (Iakp, 2022; Thompson and Williams, 2022), and there is no information on how individuals and secretions reach these sites since the exact origin and taxonomic accuracy of the sampled individuals is rarely mentioned at the application centers. In 2013, the Regional Superintendency of the Federal Police of the Brazilian State of Acre developed a technique to chemically identify the “vacina do sapo” (toad vaccine) and inhibit trafficking and smuggling, but there is no information regarding the current application of such a technique.
2 The poisonous secretion of Phyllomedusa bicolor
2.1 Skin structure of Phyllomedusa bicolor
Amphibian skin is a thin, permeable and flexible integument that is highly vascular and is responsible for water absorption, respiration, thermoregulation, osmoregulation and physical and chemical defense against predators and desiccation (Wager, 1986; Clarke, 1997; Larsen and Ramløv, 2013). It consists of the layers of the epidermis and dermis, and there are countless glands present on the entire epidermal surface of frogs (Figure 4) that constantly produce and secrete small amounts of poison (Jared et al., 2009; Govender et al., 2012). Among amphibians, arboreal frogs, such as treefrogs, spend most of their life in humid environments, where there is greater exposure of individuals to infectious diseases (Liu et al., 2021). The anuran’s skin adapts to different environmental pressures of the species’ habitat, thus resulting in great morphofunctional diversity (Toledo and Jared, 1993; Barra and Simmaco, 1995). Their secretions play an essential role in aiding cutaneous respiration, reproduction, defense against predators, as well as defense against desiccation and proliferation of microorganisms (Toledo and Jared, 1995).
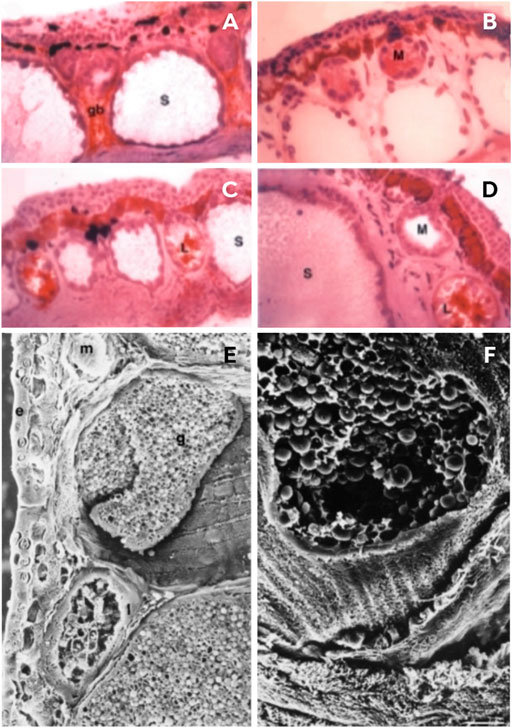
FIGURE 4. Skin structure of Phyllomedusa bicolor. (A–D) Sections of paraffin-embedded material at different stages of development; (E–F) Cryostat sections immunolabeled with I-13 antibodies by phase contrast. (A) A tadpole without a developed lipid gland, but showing a rudimentary lipid gland between two serous glands. (B–C) A juvenile immediately after metamorphosis; the serous glands are not so voluminous and not so deep in the dermis. (D) Adult stage with mature serous glands. (E–F) Sections of adult skin structure via scanning electron micrography. (E) A dorsolateral view indicating the glands in the dermis: (E) epidermis, (G) serous gland, (L) lipid gland, (m) mucous gland. (F) Serous glands broken and releasing spherical secretion granules. Photos: Adapted from Lacombe et al. (2000) (License number 5337320779774, Elsevier, Amsterdam, Netherlands).
Lacombe et al. (2000) conducted an ultrastructural study that characterized the skin of the species of Phyllomedusa according to size and activity, and presented a profile of skin glands composed of three types of cutaneous glands: lipid, mucous, and serous (Figure 4). These glands are located deep in the skin and subcutaneous connective tissue, although the serous glands are larger and lie deeper than the others (Lacombe et al., 2000). Lipid glands are mostly located in the dorsal and dorsolateral regions and are responsible for impermeabilization of the skin, and prevent water loss through desiccation (Lacombe et al., 2000; Castanho and De Luca, 2001). Mucous glands are mainly distributed in the ventral region and are practically absent from the back. These produce mucus to assist the cutaneous physiological functions such as respiration, reproduction, defense and thermoregulation (Toledo and Jared, 1995; Lacombe et al., 2000). Serous glands are the largest and are distributed all over the body; however, they are prominent in the dorsolateral region, and behind the eyes, and form the parotid glands (Lacombe et al., 2000). These glands are primarily responsible for passive defense and are the first to develop, although the gland duct lengthens according to the maturation of the epidermis, and opens to the skin surface once they reach metamorphosis (Toledo and Jared, 1995; Lacombe et al., 2000). According to Lacombe et al. (2000), there are two classes of serous glands, type I and II. The first type has a poorly developed smooth endoplasmic reticulum, which is divided into two subtypes, Ia and Ib. Type Ia is characterized by dense granules that carry out the biosynthesis of proteinaceous products reserved for exocytosis (Figure 4H), and involves both the rough endoplasmic reticulum and the Golgi apparatus (Delfino, 1991). The serous secretion of type Ib shows vesicles carrying a lucent material that undergoes maturation without condensation (Toledo and Jared, 1995). Type II is typical of P. bicolor since they present a well-developed, smooth, endoplasmic reticulum that probably plays a role in the biosynthesis of peptides (Blaylock et al., 1976; Lacombe et al., 2000). These peptides are first synthesized as prepropeptides and then, to mature these peptides, the removal of the peptide signal and the acidic propiece occurs and, later, the mature peptides are stored in round granules (Lacombe et al., 2000; Nicolas and El Amri, 2009; Calderon et al., 2011).
2.2 Composition of Phyllomedusa bicolor skin secretion
Amphibians inhabit microorganism-rich environments, especially treefrogs that live in a very humid environment for the main part of their life. As a result, they are more susceptible to pathogens and, in order to defend themselves, they produce potent antimicrobial peptides (Daly, 1995; Govender et al., 2012; Liu et al., 2021). The innate immunity system has the main responsibility for individual survival, in which the secretion produced by anuran glands inhibits diseases, and is toxic to other animals, which may prevent predation in some cases (Nicolas and Mor, 1995; Kimbrell and Beutler, 2001; Toledo et al., 2011). The release of the skin secretion is considered a very efficient defense mechanism because it provides unpalatable characteristics and/or toxicity against potential predators (Bevins and Zasloff, 1990; Daly, 1995; Williams et al., 2000; Rodriguez et al., 2011; Saporito et al., 2012; Hantak et al., 2013). Among the chemical substances responsible for anuran defense, peptides play a central role, while alkaloids are less common and are released in the tegument by some species (Saporito et al., 2009; Jeckel et al., 2015). Peptides are mostly known to act against pathogenic microorganisms; however, studies have shown that they can also have functions related to the interaction between species, such as bradykinin-like peptides released by Bombina orientalis (Bombinatoridae), which are known to be facilitators in intoxication process of predators (Rödel et al., 2013; Raaymakers et al., 2018). Even though the skin secretions of Phyllomedusa bicolor are widely known as a rich source of biologically-active peptides, so far, studies have not been carried to disccover whether the substances released in the skin secretions possess toxicity against predators (Bevins and Zasloff, 1990; Daly, 1995).
All species of Phyllomedusa are prolific producers of peptides, of which, the most representative family is the dermaseptins (Erspamer et al., 1985). Since the first record of the isolation of a peptide from this genus (dating from 1966), more than 277 peptides with unique sequences have been described (Calderon et al., 2011). Most of the bioactive peptides so far characterized have displayed potential applications in medicine, such as phyllocaeruleins with hypotensive properties, tachykinins and phyllokinins as vasodilators, dermorphins and deltorphins with opiate-like properties, and adenoregulins with antibiotic properties (Daly et al., 1992; Mor et al., 1994a; Lacombe et al., 2000; Hesselink and Sacerdote, 2019). These peptides are placed in groups according to their primary activity: antimicrobial peptides; smooth muscle active peptides; and nervous system active peptides (Erspamer et al., 1981). Due to the numerous biological activities of these substances and the similarities with the amino acid sequences related to mammalian neuropeptides and hormones, many have aroused the interest from a medical and pharmacological perspective, such as in the production of new drugs (Lazarus et al., 1999; Basir et al., 2000; Chen et al., 2002; Doyle et al., 2002; Severini et al., 2002; Conceição et al., 2006).
The venom of P. bicolor is used by natives in traditional shamanic rituals in the southwestern Amazon, and is reported to be used as a stimulant and also for healing purposes, although there is no conclusive scientific evidence of its effectiveness (Erspamer-Falconieri et al., 1970; Majic et al., 2021). So far, from the skin secretions of P. bicolor, only peptides have been characterized, from which 28 different sequences have been determined, with most of them belonging to the dermaseptin, deltorphin and demorphin families (Thompson and Williams, 2022).
2.2.1 Dermaseptins
Dermaseptins constitute a superfamily of antimicrobial cationic peptides that are produced in the skin of Phyllomedusidae. They are known to be genetically related, and similar in precursor signals and sequences, but have since diversified and given rise to structurally different peptide subfamilies (Nicolas and El Amri, 2009). Dermaseptins are derived from precursors that have a highly conserved N-terminal preprosequence (Nicolas and Amiche, 2006). Dermaseptins have related structures that differ in sequence length, as well as differences in amino acid sequences. The first dermaseptin characterized was S1 (DRS1), which is present in the skin of P. sauvagii (Mor et al., 1991) while, for P. bicolor, the dermaseptins isolated/characterized in its tegument, so far, belong to the B series (B and B1-B6), G (G3) and other classes of dermaseptin-like peptides (Table 1). With the exception of DRS-S4, all dermaseptins have lower or no toxicity to mammalian cells (Ladram and Nicolas, 2016). The selectiveness and the ability to destabilize the plasmatic membrane is the most pertinent characteristic of the antimicrobial action of dermaseptins. This family of antimicrobial peptides is mainly responsible for the anti-infective passive defense barrier, in which the killing mechanism acts fast by destroying pathogen’s plasmatic membrane in a few minutes, and is unlikely to cause antibiotic-resistance (Shai, 1995; Feder et al., 2000).
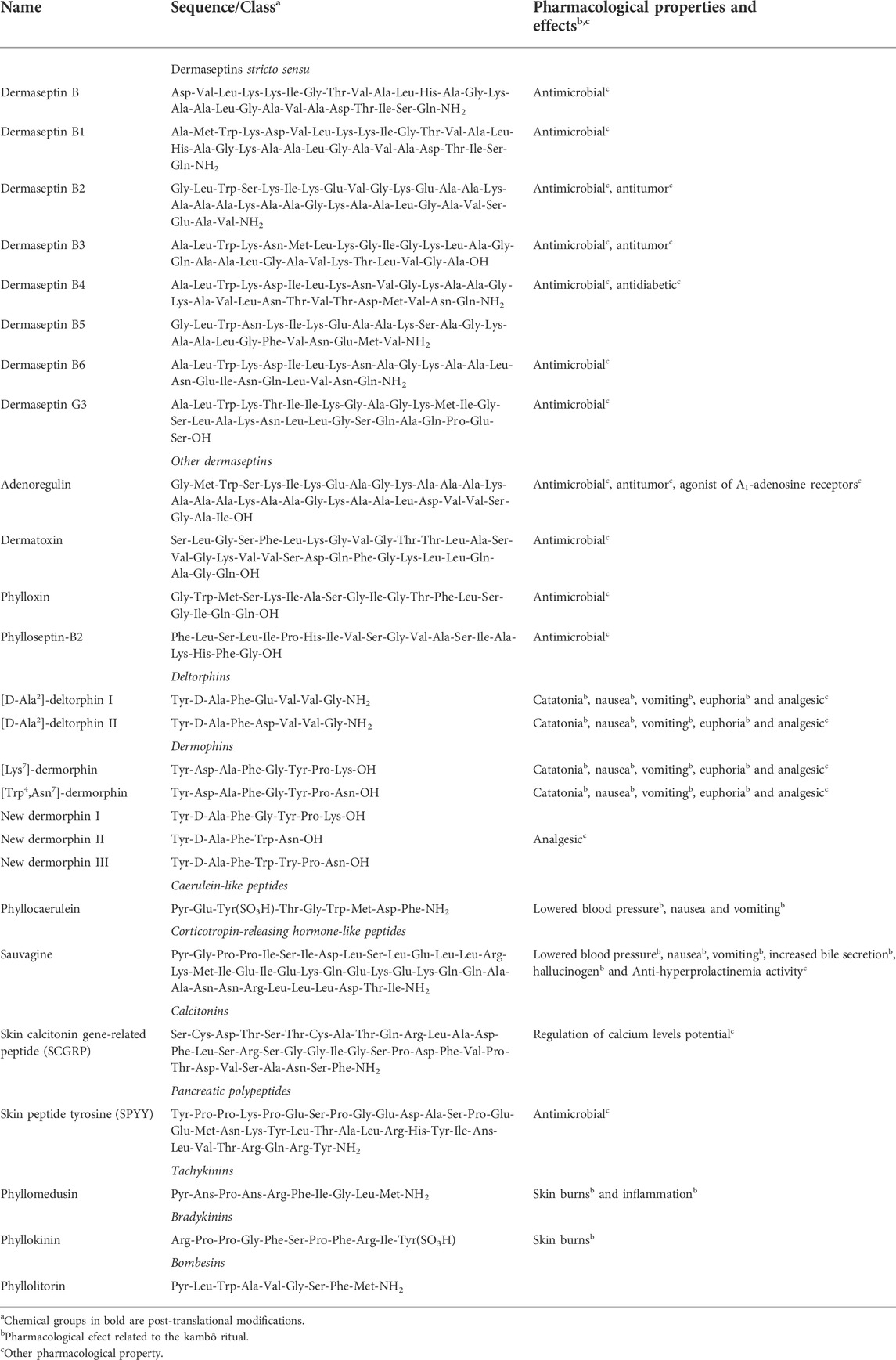
TABLE 1. Classification, sequence details and pharmacological activities and effects of peptides from Phyllomedusa bicolor.
2.2.1.1 Dermaseptins stricto sensu
As a chemical characteristic, most dermaseptins stricto sensu have the C-terminal residue amidated and have the amino acid leucine at position 2 of their primary structure, except dermaseptins B and B1, which have valine and methionine in the same position, respectively. Furthermore, they have a tryptophan residue at position 3 of the primary structure (Daly et al., 1992; Mor et al., 1994a; Charpentier et al., 1998; Fleury et al., 1998; König et al., 2012) (Table 1). Most of the peptides from P. bicolor display post-translationally modified amino acid residues, which play an important role in the activity and receptor specificity (Conibear, 2020). In addition, some modifications can enable cross-coupling of specific amino acids, thus yielding derivatives with increased biostability. Furthermore, the inclusion of such modifications can provide resistance against proteolytic degradation, especially for antimicrobial peptides (Tiwari, 2019). As they are α-helical, cationic, and amphipathic, they act directly on the cell membrane of microorganisms, destabilizing the phospholipid bases and preventing the entry and exit of substances, which leads to cell lysis (Nicolas and Amiche, 2006). For P. bicolor, seven peptide sequences of the dermaseptin stricto sensu type are known (all from the B series), namely dermaseptin B (DRS-B), dermaseptin B1 (DRS-B1), dermaseptin B2 (DRS-B2), dermaseptin B3 (DRS-B3), dermaseptin B4 (DRS-B4), dermaseptin B5 (DRS-B5) and dermaseptin B6 (DRS-B6). The number of B-series dermaseptin stricto sensu residues found in P. bicolor ranges from 24 to 33 amino acids (Charpentier et al., 1998; Fleury, 1998; König et al., 2012) (Table 1).
The DRS-B peptide was first isolated by Mor et al. (1994a), and presented itself as a peptide amide with 27 residues in its sequence, in which the lysine residues alternate hydrophobic and hydrophilic portions. DRS-B shows 78% similarity with dermaseptin S, differing only by a series of deletions and substitutions (Table 1). Mor et al. (1994a) were the first to test DRS-B against pathogenic fungi and bacteria. Minimum inhibitory concentration (MIC) against eight species of fungi and five species of bacteria were inferred. The antimicrobial potential of DRS-B was evident against the fungi Cryptococcus neoformans, Candida albicans, Microsporum canis, Tricophyton rubrum, T. mentagrophytes, Arthroderma simii, Aspergillus fumigatus and A. niger and the bacteria Aeromonas caviae, Escherichia coli, Enterococcus faecalis and Nocardia brasiliensis since MIC values of between 40 and 60 µM were observed (Mor et al., 1994a).
Likewise, all other dermaseptins stricto sensu (DRS-B1 to DRS-B6) have shown activities against microorganisms. DRSB1 has 31 amino acid residues in its primary structure and, unlike other dermaseptins stricto sensu, it has a methionine residue at position 2 of the primary sequence. On the other hand, DRS-B2 has 33 amino acid residues in its structure. Differently from what was reported for the other dermaseptins, Van Zoggel et al. (2012) demonstrated that DRS-B2 inhibited the growth of prostatic adenocarcinomas and some other cell lines. DRS-B3, on the other hand, has 28 amino acid residues in its primary structure, but differs from the others as it does not have any modifications at the C-terminal residue. Similar to DRS-B3, the DRS-B4 peptide has the same amount of amino acid residues; however, it does have a modified C-terminal group (Van Zoggel et al., 2012) (Table 1). Both DRS-B3 and DRS-B4 showed antibacterial activities with MICs ranging between 1.3 and 11.6 µM (Van Zoggel et al., 2012) (Table 1). Morevoer, DRS-B4 showed antidiabetic activity, in which the treatment of glucose-responsive BRIN-BD11 cells with this peptide led to the stimulation of insulin release (Marenah et al., 2004).
The DRS-B5 peptide, composed of 25 amino acid residues, has not been evaluated for its biological potential so far, but its antimicrobial potential is suggested, as is the case for other dermaseptins (Charpentier et al., 1998). Likewise, DRS-B6, whose primary sequence has 24 amino acid residues, has also not been studied for its pharmacological potential. DRS-B6, together with DRS-B1, were related to the chemical defense mechanisms of P. bicolor since they are secreted by the serous glands located in the tibia (König et al., 2012). Finally, the synthetic dermaseptin, named DRS-G3, was identified through the cDNA technique in P. bicolor and synthesized by using 9-fluorenylmethoxycarbonyl-protected (FMOC) amino acids (Fleury et al., 1998). This peptide has 30 residues in its primary structure with a conserved C-terminal. It is possible to note that it has the three amino acid residues (Ala, Leu and Trp) that are common to most of the other dermaseptins present in P. bicolor. Fleury et al. (1998) analyzed the biological activity of DRS-G3 and observed that, as with the known dermaseptins, it also has antimicrobial activity against bacteria. The authors tested, in addition to the MIC, the minimum lethal concentration (MLC) necessary to kill bacteria such as Mollicutes, Firmicutes, and Gracilicutes, and found MICs ranging from 3.0 to 6.25 µM and MLCs ranging from 6.25 to 100 µM (Table 1).
2.2.1.2 Other dermaseptins
Adenoregulin is a polycationic peptide composed of 33 amino acid residues in its primary structure (Table 1). This substance has an α-helical structure with lysines arranged on the external face (Daly et al., 1992). When compared to dermaseptins, adenoregulin has a series of substitutions in its amino acid residues, and no modification in the C-terminal. Cao et al. (2005) produced a recombinant adenoregulin derivative by means of heterologous expression in E. coli. This derivative differs from the original molecule due to the presence of an additional amidated glutamine residue in the C-terminal, which increased the potency of its antimicrobial activity against Tritirachium album and S. cerevisiae.
Besides its antimicrobial properties, adenoregulin has antitumor and angiostatic properties at low concentrations. Interestingly, the anticancer activity of this molecule is linked to cell necrosis (Santos et al., 2017). Additionally, the potential in the regulation of cellular metabolism is also mentioned, since this peptide can bind to adenosine A1 receptors, thus making it a potential molecule for the treatment of depression, Alzheimer’s disease, and Parkinson’s disease. (Cao et al., 2005).
Dermotoxin is a peptide derived from the same precursors of the dermaseptin family, and is a cationic and amphipathic peptide with 32 amino acid residues in its primary structure and has no modifications in the C-terminal (Amiche et al., 2000) (Table 1). In P. bicolor, it has been observed that dermotoxin is expressed both in the skin, intestines and in the brain. Dermatoxin has effective broad-spectrum antimicrobial activity, as do others from the dermaseptin family. Amiche et al. (2000) tested dermatoxin and observed antibacterial activity with MICs of between 6.25 and 100 µM for the Gram-positive bacteria Bacillus megaterium and Corynebacterium glutamicum; for bacteria without cell walls A. laidlawii and Spiroplasma melliferum; and for the Gram-negative bacterium Sinorhizobium meliloti.
Phylloxin is also derived from the dermaseptin precursor group, and has 19 amino acid residues in its primary structure without post-translational modifications (Pierre et al., 2000) (Table 1). Pierre et al. (2000) found antimicrobial activity against some strains of bacteria; however, with less inhibition capability than other dermaseptins. Phylloxin was promising against A. laidlawii, Bacillus megaterium, Spiroplasma melliferum, Escherichia coli, and Micrococcus luteus. Regarding other pharmacological properties, there is no information beyond that related to its antimicrobial activities.
The phylloseptin-B2 peptide was first isolated in Phyllomedusidae from the cutaneous secretion of P. sauvagii (Zhang et al., 2010) and, later, an analog was characterized in P. bicolor through cDNA cloning (König et al., 2012). As the peptide precursors were not previously identified in P. bicolor and the orthology with the related sequences of P. sauvagii and P. hypochondrialis are not guaranteed, the peptide was designated as phylloseptin-B2, though further studies on the subject should be conducted. These antibiotical properties of plasmatic membrane lysis of the peptides envolve several bubble-like formations that disrupt the membrane (Leite et al., 2005). The antimicrobial activity spectrum comprises Gram-negative bacteria, e.g., Acinetobacter calcoaceticus, E. coli and Pseudomonas aeruginosa (Leite et al., 2005; Resende et al., 2008); Gram-positive bacteria, e.g., Enterococcus faecalis, Klebsiella peneumoniae, Staphylococcus aureus and Streptococcus agalactiae; fungi: C. albicans (Resende et al., 2008); and protozoa, e.g., Leishmania amazonensis (promastigotes) (Kückelhaus et al., 2009), Plasmodium falciparum (rings, trophozoites and schizonts) (Kückelhaus et al., 2009), and Trypanosoma cruzi (trypomastigotes) (Leite et al., 2005). However, a toxic effect in mammalls cells was observed, but only in extremally high concetrations (Kückelhaus et al., 2007; Kückelhaus et al., 2009) and the effects on blood cells were insignificant (Leite et al., 2005).
2.2.2 Pancreatic polypeptides (PP)
Pancreatic polypeptides (PP) have been isolated during insulin preparation, and are the first of the PP family. Another peptide of this family is the tyrosine peptide (PYY), whose characteristic is the C-terminal amidated tyrosine residue (Grandt et al., 1994). In anurans, PP derivatives are known, especially in the species Xenopus laevis, whose function in the organism is still not well understood (Kim et al., 2001).
In the species P. bicolor, the only PP characterized so far is the skin peptide tyrosine (SPYY), which was isolated by Mor et al. (1994a). This substance has a primary structure composed of 36 amino acid residues, in which the C-terminal residue is amidated (Table 1). The function of this peptide in P. bicolor is still uncertain, but the inhibitory activity against the alpha-melanocyte stimulating hormone (α-MSH) was tested and it was possible to demonstrate that in vitro SPYY inhibits the secretion of α-MSH (Mor et al., 1994b). The antimicrobial activity of these peptides comprises strains of Gram-negative bacteria (e.g., Aerornonas caviae and E. coli), Gram-positive bacteria (e.g., Enterococcus faecalis and Nocardia brasiliensis), fungi (e.g., Arthroderma simii, Aspergillus fumigatus, A. niger, Microsporum canis) and protozoa (e.g., Leishmania major promastigotes) (Calderon et al., 2011).
2.2.3 Calcitonins
This family consists of peptides with from 32 to 53 amino acid residues (Hay et al., 2017), which are recognized as hormones that are capable of rapidly reducing circulating calcium levels in the body (Naot et al., 2018). The reduction occurs by inhibiting the efflux of calcium from the bone, since peptides of this class act as potent inhibitors of bone resorption (Naot et al., 2018). Other substances are well known in this family, such as amylin, and adrenoregulin (Hay et al., 2017).
Skin calcitonin gene-related peptide (SCGRP) was isolated from the skin of P. bicolor individuals by Seon et al. (2000). The primary structure of this peptide has 37 amino acid residues, and the C-terminal residue is amidated, in addition to having a disulfide bridge between the cysteines at positions two and seven (Table 1). The presence of the disulfide bond may be related to the stabilization of the whole three-dimensional arrangement of SCGRP, which can be crucial for hormonal function (Góngora-Benítez et al., 2014). The researchers who discovered this peptide performed binding assays with the calcitonin receptor and observed that SCGRP differs from all other members of calcitonins in at least nine positions. Like other peptides of this family, it showed competitive inhibition and high specific affinity binding to calcitonin receptors in the brain of rats (Seon et al., 2000).
2.2.4 Corticotropin-releasing hormone-like peptide
Sauvagine is the only peptide in the family of the corticotropin-releasing hormone-like peptides identified in P. bicolor (Erspamer et al., 1993). It has 40 amino acid residues in which the C-terminal residue is amidated, as well as the presence of pyroglutamic acid as the N-terminal residue (Table 1). This substance is also related to some effects of kambô, as well as having hypotensive and antidiuretic activities in rats (Erspamer et al., 1981). Promising results were obtained when sauvagine was evaluated against the condition hyperprolactinemia, in which 500 µg of sauvagine, administered by subcutaneous injection, produced an immediate fall of elevated serum prolactin values to normal values within 5 h (Erspamer et al., 1981).
This peptide mimics the functional consequences of stress exposure and acts as a corticotropin-releasing factor (CRF) (Lovejoy and Balment, 1999; Hesselink and Sacerdote, 2019). It has two subtypes of CRF receptor (CRFR) that binds CRFR1 and CRFR2, and it mediates anxiety due to its CRFR2 agonism (Eckart et al., 1999; Hesselink and Sacerdote, 2019). The effects in animal model studies relate a sequence of an intense, long-lasting hypotensive action followed by tachycardia, antidiuresis, decreasing glomerular filtration rate (GFR) and an increase in tubular Na+ reabsorption (Montecucchi et al., 1979; Erspamer et al., 1981; Heinrichs and Taché, 2001). Moreover, there is a decrease in body temperature caused by the D2 dopamine receptor-mediated mechanism (Hesselink and Sacerdote, 2019). In dog models, it showed different effects such as long-term increases in blood flow (Lovejoy and Balment, 1999).
2.2.5 Tachykinins
Tachykinins are a family of peptides present in amphibians and mammals that exhibit neurotransmitter activity (Steinhoff et al., 2014). They are expressed throughout the nervous and immune system, and regulate an extraordinarily diverse range of physiological processes, as well as being implicated in important pathological conditions (Steinhoff et al., 2014). In anurans, the presence of tachykinins was first described by Schäffer and Gábriel (2005), who detected the presence of this peptide in the retina of an individual of the genus Pelobates. In P. bicolor, the only known tachykinin so far is phyllomedusin.
Phyllomedusin is a decapeptide that was first characterized by Anastasi and Esparmer (1970). Interestingly, it has the pyroglutamic amino acid as an N-terminal residue, in addition to being amidated in the C-terminal (Table 1). Regarding its biological activities, it is known that this peptide promotes smooth muscle contraction, reduces blood pressure in intense compensatory tachycardia, as well as assists in the release of antidiuretic hormones with consequent antidiuresis (Anastasi and Erspamer-Falconieri, 1970; Erspamer-Falconieri et al., 1970). Additionally, in rats, this peptide has been shown to increase the plasma release of corticosterone, catecholamines and glucose, as well as the release of β-endorphins (Mathison and Solomos, 1985).
2.2.6 Bradykinin
Bradykinin-related peptides (BRPs) are a widespread class of amphibian skin peptides that mimic the actions of vertebrate bradykinin hormones, and are secreted in so-called granular or serous glands (Raaymakers et al., 2018). As Phyllomedusa species present a great richness of peptides in the tegument, it is suggested that they may increase the absorption of bradykinin by the epithelium, thus accelerating the predator intoxication process, as reported by Raaymakers et al. (2018) for Bombina orientalis. For P. bicolor phyllokinin, it is the only substance of this family to be identified from its tegument, and the use of BRPs in anti-predator defense events is unknown (König et al., 2012).
Phyllokinin has 11 amino acid residues in its primary structure, and has a sulfated tyrosine amino acid as a C-terminal residue (Anastasi et al., 1966) (Table 1). Pharmacologically, this peptide has been shown to be more potent than bradykinin in lowering blood pressure when tested in dogs; an activity that decreases considerably when the post-translational modification is not present (Anastasi et al., 1966). Regarding the kambô ritual, it is known that this peptide causes some symptoms such as increased heart rate, heat flushing, and redness of the skin (Thompson and Williams, 2022).
2.2.7 Deltorphins
Deltorphins are a small family of short-chain peptides, whose members have between six and seven amino acid residues in their primary structure (Erspamer et al., 1989). In anurans, this family of peptides is restricted to the genus Phyllomedusa (mainly P. bicolor and P. sauvageii) (Erspamer et al., 1989). Peptides of this series are recognized for having high affinity and selectivity for δ-opioid receptors, which make them promising analgesic substances, since they have a potency that is 4,000 times greater than morphine, and 40 times greater than endogenous β-endorphin receptors (Esparmer et al., 1989). These results were corroborated by in vivo studies, indicating that deltorphins have high penetration rates of the blood-brain barrier (Fiori et al., 1997). The small size of deltorphins (also dermophins) may be related to their functions and/or their biological benefits in humans. Specifically, the small peptides present in P. bicolor are the most potent analgesic compounds; however the relationship between the size and their biological benefits remains unclear.
The first deltorphin was discovered in P. sauvageii (Montecucchi et al., 1981a) and served as the basis for the designation of analogous peptide sequences later described in P. bicolor. An interesting feature of this initial discovery was that this peptide has the second amino acid residue (methionine) with inverted stereochemistry, presenting itself in the D configuration (Table 1).
In 1989, Erspamer et al. (1989) isolated two heptapeptide analogs from the cutaneous secretion of P. bicolor, which were named [D-Ala2]-deltorphin I and [D-Ala2]-deltorphin II. Unlike deltorphin, they have the substitution of methionine for alanine at position 2 of the primary sequence and two valine residues in a row in the C-terminal. Another difference between these peptide analogs is the substitution of an amino acid residue at position 4 of the primary structure, in which [D-Ala2]-deltorphin I has a glutamic acid, and [D-Ala2]-deltorphin II has an aspartic acid (Erspamer et al., 1989). In the same study, they demonstrated an affinity of these peptides for opioid receptors that is 10–200 times higher than synthetic enkephalin.
2.2.8 Dermophins
Dermorphins are opioid peptides, and were first isolated from P. sauvagii (Montecucchi et al., 1981a), P. burneisteri, and P. rohdei (Montecucchi et al., 1981b; Broccardo et al., 1981). As observed in deltorphins, they have high affinity and selectivity for opioid receptors, having been shown to produce analgesia in both humans and animals (Negri, 1992). However, the affinity and selectivity of dermorphins are for µ receptors, whereas deltorphins are specific agonists of δ receptors (Richter et al., 1990). When compared to morphine, dermorphins have a potentially more favorable adverse event profile as it is a selective agonist at the Needs correction to ϻ-opioid receptor (MOR), but does not have the relevant affinity for the ƙ-opioid receptor (KOR) (Pescatore et al., 2015; Hesselink et al., 2018b). In preclinical studies on analgesic activities with the intracerebroventricular application of the peptide dermorphin, a better performance than morphine was observed, and showed more prolonged and potent effects (Broccardo et al., 1981). Peptides of this class tend to affect the central nervous system, causing respiratory depression, in addition to high dependence on continued consumption in the kambô ritual (Nakata et al., 1986).
In P. bicolor, three new substances analogous to dermorphin have been described, with cDNAs cloned directly from the skin. These were named by Richter et al. (1990) as new dermorphin I, new dermorphin II, and new dermorphin III. The new analogs I and III are presented as heptapeptides, while the new analog II presented five amino acid residues in its sequence (Table 1). Unlike dermophin, they have a conserved C-terminal and, although deletions and substitutions are observed along their chain, it is possible to note that they share a similar sequence in the three amino acids of the N-terminal portion (Tyr-D-Ala-Phe).
After the chemical synthesis of these predicted peptides, they were evaluated against opioid receptors, in which only the pentapeptide showed activity, but with an IC50 (half maximum inhibitory concentration) that was 10 times lower than that of dermorphin (Richter et al., 1990). Subsequently, two new heptapeptides of the dermorphin class were isolated from the skin of P. bicolor (Mignogna et al., 1992). The first is called [Lys7]-dermorphin, which differs from the model peptide of the class by the presence of lysine at position seven (Mignogna et al., 1992). On the other hand, the peptide [Trp4,Asn7]-dermorphin has two modifications, a tryptophan amino acid residue at position 4 and an asparagine at position 7 (Table 1). In the position 2, these two analogs possess the amino acid alanine in the L configuration, as well as the conserved C-terminal (Mignogna et al., 1992). Like other peptides of this type, these peptides also showed affinity towards opioid receptors; however, the evaluation showed that amidated derivatives tend to be 2 to 4 times more potent than the versions without post-translational modification (Mignogna et al., 1992).
2.2.9 Caeruleins
This family is restricted to two peptides, and only the nonapeptide phyllocaerulein (Anastasi et al., 1969) has been characterized in the species P. bicolor. It is an analog of the caerulein peptide, and differs in that it has one less amino acid, but maintains all the characteristics of its congener, such as composition and the two post-translational modifications: tyrosine with sulfate and the C-terminal is amidated (Anastasi et al., 1969). Another unusual aspect of this peptide is the presence of the amino acid pyroglutamic acid as an N-terminal residue. Phyllocaerulein is considered the most abundant peptide, and co-responsible (along with sauvagine) for the gastrointestinal actions observed in the kambô ritual, in particular, nausea, vomiting and the drop in blood pressure (Thompson and Williams, 2022).
2.2.10 Bombesins
This class of peptides has nine amino acids, and the sequence has pyroglutamic acid as an N-terminal residue, as well as an amidated C-terminal (Table 1). Its occurrence in P. bicolor is limited to trace amounts, of which the phyllolitorin peptide stands out (Erspamer et al., 1985). Regarding their pharmacology, they tend to stimulate the secretion of gastric acids and increase pancreatic secretion, since they are related to the gastrin-releasing peptide. They also have been described as peptides that are capable of suppressing alcohol and food consumption after in vivo evaluation in chicks (Cline et al., 2010). It is worth mentioning that there are contradictions about the presence of bombesines in the secretions of P. bicolor (Thompson and Williams, 2022). However, research has also linked the presence of phyllolitorin with the chills observed in humans during the kambô ritual (Esakov et al., 1990).
3 Kambô ritual: From traditional use to application in large urban centers
Toads, frogs, and treefrogs are animals that are often present in folklore and culture almost everywhere and are associated with rainfall and fertility (Lima and Labate, 2007). The kambô, kapum, or toad vaccine (“vacina do sapo”, in Portuguese) is a purification ritual and is associated with healing rituals in the Amazon rainforest and urban centers around the world (Rudgley, 1993; Lima and Labate, 2007; Haddad and Martins, 2020). The kambô ritual is traditionally performed by shamans to purify the body, increase physical strength and sexual stamina, and ward off “panema” (bad luck and a type of weakness) (Balée, 2004; Lima, 2005; Bernarde and Santos, 2009; Labate and Lima, 2014). It is traditionally used by groups of native hunters in the southwest of the Amazon; among them, the Yawanahua, Kaxinawá, Matsés, Mayoruna, Yawanawá, and especially the Katukina (Daly et al., 1992; Hesselink, 2018a). Natives of the Pano language also apply kambô to the dogs they use during hunting (Souza et al., 2002). From the ethnological literature, there is a record of the use of kambô in more than 15 native groups, belonging to five linguistic families (Pano, Aruak, Arawa, Tikuna, and Tupi-Guarani) located in Bolivia, Brazil, Colombia, Peru, French Guiana, Suriname, and Venezuela (Lima, 2000; Lima, 2005; Lima, 2008; Lima, 2009; Lima, 2012; Lima, 2014a; b; Ribeiro, 2017).
In this ritual, the shaman healer burns the participant and applies the P. bicolor secretion to the wound (Daly et al., 1992). To collect the venom, they go in search of the frog at dawn by following the characteristic sound. Due to the slow movement of the frog, it is easily captured (Daly et al., 1992; Den Brave et al., 2014). After capture, the amphibian is stretched in the shape of an “x” on crossed branches with the hind and front legs tied together (Daly et al., 1992; Den Brave et al., 2014); however, some applicators extract the poison just by handling the animal (Figure 5A). The collector teases the amphibian with pokes so that the skin secretion is released. The secretion produced is then collected by carefully scraping the frog’s skin with a wooden rod and then it is stored in straws for later use (Figures 5A,B). The frog is released after collecting the secretion and, in general, it is not customary to harm the frog or remove an excessive amount of venom or even leaving the animal trapped for a long time (Den Brave et al., 2014). Native collectors believe that hurting them can irritate animal spirits (Daly et al., 1992; Lima, 2005; Den Brave et al., 2014; Hesselink, 2018b; Majić et al., 2021).
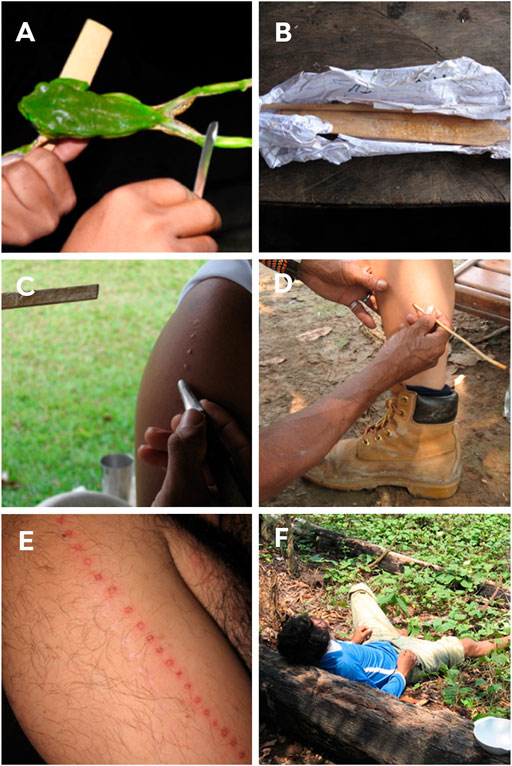
FIGURE 5. (A) Removal of skin secretion from the kambô frog (P. bicolor) by scraping the frog with a wooden rod, for later use in kambô rituals. (B) Skin secretion is stored for later use. (C) Application of secretion to the arm of a man. (D) Burns being made with a thin vine (“titica”) on the leg of a woman for subsequent application of the secretion. (E) Marks on the arms of a man right after participating in the kambô ritual. (F) Reaction after the application of secretion. Participants report apathy, lethargy and the urge to vomit. Photos: Paulo Sérgio Bernarde.
The burns are made in the form of points with the aid of a thin vine (called “titica”) (Figure 5D). The number of points and the place of application are varied and are related to the body part required for activities performed by men and women; and also consider how many times the participant has been submitted to the kambô ritual (Lima, 1994; Hesselink, 2018b). Katukinas apply the secretion to the arms (deltoid) (Figures 5C,E) and chest of men. To women, it is applied to the back of the legs (calf) (Figure 5D), due to the need to strengthen the body part most used to their social role, and the participant can receive more than a hundred “points” at once (Bernarde and Santos, 2009). In the past, triple this number could be applied. Kaxinawá use the secretion in smaller amounts on the legs or arms; they apply 2 to 10 “points”, whereas the Yawanawá apply 50 to 60 “points” (Lima, 1994; Lima, 2000; Lima, 2005; Lima and Labate, 2008). A recent study carried out with non-native populations from the Western Amazon, Rondônia, Brazil, showed that women receive fewer “points”, with an average of 8.2 and 10.3 “points” in the first and second application, respectively, while men receive 8.6 and 11, respectively.
Applications of the secretion usually take place at dawn and participants are recommended to drink approximately one to 2 L of a fresh manioc drink or just water and also fast from the night before. With a full stomach, the participant usually feels the urge to vomit (Ribeiro, 2017). It is reported that the reaction is induced within minutes after the application of a dose. Reactions are often strong and include tachycardia, sweating, and severe vomiting, though generally subside in about 60 min, followed by a state of apathy and dowsiness (Figure 5F) that can last from one to several minutes, days. Subsequently, however, the participant reports greater resistance and clarity of thoughts (Daly et al., 1992; Hesselink, 2018c; Thompson et al., 2022).
Anthropological, biochemical and pharmacological studies have been carried out; however, to date, little is known about the kambô ritual (Anastasi and Erspamer-Falconieri, 1970; Carneiro, 1970; Lima and Labate, 2008; Hesselink, 2018a; Hesselink and Winkelman, 2019). The first ethnographic observations of the kambô ritual in native populations of the upper and middle Juruá were carried out by the French missionary Constantin Tastevin in 1925 (Tastevin, 1925).. It is suggested that the primitive and mythological use of the secretion is related to a shaman of the Kaxinauá tribe in Brazil who, seeing many seriously ill natives and not being cured in rituals with ayahuasca, received a message to enter the forest. There he found a deity holding a green frog, and it was revealed to him how to remove the secretion from the frog and how to apply it (Hesselink, 2018c). The ritual was successfully performed and incorporated into the routine of the Kaxinauá and other tribes (Lagrou, 1991; Lima and Labate, 2008; Venâncio and Melo-Sampaio, 2010; Hesselink, 2018c). From the 19th century onwards, the coexistence between native people and the rubber tappers in the Amazon region, but specifically in the Juruá valley, resulted in a rich exchange of knowledge and practices, and, especially, the incorporation of the kambô ritual by riverine and rubber tappers (Balée, 2004). Francisco Gomes Muniz is credited with the expansion of the kambô ritual among the rubber tappers of a tributary of the Juruá River, in Acre, and the first applications of the secretion in urban centers (Lima and Labate, 2008; Hesselink and Winkelman, 2019).
During the 21st century, the practice has expanded to other neoshamanic circuits, and is currently widespread in therapy clinics, especially those linked to alternative therapies and to Brazilian ayahuasca religions, that is, among supporters of Santo Daime and União do Vegetal (Lima, 2005; Lopes, 2005; Lima and Labate, 2007; Lima and Labate, 2008; Bernarde and Santos, 2009). During its expansion to urban centers, the kambô ritual, however, was transformed into therapeutic approaches and a neoshamanic healing ritual, a process that was labeled as “shamanization of kambô”, but there is no knowledge of the ritual association between both practices (Lima and Labate, 2007; Hesselink, 2018c; Schmidt et al., 2020; Majić et al., 2021).
The expansion of the kambô ritual among non-native people in the Americas and Europe (Carneiro, 1970; Leban et al., 2016; Pogorzelska and Łapiński, 2017; Aquila et al., 2017; Li et al., 2018) has brought to the fore the discussion on issues related to traditional knowledge, in particular, the concern about the responsibility of traditional connoisseurs for the incorrect application of the secretion by non-traditional populations. Silva et al. (2019) warn that, in regions far from where the secretion is traditionally used, this vaccine could be administered by professionals who would not have the same experience as the traditional population that applies it, thus presenting health risks. Among non-native westerners, the kambô ritual is performed in a ceremonial context, different from what happens among native people, with the presence of singing, musical instruments, incense burning and prayers, tobacco snuff (containing Nicotiana rustica) and sananga (eye drops made from Tabernaemontana undulata), both traditional medicines, are often administered in the vicinity of the kambô ritual (Thompson and Williams, 2022). The sale and application of the secretion can lead to serious health risks for users. In 2004, the Brazilian National Health Surveillance Agency determined the suspension of advertising of this therapeutic alternative, since there is no scientific proof that ensures the quality, safety, and efficacy of the secretion for any type of disorder, imbalance, or treatment of any acute and chronic disorders (ANVISA, 2004).
Added to the non-traditional use, questions related to biopiracy have also been raised (Daly et al., 1992; Lima, 2014a; Den Brave et al., 2014; Pogorzelska and Łapiński, 2017; Hesselink, 2018c; Peleg Hasson et al., 2021). Dried secretions of P. bicolor on wooden sticks are commercially available, as “kambô sticks”, in markets and on the internet. Although the use of the secretion appears to have remained sustainable, the expansion of the use of the secretion in recent years could represent a significant environmental impact and the potential decline of the species (Cunha, 2009; Martins, 2010; Ribeiro, 2017). In 2003, the Katukinas, guided by cacique Fernando, denounced the misuse of the secretion and accused the pharmaceutical industry of having patented the kambô peptides, in addition to claiming the rights to the medicinal knowledge of the secretion. In 2004, an alliance between the Katukinas and the Brazilian government was formed to guarantee that the profits generated with the development of the secretion would benefit Brazil. This gave rise to the field project that aimed to bring together natives and researchers, mainly aiming at the regulation of its use by non-native people and the valorization and economic use of traditional knowledge regarding the use of the secretion. However, the project did not get off the ground due to the complexity of issues involving ownership and benefit-sharing (Lima, 2005; Lima, 2014b). From 2002 to 2018, 11 international patents inspired by traditional knowledge and natural genetic resources were approved, and all were issued to countries in the northern hemisphere, which may reflecting the appropriation of natural genetic resources and traditional knowledge (Shiva, 2001; Feres, 2022).
4 Medical aspects of P. bicolor envenomation
The reasons related to the use, structure, and organization of the rituals and the psychological effects of the secretion in western users are scarce and remain anecdotal (Lima and Labate, 2008; Majić et al., 2021). Majić et al. (2021) carried out an Internet-based survey investigating different aspects of use of the secretion, and observed a multitude of motivations for its use, including general healing, detoxification, and spiritual growth. According to Bernarde and Santos (2009), the reasons that lead to use by non-native populations from the Western Amazon, Rondônia, Brazil, are, in general, curiosity and health problems such as rheumatism and diabetes. Benefits to mental and physical health, including treatment of different pathologies, such as addictions, depression, chronic pain, autoimmune disorders, Hashimoto’s thyroiditis, celiac disease, post-traumatic stress disorder, diabetes, infectious diseases, cancer, hypertension, and other health conditions, have been suggested (Hesselink, 2018a; c; Silva et al., 2019; Majić et al., 2021; Thompson and Williams, 2022). Although palettes containing frog secretions can still be ordered via websites without prescriptions, benefits and important biological effects have not been demonstrated in the literature, and it has not yet been officially recognized as a drug. The beneficial effects have not been scientifically tested in randomized controlled trials, so the curative effect may just be a placebo effect (Aquila et al., 2017).
The mechanisms of action and the effects of the secretions from P. bicolor are not completely understood, especially due to the diversity of toxins present in the secretion. In vitro cytotoxic and antitumor effects on different cells have been identified, as well as antibacterial and antiprotozoal properties, besides substances with high affinity for µ-opioid receptors (Negri et al., 1992; Erspamer et al., 1993; Leite et al., 2005; Hesselink and Schatman, 2018) and dilation and increased permeability of vessels and the blood-brain barrier (Erspamer et al., 1989; Erspamer et al., 1993; Daly et al., 2009; Van Zoggel et al., 2012; Li et al., 2018). Overall, frog secretions tend to cause severe adverse side effects, including death. Short-term effects appear within minutes and include gastrointestinal problems, characterized primarily by severe vomiting and diarrhea, urination, sweating, and tachycardia (Erspamer et al., 1989; Erspamer et al., 1993). Although, some effects are considered mandatory symptoms because it is related to cleansing the body of bad influences or toxins (Hesselink, 2018d), these effects are generally dose-dependent (Daly et al., 1992; Li et al., 2018). Increased physical strength, stamina, and the ability to cope with stressful situations are prime examples of the secretion’s late effects. Other delayed effects are hallucination, drowsiness, dizziness, euphoria, and sedation (Erspamer et al., 1989; Erspamer et al., 1993). These effects are mediated by the actions of peptides present in the skin secretion such as phyllokinin, adrenoregulin, sauvagine, phyllocerulin, phyllomedusin, dermorphins, and deltorphins (Li et al., 2018; Byard, 2019). Some researchers have attempted to understand the full effect of the crude secretion, but with few and uncertain results (Thompson et al., 2022; Thompson and Williams, 2022). Generally, most studies focus on the secretion-derived peptides, with individual effects of the main isolated components (Table 2).
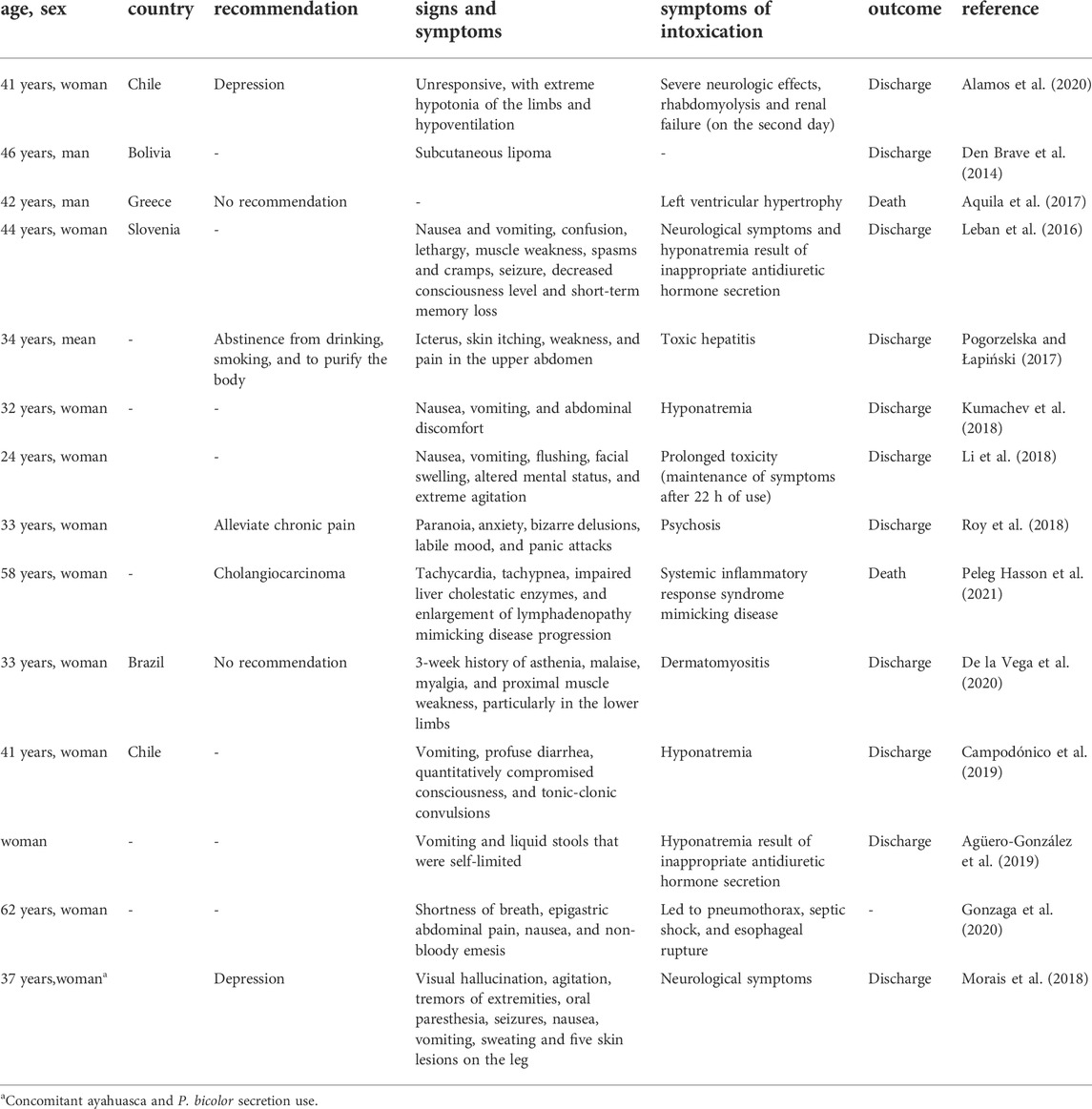
TABLE 2. Effects and recommendations reported in the literature related to the secretion from Phyllomedusa bicolor.
The most common causes of illness and death after kambô rituals are associated with the depressive impact of opioid derivatives on the CNS and the effects of toxins on the cardiovascular system. Kidneys, pancreas and liver can be damaged (Hemingway et al., 2009; Pogorzelska and Łapiński, 2017). There are a few reports of intoxication with both P. bicolor secretion and ayahuasca that led to hospitalization (Morais et al., 2018; Campodónico et al., 2019). However, there are some reports of hospitalization due to intoxication with just the secretion that presented severe side effects (Leban et al., 2016; Hesselink, 2018d; Campodónico et al., 2019; De la Vega et al., 2020; Sacco et al., 2022). In a study with 127 participants who used the secretion, Thompson et al. (2022) observed non-severe physical responses, such as facial swelling, diaphoresis, bowel movements, and syncope, and increasing the dose was responsible for the increase in facial swelling and sweating. Although other studies have shown that the kambô ritual can increase the risks of damage from hyponatremia, asphyxia from vomiting, and injury from syncope and death (Menocchi, 2008; Labate and Lima, 2007; Aquila et al., 2017; Sacco et al., 2022), due to the high concentration of bioactive peptides and potency to certain receptors, the effects can be misinterpreted as intoxication or a massive allergic reaction (Hesselink, 2018d).
5 Study perspectives
As a rich source of peptides, P. bicolor continues to entice researchers in the medical and biotechnological fields due to its vast chemodiversity (as yet not fully known), as well as for all its pharmacological potentials. Regarding this potential, there is a need for studies that aim to further elucidate the mechanisms of action of some of the peptides, especially the opioids that have the potential to be applied in the treatment of chronic diseases. In parallel, the results of studies that indicate antibiotic, antineoplastic, antiviral, immunomodulatory and antiparasitic potential should be further investigated. This need for further studies is supported by the inherent characteristics of these peptides, such as low molecular weights, high activities, low cytotoxicity, high water solubility and rapid absorption. In this way, it is expected that, in the future, the chemistry of secretions from P. bicolor will advance towards useful and safe treatments, where once again traditional knowledge helps future generations.
6 Final remarks
The species P. bicolor is prevalent in the Amazonian biome, where it is used by the indigenous populations in the folk and popular sectors of healthcare. Currently, its use has spread to other populations, still with the original objectives of prevention and treatment of a series of illnesses and traditional syndromes, as well as for use in certain religious cults. The expansion of its use has raised concerns about biopiracy, with reports of use in European countries and the United States. Excessive use or use by persons with prior health problems can result in critically-ill envenomation and deaths. There are no management protocols for these cases of envenomations, and supportive care has been adopted in the few reports found in the literature. The richness of the secretion’s bioactive peptides with different biological activities is responsible both for the signs and symptoms of their popular use and for the envenomations. Several studies have been carried out to better understand the chemical nature of these peptides and their biological effects from in vitro and limited in vivo data. From a biotechnological perspective, the antibiotic, antineoplastic, antiviral, immunomodulatory and antiparasitic potential activities are of interest. (Bahar and Ren, 2013, Mor et al., 1994c, Vanhoye et al., 2004).
Author contributions
IK, MS, MP, PB, HK, and WM conceived the main idea of this work. All authors designed and wrote most of this review’s topics. TN, AB, and MJ elaborated the figures of this review article. All authors corrected the manuscript and provided important contributions during the development of this work. All authors contributed to the article and approved the final version.
Funding
We would like to thank Conselho Nacional de Desenvolvimento Científico e Tecnológico (CNPq) for scholarships to MP (No. 307184/2020-0), WM (No. 309207/2020-7), to HK (No.305942/2020-4), to IK (No. 309473/2019-5) and PB (No. 311509/2020-7). WM acknowledges funding from Fundação de Amparo à Pesquisa do Estado do Amazonas (Pro-Estado and Posgrad calls). MP (Snakebite Roraima project coordinator) acknowledges funding support from Hamish Ogston Foundation–Global Snakebite Initiative. Support via CT&I Priority Areas Call FAPEAM 010/2021 and POSGRAD 2020–2021 was provided to HK. JS acknowledges funding from Fundação de Amparo à Pesquisa do Estado do Amazonas (Science in Public Management Program–PCGP/FAPEAM 011/2021). This research was partially supported by Brazilian CAPES (Coordenação de Aperfeiçoamento de Pessoal de Nível Superior).
Conflicts of interest
The authors declare that the research was conducted in the absence of any commercial or financial relationships that could be construed as a potential conflict of interest.
Publisher’s note
All claims expressed in this article are solely those of the authors and do not necessarily represent those of their affiliated organizations, or those of the publisher, the editors and the reviewers. Any product that may be evaluated in this article, or claim that may be made by its manufacturer, is not guaranteed or endorsed by the publisher.
References
Agüero-González, D. L., Pané-Vila, A., Gil, V., and Castro, P. (2019). Rituales de purificación con una rana amazónica (Kambô): Una peligrosa realidad en la Europa del siglo XXI. Emergencias 31 (5).
Alamos, M. F., Walker, R. H., and Miranda, M. (2020). Life-threatening risk of using Kambó in alternative medicine. Neurol. Clin. Pract. 10 (4), 1212 e35. doi:10.1212/CPJ.0000000000000749
Altig, R., and McDiarmid, R. W. (1999). “Tadpoles: The biology of Anuran Larvae,” in Body plan: Development and morphology. Editors R. W. McDiarmid, and R. Altig (The University of Chicago Press), 24–51.
Amiche, M., Seon, A. A., Wroblewski, H., and Nicolas, P. (2000). Isolation of dermatoxin from frog skin, an antibacterial peptide encoded by a novel member of the dermaseptin genes family. Eur. J. Biochem. 267 (14), 4583–4592. doi:10.1046/j.1432-1327.2000.01514.x
Anastasi, A., Bertaccini, G., Cei, J. M., De Caro, G., Erspamer, V., and Impicciatore, M. (1969). Structure and pharmacological actions of phyllocaerulein, a caerulein-like nonapeptide: Its occurrence in extracts of the skin of Phyllomedusa sauvagei and related Phyllomedusa species. Br. J. Pharmacol. 37 (1), 198–206. doi:10.1111/j.1476-5381.1969.tb09538.x
Anastasi, A., Bertaccini, G., and Erspamer, V. (1966). Pharmacological data on phyllokinin (bradykinyl‐ isoleucyl‐ tyrosine o‐ sulphate) and bradykinyl‐ isoleucyl‐ tyrosine. Br. J. Pharmacol. Chemother. 27 (3), 479–485. doi:10.1111/j.1476-5381.1966.tb01859.x
Anastasi, A., and Falconieri Erspamer, G. (1970). Occurrence of phyllomedusin, a physalaemin-like decapeptide, in the skin of Phyllomedusa bicolor. Experientia 26 (8), 866–867.
ANVISA (2004). Resolução-RE Nº8, de 29 de Abril de 2004. Agência Nacional de Vigilância Sanitária. Diário Of. União, Brasília, DF, Seção 1 Nº 82, 94, 30 abr.
Aquila, I., Gratteri, S., Sacco, M. A., Fineschi, V., Magi, S., Castaldo, P., et al. (2017). The biological effects of kambo: Is there a relationship between its administration and sudden death? J. Forensic Sci. 63 (3), 965–968. doi:10.1007/BF02114227
Bahar, A. A., and Ren, D. (2013). Antimicrobial peptides. Pharmaceuticals 6 (12), 1543–1575. doi:10.3390/ph6121543
Balée, W. (2004). Enciclopédia da floresta: o alto Juruá: Práticas e conhecimentos das populações. Mana 10 (2), 419–423. doi:10.1590/S0104-93132004000200009
Barra, D., and Simmaco, M. (1995). Amphibian skin: A promising resource for antimicrobial peptides. Trends Biotechnol. 13 (6), 205–209. doi:10.1016/S0167-7799(00)88947-7
Basir, Y. J., Knoop, F. C., Dulka, J., and Conlon, J. M. (2000). Multiple antimicrobial peptides and peptides related to bradykinin and neuromedin N isolated from skin secretions of the pickerel frog, Rana palustris. Biochimica Biophysica Acta (BBA)-Protein Struct. Mol. Enzym. 1543 (1), 95–105. doi:10.1016/S0167-4838(00)00191-6
Bernarde, P. S. (2012). Anfíbios e répteis: Introdução ao estudo da herpetofauna brasileira. Curitiba: Anolis Books.
Bernarde, P. S., and Santos, R. A. (2009). Utilização medicinal da secreção (“vacina-do-sapo”) do anfíbio kambô (Phyllomedusa bicolor)(Anura: Hylidae) por população não-indígena em Espigão do Oeste, Rondônia, Brasil. Biotemas 22 (3), 213–220. doi:10.5007/2175-7925.2009v22n3p213
Bertoluci, J. (2002). Pedal luring in the leaf-frog Phyllomedusa burmeisteri (Anura, hylidae, phyllomedusinae). Phyllomedusa J. Herpetology 1 (2), 93–95. doi:10.11606/issn.2316-9079.v1i2p93-95
Bevins, C. L., and Zasloff, M. (1990). Peptides from frog skin. Annu. Rev. Biochem. 59 (1), 395–414. doi:10.1146/annurev.bi.59.070190.002143
Blaylock, L. A., Ruibal, R., and Platt-Aloia, K. (1976). Skin structure and wiping behavior of Phyllomedusinae frogs. Copeia 1976 (2), 283–295. doi:10.2307/1443948
Broccardo, M., Erspamer, V., Falconieri, G., Improta, G., Linari, G., Melchiorri, P., et al. (1981). Pharmacological data on dermorphins, a new class of potent opioid peptides from amphibian skin. Br. J. Pharmacol. 73 (3), 625–631. doi:10.1111/j.1476-5381.1981.tb16797.x
Buchacher, C. O. (1993). Field studies on the small Surinam toad, Pipa arrabali, near Manaus, Brazil. Amphibia-Reptilia 14 (1), 59–69. doi:10.1163/156853893x00192
Byard, R. W. (2019). Is voluntary envenomation from the kambô ritual therapeutic or toxic? Forensic Sci. Med. Pathology 16 (2), 205–206. doi:10.1007/s12024-019-00192-5
Calderon, L. A., Silva, A. A. E., Ciancaglini, P., and Guerino, S. R. (2011). Antimicrobial peptides from Phyllomedusa frogs: From biomolecular diversity to potential nanotechnologic medical applications. Amino Acids 40, 29–49. doi:10.1007/s00726-010-0622-3
Campião, K. M., Morais, D. H., Dias, O. T., Aguiar, A., Toledo, G. M., Tavares, L. E. R., et al. (2014). Checklist of helminth parasites of amphibians from South America. Zootaxa 3843 (1), 1–93. doi:10.11646/zootaxa.3843.1.1
Campodónico, J., Aedo, P., Montané, M. I., Rojas, A., Aveiga, A., Silva, L., et al. (2019). Severe hyponatremia secondary to Phyllomedusa bicolor (Kambó frog) poisoning. Report of one case. Rev. Médica Chile 147, 935–939.
Cao, W., Zhou, Y., Ma, Y., Luo, Q., and Wei, D. (2005). Expression and purification of antimicrobial peptide adenoregulin with C-amidated terminus in Escherichia coli. Protein Expr. Purif. 40 (2), 404–410. doi:10.1016/j.pep.2004.12.007
Caramaschi, U., and Cruz, C. A. G. (2002). Phyllomedusa: Posição taxonômica, hábitos e biologia (Amphibia, Anura, hylidae). Phyllomedusa 1, 5–10. doi:10.11606/issn.2316-9079.v1i1p05-10
Carneiro, R. L. (1970). Hunting and hunting magic among the amahuaca of the Peruvian Montana. Ethnology 9 (4), 331–341. doi:10.2307/3773039
Castanho, L. M., and de Luca, I. M. S. (2001). Moulting behavior in leaf-frogs of the genus Phyllomedusa (Anura: Hylidae). Zoologischer Anzeiger-A J. Comp. Zoology 240 (1), 3–6. doi:10.1078/0044-5231-00001
Castroviejo-Fisher, S., Köhler, J., De la Riva, I., and Padial, J. M. (2017). A new morphologically cryptic species of Phyllomedusa (Anura: Phyllomedusidae) from Amazonian forests of northern Peru revealed by DNA sequences. Zootaxa 4269, 245–264. doi:10.11646/ZOOTAXA.4269.2.4
Chai, N. (2015). Endoscopy in Amphibians. Veterinary Clin. N. Am Exot. Animal Pract. 18 (3), 479–491. doi:10.1016/j.cvex.2015.04.006
Charpentier, S., Amiche, M., Mester, J., Vouille, V., Le Caer, J. P., Nicolas, P., et al. (1998). Structure, synthesis, and molecular cloning of dermaseptins B, a family of skin peptide antibiotics. J. Biol. Chem. 273 (24), 14690–14697. doi:10.1074/jbc.273.24.14690
Chen, F. Y., Lee, M. T., and Huang, H. W. (2002). Sigmoidal concentration dependence of antimicrobial peptide activities: A case study on alamethicin. Biophysical J. 82 (2), 908–914. doi:10.1016/S0006-3495(02)75452-0
Chen, Z., Xi, X., Lu, Y., Hu, H., Dong, Z., Ma, C., et al. (2021). In vitro activities of a novel antimicrobial peptide isolated from Phyllomedusa tomopterna. Microb. Pathog. 153, 104795. doi:10.1016/j.micpath.2021.104795
Clarke, B. T. (1997). The natural history of Amphibian skin secretions, their normal functioning and potential medical applications. Biol. Rev. Camb. Philosophical Soc. 72 (3), 365–379. doi:10.1017/s0006323197005045
Cline, M. A., Cofield, S. A., and Tachibana, T. (2010). Central litorin injection is associated with primary anorexigenic effects that coincide with activation of the magnocellular division of the paraventricular nucleus. Neuropeptides 44 (3), 247–252. doi:10.1016/j.npep.2009.12.015
Conceição, K., Konno, K., Richardson, M., Antoniazzi, M. M., Jared, C., Daffre, S., et al. (2006). Isolation and biochemical characterization of peptides presenting antimicrobial activity from the skin of Phyllomedusa hypochondrialis. Peptides 27 (12), 3092–3099. doi:10.1016/j.peptides.2006.08.005
Conibear, A. C. (2020). Deciphering protein post-translational modifications using chemical biology tools. Nat. Rev. Chem. 4, 674–695. doi:10.1038/s41570-020-00223-8
Cunha, M. C. (2009). “Cultura e cultura: Conhecimentos tradicionais e direitos intelectuais,” in Cultura com aspas (São Paulo: Cosac Naify), 311–373.
Daly, J. W., Caceres, J., Moni, R. W., Gusovsky, F., Moos, M., Seamon, K. B., et al. (1992). Frog secretions and hunting magic in the upper Amazon: Identification of a peptide that interacts with an adenosine receptor. Proc. Natl. Acad. Sci. 89 (22), 10960–10963. doi:10.1073/pnas.89.22.10960
Daly, J. W. (1995). The chemistry of poisons in amphibian skin. Proc. Natl. Acad. Sci. 92 (1), 9–13. doi:10.1073/pnas.92.1.9
Daly, J. W., Ware, N., Saporito, R. A., Spande, T. F., and Garraffo, H. M. (2009). N-Methyldecahydroquinolines: An unexpected class of alkaloids from amazonian poison frogs (Dendrobatidae). J. Nat. Prod. 72 (6), 1110–1114. doi:10.1021/np900094v
de la Vega, M., Maldonado, G., and Kraus, A. (2020). Dermatomyositis induced by the secretion of Phyllomedusa bicolor or Kambô frog - a case report. Toxicon 184, 57–61. doi:10.1016/j.toxicon.2020.05.018
Delfino, G. (1991). in Ultrastructural aspects of venom secretion in anuran cutaneous glands”Handbook of natural toxins. Editors A. T. Tu, and M. Dekker (New York, NY, USA: Marcel Dekker), 777–802.
Delfino, G., Brizzi, R., Alvarez, B. B., and Kracke-Berndorff, R. (1998). Serous cutaneous glands in Phyllomedusa hypochondrialis (Anura:hylidae): Secretory patterns during ontogenesis. Tissue Cell 30 (1), 30–40. doi:10.1016/S0040-8166(98)80004-9
den Brave, P. S., Bruins, E., and Bronkhorst, M. W. (2014). Phyllomedusa bicolor skin secretion and the Kambô ritual. J. Venom. Animals Toxins Incl. Trop. Dis. 20, 1–3. doi:10.1186/1678-9199-20-40
Doyle, J., Llewellyn, L. E., Brinkworth, C. S., Bowie, J. H., Wegener, K. L., Rozek, T., et al. (2002). Amphibian peptides that inhibit neuronal nitric oxide synthase: The isolation of lesueurin from the skin secretion of the Australian stony creek frog Litoria lesueuri. Eur. J. Biochem. 269 (1), 100–109. doi:10.1046/j.0014-2956.2002.02630.x
Duellman, W. E., Marion, A. B., and Hedges, S. B. (2016). Phylogenetics, classification, and biogeography of the treefrogs (Amphibia: Anura: Arboranae). Zootaxa 4104 (1), 1–109. doi:10.11646/ZOOTAXA.4104.1.1
Eckart, K., Radulovic, J., Radulovic, M., Jahn, O., Blank, T., Stiedl, O., et al. (1999). Actions of CRF and its analogs. Curr. Med. Chem. 6 (11), 1035–1053.
Erspamer, V., Falconieri-Erspamer, G., Severini, C., Potenza, R. L., Barra, D., Mignogna, G., et al. (1993). Pharmacological studies of “sapo” from the frog Phyllomedusa bicolor skin: A drug used by the Peruvian matses Indians in shamanic hunting practices. Toxicon 31, 1099–1111.
Erspamer, V., Melchiorri, P., Broccardo, M., Erspamer, G. F., Falaschi, P., Improta, G., et al. (1981). The brain-gut-skin triangle: New peptides. Peptides 2 (2), 7–16. doi:10.1016/0196-9781(81)90003-6
Erspamer, V., Melchiorri, P., Falconieri-Erspamer, G., Montecucchi, P. C., and de Castiglione, R. (1985). Phyllomedusa skin: A huge factory and store-house of a variety of active peptides. Peptides 6 (3), 7–12. doi:10.1016/0196-9781(85)90343-2
Erspamer, V., Melchiorri, P., Falconieri-Erspamer, G., Negri, L., Corsi, R., Severini, C., et al. (1989). Deltorphins: A family of naturally occurring peptides with high affinity and selectivity for delta opioid binding sites. Proc. Natl. Acad. Sci. 86 (13), 5188–5192. doi:10.1073/pnas.86.13.5188
Erspamer-Falconieri, G., Anastasi, A., and Cei, J. M. (1970). Pharmacological observations on phyllomedusin. J. Pharm. Pharmacol. 22 (6), 466–467. doi:10.1111/j.2042-7158.1970.tb08567.x
Esakov, A. I., Ashmarin, I. P., Serova, O. N., Obukhova, M. F., Storozheva, Z. I., Golubovich, V. P., et al. (1990). Litorin and litorin-albumin conjugate as effective regulators of body temperature in rats. Biomed. Sci. 1 (6), 610–612.
Faivovich, J., Haddad, C. F. B., Baêta, D., Jungfer, K. H., Álvarez, G. R. F., Brandão, R. A., et al. (2010). The phylogenetic relationships of the charismatic poster frogs, Phyllomedusinae (Anura, Hylidae). Cladistics 26, 227–261. doi:10.1111/j.1096-0031.2009.00287.x
Faivovich, J., Haddad, C. F. B., Garcia, P. C. A., Frost, D. R., Campbell, J. A., and Wheeler, W. C. (2005). Systematic review of the frog family hylidae, with special reference to hylinae: Phylogenetic analysis and taxonomic revision. Bull. Am. Mus. Nat. Hist. 294, 1–240. doi:10.5531/sd.sp.12
Feder, R., Dagan, A., and Mor, A. (2000). Structure-activity relationship study of antimicrobial dermaseptin S4 showing the consequences of peptide oligomerization on selective cytotoxicity. J. Biol. Chem. 275 (6), 4230–4238.
Feres, M. V. C. (2022). Biodiversity, traditional knowledge and patent rights: The case study of Phyllomedusa bicolor. Rev. Direito GV 18 (1), e2205. doi:10.1590/2317-6172202205
Fiori, A., Cardelli, P., Negri, L., Savi, M. R., Strom, R., and Erspamer, V. (1997). Deltorphin transport across the blood–brain barrier. Proc. Natl. Acad. Sci. 94 (17), 9469–9474. doi:10.1073/pnas.94.17.9469
Fleury, Y., Vouille, V., Beven, L., Amiche, M., Wróblewski, H., Delfour, A., et al. (1998). Synthesis, antimicrobial activity and gene structure of a novel member of the dermaseptin B family. Biochimica Biophysica Acta (BBA)-Gene Struct. Expr. 1396 (2), 228–236. doi:10.1016/S0167-4781(97)00194-2
Frost, D. R. (2021). Amphibian species of the world: An online reference. Version6.1. Disponível em. New York, NY, USA: American Museum of Natural History. Acessed https://amphibiansoftheworld.amnh.org/index.php April 11, 2022.
Gehara, M., Crawford, A. J., Orrico, V. G., Rodriguez, A., Loetters, S., Fouquet, A., et al. (2014). High levels of diversity uncovered in a widespread nominal taxon: Continental phylogeography of the neotropical tree frog Dendropsophus minutus. PloS One 9 (9), e103958. doi:10.1371/journal.pone.0103958
Gomez-Mestre, I., Wiens, J. J., and Warkentin, K. M. (2008). Evolution of adaptive plasticity: Risk-sensitive hatching in neotropical leafbreeding treefrogs. Ecol. Monogr. 78, 205–224. doi:10.1890/07-0529.1
Góngora-Benítez, M., Tulla-Puche, J., and Albericio, F. (2014). Multifaceted roles of disulfide bonds. Peptides as therapeutics. Chem. Rev. 114, 901–926. doi:10.1021/cr400031z
Gonzaga, E. S. R., Chamorro, M., Ganti, L., and Schneider, R. (2020). Kambo frog poison as a cause of esophageal rupture. Cureus 12 (9), e10677. doi:10.7759/cureus.10677
Govender, T., Dawood, A., Esterhuyse, A. J., and Katerere, D. R. (2012). Antimicrobial properties of the skin secretions of frogs. South Afr. J. Sci. 108 (5), 1. doi:10.10520/EJC120717
Grandt, D., Schimiczek, M., Struk, K., Shively, J., Eysselein, V. E., Goebell, H., et al. (1994). Characterization of two forms of peptide YY, PYY (1–36) and PYY (3–36), in the rabbit. Peptides 15 (5), 815–820. doi:10.1016/0196-9781(94)90035-3
Hantak, M. M., Grant, T., Reinsch, S., Mcginnity, D., Loring, M., Toyooka, N., et al. (2013). Dietary alkaloid sequestration in a poison frog: An experimental test of alkaloid uptake in Melanophryniscus stelzneri (bufonidae). J. Chem. Ecol. 39 (11), 1400–1406. doi:10.1007/s10886-013-0361-5
Hay, D. L., Garelja, M. L., Poyner, D. R., and Walker, C. S. (2017). Update on the pharmacology of calcitonin/CGRP family of peptides: IUPHAR review 25. Br. J. Pharmacol. 175 (1), 3–17. doi:10.1111/bph.14075
Heinrichs, S. C., and Taché, Y. (2001). Therapeutic potential of CRF receptor antagonists: A gut-brain perspective. Expert Opin. Investig. Drugs 10 (4), 647–659. doi:10.1517/13543784.10.4.647
Hemingway, V., Brunner, J., Speare, R., Berger, L., Heatwole, H., and Wilkinson, J. W. (2009). “Amphibian decline: Disease, parasites, maladies, and pollution,” in Amphibian Biology.
Hesselink, J. M. K. (2018a). Kambô: A shamanistic ritual arriving in the West: Description, risks and perception by the users. Int. J. Psychol. Psychoanal. 4 (2), 034. doi:10.23937/2572-4037.1510034
Hesselink, J. M. K. (2018b). Kambo: A ritualistic healing substance from an amazonian frog and a source of new treatments. Open J. Pain Med. 2 (1), 4–006. doi:10.17352/ojpm.000007
Hesselink, J. M. K. (2018c). Kambô: A shamanic medicine-personal testimonies. JOJ Case Stud. 8 (3), 555739. doi:10.19080/JOJCS.2018.08.555739
Hesselink, J. M. K. (2018d). Kambo and its multitude of biological effects: Adverse events or pharmacological effects. Int. Archives Clin. Pharmacol. 4 (1), 17. doi:10.23937/2572-3987.1510017
Hesselink, J. M. K., and Sacerdote, P. (2019). Frogs as a rich source for new treatment principles: A review of bio-active peptides in phyllomedusae (Phyllomedusa bicolor) and bombinae (Bombina variegate). SL Pharmacol. Toxicol. 2 (1), 116.
Hesselink, J. M. K., and Schatman, M. E. (2018). Rediscovery of old drugs: The forgotten case of dermorphin for postoperative pain and palliation. J. Pain Res. 11, 2991–2995. doi:10.2147/JPR.S186082
Hesselink, J. M. K., and Winkelman, M. (2019). Vaccination with Kambô against bad influences: Processes of symbolic healing and ecotherapy. J. Transpers. Psychol. 51 (1), 28–48.
Iakp (2022). International association of kambo practitioners. Available at: https://iakp.org/ (Acessed April 3, 2022).
IUCN (2021). The IUCN red list of threatened species. Version 2021-3 Accessed on: https://www.iucnredlist.org (Acessed April 11, 2022).
Jairam, R. (2019). Checklist of the amphibians and reptiles of the Lely Mountains, eastern Suriname. Amphibian Reptile Conservation 13 (2), e200.
Jared, C., Antoniazzi, M. M., Jordão, A. E., Silva, J. R. M., Greven, H., and Rodrigues, M. T. (2009). Parotoid macroglands in toad (Rhinella jimi): Their structure and functioning in passive defence. Toxicon 54 (3), 197–207. doi:10.1016/j.toxicon.2009.03.029
Jeckel, A. M., Saporito, R. A., and Grant, T. (2015). The relationship between poison frog chemical defenses and age, body size, and sex. Front. Zoology 12 (1), 1–9. doi:10.1186/s12983-015-0120-2
Kim, J. B., Johansson, Å., and Conlon, J. M. (2001). Anomalous rates of evolution of pancreatic polypeptide and peptide tyrosine-tyrosine (PYY) in a tetraploid frog, Xenopus laevis (Anura: Pipidae). Peptides 22 (3), 317–323. doi:10.1016/S0196-9781(01)00333-3
Kimbrell, D. A., and Beutler, B. (2001). The evolution and genetics of innate immunity. Nat. Rev. Genet. 2 (4), 256–267. doi:10.1038/35066006
König, E., Clark, V. C., Shaw, C., and Bininda-Emonds, O. R. (2012). Molecular cloning of skin peptide precursor-encoding cDNAs from tibial gland secretion of the Giant Monkey Frog, Phyllomedusa bicolor (Hylidae, Anura). Peptides 38 (2), 371–376. doi:10.1016/j.peptides.2012.09.010
Kückelhaus, S., Leite, J. R. S., Neves, M. P., Frota, K. S., Abdala, L. F., Muniz-Junqueira, M. I., et al. (2007). Toxicity evaluation to mice of phylloseptin-1, an antimicrobial peptide from the skin secretion of Phyllomedusa hypochondrialis (Amphibia). Int. J. Peptide Res. Ther. 13 (3), 423–429. doi:10.1007/s10989-006-9060-1
Kückelhaus, S. A., Leite, J. R. S., Muniz-Junqueira, M. I., Sampaio, R. N., Bloch, C., and Tosta, C. E. (2009). Antiplasmodial and antileishmanial activities of phylloseptin-1, an antimicrobial peptide from the skin secretion of Phyllomedusa azurea (Amphibia). Exp. Parasitol. 123 (1), 11–16. doi:10.1016/j.exppara.2009.05.002
Kumachev, A., Zipursky, J. S., Weinerman, A. S., and Thompson, M. (2018). Poisoning from the kambô ritual. Can. J. Emerg. Med. 20 (6), 962–964. doi:10.1017/cem.2018.58
Labate, B. C., and Lima, E. C. (2014). Medical drug or shamanic power plant: The uses of kambô in Brazil. Ponto Urbe 15, 1–20. doi:10.4000/pontourbe.2384
Lacombe, C., Cifuentes-Diaz, C., Dunia, I., Auber-Thomay, M., Nicolas, P., and Amiche, M. (2000). Peptide secretion in the cutaneous glands of South American tree frog Phyllomedusa bicolor: An ultrastructural study. Eur. J. Cell Biol. 79 (9), 631–641. doi:10.1078/0171-9335-00085
Ladram, A., and Nicolas, P. (2016). Antimicrobial peptides from frog skin: Biodiversity and therapeutic promises. Front. Biosci. 21, 1341–1371. doi:10.2741/4461
Lagrou, E. M. (1991). Uma etnografia da cultura Kaxinawá entre a cobra e o inca. Federal University of Santa Catarina. [Dissertation] [Florianópolis (SC)].
Larsen, E. H., and Ramløv, H. (2013). Role of cutaneous surface fluid in frog osmoregulation. Comp. Biochem. Physiology Part A Mol. Integr. Physiology 165 (3), 365–370. doi:10.1016/j.cbpa.2013.04.005
Lazarus, L. H., Bryant, S. D., Cooper, P. S., and Salvadori, S. (1999). What peptides these deltorphins be. Prog. Neurobiol. 57 (4), 377–420. doi:10.1016/S0301-0082(98)00050-1
Leban, V., Kozelj, G., and Brvar, M. (2016). The syndrome of inappropriate antidiuretic hormone secretion after giant leaf frog (Phyllomedusa bicolor) venom exposure. Toxicon 120, 107–109. doi:10.1016/j.toxicon.2016.07.007
Leite, J. R. S., Silva, L. P., Rodrigues, M. I. S., Prates, M. V., Brand, G. D., Lacava, B. M., et al. (2005). Phylloseptins: A novel class of anti-bacterial and anti-protozoan peptides from the Phyllomedusa genus. Peptides 26 (4), 565–573. doi:10.1016/j.peptides.2004.11.002
Li, K., Horng, H., Lynch, K., and Smollin, C. G. (2018). Prolonged toxicity from Kambo cleansing ritual. Clin. Toxicol. 56 (11), 1165–1166. doi:10.1080/15563650.2018.1457153
Lima, E. C., and Labate, B. C. (2007). Remédio da ciência" e" remédio da alma": Os usos da secreção do kambô (Phyllomedusa bicolor) nas cidades. Campos - Rev. Antropol. 8 (1), 71–90. doi:10.5380/cam.v8i1.9553
Lima, E. C., and Labate, B. C. (2008). A expansão urbana do kampo (Phyllomedusa bicolor): Notas etnográficas1. DroDrogas Cult. Novas Perspect., 315–344.
Lima, E. C. (1994). Katukina: Historia e organizacao social de um grupo pano do alto jurua. University of São Paulo. [Dissertation][São Paulo (SP)].
Lima, E. C. (2000). Detalhe do registro: Com os olhos da serpente: Homens, animais e espíritos nas concepções Katukina sobre a natureza. University of São Paulo. [Thesis][São Paulo (SP)].
Lima, E. C. (2005). Kampu, kampo, kambô: o uso do sapo-verde entre os katukina. Rev. do Patrimônio Histórico Artístico Nac. 32, 255–267.
Lima, E. C. (2008). “As novas formas do kampô: Elementos de uma sociologia da disseminação urbana dos saberes nativos,” in Pueblos indígenas, plantas y mercados–amazonía y gran chaco. V congreso CEISAL de Latinoamericanistas, zeta series in anthropology & sociology.
Lima, E. C. (2009). “Entre o mercado esotérico e os direitos de propriedade intelectual: o caso do kampô (Phyllomedusa bicolor),” in Dilemas do acesso à biodiversidade e aos conhecimentos tradicionais. Editors J. Kleba, and S. Kishi, (Belo Horizonte, BH: Direito. política e sociedade).
Lima, E. C. (2012). A gente é que sabe” ou sobre as coisas katukina (pano). Rev. Antropol. 55 (1), 139–169. doi:10.11606/2179-0892.ra.2012.46962
Lima, E. C. (2014a). Um objeto ou uma técnica? O caso do kampô. Rev. Antropol. UFSCar 6 (2), 21–33. doi:10.52426/rau.v6i2.120
Lima, E. C. (2014b). “A internacionalização do kampô (via ayahuasca): Difusão global e efeitos locais,” in Políticas culturais e povos indígenas. Editors M. C. Cunha, and P. N. Cesarino (São Paulo, SP: Cultura Acadêmica)), 91–112.
Lima, A. P., Magnusson, W. E., Menin, M., Erdtmann, L. K., Rodrigues, D. D. J., Keller, C., et al. (2012). Guia de sapos da Reserva Adolpho Ducke-Amazônia Central. São Paulo: Áttema Design Editorial.
Liu, Y., Shi, D., Wang, J., Chen, X., Zhou, M., Xi, X., et al. (2020). A novel amphibian antimicrobial peptide, phylloseptin-PV1, exhibits effective anti-staphylococcal activity without inducing either hepatic or renal toxicity in mice. Front. Microbiol 11, 565158. doi:10.3389/fmicb.2020.565158
Liu, H., Guo, X., Yi, T., Zhu, Y., Ren, X., Guo, R., et al. (2021). Frog skin derived peptides with potential protective effects on ultraviolet B–induced cutaneous photodamage. Front. Immunol. 12, 2127. doi:10.3389/fimmu.2021.613365
Long, Q., Wang, L., Zhou, M., Wu, Y., and Chen, T. (2018). A novel peptide Phylloseptin‐PB u from Phyllomedusa burmeisteri possesses insulinotropic activity via potassium channel and GLP‐1 receptor signalling. J. Cell. Mol. Med. 22 (5), 2804–2814. doi:10.1111/jcmm.13573
Lopes, L. A. (2005). Kambô, a medicina da floresta (experiência narrativa). [Monography][São Paulo (SP)]. University of São Paulo.
Lovejoy, D. A., and Balment, R. J. (1999). Evolution and physiology of the corticotropin-releasing factor (CRF) family of neuropeptides in vertebrates. General Comp. Endocrinol. 115 (1), 1–22. doi:10.1006/gcen.1999.7298
Majić, T., Sauter, M., Bermpohl, F., and Schmidt, T. T. (2021). Connected to the spirit of the frog: An Internet-based survey on Kambô, the secretion of the Amazonian Giant Maki Frog (Phyllomedusa bicolor): Motivations for use, settings and subjective experiences. J. Psychopharmacol. Oxf. Engl. 35 (4), 421–436. doi:10.1177/0269881121991554
Martins, H. M. (2010). “Os katukina e o kampô: Percursos de um "conhecimento tradicional" indígena,” in Conhecimentos tradicionais para o século XXI: Etnografias da intercientificidade. Editors P. E. Little, and D. F. Braília (Brasilia: IEB Press), 35–73.
Martins, M., and Oliveira, M. E. (1998). Natural history of snakes in forests of the Manaus region, Central Amazonia, Brazil. Herpetol. Nat. Hist. 6 (2), 78–150.
Mathison, R., and Solomos, D. (1985). Interaction of tachykinins with an adrenergic receptor in the rat urinary bladder. Eur. J. Pharmacol. 109 (3), 327–333. doi:10.1016/0014-2999(85)90392-9
Menin, M., Waldez, F., and Lima, A. P. (2008). Temporal variation in the abundance and number of species of frogs in 10,000 ha of a forest in Central Amazonia, Brazil. S. Am. J. Herpetology 3, 682–781. doi:10.2994/1808-9798(2008)3[68:TVITAA]2.0.CO;2
Menocchi, S. (2008). Investigada morte de homem que tomou “vacina do sapo”. Estadão (São Paulo). Available at: https://www.estadao.com.br/noticias/geral,investigada-morte-de-homem-que-tomou-vacina-do-sapo, 162933
Mignogna, G., Severini, C., Simmaco, M., Negri, L., Erspamer, G. F., Kreil, G., et al. (1992). Identification and characterization of two dermorphins from skin extracts of the Amazonian frog Phyllomedusa bicolor. FEBS Lett. 302 (2), 151–154. doi:10.1016/0014-5793(92)80427-I
Moen, D. S., and Wiens, J. J. (2009). Phylogenetic evidence for competitively driven divergence: Body-size evolution in caribbean treefrogs (hylidae: Osteopilus). Evolution 63, 195–214. doi:10.1111/j.1558-5646.2008.00538.x
Montecucchi, P. C., Henschen, A., and Erspamer, V. (1979). Structure of sauvagine, a vasoactive peptide from the skin of a frog. Struct. Activity Nat. Peptides 360, 1178. doi:10.1515/9783110861631
Montecucchi, P. C., de Castiglione, R., Piani, S., Gozzini, L., and Erspamer, V. (1981a). Amino acid composition and sequence of dermorphin, a novel opiate‐like peptide from the skin of Phyllomedusa sauvagei. Int. J. Peptide Protein Res. 17 (3), 275–283. doi:10.1111/j.1399-3011.1981.tb01993.x
Montecucchi, P. C., de Castiglione, R., and Erspamer, V. (1981b). Identification of dermorphin and Hyp6‐dermorphin in skin extracts of the Brazilian frog Phyllomedusa rhodei. Int. J. Peptide Protein Res. 17 (3), 316–321. doi:10.1111/j.1399-3011.1981.tb01997.x
Mor, A., Van Huong, N., Delfour, A., Migliore-Samour, D., and Nicolas, P. (1991). Isolation, amino acid sequence and synthesis of dermaseptin, a novel antimicrobial peptide of amphibian skin. Biochemistry 30 (36), 8824–8830.
Mor, A., Amiche, M., and Nicolas, P. (1994a). Structure, synthesis, and activity of dermaseptin b, a novel vertebrate defensive peptide from frog skin: Relationship with adenoregulin. Biochemistry 33 (21), 6642–6650. doi:10.1021/bi00187a034
Mor, A., Chartrel, N., Vaudry, H., and Nicolas, P. (1994b). Skin peptide tyrosine-tyrosine, a member of the pancreatic polypeptide family: Isolation, structure, synthesis, and endocrine activity. Proc. Natl. Acad. Sci. 91 (22), 10295–10299. doi:10.1073/pnas.91.22.10295
Mor, A., Khaled, H., and Pierre, N. (1994c). The vertebrate peptide antibiotics dermaseptins have overlapping structural features but target specific microorganisms. J. Biol. Chem. 269 (50), 31635–31641. doi:10.1016/S0021-9258(18)31742-3
Morais, D. R., Lanaro, R., Barbosa, I. L., Santos, J. M., Cunha, K. F., Hernandes, V. V., et al. (2018). Ayahuasca and Kambo intoxication after alternative natural therapy for depression, confirmed by mass spectrometry. Forensic Toxicol. 36 (1), 212–221. doi:10.1007/s11419-017-0394-5
Mota, E. P., Kaefer, I. L., da Silva Nunes, M., Lima, A. P., and Farias, I. P. (2020). Hidden diversity within the broadly distributed Amazonian giant monkey frog (Phyllomedusa bicolor: Phyllomedusidae). Amphibia-Reptilia 41 (3), 349–359. doi:10.1163/15685381-bja10003
Nakata, N., Sakurada, S., Sakurada, T., Kisara, K., Sasaki, Y., and Suzuki, K. (1986). Physical dependence of a dermorphin tetrapeptide analog, [D-Arg2, Sar4]-dermorphin (1–4) in the rat. Pharmacol. Biochem. Behav. 24 (1), 27–31. doi:10.1016/0091-3057(86)90039-0
Naot, D., Musson, D. S., and Cornish, J. (2018). The activity of peptides of the calcitonin family in bone. Physiol. Rev. 99 (1), 781–805. doi:10.1152/physrev.00066.2017
Neckel-Oliveira, S., and Wachlevski, M. (2004). Predation on the arboreal eggs of three species of Phyllomedusa in Central Amazônia. Journal of Herpetology 38 (2), 244–248. doi:10.1670/162-03A
Neckel-Sliveira, S., and Wachlevski, M. (2004). Predation on the arboreal eggs of three species of Phyllomedusa in Central Amazonia. J. Herpetology 38 (2), 244–248. doi:10.1670/162-03A
Negri, L., Erspamer, G. F., Severini, C., Potenza, R. L., Melchiorri, P., and Erspamer, V. (1992). Dermorphin-related peptides from the skin of Phyllomedusa bicolor and their amidated analogs activate two μ opioid receptor subtypes that modulate antinociception and catalepsy in the rat. Proc. Natl. Acad. Sci. 89 (15), 7203–7207. doi:10.1073/pnas.89.15.7203
Nicolas, P., and Amiche, M. (2006). “The dermaseptins,” in Handbook of biologically active peptides (Academic Press), 295–304. doi:10.1016/B978-012369442-3/50048-9
Nicolas, P., and El Amri, C. (2009). The dermaseptin superfamily: A genebased combinatorial library of antimicrobial peptides. Biochimica Biophysica Acta 1788 (8), 1537–1550. doi:10.1016/j.bbamem.2008.09.006
Nicolas, P., and Mor, A. (1995). Peptides as weapons against microorganisms in the chemical defense system of vertebrates. Annu. Rev. Microbiol. 49, 277–304. doi:10.1146/annurev.mi.49.100195.001425
Peleg Hasson, S., Shachar, E., Kessner, R., Shpigel, S., and Wolf, I. (2021). Kambô-induced systemic inflammatory response: A case report of acute disease progression of cholangiocarcinoma. Integr. Cancer Ther. 20, 1534735421999106. doi:10.1177/1534735421999106
Pescatore, R., Marrone, G. F., Sedberry, S., Vinton, D., Finkelstein, N., Katlowitz, Y. E., et al. (2015). Synthesis and pharmacology of halogenated δ -opioid-selective [d-Ala(2)]deltorphin II peptide analogues. ACS Chem. Neurosci. 6 (6), 905–910. doi:10.1021/acschemneuro.5b00060
Pierre, T. N., Seon, A. A., Amiche, M., and Nicolas, P. (2000). Phylloxin, a novel peptide antibiotic of the dermaseptin family of antimicrobial/opioid peptide precursors. Eur. J. Biochem. 267 (2), 370–378. doi:10.1046/j.1432-1327.2000.01012.x
Pinto, P., and Amésquita, A. (2022). Coloration, toxicity, and vertical distribution in larval phyllomedusine frogs: Is there an anti-predator syndrome? Authorea. doi:10.22541/au.164864450.06373836
Pinto, R. M. C., Py-Daniel, S. S., and Menin, M. (2013). Redescription of the tadpole of Phyllomedusa bicolor (Anura: Hylidae) from central amazonia. S. Am. J. Herpetology 8 (1), 67–72. doi:10.2994/SAJH-D-13-00003.1
Pogorzelska, J., and Łapiński, T. W. (2017). Toxic hepatitis caused by the excretions of the Phyllomedusa bicolor frog–a case report. Clin. Exp. Hepatology 3 (1), 33–34. doi:10.5114/ceh.2017.65228
Raaymakers, C., Verbrugghe, E., Stijlemans, B., Martel, A., Pasmans, F., and Roelants, K. (2018). The anuran skin peptide bradykinin mediates its own absorption across epithelial barriers of the digestive tract. Peptides 103, 84–89. doi:10.1016/j.peptides.2018.03.010
Resende, J. M., Moraes, C. M., Prates, M. V., Cesar, A., Almeida, F. C., Mundim, N. C., et al. (2008). Solution NMR structures of the antimicrobial peptides phylloseptin-1, -2, and-3 and biological activity: The role of charges and hydrogen bonding interactions in stabilizing helix conformations. Peptides 29 (10), 1633–1644. doi:10.1016/j.peptides.2008.06.022
Ribeiro, F. S. (2017). O Kambô na rede: Divulgação de uma prática tradicional indígena na internet [Monography]. [Curitiba (PR)]. Federal University of Paraná.
Richter, K., Egger, R., and Kreil, G. (1990). Molecular cloning of a cDNA encoding the bombesin precursor in skin of Bombina variegata. FEBS Lett. 262 (2), 353–355. doi:10.1016/0014-5793(90)80227-A
Rocha, S. M. C. da, Lima, A. P., and Kaefer, I. L. (2021). Key roles of paternal care and climate on offspring survival of an Amazonian poison frog. An. Acad. Bras. Ciências 93 (3). doi:10.1590/0001-3765202120210067
Rödel, M. O., Brede, C., Hirschfeld, M., Schmitt, T., Favreau, P., Stöcklin, R., et al. (2013). Chemical camouflage–a frog's strategy to co-exist with aggressive ants. PLoS One 8 (12), e81950. doi:10.1371/journal.pone.0081950
Rodríguez, A., Poth, D., Schulz, S., and Vences, M. (2011). Discovery of skin alkaloids in a miniaturized eleutherodactylid frog from Cuba. Biol. Lett. 7 (3), 414–418. doi:10.1098/rsbl.2010.0844
Rodríguez, L. O., and Duellman, W. E. (1994). Guide to the frogs of the iquitos region, amazonian Peru: Lawrence: Associacion de ecologia y conservacion, Amazon Center for environmental education and research. Natural History Museum, University of Kansas.
Roy, R., Baranwal, A., and Espiridion, E. D. (2018). Can overuse of kambô cause psychosis? Cureus 10 (6), e2770. doi:10.7759/cureus.2770
Ruibal, R., and Shoemaker, V. (1984). Osteoderms in anurans. J. Herpetology 18 (3), 313–328. doi:10.2307/1564085
Sacco, M. A., Zibetti, A., Bonetta, C. F., Scalise, C., Abenavoli, L., Guarna, F., et al. (2022). Kambo: Natural drug or potential toxic agent? A literature review of acute poisoning cases. Toxicol. Rep. 9, 905–913. doi:10.1016/j.toxrep.2022.04.005
Santos, C., Hamadat, S., Le Saux, K., Newton, C., Mazouni, M., Zargarian, L., et al. (2017). Studies of the antitumor mechanism of action of dermaseptin B2, a multifunctional cationic antimicrobial peptide, reveal a partial implication of cell surface glycosaminoglycans. PLoS One 12 (8), e0182926. doi:10.1371/journal.pone.0182926
Saporito, R. A., Donnelly, M. A., Spande, T. F., and Garraffo, H. M. (2012). A review of chemical ecology in poison frogs. Chemoecology 22 (3), 159–168. doi:10.1007/s00049-011-0088-0
Saporito, R. A., Spande, T. F., and Garraffo, H. M. (2009). Arthropod alkaloids in poison frogs: A review of the 'dietary hypothesis. Heterocycles 79 (1), 277–297. doi:10.3987/REV-08-SR(D)11
Sazima, I. (1974). Experimental predation on the leaf-frog Phyllomedusa rohdei by the water snake Liophis militaris. J. Herpetology 8 (4), 376–377.
Schäffer, D. A., and Gábriel, R. (2005). Two major tachykinins, substance P and substance K, are localized to distinct subsets of amacrine cells in the anuran retina. Neurosci. Lett. 386 (3), 194–198. doi:10.1016/j.neulet.2005.06.011
Schmidt, T. T., Reiche, S., Hage, C. L., Bermpohl, F., and Majić, T. (2020). Acute and subacute psychoactive effects of kambô, the secretion of the amazonian giant maki frog (Phyllomedusa bicolor): Retrospective reports. Sci. Rep. 10 (1), 1–11. doi:10.1038/s41598-020-78527-4
Seon, A. A., Pierre, T. N., Redeker, V., Lacombe, C., Delfour, A., Nicolas, P., et al. (2000). Isolation, structure, synthesis, and activity of a new member of the calcitonin gene-related peptide family from frog skin and molecular cloning of its precursor. J. Biol. Chem. 275 (8), 5934–5940. doi:10.1074/jbc.275.8.5934
Severini, C., Improta, G., Falconieri-Erspamer, G., Salvadori, S., and Erspamer, V. (2002). The tachykinin peptide family. Pharmacol. Rev. 54 (2), 285–322. doi:10.1124/pr.54.2.285
Shai, Y. (1995). Molecular recognition between membrane-spanning polypeptides. Trends Biochem. Sci. 20 (11), 460–464. doi:10.1016/S0968-0004(00)89101-X
Shine, R. (1979). Sexual selection and sexual dimorphism in Amphibia. Copeia 1979 (2), 297–306. doi:10.2307/1443418
Shiva, V. (1991). Biotechnology development and conservation of biodiversity. Econ. Political Wkly. 26 (48), 2740–2746.
Silva, F. V. A. D., Monteiro, W. M., and Bernarde, P. S. (2019). Kambô” frog (Phyllomedusa bicolor): Use in folk medicine and potential health risks. Rev. Soc. Bras. Med. Trop. 52. doi:10.1590/0037-8682-0467-2018
Silva, Y. B. S., Almeida-Santos, W., Araújo, A. S., and Costa-Campos, C. E. (2020). Notes on the reproductive biology of Phyllomedusa bicolor (Anura, Phyllomedusidae) in the Amazon forest of northern Brazil. Herpetol. Notes 13, 931–935.
Souza, M. B. (2009). Anfíbios: Reserva Extrativista do Alto Juruá e Parque Nacional da Serra do Divisor, Acre. Campinas: Federal University of Acre, 77.
Souza, M. B., Aquino, T. V., Lima, E. C., and Mendes, M. K. (2002). “Anfíbios,” in Enciclopédia da floresta: O alto Juruá: Práticas e conhecimento das populações. 1ed. Editors M. C. Cunha, and M. B. Almeida Orgs (São Paulo: Companhia das Letras), 601-614.
Steinhoff, M. S., von Mentzer, B., Geppetti, P., Pothoulakis, C., and Bunnett, N. W. (2014). Tachykinins and their receptors: Contributions to physiological control and the mechanisms of disease. Physiol. Rev. 94 (1), 265–301. doi:10.1152/physrev.00031.2013
Tastevin, C. (1925). Le fleuve murú: Ses habitants: Croyances et moeurs kachinaua. Société Géogr. 43, 401–422.
Thibaudeau, G., and Altig, R. (2012). Coloration of anuran tadpoles (Amphibia): Development, dynamics, function, and hypotheses. Int. Sch. Res. Netw. Zoology, 1–16. doi:10.5402/2012/725203
Thompson, C., Malcolm, B., and Tegzes, J. (2022). Use of Phyllomedusa bicolour secretion during kambô ritual: Observational responses, dosage, and risk of adverse events. Toxicol. Commun. 6 (1), 6–12. doi:10.1177/23978473221085746
Thompson, C., and Williams, M. L. (2022). Review of the physiological effects of Phyllomedusa bicolor skin secretion peptides on humans receiving Kambô. Toxicol. Res. Appl. 6, 23978473221085746.
Tiwari, V. (2019). Post-translational modification of ESKAPE pathogens as a potential target in drug discovery. Drug Discov. Today 24 (3), 814–822. doi:10.1016/j.drudis.2018.12.005
Toledo, L. F., Sazima, I., and Haddad, C. F. (2011). Behavioural defences of anurans: An overview. Ethol. Ecol. Evol. 23 (1), 1–25. doi:10.1080/03949370.2010.534321
Toledo, R. C., and Jared, C. (1993). Cutaneous adaptations to water balance in amphibians. Comp. Biochem. Physiology Part A Physiology 105 (4), 593–608. doi:10.1016/0300-9629(93)90259-7
Toledo, R. D., and Jared, C. (1995). Cutaneous granular glands and amphibian venoms. Comp. Biochem. Physiology Part A Physiology 111 (1), 1–29. doi:10.1016/0300-9629(95)98515-I
Tsuji-Nishikido, B. M., and Menin, M. (2011). Distribution of frogs in riparian areas of an urban forest fragment in Central Amazonia. Biota Neotropica 11 (2), 63–70. doi:10.1590/S1676-06032011000200007
van Zoggel, H., Hamma-Kourbali, Y., Galanth, C., Ladram, A., Nicolas, P., Courty, J., et al. (2012). Antitumor and angiostatic peptides from frog skin secretions. Amino Acids 42 (1), 385–395. doi:10.1007/s00726-010-0815-9
Vanhoye, D., Bruston, F., El Amri, S., Ladram, A., Amiche, M., and Nicolas, P. (2004). Membrane association, electrostatic sequestration, and cytotoxicity of Gly-Leu-rich peptide orthologs with differing functions. Biochemistry 43 (26), 8391–8409. doi:10.1021/bi0493158
Venâncio, N. M., and Melo-Sampaio, P. R. (2010). Reproductive behavior of the giant leaf frog Phyllomedusa bicolor (Anura: Hylidae) in the Western Amazon. Phyllomedusa J. Herpetology 9 (1), 63–67. doi:10.11606/issn.2316-9079.v9i1p63-67
Wagler, J. (1830). Adaptative plasticity in hatching age: A response to predation risk tradeoffs Natürliches system der Amphibien, mit vorangehender Classification der Säugthiere und Vägel. München, J.G. Cotta vi + 354
Warkentin, K. M. (1995). Adaptative plasticity in hatching age: A response to predation risk tradeoffs. Proc. Natl. Acad. Sci. 92 (8), 3507–3510. doi:10.1073/pnas.92.8.3507
Wiens, J. J., Fetzner, J. W., Parkinson, C. L., and Reeder, T. W. (2005). Hylid frog phylogeny and sampling strategies for speciose clades. Syst. Biol. 54 (5), 719–748. doi:10.1080/10635150500234625
Wiens, J. J., Graham, C. H., Moen, D. S., Smith, S. A., and Reeder, T. W. (2006). Evolutionary and ecological causes of the latitudinal diversity gradient in hylid frogs: Treefrog trees unearth the roots of high tropical diversity. Am. Nat. 168, 579–596. doi:10.1086/507882
Williams, C. R., Brodie, E. D., Tyler, M. J., and Walker, S. J. (2000). Antipredator mechanisms of Australian frogs. J. Herpetology 34 (3), 431–443. doi:10.2307/1565367
Keywords: bicoulored tree-frog, giant leaf frog, giant monkey frog, kapum, kampô, two-colored leaf frog, vacina do sapo, frog vaccine
Citation: Nogueira TAC, Kaefer IL, Sartim MA, Pucca MB, Sachett J, Barros AL, Júnior MBA, Baía-da-Silva DC, Bernarde PS, Koolen HHF and Monteiro WM (2022) The Amazonian kambô frog Phyllomedusa bicolor (Amphibia: Phyllomedusidae): Current knowledge on biology, phylogeography, toxinology, ethnopharmacology and medical aspects. Front. Pharmacol. 13:997318. doi: 10.3389/fphar.2022.997318
Received: 18 July 2022; Accepted: 24 August 2022;
Published: 06 October 2022.
Edited by:
Yucheng Xiao, Indiana University, United StatesReviewed by:
Liping Jiang, Central South University, ChinaMeichun Deng, Central South University, China
Copyright © 2022 Nogueira, Kaefer, Sartim, Pucca, Sachett, Barros, Júnior, Baía-da-Silva, Bernarde, Koolen and Monteiro. This is an open-access article distributed under the terms of the Creative Commons Attribution License (CC BY). The use, distribution or reproduction in other forums is permitted, provided the original author(s) and the copyright owner(s) are credited and that the original publication in this journal is cited, in accordance with accepted academic practice. No use, distribution or reproduction is permitted which does not comply with these terms.
*Correspondence: Igor Luis Kaefer, kaeferigor@gmail.com; Wuelton M. Monteiro, wueltonmm@gmail.com