Stem Cells as Therapy for Necrotizing Enterocolitis: A Systematic Review and Meta-Analysis of Preclinical Studies
- 1Department of Pediatrics, Maastricht University Medical Center (MUMC+), School for Oncology and Developmental Biology (GROW), Maastricht, Netherlands
- 2Department for Health Evidence Unit SYRCLE, Radboud University Medical Center, Nijmegen, Netherlands
Background: Necrotizing enterocolitis (NEC) is the most common life-threatening gastrointestinal condition among very and extremely preterm infants. Stem cell therapy has shown some promising protective effects in animal models of intestinal injury, including NEC, but no systematic review has yet evaluated the preclinical evidence of stem cell therapy for NEC prevention or treatment.
Methods: PubMed and EMBASE databases were searched for studies using an animal model of NEC with stem cells or their products. The SYRCLE tool was used for the assessment of risk of bias. A random-effects model was used to pool odds ratios (ORs) and 95% confidence interval (CI).
Results: We screened 953 studies, of which nine (eight rat and one mouse models) met the inclusion criteria. All animal models induced NEC by a combination of hypothermia, hypoxia, and formula feeding. Risk of bias was evaluated as unclear on most items for all studies included. Meta-analysis found that both mesenchymal and neural stem cells and stem cell-derived exosomes reduced the incidence of all NEC (OR 0.22, 95% CI 0.16–0.32, k = 16), grade 2 NEC (OR 0.41, 95% CI 0.24–0.70, k = 16), and grade 3–4 NEC (OR 0.28, 95% CI 0.19–0.42, k = 16). k represents the number of independent effect sizes included in each meta-analysis. The effect of the exosomes was similar to that of the stem cells. Stem cells and exosomes also improved 4-day survival (OR 2.89 95% CI 2.07–4.04, k = 9) and 7-day survival (OR 3.96 95% CI 2.39–6.55, k = 5) after experimental NEC. Meta-analysis also found that stem cells reduced other indicators of intestinal injury.
Conclusion: The data from this meta-analysis suggest that both stem cells and stem cell-derived exosomes prevented NEC in rodent experimental models. However, unclear risk of bias and incomplete reporting underline that poor reporting standards are common and hamper the reliable interpretation of preclinical evidence for stem cell therapy for NEC.
Introduction
Necrotizing enterocolitis (NEC) is the most common life-threatening gastrointestinal condition among very and extremely preterm infants (1–4). Severe NEC is characterized by full-thickness destruction of the intestine leading to intestinal perforation, peritonitis, bacterial invasion of blood stream, and generalized infection (1–4). NEC has a multifactorial etiology that is largely related to prematurity and the consequent immaturity of the gastrointestinal tract (1–10). However, besides low gestational age (GA), several risk factors such as formula feeds, gut dysbiosis, infection, or intestinal hypoperfusion have been implicated in the etiopathogenesis of NEC (1–10). Furthermore, a clear definition of NEC remains elusive because NEC likely represents different conditions with intestinal injury or necrosis as final outcome (4, 11).
A number of animal models have been developed to investigate the pathophysiology of NEC and to test different preventive and therapeutic strategies (12–18). The most widely used experimental animals are rats, mice, and piglets (12–18). The experimental models try to reproduce the pathogenesis of NEC using one or a combination of potential contributory factors such as intestinal immaturity, formula feeding, bacteria and/or their byproduct, and hypoxic–ischemic stress (12–18). However, after more than five decades of preclinical and clinical research on NEC, the promise of a “magic bullet” for the prevention and/or treatment of NEC has yet to become a reality (11).
Stem cell therapy is increasingly proposed as a novel therapeutic approach for a number of complications of prematurity such as bronchopulmonary dysplasia (19–22), brain injury (22, 23), or retinopathy of prematurity (24) with encouraging preclinical results auspicious for clinical translation (19, 21, 22). A growing number of preclinical studies have investigated the potential therapeutic role of stem cells in experimental NEC (13–21). However, to use stem cell therapy, we need to answer five questions: which cells to give, at what time, via which route, in which dose, and to which babies (25).
Systematic reviews and meta-analyses are common practice in human clinical research, particularly for randomized controlled trials. Although most animal experiments are performed to enrich our clinical understanding of human diseases, systematic reviews of preclinical studies are still scarce (26, 27). Meta-analyses of preclinical studies can be used to inform clinical trial design, to understand the possible discrepancies between findings from preclinical and clinical studies and to improve the quality and translational utility of animal experimentation (28–30). To date, there has been no systematic review and/or meta-analysis on the therapeutic potential of stem cells in experimental NEC. We aimed to provide a comprehensive overview of all studies using stem cells in animal models of NEC and to quantify the effects of stem cells in mortality and development of intestinal injury.
Methods
Protocol
A protocol was registered on PROSPERO (CRD42018110084) before starting the review (31). We used the Preferred Reporting Items for Systematic Reviews and Meta-Analyses (PRISMA) checklist for the manuscript (32).
Sources and Search Strategy
We searched PubMed and EMBASE databases for original, preclinical studies concerning the effects of stem cells or stem cell products on NEC published until June 2019. The search strategy involved the following four search components: stem cells (and all synonyms) AND (necrotizing enterocolitis OR [neonate AND (intestinal injury OR necrosis)] AND animals (33, 34) (for our complete search strategy, see S 1). No language or date restrictions were applied. Electronic search alerts were set up using Google Scholar to be able to include any studies published after the last search.
Inclusion Criteria and Study Selection
Studies were included in this systematic review when they met all of the following criteria, defined a priori in our published protocol: (1) original in vivo animal studies using a neonatal experimental NEC model; (2) intervention was randomized, quasi-randomized, or non-randomized; (3) tested as intervention stem cells or stem cell-derived products; and (4) reported on any of the following outcome measures: survival, NEC incidence and/or severity, intestinal injury, weight gain, and clinical sickness scores. Non-interventional studies, studies without controls, and non-neonatal models of intestinal injury were excluded.
The primary outcome was NEC incidence. Secondary outcomes were survival and severity of NEC. Therefore, studies that reported on survival, NEC incidence (determined histologically), and/or severity of NEC (determined histologically and with predetermined scales) were included.
Two independent reviewers (TH and EV-M) screened all studies for inclusion. The first screening was based on title and abstract using Rayyan RCI software (35). In case of doubt, the full text of the article was evaluated. The full text of all preselected publications was subsequently assessed by two independent reviewers (TH and EV-M). Studies were included when they met all the inclusion criteria. Disagreements about inclusion were resolved through discussion and consensus among three reviewers (TH, EV-M, and CH).
One reviewer (TH) extracted the data using a predetermined data extraction sheet. A second reviewer (EV-M) checked data for accuracy. Data were extracted for study characteristics (authors, year of publication, and study location), study design (sample size for intervention, control, and sham groups), intervention characteristics (timing, dose, and mode of stem cell administration), and outcome measures (primary and secondary outcomes as described above). Dichotomous and continuous data provided in numbers were extracted directly. Data on survival and NEC incidence/severity were converted to odds ratios (ORs), and data on intestinal injury (intestinal permeability and motility), weight, and sickness/severity scores were converted to standardized differences in means. If only graphs were available, Web Plot Digitizer was used to extract numerical values (36).
Risk of Bias
We used the SYRCLE tool (37) to assess the risk of bias in the included studies. Two reviewers (TH and EV-M) independently evaluated the studies, and discrepancies in scoring were resolved through discussion. A “yes” score indicates low risk of bias; a “no” score indicates high risk of bias; and a “?” score indicates unknown risk of bias.
In order to avoid the problem of too many items being evaluated as “unclear risk of bias” due to lack of reporting of experimental details on animals, methods, and materials (38), we added three items on reporting and evaluated whether studies reported (1) any measure of randomization, (2) any measure of blinding, and (3) a sample size calculation. For these three items, a “yes” score indicates “reported,” and a “no” score indicates “unreported.”
Meta-Analysis, Subgroup Analyses, and Publication Bias
Studies were combined and analyzed using Comprehensive Meta-Analysis V3.0 software (Biostat Inc., Englewood, NJ, USA). The unit of analysis for the meta-analysis (represented as k) was the individual experiments (i.e., one reference could contain multiple independent experiments). All outcome measures that were reported in more than one study were included in meta-analyses. For dichotomous outcomes, the OR with 95% confidence interval (CI) was calculated from the data provided in the studies. For continuous outcomes, we extracted the mean, SD, and n to calculate the standardized mean difference (SMD). If studies used a single control group and multiple experimental groups, we corrected for this by dividing the sample size of the control group by the number of experimental groups. When one of the cells contained a zero value or the risk in either the control or experimental group was 100%, we added 0.5 to each cell to calculate the OR.
Due to anticipated heterogeneity, summary statistics were calculated with a random-effects model. This model accounts for variability between studies as well as within studies. Statistical heterogeneity was assessed by Cochran's Q statistic and by the I2 statistic, which is derived from Q and describes the proportion of total variation that is due to heterogeneity beyond chance (39).
Subgroup analyses were predefined in the protocol and only carried out if there were at least three independent comparisons per subgroup (k ≥ 3). The criteria we planned on using for subgroup analyses included cell-type, animal/species, stem cells vs. cell-derived products, control group (placebo/sham vs. no intervention), and definition of neonatal (term vs. preterm model). Subgroup analyses were conducted according to the mixed-effects model (40). In this model, a random-effects model is used to combine studies within each subgroup, and a fixed-effects model is used to combine subgroups and yield the overall effect. We expected the variance to be comparable within the subgroups; therefore, we assumed a common among-study variance across subgroups. For subgroup analyses, we adjusted our significance level according to the conservative Bonferroni method to account for multiple analyses (p/number of comparisons).
Publication bias analysis was carried out for the outcomes with k > 10. We used visual inspection of the funnel plot, Duval and Tweedie's trim and fill, and Egger's regression test to assess publication bias (40).
Results
Study Characteristics and Experimental Models
After duplicates were removed, 953 references needed to be screened for eligibility. Figure 1 shows the PRISMA diagram of the comprehensive search and the reasons for excluding studies. Finally, nine studies could be included in this systematic review (13–21), which encompassed 23 comparisons in total. We did not find additional studies after the search through electronic alerts (until December 2019). Six of the included studies (41–46) were carried out in the same research facility in Columbus, Ohio (USA). One study (47) was carried out in Turkey, and two studies were carried out in the United Kingdom (48, 49). The characteristics of included studies are summarized in Table 1. Eight studies used rats, and the study of Wei et al. used mice. All included studies used the method of Barlow et al. (50) for inducing experimental NEC through formula feeding, hypoxia, and hypothermia.
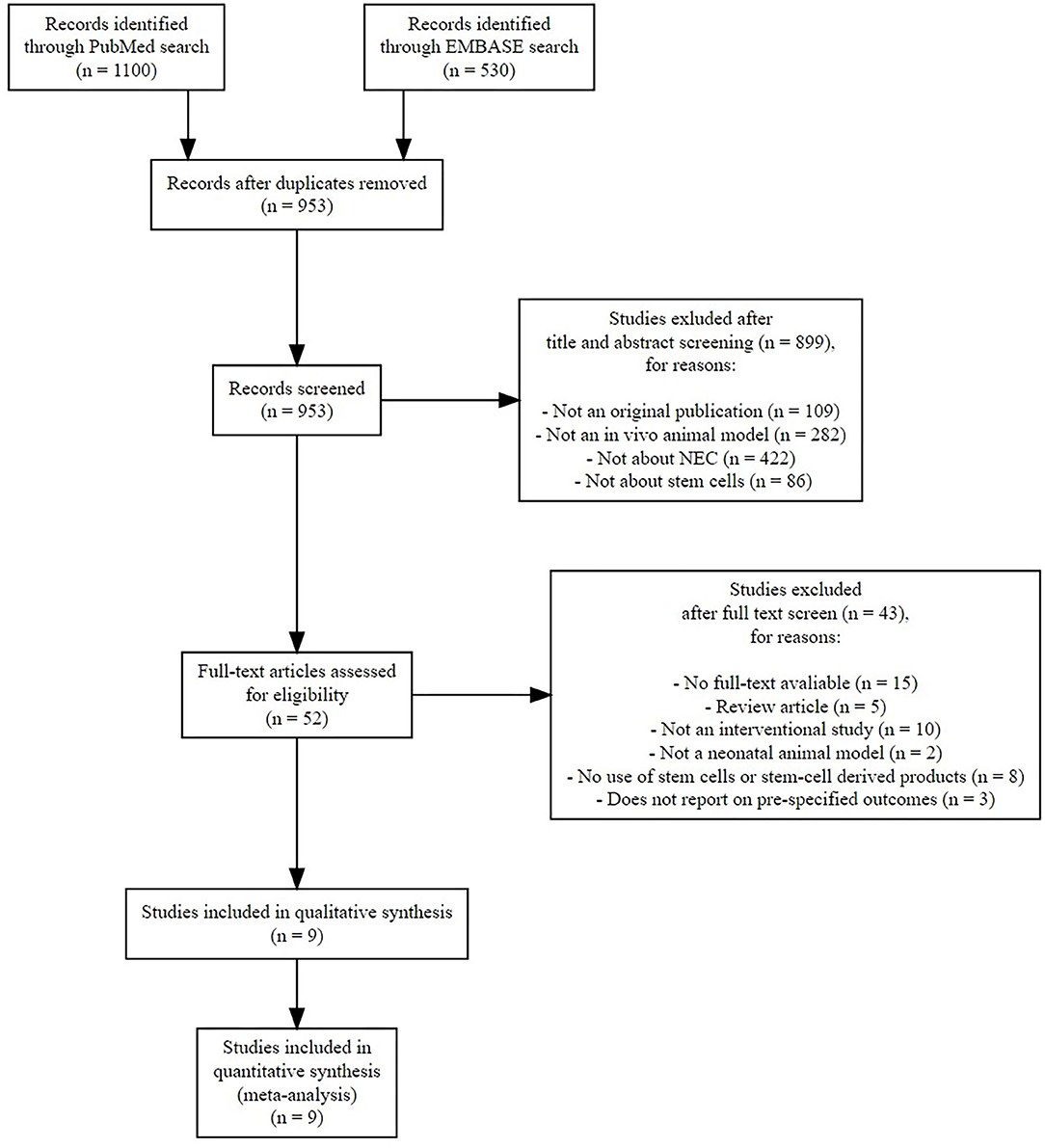
Figure 1. Preferred Reporting Items for Systematic Reviews and Meta-Analyses (PRISMA) flow diagram of systematic search.
Intervention: Stem Cells and Stem Cell-Derived Products
As shown in Table 1, seven studies examined stem cells as the intervention (42, 44–49), one study examined both stem cells and exosomes (43), and one study examined only exosomes as intervention (41). Regarding the type of stem cells, one study (one experiment) (42) examined amniotic fluid (AF) mesenchymal stem cells (MSCs), and two studies (three experiments) (48, 49) examined AF stem cells (AFSCs). AFSCs stained positively for a number of surface markers that are characteristic of MSCs and/or neural stem cells (NSCs) (51, 52) and therefore were analyzed separately from AFMSCs. Five studies (seven experiments) (42, 43, 45, 47, 48) examined bone marrow MSCs (BMMSCs), three studies (three experiments) (42, 44, 46) examined enteric NSCs (ENSCs), and one study (one experiment) (42) examined AFNSCs. Two studies studied exosomes of stem cells as the intervention, with McCulloh et al. (41) studying exosomes of AFMSCs, BMMSCs, ENSCs, and AFNSCs in two experiments, and Rager et al. (43) studying BMMSC exosomes in one experiment. The dosages and timing for each intervention are detailed in Table 1.
Reported Outcomes
Primary Outcomes
Four studies (16 experiments) (41–43, 45) reported on incidence of any grade NEC, incidence of grade 2 NEC, and incidence of severe NEC (grades 3 and 4). Five studies (nine experiments) reported on survival at day 4 of life (45–49), and two studies (five experiments) reported on survival at day 7 of life (46, 48).
Secondary Outcomes
Several studies reported on indicators of intestinal injury. Four studies (10 experiments) (42, 43, 45, 48) reported on intestinal permeability. Two studies (two experiments) reported on intestinal motility (46, 49). Two studies (two experiments) reported on clinical sickness (47, 48). One study (one experiment) reported on NEC severity score (48).
Risk of Bias Assessment
Selection Bias and Performance Bias
The details for the risk of bias analysis are shown in Table 2. None of nine included studies provided sufficient information to assess the risk of bias as either low or high risk of bias for selection bias (sequence generation, baseline characteristics, and allocation concealment) and performance bias (random housing and investigator/caregiver blinding). We evaluated all six of these items as “unclear” risk of bias due to poor reporting of essential experimental details.
Detection Bias
We evaluated risk of bias for blinding of outcome assessors (detection bias) separately for each outcome reported, and the details of this evaluation are provided in Table 3. Five studies (41, 42, 44, 45, 47) had low risk of bias for blinding of outcome assessors, for the outcome NEC incidence and severity. One study (48) blinded outcome assessors for intestinal motility and scored “low” risk of bias for this outcome, whereas another (46) did not clarify if outcome assessors were blinded and scored “unclear” risk of bias. For all other outcomes (survival, intestinal permeability, and clinical sickness score), studies did not clarify if outcome assessors were blinded, and they were evaluated as “unclear” risk of bias. No study provided information on random outcome assessment (detection bias), and we evaluated this for all studies as “unclear” risk of bias.
Attrition Bias
Attrition bias (incomplete outcome data) was evaluated as “low” in one study (44), “high” in another study (41), and “unclear” in seven studies (42, 43, 45–49).
Reporting Bias and Other Bias
Reporting bias (selective outcome reporting) was evaluated as “high” in one study (48) and unclear in eight studies (41–47, 49).
We evaluated seven out of nine studies as low risk of “other biases”: there were no indications of unit of analysis errors, and they reported information on funding and conflicts of interest. Two studies did not provide funding and conflict of interest information and were evaluated as “unclear” risk of bias in this category.
Meta-Analyses of Primary Outcomes
Necrotizing Enterocolitis Incidence
As shown in Figure 2, meta-analysis showed that stem cells and stem cell-derived products significantly reduced the incidence of any grade of NEC (k = 16, OR 0.22, 95% CI 0.16–0.32). Meta-analysis also found that stem cells and stem cell-derived products significantly reduced incidence of grade 2 NEC (k = 16, OR 0.41, 95% CI 0.24–0.70, Figure 3), as well as incidence of grade 3–4 NEC (k = 16, OR 0.28, 95% CI 0.19–0.42, Figure 4). As shown in Figures 2–4, the protective effects of stem cells were similarly observed in the subgroups of mesenchymal SC, neural SC, and SC-derived exosomes. Sensitivity analyses showed that the removal of the study that used mice instead of rats did not affect the significance of the results, nor did removal of experiments that used SC-derived exosomes (instead of SC) as the intervention (Supplementary Table 1).
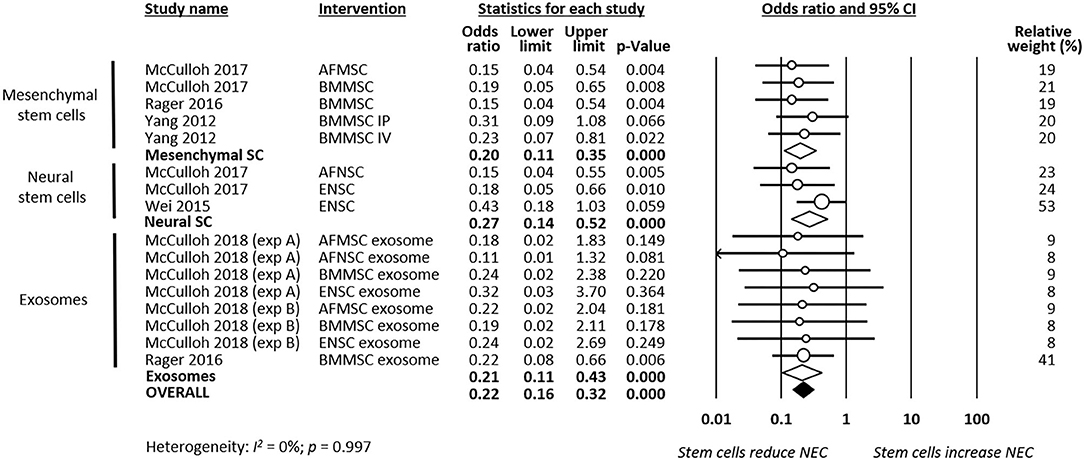
Figure 2. Meta-analysis on stem cells/stem cell-derived products and incidence of any grade necrotizing enterocolitis (NEC). AF, amniotic fluid; BM, bone marrow; ENSC, enteric neural stem cell; IP, intraperitoneal; IV, intravenous; MSC, mesenchymal stem cell; NSC, neural stem cell; SC, stem cell.
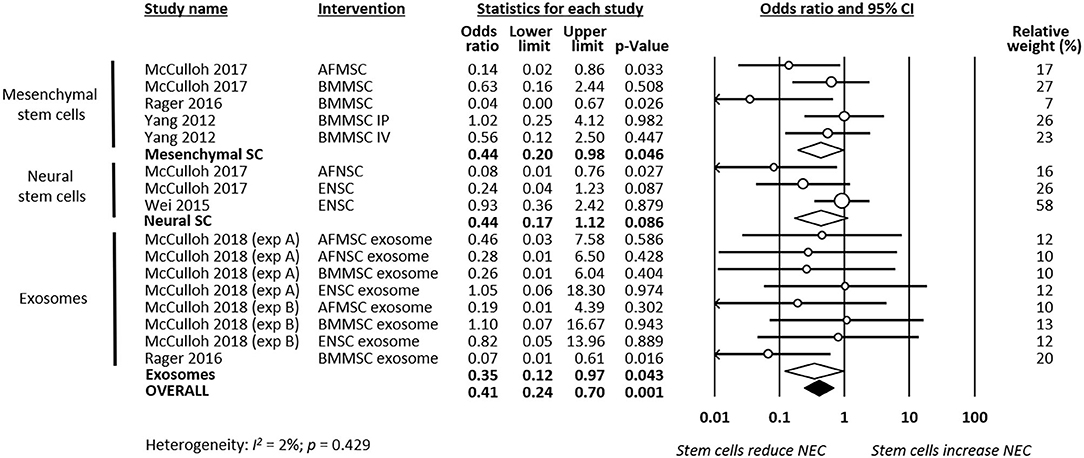
Figure 3. Meta-analysis on stem cells/stem cell-derived products and incidence of grade 2 necrotizing enterocolitis (NEC). AF, amniotic fluid; BM, bone marrow; ENSC, enteric neural stem cell; IP, intraperitoneal; IV, intravenous; MSC, mesenchymal stem cell; NSC, neural stem cell; SC, stem cell.
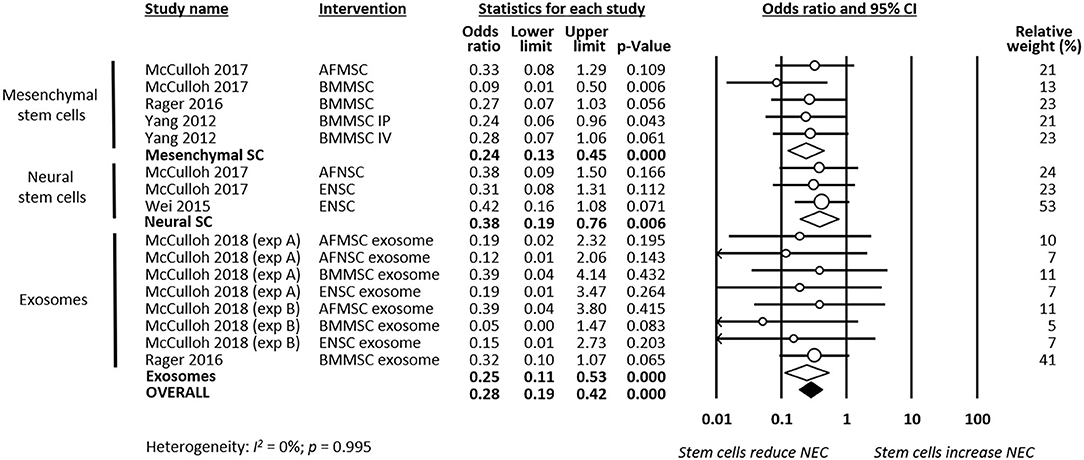
Figure 4. Meta-analysis on stem cells/stem cell-derived products and incidence of grade 3–4 necrotizing enterocolitis (NEC). AF, amniotic fluid; BM, bone marrow; ENSC, enteric neural stem cell; IP, intraperitoneal; IV, intravenous; MSC, mesenchymal stem cell; NSC, neural stem cell; SC, stem cell.
Survival
Meta-analysis showed that stem cells significantly improved survival at day 4 of life (k = 9, OR 2.89, 95% CI 2.07–4.04, Figure 5). Moreover, meta-analysis showed that stem cells significantly improved chance of survival at day 7 of life (k = 5, OR 3.96, 95% CI 2.39–6.55, Figure 6). Subgroup analysis based on the type of stem cells was not performed for this outcome due to the small number of studies in each subgroup.
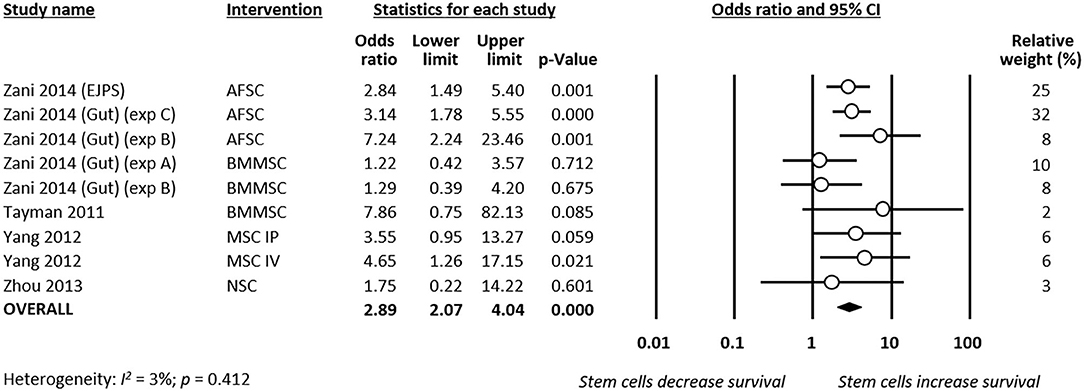
Figure 5. Meta-analysis on stem cells and survival at day 4 of life following necrotizing enterocolitis (NEC). AFSC, amniotic fluid stem cell; BMMSC, bone marrow mesenchymal stem cell; IP, intraperitoneal; IV, intravenous; MSC, mesenchymal stem cell; NSC, neural stem cell.
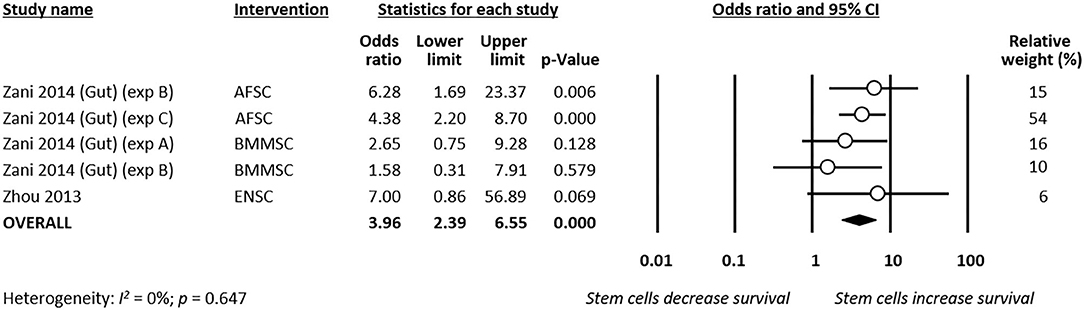
Figure 6. Meta-analysis on stem cells and survival at day 7 of life following necrotizing enterocolitis (NEC). AFSC, amniotic fluid stem cell; BMMSC, bone marrow mesenchymal stem cell; ENSC, enteric neural stem cell.
Meta-Analyses of Secondary Outcomes
Meta-analysis showed that stem cells and stem cell exosomes significantly reduced intestinal permeability (k = 10, SMD −3.48, 95% CI −3.90 to −3.05, Figure 7). Sensitivity analyses showed that the reduction remained significant after removing the sole experiment that used exosomes as the intervention and after removing the sole experiment that used mice as the experimental animal (Supplementary Table 1). Meta-analysis also found that stem cells improved intestinal motility (k = 2, SMD 3.73, 95% CI 3.09–4.38, Supplementary Figure 1) and reduced clinical sickness score (SMD −3.49, 95% CI −6.58 to −0.40, Supplementary Figure 2).
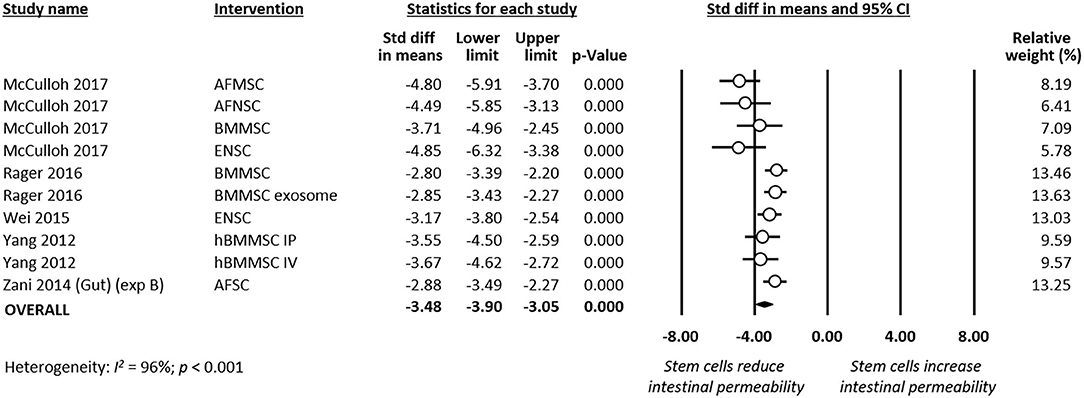
Figure 7. Meta-analysis on stem cells/stem cell-derived products and intestinal permeability following experimental necrotizing enterocolitis (NEC). AF, amniotic fluid; BM, bone marrow; ENSC, enteric neural stem cell; h, human; IP, intraperitoneal; IV, intravenous; MSC, mesenchymal stem cell; SC, stem cell.
Publication Bias
There were sufficient independent effect sizes (k > 10) to test two outcomes (any grade NEC and grade 3–4 NEC incidence) for publication bias. For any grade NEC (k = 16), the visual inspection and trim-and-fill analysis suggested funnel plot asymmetry (Supplementary Figure 3), but Egger's regression test was non-significant (p = 0.090). For the outcome NEC grade 3–4 (k = 16), the visual inspection and trim-and-fill analysis suggested funnel plot asymmetry (Supplementary Figure 4), and Egger's regression test supported the presence of significant publication bias (p < 0.001).
Discussion
To the best of our knowledge, this is the first systematic review and meta-analysis of preclinical studies investigating the effects of stem cells in experimental NEC. Meta-analysis showed that stem cells and stem cell-derived exosomes increased survival and decreased both incidence and severity of histologically proven NEC in rodent models of the condition. The beneficial effects of stem cells were consistent despite the heterogeneity in the sort of cells or exosomes across the different studies.
The studies included in our review used bone marrow- and AF-derived MSCs, and AF- and fetal intestine-derived NSCs. Among non-embryonic stem cells, MSCs are considered to have a high therapeutic potential due to their ability of proliferation and multilineage differentiation (22, 53–55). MSCs are found in multiple tissues, including bone marrow, adipose tissue, placenta, chorion, amnion, umbilical cord, umbilical blood, and breast milk (22, 55). The data of the meta-analysis suggest that MSCs and NSCs have similar effects on experimental NEC. However, NSCs, compared with MSCs, are challenging to isolate and culture, potentially limiting their clinical utility (22). Two studies investigated the effects of AFSC (48, 49). AFSC are considered as an intermediate type between embryonic and adult stem cells, and they stained positively for a number of surface markers characteristic of MSCs and NSCs (51, 52). Interestingly, it has been shown that NSCs can be purified and derived from AFSC. As reviewed by McCulloch et al., these amniotic-fluid derived NSCs may have the ability to provide the same therapeutic effects than other NSCs but with the advantage of a greater easiness in obtaining them (52).
The results from this meta-analysis also suggest that exosomes are just as effective in reducing the incidence and severity of experimental NEC as the stem cells from which they derive. Accordingly, a previous meta-analysis showed that cell-free MSC-derived conditioned media had significant therapeutic effects in hyperoxic rodent models of bronchopulmonary dysplasia (21). Paracrine mediators such as stem cell-derived exosomes are emerging as a novel therapeutic strategy to overcome some of the limitations of stem cell therapy (56). Exosomes are small membrane vesicles of endocytic origin that exert their therapeutic actions by involving cell–cell interactions and transferring proteins, RNAs, and microRNAs (56). Interestingly, breast milk-derived exosomes, which are produced by a variety of cells, stimulate intestinal cell proliferation and differentiation (57, 58) and may be protective in experimental NEC (59).
The strengths of our systematic review included the breadth of the search strategy, the clear definition of inclusion and exclusion criteria, and the rigorous data evaluation and reporting by the use of international guidance and standards. However, the review has also a number of limitations that should be discussed. First, the included studies generally had small sample sizes that were not justified by power calculations. Second, evidence of publication bias was detected in some of the analyses (see supplementary Figure 4). Publication and other forms of reporting bias appear to occur in a greater proportion in preclinical than in clinical studies (60, 61). Therefore, studies with less positive results might not have been published. Third, a high number of the included studies came from the same research group (41–46). Fourth, although no statistical heterogeneity was detected, there was evident heterogeneity in the design of the studies, particularly in the type of stem cells (or exosomes) that were used. We conducted subgroup analyses to determine whether some of the stem cell types were more effective than others. However, these subgroups included a limited number of studies. Therefore, the results of the subgroup analysis should be interpreted with caution, and their main value is the generation of hypotheses for future research.
The fifth limitation to discuss was that the risk of bias assessment for the primary studies was hampered by low quality and/or completeness of reporting on relevant domains. This fact has already been highlighted in other systematic reviews of preclinical studies on neonatal pathological conditions (21, 62, 63). In general, studies blinded outcome assessment and had low risk of bias for selective outcome reporting. However, for all other elements of risk of bias, including sequence generation, randomization of intervention, comparability of baseline characteristics, allocation concealment, random housing, investigator blinding, random outcome assessment, and reporting bias, the information contained in the articles was insufficient to draw conclusions about risk of bias. It should be considered that the information may be incomplete because the authors considered some aspects of study design not sufficiently relevant to be mentioned (64). Despite the publication of extensive guidelines such as the ARRIVE guidelines (65), or the Gold Standard Publication Checklist (66), underreporting is a point of concern that harms the internal validity and the generalizability of the results (64). Therefore, it is imperative that studies report all characteristics of their animal, experimental, and intervention models (62, 64). This information, along with information regarding the experimental conditions, is especially important for future study comparisons and meta-analyses and, consequently, to optimize interventions for future translational and clinical studies (62, 64).
One final limitation that is inherent to all preclinical studies is the so-termed indirectness, which is defined as “how well the results translate from animals to the clinical situation” (28). Rodent models of NEC are frequently used by investigators because of their relative low costs and ease of breeding (14, 15). However, the inability to provide intensive care and parenteral nutrition to the pups limits the model to only a few days after NEC induction (14, 15). Moreover, it has been argued that the combination of insults (hypoxia, hypothermia, formula feeds, and bacterial products) that is used in rodent models is not what commonly leads to classical late-onset NEC in very and extremely preterm infants (63). In addition, the effects of an intervention may be species specific, making the extrapolation of experimental NEC to human preterm infants difficult (63). In this sense, it should be noted that some studies were carried out in term pups (see Table 1). Finally, the translational applicability of the studies included in this review is limited by the clinical difficulty of identifying those preterm infants who are starting to develop NEC and are therefore susceptible to early treatment with stem cells. Also related to translational applicability, the majority of the included studies administered the stem cells by intraperitoneal (IP) injection. IP administration of drugs in adults seems to be safe (67), but it is not known if this can be extrapolated to very preterm infants. Nevertheless, the IP route appears to be the most adequate for stem cell administration for the treatment of gastrointestinal disorders (68).
In conclusion, the data form this meta-analysis suggest that both stem cells and stem cell-derived exosomes prevented NEC in rodent experimental models. However, there is a need to explore this effect in other species and NEC models. Preclinical studies using animal models are invaluable tools for enriching our understanding of the etiopathogenesis and treatment of human diseases. Nevertheless, there is a need for greater homogeneity and clarity in both the experimental designs and in the way the design and results are reported in the publications. The participation of clinicians in the design of the experimental models would contribute to increase their translational applicability.
Data Availability Statement
All datasets generated for this study are included in the article/Supplementary Material.
Author Contributions
EV-M conceptualized the study, wrote the review protocol, carried out the systematic search, selected studies for inclusion, supervised data collection, carried out statistical analysis, and reviewed and revised the manuscript. TH carried out the systematic search, selected studies for inclusion, extracted study data, and drafted the first version of the manuscript. BK contributed to interpretation of results and reviewed and revised the manuscript. CH co-wrote the review protocol, supervised study inclusion and data collection, contributed to statistical analyses and interpretation of results, and reviewed and revised the manuscript. EV conceptualized and supervised the study, contributed to statistical analyses and interpretation of results, and reviewed and revised the manuscript. All authors contributed to the article and approved the submitted version.
Funding
This study was funded by a ZonMw grant Synthesis of Evidence in practice, within the program More Knowledge with Fewer Animals, project number 114024123 (in Dutch: Meer Kennis met Minder Dieren—Module Kennisinfrastructuur ‘De praktijk van een Synthesis of Evidence’ voor dierexperimenteel onderzoek).
Conflict of Interest
The authors declare that the research was conducted in the absence of any commercial or financial relationships that could be construed as a potential conflict of interest.
Supplementary Material
The Supplementary Material for this article can be found online at: https://www.frontiersin.org/articles/10.3389/fped.2020.578984/full#supplementary-material
References
1. Neu J, Walker WA. Necrotizing enterocolitis. N Engl J Med. (2011) 364:255–64. doi: 10.1056/NEJMra1005408
2. Neu J. Necrotizing enterocolitis: the mystery goes on. Neonatology. (2014) 106:289–95. doi: 10.1159/000365130
3. Hunter CJ, Upperman JS, Ford HR, Camerini V. Understanding the susceptibility of the premature infant to necrotizing enterocolitis (NEC). Pediatr Res. (2008) 63:117. doi: 10.1203/PDR.0b013e31815ed64c
4. Neu J, Pammi M. Necrotizing enterocolitis: the intestinal microbiome, metabolome and inflammatory mediators. Semin Fetal Neonatal Med. (2018) 23:400–5. doi: 10.1016/j.siny.2018.08.001
5. Cuna A, George L, Sampath V. Genetic predisposition to necrotizing enterocolitis in premature infants: current knowledge, challenges, and future directions. Semin Fetal Neonatal Med. (2018) 23:387–93. doi: 10.1016/j.siny.2018.08.006
6. Mai V, Young CM, Ukhanova M, Wang X, Sun Y, Casella G, et al. Fecal microbiota in premature infants prior to necrotizing enterocolitis. PLoS ONE. (2011) 6:e20647. doi: 10.1371/journal.pone.0020647
7. Wu SF, Caplan M, Lin HC. Necrotizing enterocolitis: old problem with new hope. Pediatr Neonatol. (2012) 53:158–63. doi: 10.1016/j.pedneo.2012.04.001
8. Lim JC, Golden JM, Ford HR. Pathogenesis of neonatal necrotizing enterocolitis. Pediatr Surg Int. (2015) 31:509–18. doi: 10.1007/s00383-015-3697-9
9. Rose AT, Patel RM. A critical analysis of risk factors for necrotizing enterocolitis. Semin Fetal Neonatal Med. (2018) 23:374–9. doi: 10.1016/j.siny.2018.07.005
10. Bowker RM, Yan X, De Plaen IG. Intestinal microcirculation and necrotizing enterocolitis: the vascular endothelial growth factor system. Semin Fetal Neonatal Med. (2018) 23:411–5. doi: 10.1016/j.siny.2018.08.008
11. Gordon PV, Swanson JR, MacQueen BC, Christensen RD. A critical question for NEC researchers: can we create a consensus definition of NEC that facilitates research progress? Semin Perinatol. (2017) 41:7–14. doi: 10.1053/j.semperi.2016.09.013
12. Xing T, Camacho Salazar R, Chen YH. Animal models for studying epithelial barriers in neonatal necrotizing enterocolitis, inflammatory bowel disease and colorectal cancer. Tissue Barriers. (2017) 5:e1356901. doi: 10.1080/21688370.2017.1356901
13. Topalian SL, Ziegler MM. Necrotizing enterocolitis: a review of animal models. J Surg Res. (1984) 37:320–36. doi: 10.1016/0022-4804(84)90196-3
14. Sulistyo A, Rahman A, Biouss G, Antounians L, Zani A. Animal models of necrotizing enterocolitis: review of the literature and state of the art. Innov Surg Sci. (2018) 3:87–92. doi: 10.1515/iss-2017-0050
15. Sodhi C, Richardson W, Gribar S, Hackam DJ. The development of animal models for the study of necrotizing enterocolitis. Dis Model Mech. (2008) 1:94–8. doi: 10.1242/dmm.000315
16. Lu P, Sodhi CP, Jia H, Shaffiey S, Good M, Branca MF, et al. Animal models of gastrointestinal and liver diseases. animal models of necrotizing enterocolitis: pathophysiology, translational relevance, and challenges. Am J Physiol Gastrointest Liver Physiol. (2014) 306:G917–28. doi: 10.1152/ajpgi.00422.2013
17. Crissinger KD. Animal models of necrotizing enterocolitis. J Pediatr Gastroenterol Nutr. (1995) 20:17–22. doi: 10.1097/00005176-199501000-00004
18. Ares GJ, McElroy SJ, Hunter CJ. The science and necessity of using animal models in the study of necrotizing enterocolitis. Semin Pediatr Surg. (2018) 27:29–33. doi: 10.1053/j.sempedsurg.2017.11.006
19. Thebaud B. Stem cells for extreme prematurity. Am J Perinatol. (2019) 36(S 02):S68–73. doi: 10.1055/s-0039-1691774
20. Pierro M, Thebaud B, Soll R. Mesenchymal stem cells for the prevention and treatment of bronchopulmonary dysplasia in preterm infants. Cochrane Database Syst Rev. (2017) 11:CD011932. doi: 10.1002/14651858.CD011932.pub2
21. Augustine S, Avey MT, Harrison B, Locke T, Ghannad M, Moher D, et al. Mesenchymal stromal cell therapy in bronchopulmonary dysplasia: systematic review and meta-analysis of preclinical studies. Stem Cells Transl Med. (2017) 6:2079–93. doi: 10.1002/sctm.17-0126
22. Nitkin CR, Rajasingh J, Pisano C, Besner GE, Thébaud B, Sampath V. Stem cell therapy for preventing neonatal diseases in the 21st century: Current understanding and challenges. Pediatr Res. (2019) 2019:1–12. doi: 10.1038/s41390-019-0425-5
23. Finch-Edmondson M, Morgan C, Hunt RW, Novak I. Emergent prophylactic, reparative and restorative brain interventions for infants born preterm with cerebral palsy. Front Physiol. (2019) 10:15. doi: 10.3389/fphys.2019.00015
24. Ma QQ, Liu FY, Shi M, Sun CH, Tan Z, Chang XD, et al. Bone marrow mesenchymal stem cells modified by angiogenin-1 promotes tissue repair in mice with oxygen-induced retinopathy of prematurity by promoting retinal stem cell proliferation and differentiation. J Cell Physiol. (2019) 234:21027–38. doi: 10.1002/jcp.28706
25. Mueller M, Kramer BW. Stem cells and bronchopulmonary dysplasia-the five questions: which cells, when, in which dose, to which patients via which route? Paediatr Respir Rev. (2017) 24:54–9. doi: 10.1016/j.prrv.2016.12.003
26. Sena ES, Currie GL, McCann SK, Macleod MR, Howells DW. Systematic reviews and meta-analysis of preclinical studies: why perform them and how to appraise them critically. J Cereb Blood Flow Metabol. (2014) 34:737–42. doi: 10.1038/jcbfm.2014.28
27. Vesterinen H, Sena E, Egan K, Hirst T, Churolov L, Currie G, et al. Meta-analysis of data from animal studies: a practical guide. J Neurosci Methods. (2014) 221:92–102. doi: 10.1016/j.jneumeth.2013.09.010
28. Hooijmans CR, de Vries RB, Ritskes-Hoitinga M, Rovers MM, Leeflang MM, IntHout J, et al. Facilitating healthcare decisions by assessing the certainty in the evidence from preclinical animal studies. PLoS ONE. (2018) 13:e0187271. doi: 10.1371/journal.pone.0187271
29. Hooijmans CR, IntHout J, Ritskes-Hoitinga M, Rovers MM. Meta-analyses of animal studies: an introduction of a valuable instrument to further improve healthcare. ILAR J. (2014) 55:418–26. doi: 10.1093/ilar/ilu042
30. Hooijmans C, Ritskes-Hoitinga M. Progress in using systematic reviews of animal studies to improve translational research. PLoS Med. (2013) 10:e1001482. doi: 10.1371/journal.pmed.1001482
31. Villamor-Martinez E, Hunscheid T, Kramer B, Hooijmans C, Villamor E. Stem Cells for the Prevention of Experimental Necrotizing Enterocolitis: a Systematic Review and Meta-Analysis of Preclinical Studies: PROSPERO;. (2018). Available online at: https://www.crd.york.ac.uk/prospero/display_record.php?ID=CRD42018110084 (accessed March 12, 2020).
32. Moher D, Liberati A, Tetzlaff J, Altman DG, The PG. Preferred reporting items for systematic reviews and meta-analyses: The PRISMA statement. PLOS Med. (2009) 6:e1000097. doi: 10.1371/journal.pmed.1000097
33. de Vries RB, Hooijmans CR, Tillema A, Leenaars M, Ritskes-Hoitinga M. Updated version of the embase search filter for animal studies. Lab Anim. (2014) 48:88. doi: 10.1177/0023677213494374
34. Hooijmans CR, Tillema A, Leenaars M, Ritskes-Hoitinga M. Enhancing search efficiency by means of a search filter for finding all studies on animal experimentation in PubMed. Lab Anim. (2010) 44:170–5. doi: 10.1258/la.2010.009117
35. Ouzzani M, Hammady H, Fedorowicz Z, Elmagarmid A. Rayyan—a web and mobile app for systematic reviews. Syst Rev. (2016) 5:210. doi: 10.1186/s13643-016-0384-4
36. Rohatgi A. Web Plot Digitizer San Francisco, California, USA2019. (2019). Available online at: https://automeris.io/WebPlotDigitizer (accessed March 12, 2020).
37. Hooijmans CR, Rovers MM, de Vries RB, Leenaars M, Ritskes-Hoitinga M, Langendam MW. SYRCLE's risk of bias tool for animal studies. BMC Med Res Methodol. (2014) 14:43. doi: 10.1186/1471-2288-14-43
38. Kilkenny C, Parsons N, Kadyszewski E, Festing MF, Cuthill IC, Fry D, et al. Survey of the quality of experimental design, statistical analysis and reporting of research using animals. PLoS ONE. (2009) 4:e7824. doi: 10.1371/journal.pone.0007824
39. Borenstein M, Hedges LV, Higgins J, Rothstein HR. Identifying and quantifying heterogeneity. In: Introduction to Meta-Analysis. Chichester: John Wiley & Sons, Ltd (2009). p. 107–26. doi: 10.1002/9780470743386.ch16
40. Borenstein M, Hedges LV, Higgins JP, Rothstein HR. Publication bias. In: Introduction to Meta-Analysis. Chichester: John Wiley & Sons, Ltd (2009). p. 277:292. doi: 10.1002/9780470743386.ch30
41. McCulloh CJ, Olson JK, Wang Y, Zhou Y, Tengberg NH, D, et al. Treatment of experimental necrotizing enterocolitis with stem cell-derived exosomes. J Pediatr Surg. (2018) 53:1215–20. doi: 10.1016/j.jpedsurg.2018.02.086
42. McCulloh CJ, Olson JK, Zhou Y, Wang Y, Besner GE. Stem cells and necrotizing enterocolitis: a direct comparison of the efficacy of multiple types of stem cells. J Pediatr Surg. (2017) 52:999–1005. doi: 10.1016/j.jpedsurg.2017.03.028
43. Rager TM, Olson JK, Zhou Y, Wang Y, Besner GE. Exosomes secreted from bone marrow-derived mesenchymal stem cells protect the intestines from experimental necrotizing enterocolitis. J Pediatr Surg. (2016) 51:942–7. doi: 10.1016/j.jpedsurg.2016.02.061
44. Wei J, Zhou Y, Besner GE. Heparin-binding EGF-like growth factor and enteric neural stem cell transplantation in the prevention of experimental necrotizing enterocolitis in mice. Pediatr Res. (2015) 78:29–37. doi: 10.1038/pr.2015.63
45. Yang J, Watkins D, Chen CL, Bhushan B, Zhou Y, Besner GE. Heparin-binding epidermal growth factor-like growth factor and mesenchymal stem cells act synergistically to prevent experimental necrotizing enterocolitis. J Am Coll Surg. (2012) 215:534–45. doi: 10.1016/j.jamcollsurg.2012.05.037
46. Zhou Y, Yang J, Watkins DJ, Boomer LA, Matthews MA, Su Y, et al. Enteric nervous system abnormalities are present in human necrotizing enterocolitis: potential neurotransplantation therapy. Stem Cell Res Ther. (2013) 4:157. doi: 10.1186/scrt387
47. Tayman C, Uckan D, Kilic E, Ulus AT, Tonbul A, Murat Hirfanoglu I, et al. Mesenchymal stem cell therapy in necrotizing enterocolitis: a rat study. Pediatr Res. (2011) 70:489–94. doi: 10.1203/PDR.0b013e31822d7ef2
48. Zani A, Cananzi M, Fascetti-Leon F, Lauriti G, Smith VV, Bollini S, et al. Amniotic fluid stem cells improve survival and enhance repair of damaged intestine in necrotising enterocolitis via a COX-2 dependent mechanism. Gut. (2014) 63:300–9. doi: 10.1136/gutjnl-2012-303735
49. Zani A, Cananzi M, Lauriti G, Fascetti-Leon F, Wells J, Siow B, et al. Amniotic fluid stem cells prevent development of ascites in a neonatal rat model of necrotizing enterocolitis. Eur J Pediatr Surg. (2014) 24:57–60. doi: 10.1055/s-0033-1350059
50. Barlow B, Santulli TV. Importance of multiple episodes of hypoxia or cold stress on the development of enterocolitis in an animal model. Surgery. (1975) 77:687–90.
51. Perin L, Sedrakyan S, Da Sacco S, De Filippo R. Characterization of human amniotic fluid stem cells and their pluripotential capability. Methods Cell Biol. (2008) 86:85–99. doi: 10.1016/S0091-679X(08)00005-8
52. McCulloh C, Zhou Y, Besner GE. Transplantation of amniotic fluid–derived neural stem cells. Perinatal Stem Cells. (2018) 2018:39–51. doi: 10.1016/B978-0-12-812015-6.00003-0
53. Slack JM. What is a stem cell? Wiley Interdiscipl Rev Dev Biol. (2018) 7:e323. doi: 10.1002/wdev.323
54. McCulloch EA, Till JE. Perspectives on the properties of stem cells. Nat Med. (2005) 11:1026. doi: 10.1038/nm1005-1026
55. Cananzi M, Atala A, De Coppi P. Stem cells derived from amniotic fluid: new potentials in regenerative medicine. Reprod Biomed Online. (2009) 18:17–27. doi: 10.1016/S1472-6483(10)60111-3
56. Prathipati P, Nandi SS, Mishra PK. Stem cell-derived exosomes, autophagy, extracellular matrix turnover, and miRNAs in cardiac regeneration during stem cell therapy. Stem Cell Rev Rep. (2017) 13:79–91. doi: 10.1007/s12015-016-9696-y
57. Chen T, Xie M-Y, Sun J-J, Ye R-S, Cheng X, Sun R-P, et al. Porcine milk-derived exosomes promote proliferation of intestinal epithelial cells. Sci Rep. (2016) 6:33862. doi: 10.1038/srep33862
58. Hock A, Miyake H, Li B, Lee C, Ermini L, Koike Y, et al. Breast milk-derived exosomes promote intestinal epithelial cell growth. J Pediatr Surg. (2017) 52:755–9. doi: 10.1016/j.jpedsurg.2017.01.032
59. Li B, Hock A, Wu RY, Minich A, Botts SR, Lee C, et al. Bovine milk-derived exosomes enhance goblet cell activity and prevent the development of experimental necrotizing enterocolitis. PLoS ONE. (2019) 14:e0211431. doi: 10.1371/journal.pone.0211431
60. Van der Worp HB, Howells DW, Sena ES, Porritt MJ, Rewell S, O'Collins V, et al. Can animal models of disease reliably inform human studies? PLoS Med. (2010) 7:e1000245. doi: 10.1371/journal.pmed.1000245
61. Tsilidis KK, Panagiotou OA, Sena ES, Aretouli E, Evangelou E, Howells DW, et al. Evaluation of excess significance bias in animal studies of neurological diseases. PLoS Biol. (2013) 11:e1001609. doi: 10.1371/journal.pbio.1001609
62. Archambault J, Moreira A, McDaniel D, Winter L, Sun L, Hornsby P. Therapeutic potential of mesenchymal stromal cells for hypoxic ischemic encephalopathy: a systematic review and meta-analysis of preclinical studies. PLoS ONE. (2017) 12:e0189895. doi: 10.1371/journal.pone.0189895
63. Athalye-Jape G, Rao S, Patole S. Effects of probiotics on experimental necrotizing enterocolitis: a systematic review and meta-analysis. Pediatr Res. (2018) 83:16–22. doi: 10.1038/pr.2017.218
64. Leenaars CH, Kouwenaar C, Stafleu FR, Bleich A, Ritskes-Hoitinga M, De Vries RB, et al. Animal to human translation: a systematic scoping review of reported concordance rates. J Transl Med. (2019) 17:223. doi: 10.1186/s12967-019-1976-2
65. Kilkenny C, Browne WJ, Cuthill IC, Emerson M, Altman DG. Improving bioscience research reporting: the ARRIVE guidelines for reporting animal research. PLoS Biol. (2010) 8:e1000412. doi: 10.1371/journal.pbio.1000412
66. Hooijmans CR, Leenaars M, Ritskes-Hoitinga M. A gold standard publication checklist to improve the quality of animal studies, to fully integrate the Three Rs, and to make systematic reviews more feasible. Altern Lab Anim. (2010) 38:167–82. doi: 10.1177/026119291003800208
67. Fonnes S, Holzknecht BJ, Arpi M, Rosenberg J. Characterisation and safety of intraperitoneal perioperative administration of antibacterial agents: a systematic review. Drug Res. (2017) 67:688–97. doi: 10.1055/s-0043-109565
Keywords: stem cells, necrotizing enterocolitis (NEC), preclinical (in-vivo) studies, meta-analysis, risk of bias assessment
Citation: Villamor-Martinez E, Hundscheid T, Kramer BW, Hooijmans CR and Villamor E (2020) Stem Cells as Therapy for Necrotizing Enterocolitis: A Systematic Review and Meta-Analysis of Preclinical Studies. Front. Pediatr. 8:578984. doi: 10.3389/fped.2020.578984
Received: 01 July 2020; Accepted: 13 October 2020;
Published: 09 December 2020.
Edited by:
Geok Chin Tan, National University of Malaysia, MalaysiaReviewed by:
Chin Seng Gan, University of Malaya, MalaysiaMaryAnn Volpe, Tufts University School of Medicine, United States
Copyright © 2020 Villamor-Martinez, Hundscheid, Kramer, Hooijmans and Villamor. This is an open-access article distributed under the terms of the Creative Commons Attribution License (CC BY). The use, distribution or reproduction in other forums is permitted, provided the original author(s) and the copyright owner(s) are credited and that the original publication in this journal is cited, in accordance with accepted academic practice. No use, distribution or reproduction is permitted which does not comply with these terms.
*Correspondence: Eduardo Villamor, e.villamor@mumc.nl