- 1Department of Oral and Maxillofacial Surgery, Nanjing Stomatological Hospital, Medical School of Nanjing University, Nanjing, China
- 2Department of Geriatrics, Zhongda Hospital Southeast University, Nanjing, China
- 3Department of Pediatric Dentistry, Nanjing Stomatological Hospital, Medical School of Nanjing University, Nanjing, China
- 4Department of Periodontology, Nanjing Stomatological Hospital, Medical School of Nanjing University, Nanjing, China
Background: Many studies have shown that c-Myc plays a critical role in tumorigenesis. However, the molecular role of c-Myc in head and neck squamous cell carcinoma (HNSC) remains unclear.
Methods: Several biological databases, including UALCAN, TIMER2.0, TCGAportal, GEPIA, KM plotter, OncoLnc, LinkedOmics, GSCA, and TCIA, were used to analyze the molecular role of c-Myc in HNSC. The expression levels of c-Myc were validated by real-time PCR (RT–PCR) and Western blot in CAL-27 cells.
Results: The expression of c-Myc mRNA were significantly increased in HPV-negative HNSC tissues. The expression of c-Myc gene level was correlated with TP53 mutation status. HNSC also showed hypomethylated c-Myc compared with normal tissues. c-Myc was identified as an ominous prognostic factor for HNSC patients and correlated with immune infiltrating levels. Moreover, high c-Myc expression was associated with decreased expression of a series of immune checkpoints, resulting in a dampened immune response. c-Myc potentially mediated IL-17 signaling pathway and Th1 and Th2 cell differentiation. Inhibition of c-Myc expression increased apoptosis of CAL-27 cells.
Conclusions: These findings suggest a new mechanism of c-Myc in the prognosis of HNSC, implying the potential of c-Myc as a therapeutic target for HNSC patients.
Introduction
Head and neck squamous cell carcinoma (HNSC) is the eighth most frequent tumor worldwide and is associated with a high rate of morbidity and mortality (1). Despite great advances in diagnostic and therapeutic methods, a high rate of local and distant failure after treatment of advanced HNSC was observed (2). The prognosis for these patients with recurrent and metastatic (R/M) HNSC receiving platinum-based therapy is even worse (3). Hence, there is a high need for improved therapy for this population. A variety of genomic imbalances are involved in head and neck carcinogenetic processes (4–6).
The c-Myc proto-oncogene located at 8q24 has been implicated in the regulation of cell growth, differentiation and apoptosis (7). c-Myc overactivation is a frequently detected and crucial genetic event in HNSC. c-Myc gene amplification is involved in the pathogenesis of HNSC, especially in laryngeal squamous cell carcinoma (LSCC), which is the prominent histopathological entity among HNSC (8). Significant c-Myc amplification detected by implementing the polymerase chain reaction technique was observed in another study (9). c-Myc promotes the activation of poly (ADP-ribose) polymerase (PARP)-dependent DNA repair pathways, resulting in chemoresistance (10). c-Myc also promotes CHK1 and CHK2 expression to mediate the DNA damage checkpoint response, resulting in radioresistance (11). Overactivation of c-Myc seems to be correlated with aggressive biological behavior in HNSC, so its detailed mechanisms necessitate further study. The purpose of our study was to investigate the molecular role of c-Myc in HNSC to provide a new effective treatment option for HNSC patients.
Materials and methods
Expression and methylation of c-Myc in HNSC
UALCAN (12) and TIMER2.0 (13) were applied to evaluate the expression c-Myc in HNSC patients. The comparison of c-Myc expression between HNSC and normal samples was conducted using TCGA-HNSC dataset. The expression of c-Myc in subgroups of HNSC was subdivided based on TP 53 mutation status.
Survival analysis of c-Myc in HNSC
TCGAportal (http://www.tcgaportal.org), GEPIA (14), KM plotter (15), and OncoLnc (16) were used to evaluate the impact of c-Myc mRNA expression on OS in HNSC patients based on the TCGA database.
Relationships between c-Myc and immune checkpoints in HNSC
The relationships between c-Myc expression and immune checkpoints in HNSC patients from TCGA database were further investigated via LinkedOmics (17).
Immune infiltration analysis of c-Myc in HNSC
GSCA (18) was used to detect the correlation between c-Myc expression and the immune microenvironment in HNSC. TIMER2.0 (13) and TCIA (19) were used to estimate the impact of immune cells on the OS of HNSC patients.
Explore c-Myc related pathways in HNSC
Kyoto Encyclopedia of Genes and Genomes (KEGG) pathway enrichment analyses were conducted to understand the functions of c-Myc via LinkedOmics (17).
Materials and cell culture conditions
Triptonide (purity > 98%) was purchased from Sigma (St. Louis, USA) and dissolved in dimethyl sulfoxide (DMSO) as a 5 mM stock and then freshly diluted in culture media at 10 nM, 30 nM, and 50 nM. Oral adenosquamous carcinoma cells, CAL-27 cells, were obtained from the Chinese Academy of Sciences Cell Bank (Shanghai, China). CAL-27 cells were cultured in RPMI-1640 supplemented with 10% fetal bovine serum in a humidified atmosphere containing 5% CO2 at 37°C.
c-Myc siRNA
Synthetic c-Myc siRNA was used to knockdown the expression of c-Myc in CAL-27 cells. c-Myc siRNA (target sequence: 5′- GAGGAUAUCUGGAAGAAAUTT -3′; antisense:5′- AUUUCUUCCAGAUAUCCUCTT -3′) was purchased from Kaiji (Nanjing, China). Lipofectamine RNAiMax was purchased from Invitrogen (Grand Island, USA). Transfection was performed according to the manufacturer’s instructions. Human non-specific siRNA ((Kaiji, Nanjing, China)) was used as a negative control (NC).
Apoptosis analysis
Annexin V/propidium iodide (PI) apoptosis detection kit (Biyuntian, Shanghai, China) was used to detect cell apoptosis according to the manufacturer’s protocol. CAL-27 cells were exposed to different concentrations of triptonide (10 nM, 30 nM, or 50 nM) for 72 h and then collected. Cells were resuspended in 200 μL binding buffer containing 5 μL Annexin V and 10 μL PI fluorescence dye, and then apoptosis was detected by flow cytometry (Becton Dickinson FACS Calibur).
Western blot
Total proteins were extracted using SDS lysis buffer (Beyotime, Shanghai, China) supplemented with protease and phosphatase inhibitor cocktail (Beyotime). Protein samples were subjected to 12% SDS–PAGE and then transferred to PVDF membranes (Bio–Rad, California, USA). PVDF membranes containing proteins were blocked by QuickBlock Blocking Buffer at room temperature for 20 min and then incubated overnight at 4 °C with primary antibody. After washing, the blots were soaked with horseradish peroxidase (HRP)-conjugated secondary antibody at room temperature for 1 h. Finally, blots were visualized using Immobilon Western Chemiluminescent HRP Substrate (Millipore).
Real-time PCR
Total RNA was extracted using the RNA-Quick Purification Kit (Esunbio, Hangzhou, China) and then used in a reverse transcriptase reaction with the PrimeScript™ RT reagent kit (Takara, Kyoto, Japan). The TB Green™ Premix Ex Taq™ kit (Takara) was used for the thermocycling reaction in an ABI-7500 fast Real Time PCR machine (Thermo Scientific). The primer sequences used in the experiment were as follows: c-Myc (Forward: 5′-CGCCAGAGGAGGAACGAGCTAA-3′; Reverse: 5′- TCTGCTTGGACGGACAGGATGT- 3′).
Human oral squamous cell carcinoma tissues
Twenty of the oral squamous cell carcinoma (OSCC) tissue samples were obtained from the tissue bank of the department of pathology, Nanjing Stomatological Hospital. The study was approved by the institutional review board of the hospital. Informed consent was collected from all patients or their direct relatives. All diagnoses were histologically confirmed.
Tissue microarrays (TMA) construction and immunohistochemistry (IHC)
Protein expression of c-Myc, PDCD1 and LAG3 was analyzed by IHC using a tissue microarray (TMA) platform. The TMA was constructed using pretreatment formalin-fixed paraffin-embedded (FFPE) tumor specimens from 20 adult patients with OSCC. The samples included 11 (55.0%) males and 9 (45.0%) females, with the mean age of 62.0 years old (range: 23-77 years old). Sections (5 μm) were then cut from each TMA and stained with antibodies to c-Myc (1:400; Abcam, Cambridge, USA), PDCD1 (1:200; Abcam, Cambridge, USA), and LAG3 (1:500; Abcam, Cambridge, USA). IHC staining was performed as previously reported (20, 21).
Statistical analysis
Student’s t test, χ2 test and one-way ANOVA were used for comparisons, as appropriate. The difference was considered statistically significant if P<0.05. All analyses were performed using GraphPad Prism 8 (GraphPad Software, California, USA).
Results
c-Myc is significantly differentially expressed between TP53 wild-Type and mutant HNSC
No significant difference in c-Myc mRNA expression in HNSCs was observed (Figures 1A, B). However, patients with HPV-negative HNSC had significantly higher c-Myc mRNA expression (Figure 1A). c-Myc expression was correlated with the mutation status of TP53 (Figures 1C, D). There was no correlation between c-Myc and TP53 expression (Figure 1E).
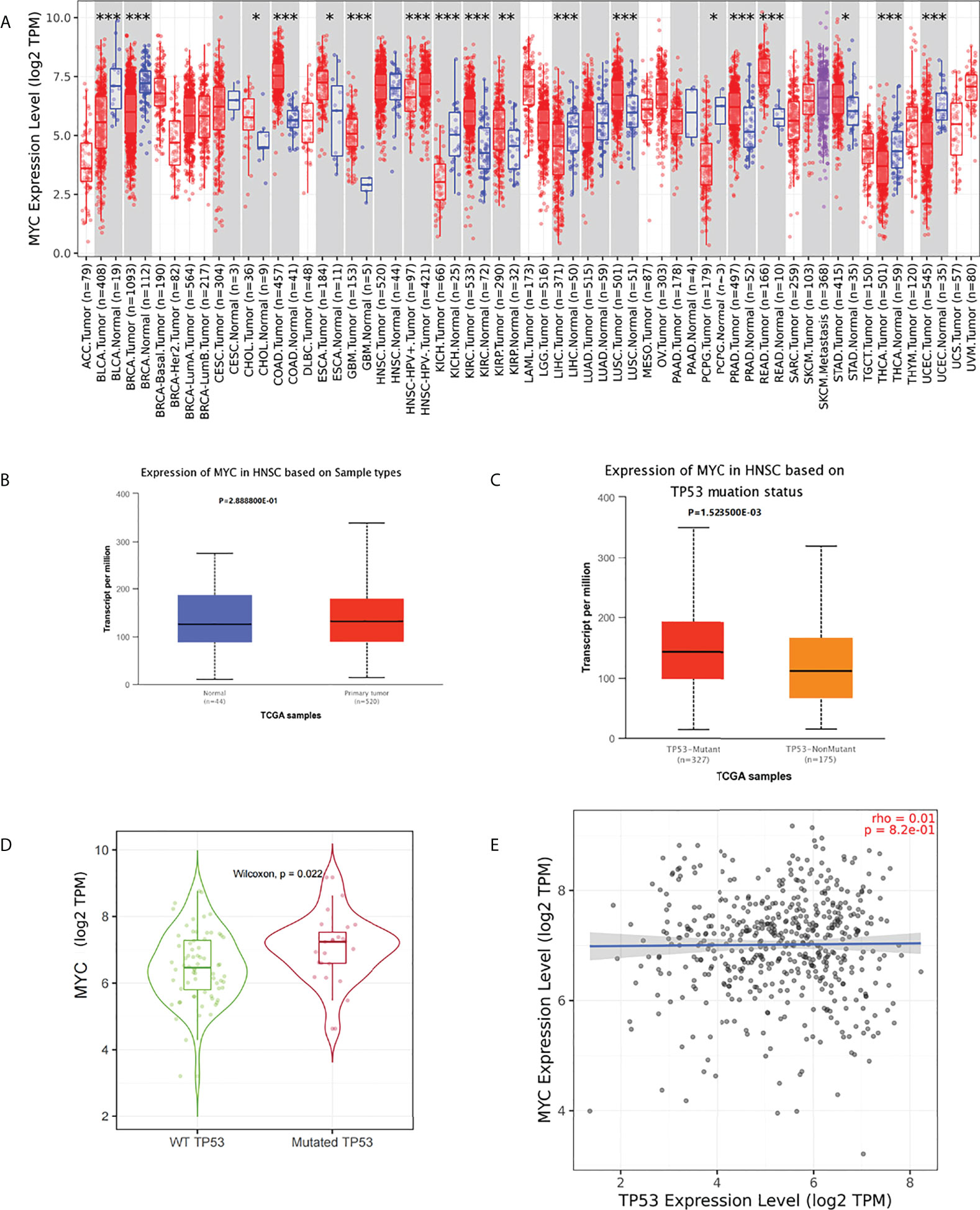
Figure 1 (A) c-Myc mRNA expression in pancancer (*: p-value < 0.05; **: p-value <0.01; ***: p-value <0.001). (B) c-Myc mRNA expression in HNSC (UALCAN). (C) c-Myc mRNA expression based on TP 53 mutation status (UALCAN). (D) c-Myc mRNA expression based on TP 53 mutation status (TIMER2.0). (E) Correlation between MYC and TP53 expression (TIMER2.0Fig 1E).
c-Myc is a prognostic biomarker in HNSC
The overall survival (OS) of HNSC patients with low c-Myc mRNA expression was superior to that of patients with high c-Myc mRNA expression (Figures 2A–D). The cutoff values of c-Myc mRNA expression were different in these databases. These results revealed that c-Myc mRNA is a valuable prognostic biomarker in HNSC. TP53 mutant significantly deteriorated the survival of HNSC patients with low c-Myc mRNA expression (Figures 3A, B).
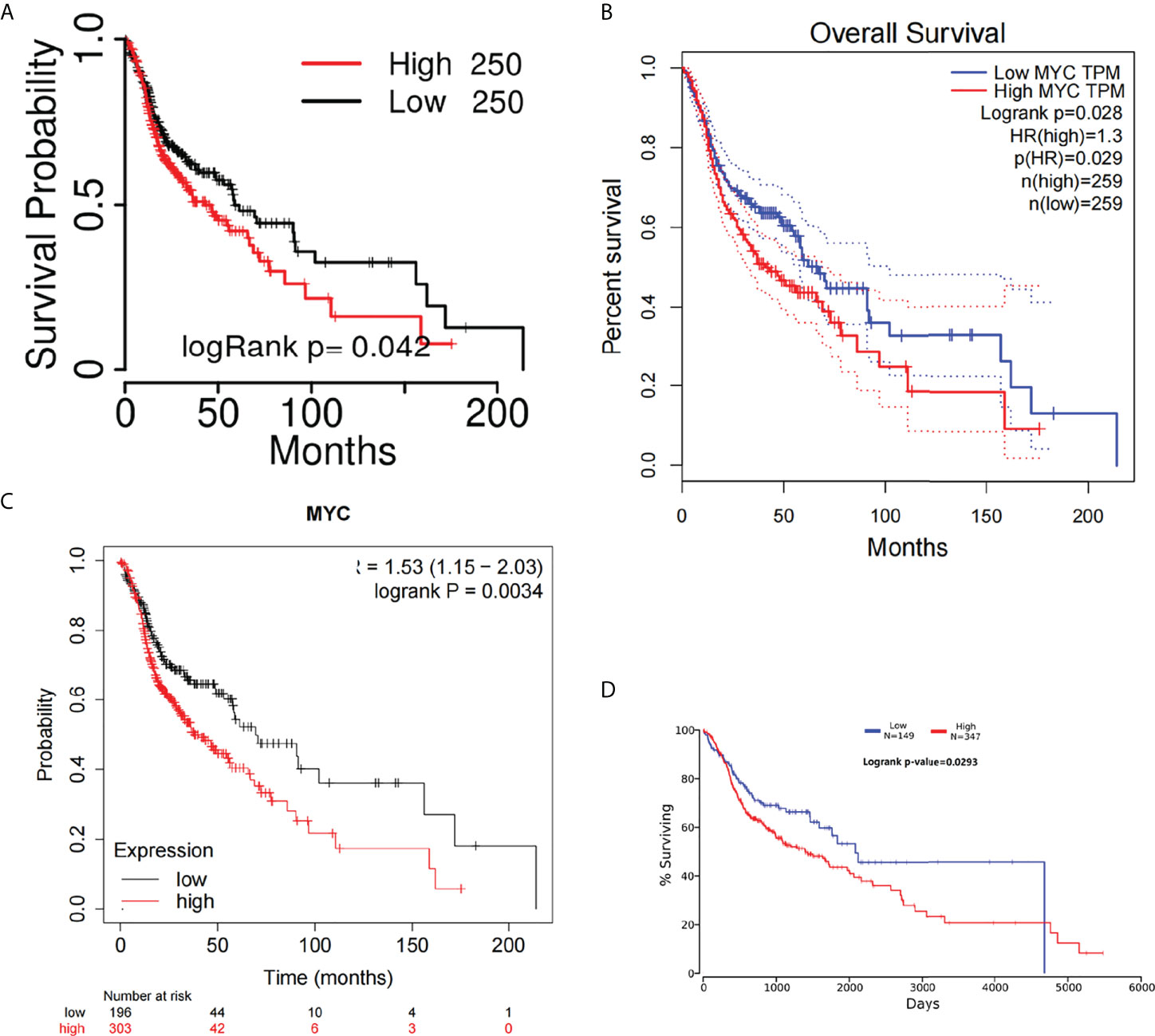
Figure 2 Overall survival curves of c-Myc in HNSC from different databases. (A) TCGAportal. (B) GEPIA. (C) KM-plotter. (D) OncoLnc.
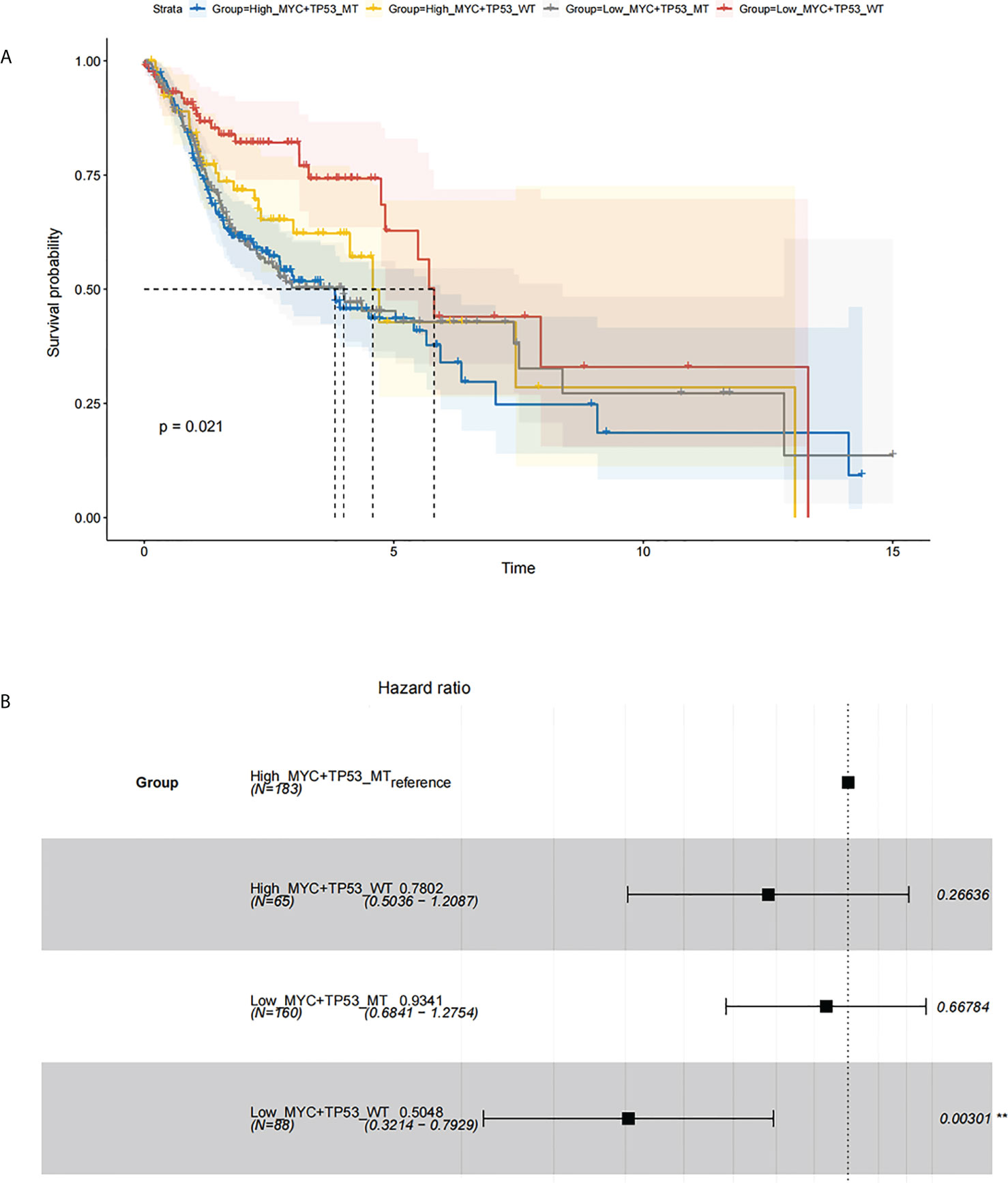
Figure 3 Survival analysis based on c-Myc expression and TP53 mutation status. (A) Kaplan Meier model. (B). Cox proportional hazards model.
c-Myc expression is related to immune checkpoint (ICP) genes in HNSC
In this study, we analyzed the association of c-Myc expression and TP53 mutation status with immune checkpoints in the TCGA database. As shown in Figure 4, some inhibitory checkpoint molecules, including CTLA4, HAVCR2, LAG3 and PDCD1, had negative correlations with c-Myc mRNA levels in HNSCs. HNSC patients with TP53 mutant had low expression of CTLA4, HAVCR2, LAG3 and PDCD1 (Figures 4E–H).
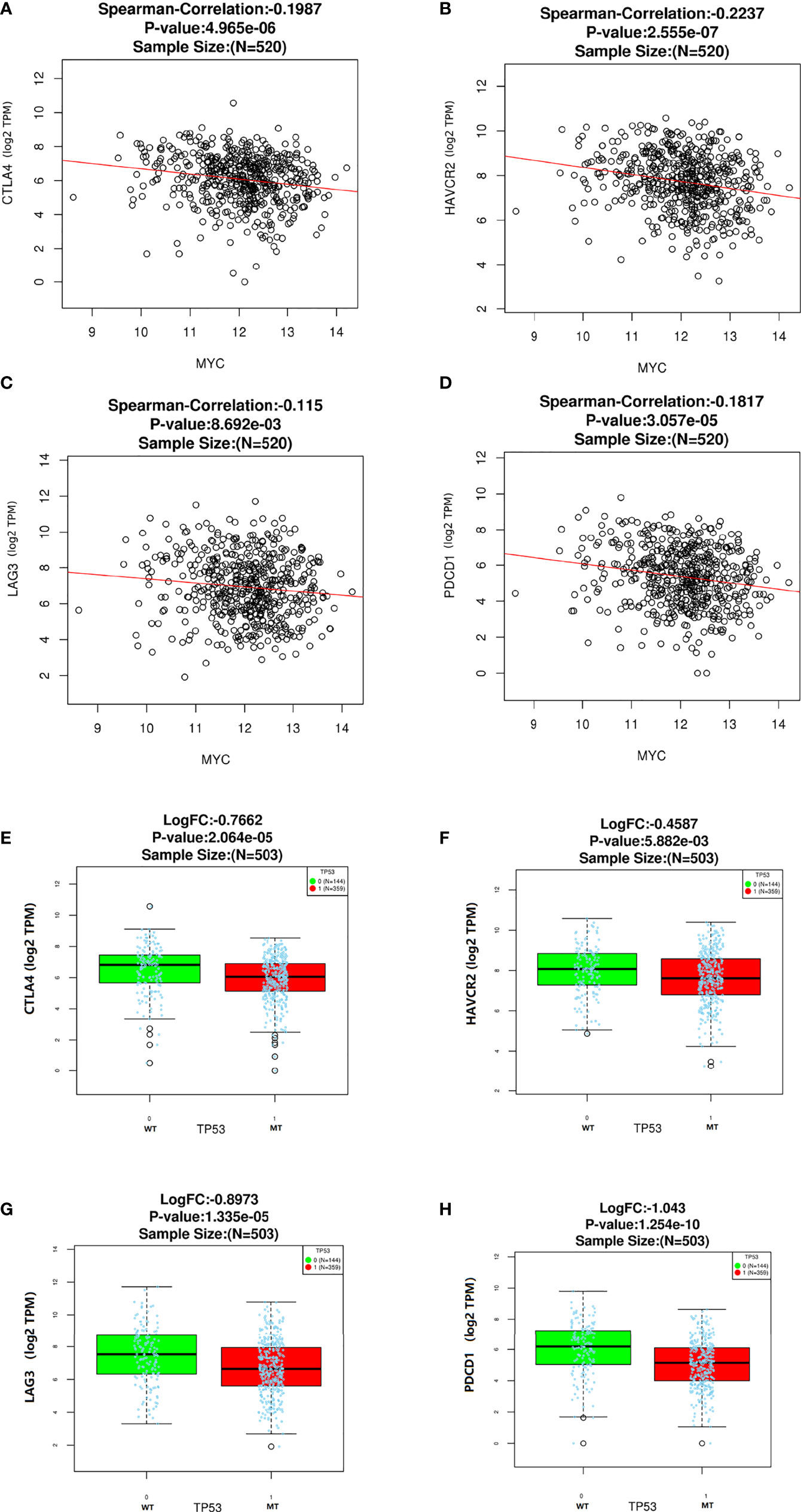
Figure 4 Correlation between c-Myc and immune checkpoints. (A) CTLA4. (B) HAVCR2. (C) LAG3. (D)Immune checkpoints expression based on TP 53 mutation status. (E) CTLA4. (F) HAVCR2. (G) LAG3. (H) PDCD1.
c-Myc expression is correlated with immune cell infiltration in HNSC
We aimed to explore the correlation of c-Myc expression with immune cell infiltration in HNSC. The expression of c-Myc was negatively correlated with the infiltration of B cells, CD8+ T cells, CD4+ T cells, gamma delta T cells, and NK cells in HNSC patients (Figures 5A, C, E, G, K). In contrast, c-Myc expression had a positive correlation with the enrichment of neutrophils and Th17 cells (Figures 5I, M). As expected, the association of these tumor-infiltrating immune cells with OS was also observed in HNSC (Figures 5B, D, F, H, J, L, N). LinkedOmics was used to construct KEGG pathway enrichment analyses (Figure 6). c-Myc potentially mediated IL-17 signaling pathway and Th1 and Th2 cell differentiation to affect immune cell infiltration.
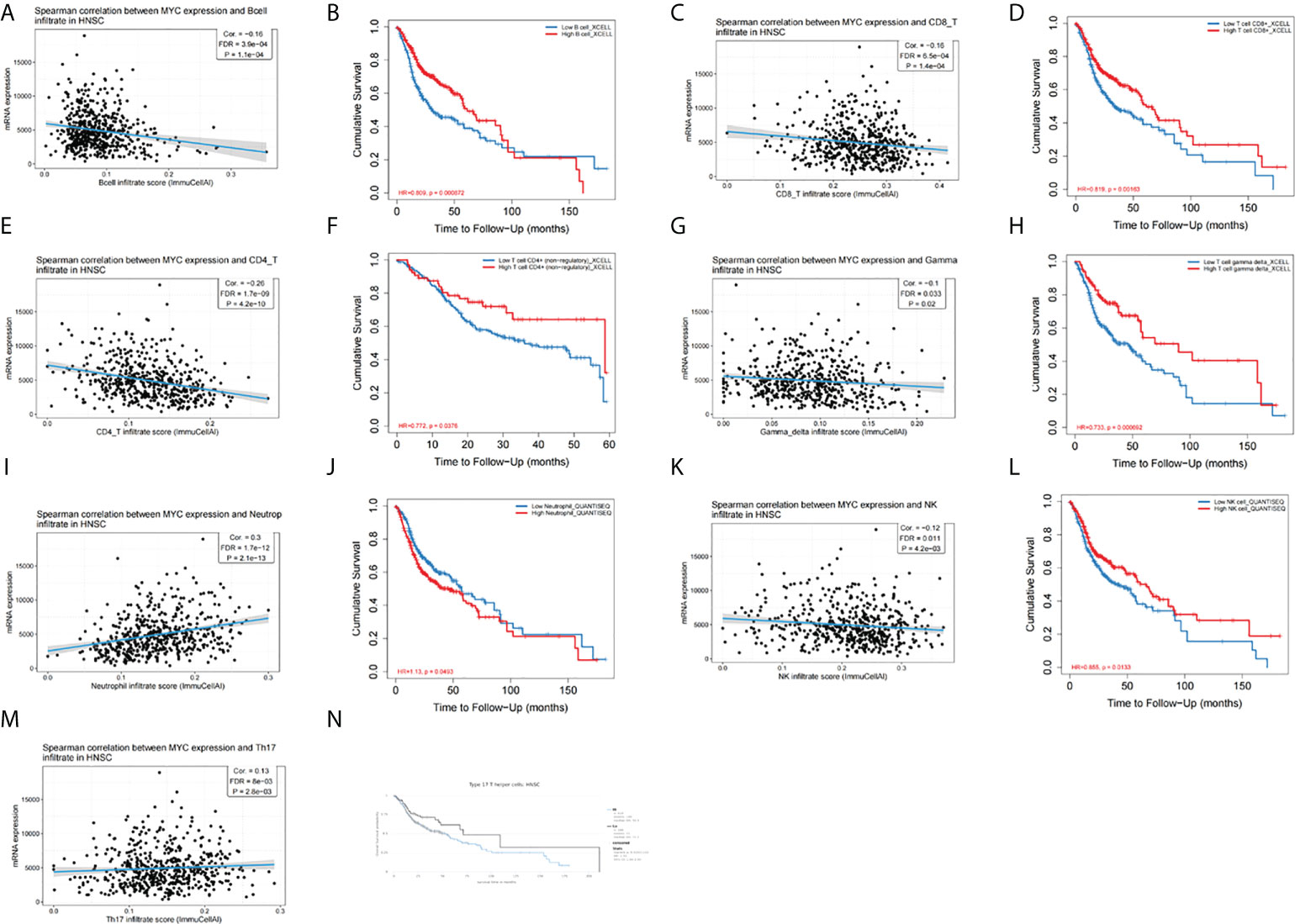
Figure 5 c-Myc expression is correlated with immune infiltration of (A) B cells, (C) CD8+ T cells, (E) CD4+ T cells, (G) gamma delta T cells, (I) neutrophils, (K) NK cells, and (M) Th17 cells. Tumor-infiltrating immune cells were associated with OS (B, D, F, H, J, L, N).
Triptonide induces apoptosis of CAL-27 cells via c-Myc signaling
The apoptosis ratio of CAL-27 cells was investigated using Annexin V-FITC/PI staining and flow cytometry (Figure 7A). After incubation with 50 nM triptonide for 72 h, the percentage of apoptotic cells was 24.05 ± 0.45% (P < 0.01). Interestingly, low-dose (10 nM) triptonide treatment for 72 h also resulted in obvious apoptosis of CAL-27 cells. The percentage of apoptotic cells increased from 3.58 ± 1.04% to 6.94 ± 0.16% (P < 0.01) at 10 nM for 72 h. c-Myc specific siRNA also significantly increased the percentage of apoptotic cells. On the basis of triptonide treatment, we added c-Myc-specific siRNA to knockdown c-Myc expression (Figures 7D, F) and observed that the percentage of apoptotic cells still increased significantly (Figure 7B). We treated CAL-27 cells with 10 nM triptonide for 72 h and then collected cells for analysis of c-Myc protein levels using Western blotting. We observed that triptonide treatment and c-Myc specific siRNA induced a decrease in c-Myc protein (Figures 7C, D). To reveal the mechanism by which triptonide reduces c-Myc proteins, we tested the c-Myc mRNA level in CAL-27 cells (Figure 7E) and observed that triptonide treatment led to a decrease in c-My, suggesting that trip¬tonide regulates c-Myc at the transcriptional level.
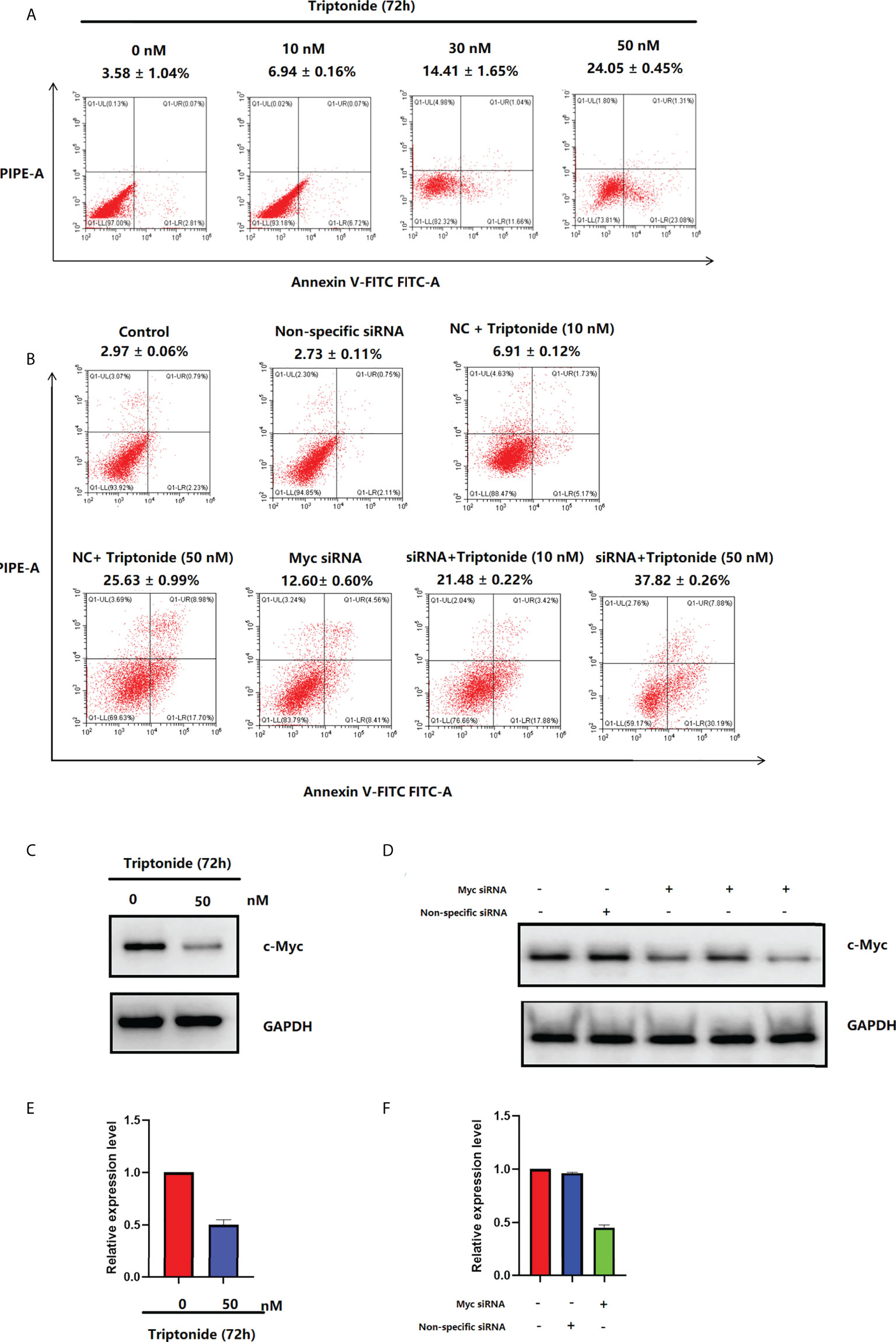
Figure 7 (A) Triptonide induces cell apoptosis. (B) c-Myc siRNA transfection induces cell apoptosis. (C) c-Myc levels in CAL-27 cells after treatment with triptonide, assessed by western blot. (D) The expression of c-Myc measured by western blot after c-Myc siRNA transfection. (E) c-Myc levels in CAL-27 cells after treatment with triptonide, assessed by RT–PCR. (F) The expression of c-Myc measured by RT–PCR after c-Myc siRNA transfection.
LAG3 expression tended to increase in patients with low expression of c-Myc
No significant relationship was found between c-Myc expression and gender, age, tumor staging, and differentiation (Table 1). LAG3 protein was expressed on the tumor-infiltrating lymphocytes (TILs) in 15 out of 20 (75%) samples. 45.0% tissues exhibited PDCD1 immunostaining (Figure 8). There was a trend that more LAG3 expression was found in patients with low c-Myc expression (p=0.072). There was no significant relationship between c-Myc and PDCD1 (p=0.653).
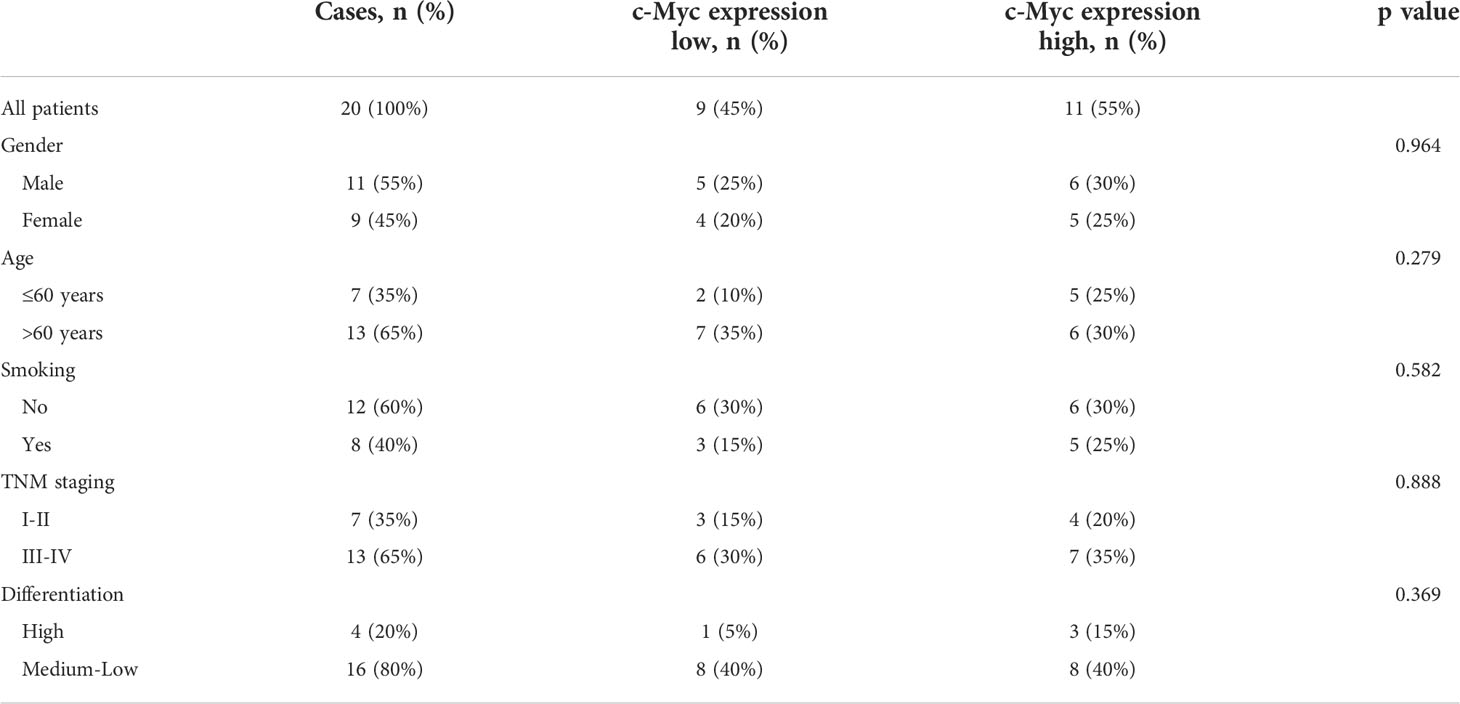
Table 1 The relationship between c-Myc expression and clinicopathological features of OSCC patients.
Discussion
Identifying novel molecular prognostic biomarkers is of great importance for improving outcome of HNSC, which develops as a result of a series of genetic and epigenetic alterations in “cancer genes” (tumor suppressors and oncogenes). c-Myc is mainly involved in apoptosis, cell cycle, metabolism, proliferation, and ribosome biogenesis. The overexpression of c-Myc has been almost invariably linked to tumorigenesis. Our results indicated that c-Myc was highly expressed in TP53-mutated HNSC patients. Additionally, high expression of c-Myc in CAL-27 cells was also detected by Western blot. Subsequently, the prognosis analysis results demonstrated that the expression of c-Myc in HNSC could be an independent predictive factor. As a result, due to the downregulation of c-Myc expression in CAL-27 cells, the percentage of apoptotic cells was increased. c-Myc silencing by either shRNA c-Myc or c-Myc inhibitor (10058-F4) resulted in a dose-dependent reduction in long non-coding RNA SNHG16 levels, which induced cell apoptosis in CAL-27 and TSCCA cells (22).
The tumor-immune microenvironment (TME) in HNSC is immunosuppressive and may play an important role in HNSC progression and treatment resistance (23). Understanding the landscape of the tumor-immune microenvironment is critical for improving the efficacy of current immunotherapies in HNSC. The TME is composed of different subsets of cells, such as T cells, B cells, neutrophils, macrophages, regulatory T (Treg) cells, natural killer (NK) cells and mast cells (24). Tumors often evade host immune surveillance by suppressing cytotoxic T cell function or by activating and expanding immunosuppressive cell populations. It is now clear that c-Myc plays a crucial role in instructing the tumor microenvironment (25, 26). c-Myc regulates the TME through effects on both immunoregulatory proteins and immune effector cells. Our results also showed that c-Myc expression was associated with immune cell infiltration. Enhanced tumor infiltration of CD3+CD4+, CD3+CD8+ T cells and NK cells were observed in the Myc-dependent prostate cancer (MycCaP) tissues after small molecule inhibitors (MYCi) treatment (27). Our results showed that an increase percentage of these tumor-infiltrating immune cells were associated with better OS in HNSC patients. MYC inhibitor treatment may induce immunogenic cell death (ICD), which could activate the immune response in tumors leading to immune cell infiltration (27). Myc inhibitor prodrug (MI3-PD) could reduce M2 macrophages in the tumor microenvironment while sparing M1 antitumor macrophages (28). c-Myc inhibitor JQ1 could block M2 polarization of macrophages via S1PR1 (29). Mst1-deficiency may induce the hyperactivation of dendritic cells (DCs) (30). IL-23 was increased in Mst1 -/- dendritic cells (DCs), which also exhibited an increase in c-Myc protein levels. c-Myc inhibitor could downregulated the increased expression of IL-23 observed in Mst1 -/- DCs (30). Upon c-Myc activation, there is an immediate exclusion of T, B and NK cells within the tumor microenvironment via IL-23 signaling (25). c-Myc also cooperates with Ras to regulate the regulate the secretion of CCL9 and IL-23 (25), thereby promoting the recruitment of immunosuppressive cells and the exclusion of adaptive T and B cells and innate immune NK cells to facilitate tumor immune escape. IL-23 plays a critical role in enhancing IL-17 production in vivo (31). KEGG results showed that IL-17 signaling pathway was actived in c-Myc overexpressed HNSC patients. Th17 cells are maintained and expanded by IL-23 via tumor-secreted PGE2 (32). Th17 cells further produce IL-17 interacting with IL-17RA/RC complex on receptor carrying cells to regulate functionality of DCs and create a self-sustaining feedback loop via IL-23 (33). Induced c-Myc expression could enhanced tumor infiltration of neutrophils, which play a stimulating role in c-Myc-induced liver tumorigenesis (34). Neutrophils play a key role in mediating tumor angiogenesis, however, the increase of neutrophil infiltration could be suppressed by the inhibitor of angiogenesis (34).
The mutational profile of TP53 was an independent prognostic factor in HNSC (35). TP53 mutations occurred in HNSC with a frequency of 72% in a whole-exome sequencing analyses study (36). Mutant p53 proteins not only lose tumor-suppressive functions but also frequently exert oncogenic gain-of-function (GOF) properties through their ability to modulate gene expression (37). c-Myc acted as a major mediator of mutant p53 GOF in HNSC (38). Mutant p53 gains its function via c-Myc activation upon CDK4 phosphorylation in HCC (39). Mutant p53 can regulate the expression of the endogenous c-Myc gene and could sustain active the c-Myc promoters (38, 40). Predictive power of coexpression of mutant p53 and c-Myc proteins in outcome of HNSC is more accurately than what these proteins do individually (41). Mutant p53 not only contributes to c-Myc hyperactivation but also enhances c-Myc protein stability by preventing FBW4A-mediated ubiquitination and degradation in HCC cells (42).
HNSC patients exhibited higher c-Myc expression, and the expression of most immune checkpoint molecules, such as CTLA4, HAVCR2, LAG3, and PDCD1, had negative correlations with c-Myc mRNA levels in HNSC in our study. A trend that more LAG3 protein expression was found in patients with low c-Myc expression (p=0.072) was observed in our study. Depending on the cellular context, the c-Myc oncogene could serve as a positive or negative regulator of immune checkpoint molecules expression (43). Knockdown of c-Myc expression in hepatocellular carcinoma cells exposed to IFN-γ using siRNA assay increased expression of PD-L1 both at mRNA and protein levels (44). The expression of PD-L1 could be mediated by the IFNR/JAK/STAT1/IRF1 pathway, yet c-Myc could suppress the expression of STAT1 both at mRNA level and protein level (44). Up-regulation of c-Myc expression during T-cell priming was inhibited by treatment with I-BET-762, which also led to increased expression of the inhibitory cell-surface receptor LAG3 (45). CTLA4 mRNA was significantly less expressed in lymph nodes chronic lymphocytic leukemia (LN-CLL) cells, and c-Myc mRNA was significantly overexpressed in LN-CLL cells (44). Suppression of CTLA4 in chronic lymphocytic leukemia (CLL) patient samples caused a reduction in the levels of c-Myc messenger RNA and protein (46). These results indicate that there was a relationship between c-Myc and CTLA4. HNSC patients with higher CTLA4 levels had longer OS than those with lower CTLA4 levels (P < 0.001) (47). Altogether, it is still unclear how c-Myc might downregulate the expression of immune checkpoint molecules in HNSC.
HERB database, a high-throughput experiment- and reference-guided database of traditional Chinese medicine, was used to find that c-Myc is the target gene of Tripterygium wilfordii Hook (48). Triptonide is a small molecule monomer extract from the ancient Chinese herb Tripterygium wilfordii Hook, which has historically been used in traditional Chinese medicine to treat rheumatoid arthritis for centuries (49). TN induced nasopharyngeal carcinoma (NPC) cell cycle arrest and apoptosis activation through downregulation of c-Myc (50). Our results also showed that Triptonide regulated c-Myc at the transcriptional level to induce apoptosis of CAL-27 cells. A combination of Triptonide and c-Myc-specific siRNA could induce more apoptotic cells than Triptonide used alone, therefore, we suspected that Triptonide could induce the apoptosis of CAL-27 cells through other signaling pathways, such as ERK/MAPK pathway (51).
Several conceptual and practical difficulties, including the lack of defined “pockets” and potential toxicity to normal tissues have led to c-Myc being difficult to target (52). Recent strategies of targeting c-Myc indirectly, such as with BRD4 or CDK7 inhibitors showed a therapeutic window for targeting c-Myc, alleviating the above concerns (53, 54).Han et al. developed the c-Myc inhibitors with well tolerability that disrupted MYC/MAX heterodimerization, enhanced c-Myc degradation, and impaired c-Myc-driven gene expression to increase tumor immune cell infiltration, and sensitize tumors to anti-PD1 immunotherapy (27). The development of novel agents to inhibit c-Myc activity should be highly sought after and a very promising approach for applying targeted therapeutic strategies for cancer therapy.
Several study limitations could have affected our results as well. First, specific data on surgery, chemotherapy, radiotherapy, tumor size and other factors were not available to perform subgroup analyses. Second, the study is based on data from public databases, and the quality of data may affect the results. However, we obtained similar results by analyzing multiple databases. Third, it would be more informative to analyze the difference in immune cell infiltration between different c-MYC expression and TP53 mutation status, since TP53 also highly influences the immunogenic profiles. Forth, only CAL-27 cell line was used to justify the results of bioinformatics analysis and the effect on the cell line may be a random effect. Fifth, we chose Triptonide to interfere with c-Myc expression according to HERB database. In fact, targeting c-Myc by genetic ablation or pharmacological inhibitors may be the best choice to verify the role of c-Myc in HNSC. Lastly, we investigated the molecular functions and biological effects of c-Myc in HSCC by “in silico analysis”, which is within a matter of speculation. The molecular functions and biological effects of c-Myc which should be intensively studied in future investigations.
In conclusion, the current study showed that c-Myc can be considered a therapeutic target for HNSC. However, further functional studies are required to clarify the role of c-Myc in HNSC.
Data availability statement
The original contributions presented in the study are included in the article/Supplementary Material. Further inquiries can be directed to the corresponding authors.
Ethics statement
The studies involving human participants were reviewed and approved by institutional review board of Nanjing Stomatological Hospital. The patients/participants provided their written informed consent to participate in this study.
Author contributions
All authors made a significant contribution to the work reported, whether that is in the conception, study design, execution, acquisition of data, analysis and interpretation, or in all these areas; took part in drafting, revising or critically reviewing the article; gave final approval of the version to be published; have agreed on the journal to which the article has been submitted; and agree to be accountable for all aspects of the work.
Funding
Jiangsu Provincial traditional Chinese medicine science and technology development project, NO. 2021041. Nanjing special fundation for health science and technology development project, NO.ZKX21056.
Acknowledgments
Thanks are due to Dr. Jing Wang (ORCID 0000-0001-9981-5530) for assistance with the bioinformatic analysis.
Conflict of interest
The authors declare that the research was conducted in the absence of any commercial or financial relationships that could be construed as a potential conflict of interest.
Publisher’s note
All claims expressed in this article are solely those of the authors and do not necessarily represent those of their affiliated organizations, or those of the publisher, the editors and the reviewers. Any product that may be evaluated in this article, or claim that may be made by its manufacturer, is not guaranteed or endorsed by the publisher.
Supplementary material
The Supplementary Material for this article can be found online at: https://www.frontiersin.org/articles/10.3389/fonc.2022.820587/full#supplementary-material
References
1. Bray F, Ferlay J, Soerjomataram I, Siegel RL, Torre LA, Jemal A. Global cancer statistics 2018: GLOBOCAN estimates of incidence and mortality worldwide for 36 cancers in 185 countries. CA Cancer J Clin (2018) 68(6):394–424. doi: 10.3322/caac.21492
2. Pulte D, Brenner H. Changes in survival in head and neck cancers in the late 20th and early 21st century: A period analysis. Oncologist (2010) 15(9):994–1001. doi: 10.1634/theoncologist.2009-0289
3. León X, Hitt R, Constenla M, Rocca A, Stupp R, Kovács AF, et al. A retrospective analysis of the outcome of patients with recurrent and/or metastatic squamous cell carcinoma of the head and neck refractory to a platinum-based chemotherapy. Clin Oncol (R Coll Radiol) (2005) 17(6):418–24. doi: 10.1016/j.clon.2005.02.014
4. Yi L, Wu G, Guo L, Zou X, Huang P. Comprehensive analysis of the PD-L1 and immune infiltrates of m6A RNA methylation regulators in head and neck squamous cell carcinoma. Mol Ther Nucleic Acids (2020) 21:299–314. doi: 10.1016/j.omtn.2020.06.001
5. Deng X, Jiang Q, Liu Z, Chen W. Clinical significance of an m6A reader gene, IGF2BP2, in head and neck squamous cell carcinoma. Front Mol Biosci (2020) 7:68. doi: 10.3389/fmolb.2020.00068
6. Sakai A, Ando M, Fukusumi T, Ren S, Liu C, Qualliotine J, et al. Aberrant expression of CPSF1 promotes head and neck squamous cell carcinoma via regulating alternative splicing. PloS One (2020) 15(5):e0233380. doi: 10.1371/journal.pone.0233380
7. Croce CM, Thierfelder W, Erikson J, Nishikura K, Finan J, Lenoir GM, et al. Transcriptional activation of an unrearranged and untranslocated c-myc oncogene by translocation of a c lambda locus in burkitt. Proc Natl Acad Sci U S A (1983) 80(22):6922–6. doi: 10.1073/pnas.80.22.6922
8. Baltaci E, Karaman E, Dalay N, Buyru N. Analysıs of gene copy number changes ın head and neck cancer. Clin Otolaryngol (2018) 43(4):1004–9. doi: 10.1111/coa.12686
9. Bhattacharya N, Roy A, Roy B, Roychoudhury S, Panda CK. MYC gene amplification reveals clinical association with head and neck squamous cell carcinoma in Indian patients. J Oral Pathol Med (2009) 38(10):759–63. doi: 10.1111/j.1600-0714.2009.00781.x
10. Ganesan S. MYC, PARP1, and chemoresistance: BIN there, done that? Sci Signal (2011) 4(166):pe15. doi: 10.1126/scisignal.2001946
11. Wang WJ, Wu SP, Liu JB, Shi YS, Huang X, Zhang QB, et al. MYC regulation of CHK1 and CHK2 promotes radioresistance in a stem cell-like population of nasopharyngeal carcinoma cells. Cancer Res (2013) 73(3):1219–31. doi: 10.1158/0008-5472.CAN-12-1408
12. Chandrashekar DS, Bashel B, Balasubramanya SAH, Creighton CJ, Ponce-Rodriguez I, Chakravarthi BVSK, et al. UALCAN: A portal for facilitating tumor subgroup gene expression and survival analyses. Neoplasia (2017) 19(8):649–58. doi: 10.1016/j.neo.2017.05.002
13. Li T, Fu J, Zeng Z, Cohen D, Li J, Chen Q, et al. TIMER2.0 for analysis of tumor-infiltrating immune cells. Nucleic Acids Res (2020) 48(W1):W509–14. doi: 10.1093/nar/gkaa407
14. Tang Z, Li C, Kang B, Gao G, Li C, Zhang Z. GEPIA: a web server for cancer and normal gene expression profiling and interactive analyses. Nucleic Acids Res (2017) 45(W1):W98–W102. doi: 10.1093/nar/gkx247
15. Győrffy B. Survival analysis across the entire transcriptome identifies biomarkers with the highest prognostic power in breast cancer. Comput Struct Biotechnol J (2021)19:4101–09. doi: 10.1016/j.csbj.2021.07.014
16. Anaya J. Guide to using OncoLnc, a new TCGA data portal. Figshare (2016). doi: 10.6084/m9.figshare.2991640.v1
17. Vasaikar SV, Straub P, Wang J, Zhang B. LinkedOmics: analyzing multi-omics data within and across 32 cancer types. Nucleic Acids Res (2018) 46(D1):D956–63. doi: 10.1093/nar/gkx1090
18. Liu CJ, Hu FF, Xia MX, Han L, Zhang Q, Guo AY. GSCALite: a web server for gene set cancer analysis. Bioinformatics (2018) 34(21):3771–2. doi: 10.1093/bioinformatics/bty411
19. Charoentong P, Finotello F, Angelova M, Mayer C, Efremova M, Rieder D, et al. Pan-cancer immunogenomic analyses reveal genotype-immunophenotype relationships and predictors of response to checkpoint blockade. Cell Rep (2017) 18(1):248–62. doi: 10.1016/j.celrep.2016.12.019
20. Wang J, Zhou M, Xu JY, Chen B, Ouyang J. Combination of BCL-2 and MYC protein expression improves high-risk stratification in diffuse large b-cell lymphoma. Oncol Targets Ther (2015) 8:2645–50. doi: 10.2147/OTT.S86093
21. Xu J, Shen D, Zhang T, Wang J, De W, Zhang J. Lymphocyte-activated gene-3 (LAG3) protein expressed in tumor-infiltrating lymphocytes of colorectal cancer. Pol J Pathol (2021) 72(4):324–30. doi: 10.5114/pjp.2021.114177
22. Li S, Zhang S, Chen J. C-myc induced upregulation of long non-coding RNA SNHG16 enhances progression and carcinogenesis in oral squamous cell carcinoma. Cancer Gene Ther (2019) 26(11-12):400–10. doi: 10.1038/s41417-018-0072-8
23. Desrichard A, Kuo F, Chowell D, Lee KW, Riaz N, Wong RJ, et al. Tobacco smoking-associated alterations in the immune microenvironment of squamous cell carcinomas. J Natl Cancer Inst (2018) 110(12):1386–92. doi: 10.1093/jnci/djy060
24. Kim J, Bae JS. Tumor-associated macrophages and neutrophils in tumor microenvironment. Mediators Inflamm (2016) 2016:6058147. doi: 10.1155/2016/6058147
25. Kortlever RM, Sodir NM, Wilson CH, Burkhart DL, Pellegrinet L, Brown Swigart L, et al. Myc cooperates with ras by programming inflammation and immune suppression. Cell (2017) 171(6):1301–1315.e14. doi: 10.1016/j.cell.2017.11.013
26. Casacuberta-Serra S, Soucek L. Myc and ras, the bonnie and Clyde of immune evasion. Transl Cancer Res (2018) 7(Suppl 4):S457–9. doi: 10.21037/tcr.2018.03.09
27. Han H, Jain AD, Truica MI, Izquierdo-Ferrer J, Anker JF, Lysy B, et al. Small-molecule MYC inhibitors suppress tumor growth and enhance immunotherapy. Cancer Cell (2019) 36(5):483–97. doi: 10.1016/j.ccell.2019.10.001
28. Esser AK, Ross MH, Fontana F, Su X, Gabay A, Fox GC, et al. Nanotherapy delivery of c-myc inhibitor targets protumor macrophages and preserves antitumor macrophages in breast cancer. Theranostics (2020) 10(17):7510–26. doi: 10.7150/thno.44523
29. Zhou X, Liu X, Yang X, Wang L, Hong Y, Lian K, et al. Tumor progress intercept by intervening in caveolin-1 related intercellular communication via ROS-sensitive c-myc targeting therapy. Biomaterials (2021) 275:120958. doi: 10.1016/j.biomaterials.2021.120958
30. Cho KM, Kim MS, Jung HJ, Choi EJ, Kim TS. Mst1-deficiency induces hyperactivation of monocyte-derived dendritic cells via Akt1/c-myc pathway. Front Immunol (2019) 10:2142. doi: 10.3389/fimmu.2019.02142
31. Stritesky GL, Yeh N, Kaplan MH. IL-23 promotes maintenance but not commitment to the Th17 lineage. J Immunol (2008) 181(9):5948–55. doi: 10.4049/jimmunol.181.9.5948
32. Qian X, Gu L, Ning H, Zhang Y, Hsueh EC, Fu M, et al. Increased Th17 cells in the tumor microenvironment is mediated by IL-23 via tumor-secreted prostaglandin E2. J Immunol (2013) 190(11):5894–902. doi: 10.4049/jimmunol.1203141
33. Bunte K, Beikler T. Th17 cells and the IL-23/IL-17 axis in the pathogenesis of periodontitis and immune-mediated inflammatory diseases. Int J Mol Sci (2019) 20(14):3394. doi: 10.3390/ijms20143394
34. Zhao Y, Huang X, Ding TW, Gong Z. Enhanced angiogenesis, hypoxia and neutrophil recruitment during myc-induced liver tumorigenesis in zebrafish. Sci Rep (2016) 6:31952. doi: 10.1038/srep31952
35. Caponio VCA, Troiano G, Adipietro I, Zhurakivska K, Arena C, Mangieri D, et al. Computational analysis of TP53 mutational landscape unveils key prognostic signatures and distinct pathobiological pathways in head and neck squamous cell cancer. Br J Cancer (2020) 123(8):1302–14. doi: 10.1038/s41416-020-0984-6
36. Cancer Genome Atlas Network. Comprehensive genomic characterization of head and neck squamous cell carcinomas. Nature (2015) 517(7536):576–82. doi: 10.1038/nature14129
37. Oren M, Rotter V. Mutant p53 gain-of-function in cancer. Cold Spring Harb Perspect Biol (2010) 2(2)::a001107. doi: 10.1101/cshperspect.a001107
38. Ganci F, Pulito C, Valsoni S, Sacconi A, Turco C, Vahabi M, et al. PI3K inhibitors curtail MYC-dependent mutant p53 gain-of-Function in head and neck squamous cell carcinoma. Clin Cancer Res (2020) 26(12):2956–71. doi: 10.1158/1078-0432.CCR-19-2485
39. Liao P, Zeng SX, Zhou X, Chen T, Zhou F, Cao B, et al. Mutant p53 gains its function via c-myc activation upon CDK4 phosphorylation at serine 249 and consequent PIN1 binding. Mol Cell (2017) 68(6):1134–46. doi: 10.1016/j.molcel.2017.11.006
40. Frazier MW, He X, Wang J, Gu Z, Cleveland JL, Zambetti GP. Activation of c-myc gene expression by tumor-derived p53 mutants requires a discrete c-terminal domain. Mol Cell Biol (1998) 18(7):3735–43. doi: 10.1128/MCB.18.7.3735
41. Waitzberg AF, Nonogaki S, Nishimoto IN, Kowalski LP, Miguel RE, Brentani RR, et al. Clinical significance of c-myc and p53 expression in head and neck squamous cell carcinomas. Cancer Detect Prev (2004) 28(3):178–86. doi: 10.1016/j.cdp.2004.02.003
42. Wang H, Liao P, Zeng SX, Lu H. Co-Targeting p53-R249S and CDK4 synergistically suppresses survival of hepatocellular carcinoma cells. Cancer Biol Ther (2020) 21(3):269–77. doi: 10.1080/15384047.2019.1685289
43. Glorieux C, Xia X, Huang P. The role of oncogenes and redox signaling in the regulation of PD-L1 in cancer. Cancers (Basel) (2021) 13(17):4426. doi: 10.3390/cancers13174426
44. Zou J, Zhuang M, Yu X, Li N, Mao R, Wang Z, et al. MYC inhibition increases PD-L1 expression induced by IFN-γ in hepatocellular carcinoma cells. Mol Immunol (2018) 101:203–9. doi: 10.1016/j.molimm.2018.07.006
45. Bandukwala HS, Gagnon J, Togher S, Greenbaum JA, Lamperti ED, Parr NJ, et al. Selective inhibition of CD4+ T-cell cytokine production and autoimmunity by BET protein and c-myc inhibitors. Proc Natl Acad Sci U S A (2012) 109(36):14532–7. doi: 10.1073/pnas.1212264109
46. Mittal AK, Chaturvedi NK, Rohlfsen RA, Gupta P, Joshi AD, Hegde GV, et al. Role of CTLA4 in the proliferation and survival of chronic lymphocytic leukemia. PloS One (2013) 8(8):e70352. doi: 10.1371/journal.pone.0070352
47. Liu JN, Kong XS, Huang T, Wang R, Li W, Chen QF. Clinical implications of aberrant PD-1 and CTLA4 expression for cancer immunity and prognosis: A pan-cancer study. Front Immunol (2020) 11:2048. doi: 10.3389/fimmu.2020.02048
48. Fang S, Dong L, Liu L, Guo J, Zhao L, Zhang J, et al. HERB: a high-throughput experiment- and reference-guided database of traditional Chinese medicine. Nucleic Acids Res (2021) 49(D1):D1197–206. doi: 10.1093/nar/gkaa1063
49. Leuenroth SJ, Okuhara D, Shotwell JD, Markowitz GS, Yu Z, Somlo S, et al. Triptolide is a traditional Chinese medicine-derived inhibitor of polycystic kidney disease. Proc Natl Acad Sci U S A (2007) 104(11):4389–94. doi: 10.1073/pnas.0700499104
50. Wang SS, Lv Y, Xu XC, Zuo Y, Song Y, Wu GP, et al. Triptonide inhibits human nasopharyngeal carcinoma cell growth via disrupting lnc-RNA THOR-IGF2BP1 signaling. Cancer Lett (2019) 443:13–24. doi: 10.1016/j.canlet.2018.11.028
51. Zheng L, Fang S, Hui J, Rajamanickam V, Chen M, Weng Q, et al. Triptonide modulates MAPK signaling pathways and exerts anticancer effects via ER stress-mediated apoptosis induction in human osteosarcoma cells. Cancer Manag Res (2020) 12:5919–29. doi: 10.2147/CMAR.S258203
52. McKeown MR, Bradner JE. Therapeutic strategies to inhibit MYC. Cold Spring Harb Perspect Med (2014) 4(10):a014266. doi: 10.1101/cshperspect.a014266
53. Yin M, Guo Y, Hu R, Cai WL, Li Y, Pei S, et al. Potent BRD4 inhibitor suppresses cancer cell-macrophage interaction. Nat Commun (2020) 11(1):1833. doi: 10.1038/s41467-020-15290-0
Keywords: head and neck squamous cell carcinoma, c-Myc, survival, mRNA, triptonide
Citation: Zhao S, An L, Yang X, Wei Z, Zhang H and Wang Y (2022) Identification and validation of the role of c-Myc in head and neck squamous cell carcinoma. Front. Oncol. 12:820587. doi: 10.3389/fonc.2022.820587
Received: 23 November 2021; Accepted: 10 August 2022;
Published: 31 August 2022.
Edited by:
Steve Oghumu, The Ohio State University, United StatesReviewed by:
Sk. Kayum Alam, University of Minnesota Twin Cities, United StatesNetty Santoso, The Ohio State University, United States
Copyright © 2022 Zhao, An, Yang, Wei, Zhang and Wang. This is an open-access article distributed under the terms of the Creative Commons Attribution License (CC BY). The use, distribution or reproduction in other forums is permitted, provided the original author(s) and the copyright owner(s) are credited and that the original publication in this journal is cited, in accordance with accepted academic practice. No use, distribution or reproduction is permitted which does not comply with these terms.
*Correspondence: Sufeng Zhao, doctorsfz@yeah.net; Xudong Yang, zsfnjkq@163.com
†These authors have contributed equally to this work