Dietary Choline-Enhanced Skin Immune Response of Juvenile Grass Carp Might Be Related to the STAT3 and NF-kB Signaling Pathway (Ctenopharyngodon idella)
- 1Animal Nutrition Institute, Sichuan Agricultural University, Chengdu, China
- 2Fish Nutrition and Safety Production University Key Laboratory of Sichuan Province, Sichuan Agricultural University, Chengdu, China
- 3Key Laboratory for Animal Disease-Resistance Nutrition of China Ministry of Education, Sichuan Agricultural University, Chengdu, China
- 4Animal Nutrition Institute, Sichuan Academy of Animal Science, Chengdu, China
To investigate the effects and potential mechanisms of dietary choline on immune function in the skin of juvenile grass carp (Ctenopharyngodon idella), fish were fed different diets containing different levels of choline (142. 2, 407.4, 821.6, 1215.8, 1589.3, and 1996.6 mg/kg) for 70 d and then sampled after a 6-d challenge test. The results exhibited that dietary choline (1) advanced the contents of phosphatidylcholine (PC), betaine, and choline in grass carp skin (P < 0.05) and upregulated the mRNA abundance of choline transporter high-affinity choline transporter 1 (CHT1), choline transporter-like protein 1 (CTL1), and choline transporter-like protein 5 (CTL5), indicating that dietary choline could increase the contents of choline which might be connected with choline transporters in the grass carp skin; (2) receded skin rot symptom after infection with A. hydrophila (Aeromonas hydrophila), increased the levels of IgM, C4, and C3 and the activities of acid phosphatase (ACP) and lysozyme (LZ), raised mucin2, β-defensin, hepcidin, and LEAP-2B mRNA abundance (rather than LEAP-2A), downregulated pro-inflammatory cytokine mRNA abundance (IFN-γ2, IL-15, TNF-α, IL-6, IL-12P40, and IL-1β) in skin of juvenile grass carp (P < 0.05), and upregulated anti-inflammatory cytokine mRNA abundance (IL-10, IL-4/13A, TGF-β1, IL-11, and IL-4/13B) in grass carp skin (P < 0.05), demonstrating that choline enhanced the skin immune function; and (3) downregulated the mRNA abundance of IKKγ, NF-κBp52, IKKβ, c-Rel, NF-κBp65, STAT3b2, STAT3b1, JAK1, and JAK2 as well as protein level of NF-κBp65, p-STAT3 Tyr705, and p-STAT3 Ser727 in nucleus and inhibited the mRNA and protein level of IkBα (P < 0.05), indicating that choline-enhanced immune function might be relevant to the JAK1, 2 /STAT3, and NF-κB signaling pathway in fish skin. In conclusion, choline enhanced the skin immune function which might be related to JAK1, 2/STAT3, and NF-κB signaling molecules in fish. Furthermore, based on immune indices of grass carp (9.28–108.97 g) skin (C3 and IgM contents as well as ACP activities), the choline requirements were estimated to be 1475.81, 1364.24, and 1574.37 mg/kg diet, respectively.
Introduction
Choline is an indispensable vitamin B for fish (1). Our previous study found that optimal choline supplementation promoted the growth performances of grass carp (Ctenopharyngodon idella) (2, 3). Skin is a crucial immune organ in fish (4), whose health is important for fish growth and disease resistance (5). Nevertheless, no studies had reported the effect of choline on grass carp skin. Intensive aquaculture increases disease infection risk of fish (6). Improving immunity is crucial for the prevention and control of diseases in fish (7). Fish skin immune function is tightly correlated with specific immune factors like immunoglobulins and non-specific immune factors like LZ (2). Furthermore, fish skin immunity is closely related to the cytokines. NF-κB and STAT3 each control the expression of a large number of downstream genes that control cell proliferation, survival, stress responses, and immune functions. Some of the target genes for NF-κB and STAT3 overlap, and in addition, the two transcription factors are engaged in both positive and negative cross talk (8). Murray (9) found that anti-inflammatory cytokines could be regulated by STAT3 as well as upstream signaling molecule Janus kinases (JAK) in humans (9), while pro-inflammatory cytokine expression could be mediated by NF-κB (10). Choline enters the cell via choline transporters and produces corresponding metabolites to play biological functions (11). Betaine is one of vital metabolites which could decrease the content of tumor necrosis factor-α (TNF-α) in rat liver (12, 13). In mouse brain, choline enhanced the acetylcholine (ACh) level which could activate the JAK2/STAT3 signaling pathway in mouse PC12 cells (14, 15). In human, choline synthesis PC restrained the NF-κBp65 protein level in rat IEC-6 cells (16, 17). Thus, there might be a relationship between immune function and choline, which may be related to the JAK/STAT3 and NF-κB signaling pathway in fish skin.
Most researches of vitamins concentrated on liver health and nutritional requirements (1, 18). So far, reports regarding the impacts of vitamin on fish skin immunity are scattered. However, the following restrictions still remain: (1) in limited researches, we found that those researches are not deeply and systematically enough. Most studies mainly focused on the antibiotic substance contents and the inflammatory factor gene expression and did not investigate the involved mechanisms (19, 20). (2) The in-depth mechanisms of various vitamins on fish skin immune function are contrasting. For example, dietary VB7 supplement-enhanced skin immune function might be connected with key enzyme activity such as acid phosphatase (ACP) (20), whereas VC did not change the activity of ACP in fish skin (19). Hence, it is necessary to systematically research the impacts of choline on fish skin immunity.
In the current study, the growth trial was similar to our prior study. The study determines the dietary choline-enhanced fish growth performances (3) which were influenced by the skin immune function (21). Grass carp (Ctenopharyngodon idella) is a typical herbivorous finfish without stomach. In its natural environment, it eats water plant. Grass carp is the largest aquaculture of fish in China. The breeding of grass carp is of great significance to the whole fishery of China (22). Red skin disease is one of the main diseases threatening fish culture, which often occurs in grass carp. Hence, we explored the influences of choline on fish skin immune function, which partially declared the impact of choline on skin immune function and underlying mechanisms in fish. Simultaneously, vitamin requirements based on fish production performance are lower than the immune function of juvenile grass carp (19), so we determined the optimum choline requirements depending on the immune indicator for juvenile grass carp, which may provide the basis for production practice.
Materials and Methods
Experiments and Feeding Management
The current study used the same animal trial as our prior research (3). The feed formula is shown in Table 1, which was commensurate with prior research. Fishes were fed with six various gradient choline diets for 70 days. The choline levels were 142.2 (0), 407.4 (400), 821.6 (800), 1215.8 (1,200), 1589.3 (1,600), and 1996.6 (2,000) mg/kg in six diets, respectively (the value in front of the brackets is the measured value, and the value in the brackets is the design value). The actual choline level was determined by Ding and Mou (25).
All experimental procedures were approved by the Animal Protection Advisory Committee of Sichuan Agricultural University (23). Fishes were purchased from the fishery (Chengdu, China). Before the experiment, a 4-week feeding was adapted to the environment. After that, 1,440 fishes with average weight of 9.29 g were randomly assigned to 36 aquariums (0.144 m3). The same continuous aeration and recirculating water was maintained to each aquarium (26). During the growth experiment, the dissolved oxygen level ranged from 6.2 mg/L to 7.0 mg/L; pH was measured at 7.0 ± 0.3 and water temperature at 28 ± 2°C. Fishes were fed four times every day (23, 27).
Challenge Test
The bacteria were supplied by the College of Veterinary Medicine, Sichuan Agricultural University. Prior to initiation of the challenge test, based on skin lesion morbidity and without causing any death, we determined an appropriate challenge concentration (2.1 × 106 CFU/ml) after injection-graded levels (physiological saline, 2.1 × 105, 2.1 × 106, 2.1 × 107, and 2.1 × 108 CFU/ml) of Aeromonas hydrophila (A. hydrophila) in fish within 6 days (28).
After a 70-day growth experiment, we used a challenge test to investigate the effect of choline diets on fish immune function of juvenile grass carp. Forty-two fishes (same weight) from each treatment were intraperitoneal challenge injection with 2.1 × 106 CFU/ml A. hydrophila. After injection for 6 days, the symptoms were recorded and the skin of each group was put into liquid nitrogen and reserved in −80°C.
Measurement of Choline Metabolite Contents and Immune Parameter Activities
A preparation of 10% skin tissue homogenate was used to measure choline metabolite content and antibacterial material level as previously described (6). IgM, C4, C3 ACP, and LZ were determined using the corresponding kit (Nanjing Jiancheng Bioengineering Institute, Nanjing, China) following the manufacturer's protocols according to Takemura (29) and Huang et al. (30). LZ used turbidimetry; ACP used the colorimetric method; and IgM, C4, and C3 used immunoturbidimetry. The levels of ACh, betaine, PC, and choline were determined by ELISA kits (Shanghai Kexing Trading Co., Ltd, China) following the manufacturer's protocols and measured spectrophotometrically at a wavelength of 450 nm. The levels of ACh, betaine, PC, and choline were calculated on the basis of standard curves.
Quantitative Real-Time PCR
The skin total RNA was extracted using the RNAiso Plus kit (TaKaRa Bio Inc., Japan) following the specification. Thenceforth, the instruction was referred to synthase cDNA using the PrimeScript™ RT Reagent Kit (TaKaRa). The qRT-PCR primers were referred to the published sequences of grass carp in our lab prior to the study, which are shown in Table 2. The gene amplification efficiency was measured by the melting curve. The 2−ΔΔCT method was used to calculate the gene expression as described by Yao et al. (31) and Zhang et al. (32).
Western Blotting
Lysis buffer, protease inhibitor cocktail, and phosphatase inhibitor cocktail were used to prepare skin homogenate (10). The protein level was determined by BCA assay kit (Beyotime Biotechnology Inc.). β-Actin, laminB1, p-STAT3Tyr705, NF-κBp65, and IκBα antibodies are similar to our early research (28, 33, 34). P-STAT3Ser727 antibodies were purchased from commercial antibodies (Hua'an Biology Co., Ltd.). LaminB1 and β-actin were used as control proteins for nuclei and cell total proteins, respectively. Equal amounts of protein were separated by sodium dodecyl sulfate polyacrylamide gel electrophoresis (SDS-PAGE) and transferred to a PVDF membrane. Membranes were blocked for 1 h at room temperature (RT) before being washed thrice with TBST (10 min each) and incubated with a primary antibody overnight at 4°C. Next, the membranes were again washed three times before incubation with an HRP-conjugated secondary antibody in TBST for 2 h. Immune complexes were visualized with an ECL kit (Millipore). Densitometric analyses of the protein bands were performed in ImageJ (NIH, USA). Different treatments were expressed relative to the level observed in the control group. The experiment was repeated at least three times, and similar results were obtained each time.
Statistical Analysis
The Shapiro–Wilk and Levene tests were used to test the homogeneity and normal distribution of variance by SAS 8.1 (SAS Institute), respectively. One-way variance (ANOVA) was used to analyze the data (35). The significant differences between every treatment means were contrasted by Duncan's multiple-range tests (significant difference at the 5% level of significance, P < 0.05) (36). The choline requirement and correlations were estimated by broken-line mode and Pearson's correlation, respectively (23).
Results
The Contents of Choline and Its Metabolites and the Gene Expression of the Choline Transporter
As shown in Table 3, the levels of PC, betaine, and choline were increased with dietary choline up to 1589.3, 1589.3, and 1215.8 mg/kg (P < 0.05), respectively, and then plateaued. However, the content of ACh did not change with the increase in choline content (P > 0.05). The choline transporter mRNA abundance of skin is exhibited in Figure 1; CTL2 and CTL5 mRNA abundance was raised with dietary choline by 1215.8 and 1589.3 mg/kg (P < 0.05), respectively, then plateaued. CHT1 mRNA abundance was slowly increased with dietary choline addition (P < 0.05). Dietary choline did not change the mRNA abundance of CTL1 and CTL4 in fish skin (P > 0.05).
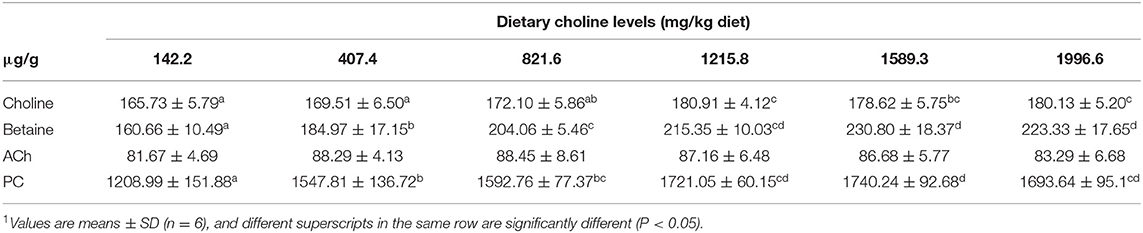
Table 3. Effects of dietary choline (mg/kg diet) on choline, phosphatidylcholine (PC), betaine, and acetylcholine (ACh) contents in the skin of grass carp (Ctenopharyngodon idella)1.
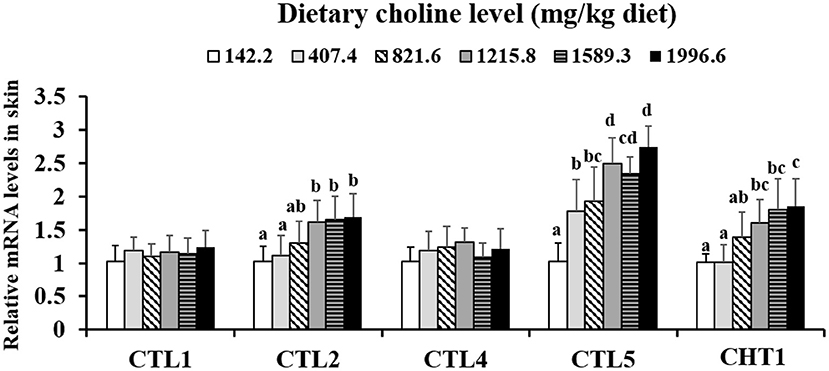
Figure 1. Effects of dietary choline on choline transporter gene level in the skin of juvenile grass carp (Ctenopharyngodon idella) after infection with A. hydrophila. Data represent means of six fish in each group, error bars indicate S.D. Values having different letters are significantly different (P < 0.05).
Skin Rot Morbidity and Activities of Immune Parameters in Grass Carp Skin
Choline insufficiency caused skin rot symptom, as shown in Figure 2. As exhibited in Table 4, the activities of ACP and LZ in grass carp skin increased as the dietary choline level increased to 1215.8 mg/kg (P < 0.05), then plateaued. The levels of C3, IgM, and C4 in the skin increased as dietary choline supplements to 1589.3, 821.6, and 1215.8 mg/kg (P < 0.05), respectively, then all plateaued. The antibacterial peptide mRNA abundance of the skin is presented in Figure 3A; mucin2 and LEAP-2B mRNA abundance was raised with dietary choline level addition to 1215.8 and 821.6 mg/kg (P < 0.05), respectively, then plateaued. The mRNA levels of hepcidin and β-defensin-1 increased with choline supplements to 1589.3 mg/kg (P < 0.05), then decreased. Nevertheless, mRNA abundance of LEAP-2A was not influenced by choline (P > 0.05). As exhibited in Figure 3B, the mRNA abundance of TNF-α, IL-15, IL-6, IL-12p40, and IL-1β in skin of juvenile grass carp decreased with dietary choline level increase to 821.6, 1215.8, 1589.3, 1215.8, and 1215.8 mg/kg, respectively (P < 0.05), and then plateaued. The mRNA abundance of IFN-γ2 in skin of juvenile grass carp decreased with dietary choline addition (P < 0.05). In Figure 3C, the mRNA abundance of anti-inflammatory cytokine IL-4/13B, IL-10, IL-11, and IL-4/13A in skin of fish (P < 0.05) was elevated with choline level increase to 1589.3, 1215.8, 1589.3, and 1589.3 mg/kg, respectively (P < 0.05). TGF-β1 mRNA abundance in fish skin was elevated with dietary choline addition (P < 0.05). Remarkably, the mRNA abundance of IL-17D, TGF-β2, and IL-12p35 was not impacted by dietary choline (P > 0.05).
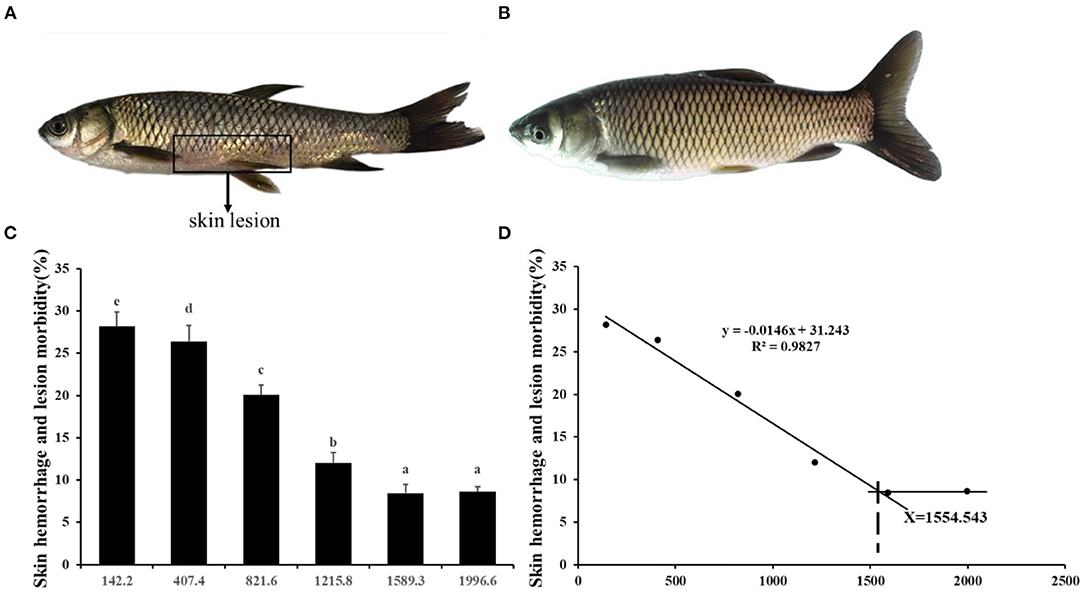
Figure 2. Effects of dietary choline level (mg/kg diet) on skin rot morbidity of juvenile grass carp (Ctenopharyngodon idella) after infection with A. hydrophila. (A) 142.2 mg/kg diet. (B) 1589.3 mg/kg diet. Values are means, and standard error of the mean represented by vertical bars. N = 6*5 for each choline level. Values having different letters are significantly different (P < 0.05). (C) Skin hemorrhage and lesion morbidity (%). (D) Choline requirement is assessed by morbidity.
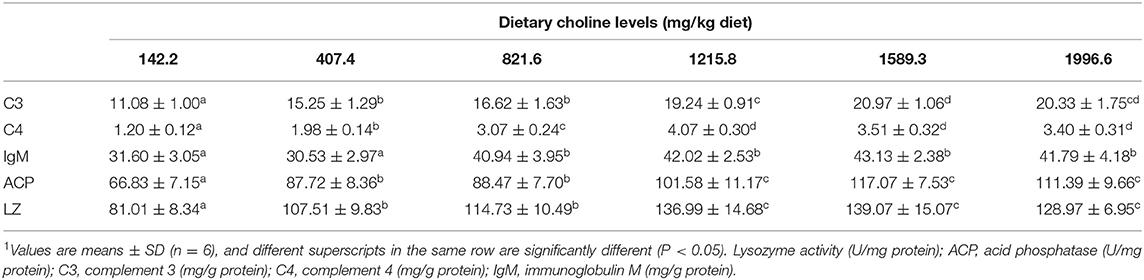
Table 4. Effects of dietary choline (mg/kg diet) on immune parameters in juvenile grass carp (Ctenopharyngodon idella) skin1.
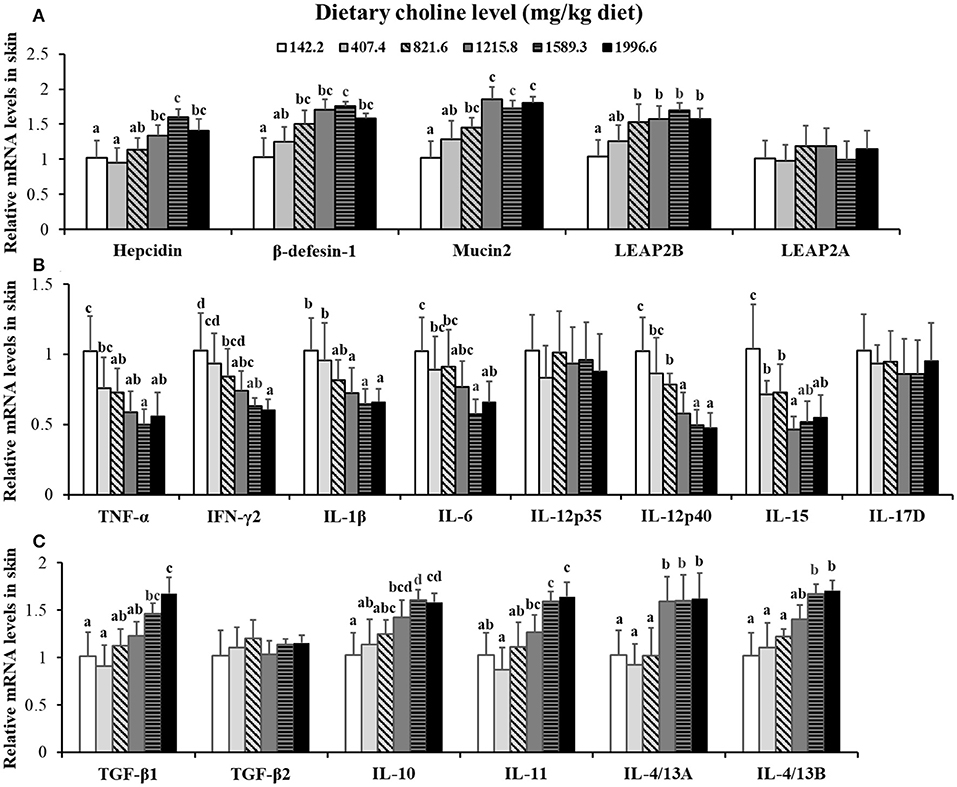
Figure 3. Effects of dietary choline on parameter mRNA levels in the skin of grass carp after infection with A. hydrophila. This analysis was repeated six times with similar results. Data represent means of six fish in each group, and error bars indicate S.D. Values having different letters are significantly different (P < 0.05). (A) Relative mRNA level of Hepcidin, β-defensin-1, Mucin2, LEAP-2A, and LEAP-2B in the skin. (B) Relative mRNA level of pro-inflammatory cytokines in the skin. (C) Relative mRNA levels of anti-inflammatory cytokines in the skin.
JAK/STAT3 Signaling Pathway and NK-kB Signaling Pathway mRNA Abundance in Fish Skin
As exhibited in Figure 4, in juvenile grass carp skin, the mRNA abundance of IKK-γ, NF-κB p65, c-Rel, NF-κB p52, and IKK-β decreased with dietary choline levels of 1589.3, 1215.8, 1215.8, 1589.3, and 1589.3 mg/kg, respectively (P < 0.05), and then plateaued. IKBα mRNA levels were increased with a dietary choline level up to 1589.3 (P < 0.05), and then plateaued. In Figure 5, the mRNA abundances of JAK1, JAK2, STAT3b1, and STAT3b2 increased with choline addition to 1215.8, 1589.3, 1589.3, and 1589.3 mg/kg, respectively (P < 0.05), and then plateaued. Tyk2 mRNA levels were highest in 407.4 mg/kg choline diet (P < 0.05). However, dietary choline had no influence on mRNA abundance of IKKα, JAK3, and STAT3a in skin of juvenile grass carp. As shown in Figure 6, the NF-κB p65 protein level in the nucleus decreased with choline supplements to 1215.8 mg/kg (P < 0.05) then plateaued. The IKBα, p-STAT3 Tyr705, and p-STAT3 Ser727 protein levels in the nucleus were elevated with dietary choline addition to 1589.5 mg/kg, respectively (P < 0.05).
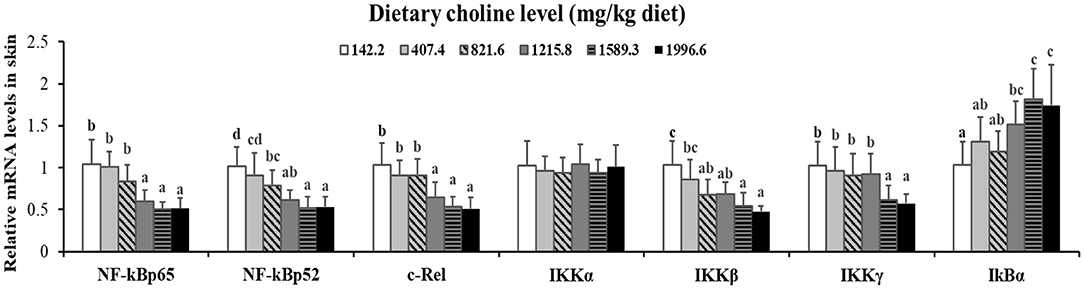
Figure 4. Effects of dietary choline level (mg/kg diet) on relative expression of NF-κB signaling molecules in skin of juvenile grass carp (Ctenopharyngodon idella) after infection with A. hydrophila. Data represent means of six fish in each group; error bars indicate S.D. Values having different letters are significantly different (P < 0.05).
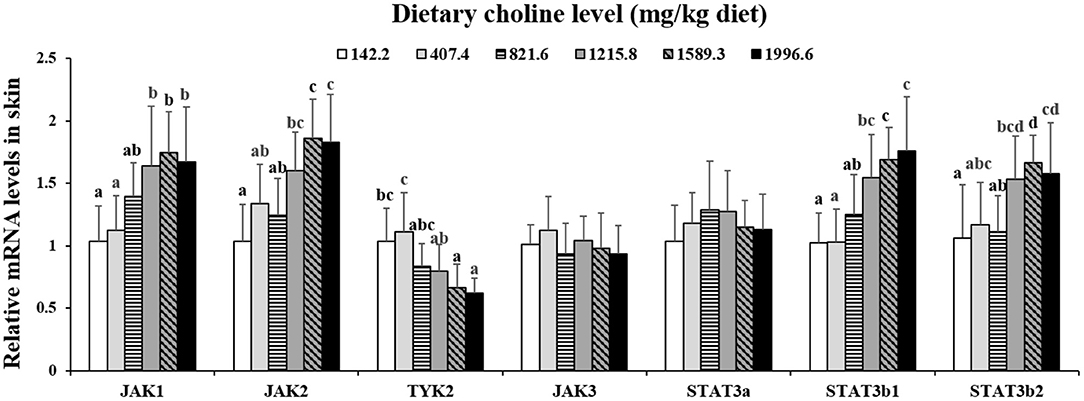
Figure 5. Effects of dietary choline level (mg/kg diet) on relative expression of JAK/STAT3 in skin of juvenile grass carp (Ctenopharyngodon idella) after infection with A. hydrophila. Data represent means of six fish in each group, error bars indicate S.D. Values having different letters are significantly different (P < 0.05).
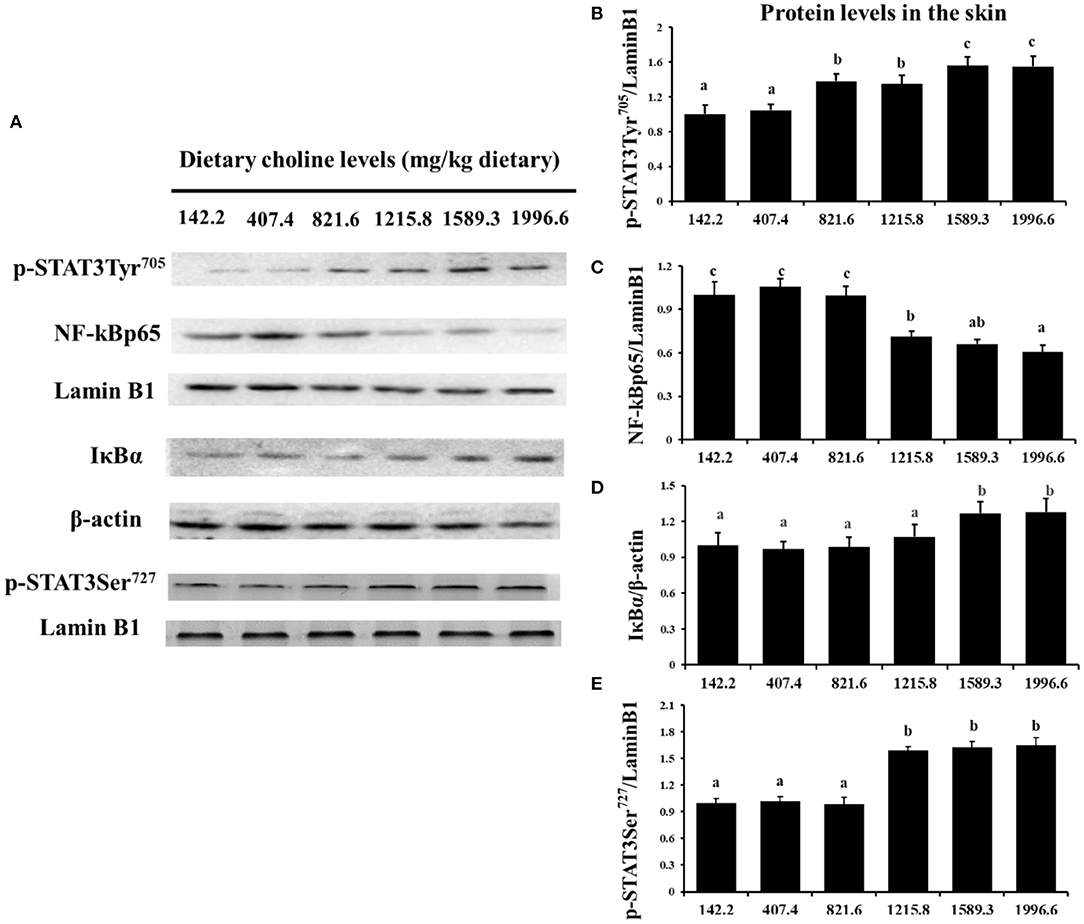
Figure 6. Western blot analysis of p-STAT3Tyr705, p-STAT3 Ser727, NF-κBp65, and IκBα protein level in the skin of juvenile grass carp fed diet containing different levels of choline after infection with A. hydrophila. Values are means (six replicates per group), and standard error represented by vertical bars. a,b,cMean values with unlike letters were significantly different between treatments (P < 0.05). (A) Protein bands of p-STAT3 Tyr705, NF-κBp65, and IκBα. (B) The relative protein expression of p-STAT3 Tyr705/laminB1. (C) The relative protein expression of NF-Kp65/laminB1. (D) The relative protein expression of IκBα/laminB1. (E) The relative protein expression of p-STAT3 Ser727/laminB1.
Discussion
This research used a growth experiment similar to our earlier study (3), which found choline improved fish growth performances of juvenile grass carp (3). Furthermore, fish growth performances were deeply related to the immune function of immune organs (28), Hence, we explored how choline impacts the skin immune function of fish in this study.
Dietary Choline Increased the Content of Choline and Its Metabolites and Upregulated the Gene Expression of Choline Transporter in Fish Skin
Dietary choline was absorbed by guts and through blood travels to different tissues where it can be oxidized, phosphorylated, and acetylated and can produce corresponding metabolites, such as betaine, PC, and ACh. In our research, dietary choline could increase the content of betaine, PC, and choline in fish skin. Results show that the optimal choline increased the choline content and corresponding metabolite in fish skin. In mammal enterocytes, choline can be transported through transport systems (11), such as choline transporter-like proteins (CTLs) and high-affinity choline transporter (CHT1) (11). In this research, we found that optimal dietary choline upregulated the mRNA levels of CTL2, CTL5, and CHT1, which indicated that skin absorbed choline through choline transporters CTL2, CTL5, and CHT1. Based on choline content and choline transporter mRNA abundance, the positive correlation is found in Table 5; we conjectured that the upregulation of those choline transporters' mRNA abundance might be related to the increase of choline contents in fish skin.
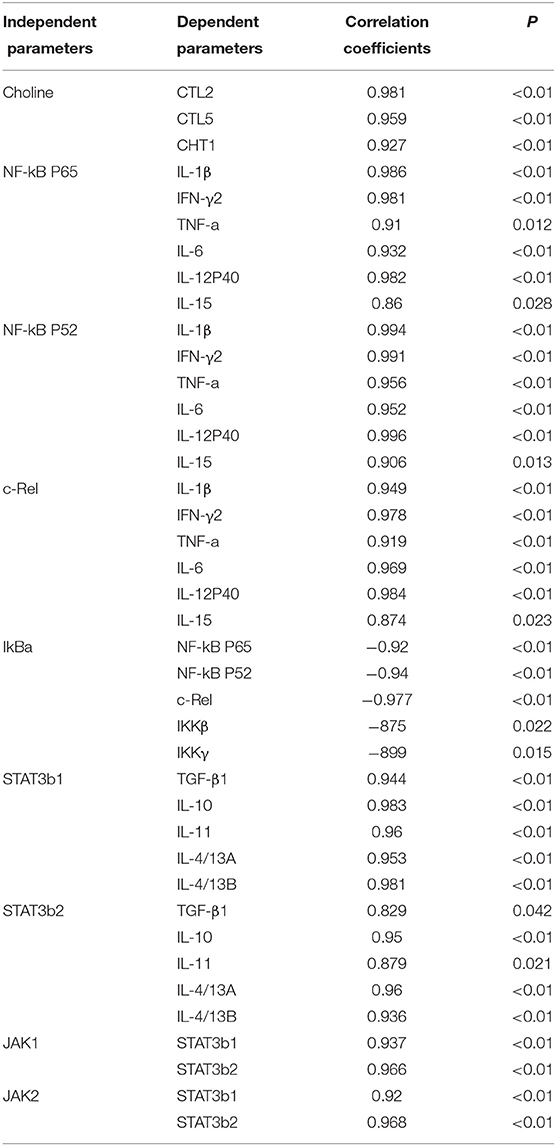
Table 5. Correlation analysis of parameters in the skin of juvenile grass carp (Ctenopharyngodon idella).
Strikingly, choline has no impact on fish skin CTL4 mRNA abundance, which might be associated with ACh. CTL4 is involved in the synthesis of ACh in animals (37). However, in our research, choline did not influence the ACh content of grass carp skin, which provides an important basis for our hypothesis.
Dietary Choline Reduced Skin Lesion Morbidity and Enhanced Immune Function in Fish Skin
A. hydrophila is a potentially pathogenic bacterium that can cause fish skin lesion and even lead to death (28). Hence, at the end of the growth test using A. hydrophila trail to study the skin lesion degree. During challenge with A. hydrophila, we found that dietary choline deficiency led to peak skin lesion morbidity (28.17%), whereas sufficient choline abundance decreased skin lesion morbidity (8.45%) in grass carp. Furthermore, the skin lesion resistance partially relies on skin immune response in fish (38). These results declared that sufficient dietary choline reduced skin lesion morbidity. In fish, the immune function was mainly influenced by antimicrobial peptides such as LEAP-2 and hepcidin and humoral components like C4 and IgM (39). The above results indicated that optimal dietary choline enhanced the ACP and LZ activities and C3, IgM, and C4 contents and increased mucin2, β-defensin LEAP-2B, and hepcidin mRNA abundance in fish skin. These results investigated that choline addition heightened the immune function in fish. The concentration of C4 in the optimal choline supplementation group was 3.39-fold that of the 142.2-mg/kg choline group, which was significantly higher than other enzyme activities and C3 content (1.36–1.89-fold). This indicates that the regulation of C4 by choline is more active and effective.
Interestingly, considering the differences between the vitamins mentioned in the introduction, we compared choline with VB7 and α-lipoic acid (20, 40). Unlike two other vitamins, choline deficiency did not affect the LEAP-2A mRNA level, which might be partly relevant to IKKα. IKKα improved the IL-22 level in mice (41), which enhanced LEAP-2A mRNA abundance in rainbow trout splenocytes (42). However, in this research, choline supplementation did not affect IKKα mRNA abundance in fish skin, which might support our hypothesis. Moreover, these data also illustrated that LEAP-2A might be more highly conscious by VB7 and α-lipoic acid in fish skin than dietary choline.
Dietary Choline Enhanced Immune Function Referring to the mRNA Abundance of Inflammatory Factors in Fish Skin
Inflammation is a host defense mechanism, but overregulation of the inflammatory response disrupts the balance of immune function, leading to the deterioration of human diseases. Morimoto et al. observed that the inflammatory function of fish immune organs was affected by inflammatory cytokines (43). However, no research has studied the impacts of choline addition on inflammatory response in fish skin. In this research, we for the first time investigated that choline addition decreased the mRNA level of proinflammatory cytokines IFN-γ2, TNF-α, IL-15, IL-12p40, IL-6, and IL-1β and upregulation of the mRNA abundance of anti-inflammatory cytokines TGF-β1, IL-11, IL-10, and IL-4/13A in grass carp skin. In short, all of the above results illustrated that dietary choline supplement improved the immune function in fish skin.
Interestingly, the potential reasons for differential result are discussed as follows. First, our previous research found that an appropriate dietary VB7 could descend the mRNA abundance of IL-12p35 (rather than IL-12p40) (20). In contrast, in our study, appropriate choline increased fish skin IL-12p40 (not IL-12p35) mRNA abundance. This result might be related to TNF-α. In this research, appropriate dietary choline downregulated TNF-α mRNA levels in grass carp skin. In Atlantic salmon HK cells, TNF-α could increase the IL-12p40 (not IL-12p35) gene level (44), which supports our speculation. Moreover, these data also indicated that choline and VB7 had different regulatory mechanisms between different subtypes of the same gene (such as IL-12p35 and IL-12p40). Second, dietary α-lipoic acid and VB7 decreased the mRNA abundance of IL-17D, but dietary choline did not influence the IL-17D mRNA abundance in fish skin which might be relevant to tryptophan. Tryptophan catabolites could inhibit the production of IL-17 in mice (45). Previous studies have found that choline did not affect the content of tryptophan in the intestine (3), which might support our hypothesis and needs further investigation. In addition, these data also indicated that IL-17D is more easily regulated by dietary choline rather than α-lipoic acid and VB7 in fish skin. Third, choline insufficiency did not influence the TGF-β2 mRNA abundance in fish skin, which might be partly related to methionine. Methionine regeneration was mediated by choline in bovine neonatal hepatocytes (46). Methionine dipeptide supplementation has no impact on the TGF-β2 mRNA abundance of grass carp intestine (47), which needs further study. Moreover, previous studies found that dietary α-lipoic acid could upregulate the mRNA abundance of TGF-β2, indicating that TGF-β2 is more easily regulated by dietary α-lipoic acid rather than choline in fish skin.
Dietary Choline Enhanced Immune Function Referring to JAK/STAT3 and NF-κB Signaling Molecules in the Fish Skin
A previous study found that inflammatory response is related to the mRNA expression of proinflammatory cytokines in fish (48). NF-κB p52, p65, and c-Rel are very important members of NF-κB signaling molecules which regulate the proinflammatory cytokines (49). NF-κB p65 and p52 were inhibited by IkBα in mammalian cells (50). Our data showed that the proinflammatory cytokine (IFN-γ2, IL1β, IL-6, TNF-α, IL-12p40, and IL-15) mRNA abundance was downregulated by optimal dietary choline in grass carp skin, implying that optimal dietary choline enhanced inflammatory function in fish skin. Furthermore, optimal dietary choline restrained the NF-κB signaling pathway by activating the IKBα protein level and decreasing the nuclear NFκBp65 protein level, as well as C-Rel, IKKβ, NF-κBp65, IKKγ, and NF-κBp52 (not IKKα) mRNA abundance in grass carp skin. C-Rel, NF-κB p52, and NF-κBp65 mRNA abundance positively correlated the pro-inflammatory cytokine mRNA abundance, shown in Table 5. At the same time, c-Rel, NF-κB p52, and NF-κB p65 mRNA abundance was positively related with IKKβ and IKKγ mRNA abundance, which was inversely correlated with IκBα mRNA abundance. These data elucidated that the optimal dietary choline downregulated the proinflammatory cytokine mRNA abundance which might be relevant to IKKβ and IKKγ/IκBα/NF-κB signaling in fish skin. Our results show that choline affects both canonical and non-canonical NF-κB signaling. However, there are few studies about the effect of choline on the NF-κB signaling pathway and only focus on canonical NF-κB. We speculate that cholesterol may be involved. Won et al. (51) found that plasma cholesterol was significantly influenced by the graded choline levels in juvenile olive flounder (Paralichthys olivaceus) (51). Optimal cholesterol inhibited both canonical and non-canonical NF-kappaB pathways in grass carp kidney and spleen (6), which might support our hypothesis and needs further investigation.
The STAT3 signaling pathway plays an important role in promoting the gene expressions of anti-inflammatory cytokines in humans (9). STAT3 (phosphorylation at tyrosine 705, Tyr705, and/or serine 727, Ser727) is also a member of the STAT family, which can be activated by the upstream signaling molecule Janus kinases (e.g., JAK2) (52). In this research, we for the first time investigated that choline addition increased the mRNA abundance of STAT3b1, STAT3b2, JAK1, and JAK2, as well as p-STAT3 Tyr705 and p-STAT3 Ser727 protein levels in fish skin (Figure 5), which implies that dietary choline upregulated most of anti-inflammatory cytokines which might be partly related to the STAT3 signaling pathway. Correlation analyses (Table 5) showed that anti-inflammatory cytokines (IL-10, TGF-β1, IL-4/13A, IL-11, and IL-4/13B) were positively correlated with the STAT3b1 and STAT3b2 mRNA abundance in fish skin. Our data showed that the weakened effect of the anti-inflammatory cytokine by dietary choline might be relevant to JAK1 and JAK2/STAT3 signaling in grass carp skin.
Interestingly, choline has no impact on IKKα mRNA abundance of grass carp skin, which may be relevant to IFN-γ. This research indicated that dietary choline deficiency increased fish skin IFN-γ mRNA abundance. In U937 cells, IFN-γ enhanced the protein kinase Cζ level (53), which increased IKKβ and IKKγ (not IKKα) gene abundance in Kupffer cells (54) Further research is needed to confirm this hypothesis. Similar phenomena were discovered in VB7, and α-lipoic acid from our previous research in grass carp skin, which may illustrate that vitamin B is not sensitive enough to IKKα in skin. Moreover, in human myeloid cells, the macrophage colony-stimulating factor (GM-CSF) could activate STAT3b (rather than STAT3a) (55). However, choline supplementation had no effect on GM-CSF concentrations in rat placenta (56). Therefore, we speculated that choline deficiency downregulated STAT3b1/b2 rather than STAT3a gene expressions in fish skin which may be caused by the decrease of GM-CSF. Further research is needed to confirm this hypothesis.
Choline Requirements Based on Immune Indices
The appropriate choline group advanced most of inflammatory indicators and receded skin lesion. Further, based on the skin immune indices (C3 and IgM contents as well as ACP activities) the choline requirements for grass carp (9.28–108.97 g) were estimated to be 1475.81, 1364.24, and 1574.37 mg/kg diet, respectively (As exhibited in Figure 7). The requirements for the majority of the skin immune index were higher than those for the growth (feed efficiency 1283.4 mg/kg diet) (3). Similar results were found in other vitamins like VB7 and VC in grass carp (19, 20). This result may illustrate that more choline or metabolites was needed for fish to resistance to bacterial infection.
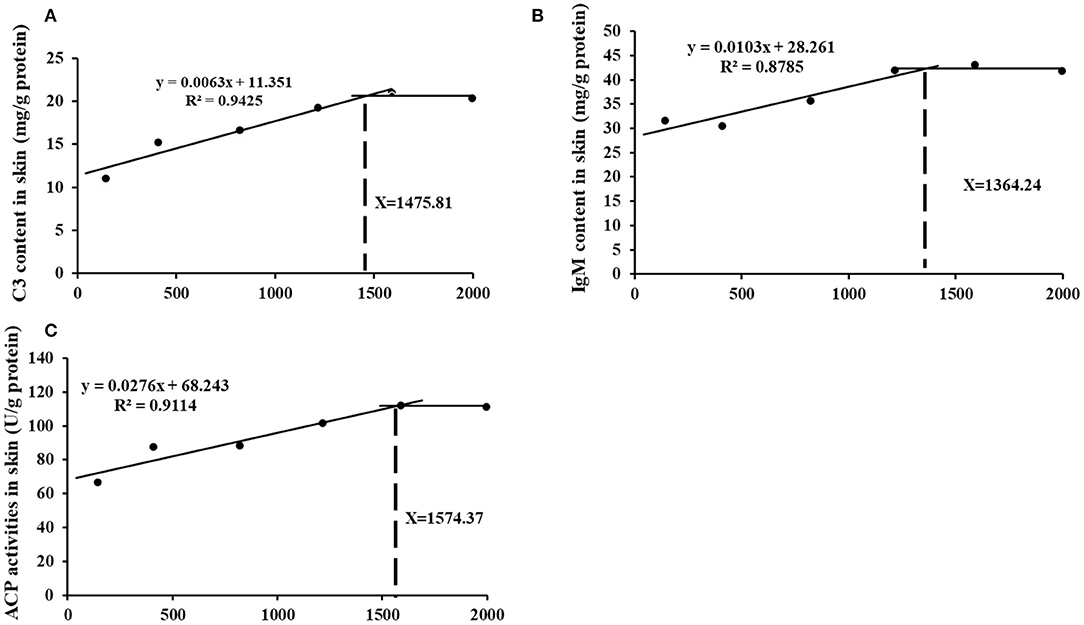
Figure 7. Broken-line analysis of C3 (A), IgM (B), and ACP content (C) in skin for grass carp (Ctenopharyngodon idella) fed diets containing graded levels of choline after infection with A. hydrophila.
Conclusions
In summary, we reveal four primary, innovative, and interesting results. The results exhibited that dietary choline (1) could increase the contents of choline and its metabolites as well as choline transporter expression in the skin; (2) reduced the skin lesion and increased the contents of C3, IgM, and C4 and the activities of ACP and LZ, advanced mucin2, LEAP-2B, hepcidin, and β-defensin mRNA abundance, upregulated the mRNA abundance of IL-1β, IFN-γ2, TNF-α, IL-6, IL-12P40, and IL-15 in skin of juvenile grass carp, and downregulated the mRNA abundance of TGF-β1, IL-4/13A, IL-4/13B IL-11, and IL-10 in grass carp skin showed that choline enhanced the immune function of the skin; and (3) downregulated the mRNA abundance of IKKγ, IKKβ, c-Rel, NF-κBp65, and NF-κBp52 as well as protein level of NF-κBp65 in the nucleus, upregulated the mRNA and protein level of IkBα, and advanced the mRNA abundance of STAT3b1, STAT3b2, JAK1, and JAK2 as well as p-STAT3 Tyr705 and p-STAT3 Ser727 protein level in grass carp skin, indicating that choline-protected immune function might relate to the JAK/STAT3 and NF-κB signaling pathway in fish skin. In conclusion, choline enhanced the skin immune function relevant to JAK 1, 2/STAT3, and NF-κB signaling molecules in fish. Furthermore, based on the skin immune indices (C3 and IgM contents as well as ACP activities), the choline requirements for grass carp (9.28–108.97 g) were estimated to be 1475.81, 1364.24, and 1574.37 mg/kg diet, respectively.
Data Availability Statement
The datasets presented in this study can be found in online repositories. The names of the repository/repositories and accession number(s) can be found in the article/supplementary material.
Ethics Statement
The animal study was reviewed and approved by Institutional Animal Care and Use Committee of Sichuan Agricultural University.
Author Contributions
Z-HY performed formal analysis, investigation, and writing—original draft. LF performed conceptualization, methodology, validation, data curation, and project administration. W-dJ performed data curation, validation, project administration, and writing—review & editing. PW performed conceptualization, funding acquisition, and resources. YL performed project administration. S-yK and LT performed resources. X-qZ performed conceptualization, methodology, supervision, funding acquisition, and supervision. All authors contributed to the article and approved the submitted version.
Funding
This research was financially supported by the National Natural Science Foundation of China (31772866), National Key R&D Program of China (2019YFD0900200, 2018YFD0900400), National Natural Science Foundation of China for Outstanding Youth Science Foundation (31922086), the Young Top-Notch Talent Support Program of National Ten-Thousand Talents Program, the Earmarked Fund for China Agriculture Research System (CARS-45), Outstanding Talents and Innovative Team of Agricultural Scientific Research (Ministry of Agriculture), Key Research and Development Plan in Sichuan Province (2018NZ0007), and Sichuan Science and Technology Program (2019YFN0036).
Conflict of Interest
The authors declare that the research was conducted in the absence of any commercial or financial relationships that could be construed as a potential conflict of interest.
Acknowledgments
The authors would like to express their sincere thanks to the personnel of these teams for their kind assistance.
References
1. Khosravi S, Jang J, Rahimnejad S, Song J, Lee K. Choline essentiality and its requirement in diets for juvenile parrot fish (Oplegnathus fasciatus). Asian Austr J Animal Sci. (2015) 28:647–53. doi: 10.5713/ajas.14.0532
2. Zhao HF, Jiang WD, Liu Y, Jiang J, Wu P, Kuang SY, et al. Dietary choline regulates antibacterial activity, inflammatory response and barrier function in the gills of grass carp (Ctenopharyngodon idella). Fish Shellfish Immunol. (2016) 52:139–50. doi: 10.1016/j.fsi.2016.03.029
3. Yuan ZH, Feng L, Jiang WD, Wu P, Liu Y, Jiang J, et al. Choline deficiency decreased the growth performances and damaged the amino acid absorption capacity in juvenile grass carp (Ctenopharyngodon idella). Aquaculture. (2020) 518:734829. doi: 10.1016/j.aquaculture.2019.734829
4. Evans DH, Piermarini PM, Choe KP. The multifunctional fish gill: dominant site of gas exchange, osmoregulation, acid-base regulation, and excretion of nitrogenous waste. Physiol Rev. (2005) 85:97–177. doi: 10.1152/physrev.00050.2003
5. Koppang EO, Kvellestad A, Fischer U. Fish mucosal immunity: gill. In: Mucosal Health in Aquaculture. Elsevier Inc (2015). p. 93–133. doi: 10.1016/B978-0-12-417186-2.00005-4
6. Wang XZ, Jiang WD, Feng L, Wu P, Liu Y, Zeng YY, et al. Low or excess levels of dietary cholesterol impaired immunity and aggravated inflammation response in young grass carp (Ctenopharyngodon idella). Fish Shellfish Immunol. (2018) 78:202–21. doi: 10.1016/j.fsi.2018.04.030
7. Pohlenz C, Gatlin DM. Interrelationships between fish nutrition and health. Aquaculture. (2014) 431:111–7. doi: 10.1016/j.aquaculture.2014.02.008
8. He G, Karin M. NF-kappaB and STAT3 - key players in liver inflammation and cancer. Cell Res. (2011) 21:159–68. doi: 10.1038/cr.2010.183
9. Murray PJ. Understanding and exploiting the endogenous interleukin-10/STAT3-mediated anti-inflammatory response. Curr Opin Pharmacol. (2006) 6:379–86. doi: 10.1016/j.coph.2006.01.010
10. Zheng L, Feng L, Jiang WD, Wu P, Tang L, Kuang SY, et al. Selenium deficiency impaired immune function of the immune organs in young grass carp (Ctenopharyngodon idella). Fish Shellfish Immunol. (2018) 77:53–70. doi: 10.1016/j.fsi.2018.08.038
11. Horie A, Ishida K, Watanabe Y, Shibata K, Hashimoto Y. Membrane transport mechanisms of choline in human intestinal epithelial LS180 cells. Biopharm Drug Dispos. (2014) 35:532–42. doi: 10.1002/bdd.1917
12. Ueland PM. Choline and betaine in health and disease. J Inherit Metab Dis. (2011) 34:3–15. doi: 10.1007/s10545-010-9088-4
13. Hasanzadeh-Moghadam M, Khadem-Ansari MH, Farjah GH, Rasmi Y. Hepatoprotective effects of betaine on liver damages followed by myocardial infarction. Vet Res Forum. (2018) 9:129–35. doi: 10.30466/vrf.2018.30834
14. Marrero MB, Bencherif M. Convergence of alpha 7 nicotinic acetylcholine receptor-activated pathways for anti-apoptosis and anti-inflammation: central role for JAK2 activation of STAT3 and NF-kappaB. Brain Res. (2009) 1256:1–7. doi: 10.1016/j.brainres.2008.11.053
15. Tabassum S, Haider S, Ahmad S, Madiha S, Parveen T. Chronic choline supplementation improves cognitive and motor performance via modulating oxidative and neurochemical status in rats. Pharmacol Biochem Behav. (2017) 159:90–9. doi: 10.1016/j.pbb.2017.05.011
16. Treede I, Braun A, Sparla R, Kuhnel M, Giese T, Turner JR, et al. Anti-inflammatory effects of phosphatidylcholine. J Biol Chem. (2007) 282:27155–64. doi: 10.1074/jbc.M704408200
17. Yan J, Jiang X, West AA, Perry CA, Malysheva OV, Brenna JT, et al. Pregnancy alters choline dynamics: results of a randomized trial using stable isotope methodology in pregnant and nonpregnant women. Am J Clin Nutr. (2013) 98:1459–67. doi: 10.3945/ajcn.113.066092
18. Jiang WD, Zhang L, Feng L, Wu P, Liu Y, Jiang J, et al. Inconsistently impairment of immune function and structural integrity of head kidney and spleen by vitamin A deficiency in grass carp (Ctenopharyngodon idella). Fish Shellfish Immunol. (2020) 99:243–56. doi: 10.1016/j.fsi.2020.02.019
19. Xu HJ, Jiang WD, Feng L, Liu Y, Wu P, Jiang J, et al. Dietary vitamin C deficiency depressed the gill physical barriers and immune barriers referring to Nrf2, apoptosis, MLCK, NF-kappaB and TOR signaling in grass carp (Ctenopharyngodon idella) under infection of Flavobacterium columnare. Fish Shellfish Immunol. (2016) 58:177–92. doi: 10.1016/j.fsi.2016.09.029
20. He P, Jiang WD, Liu XA, Feng L, Wu P, Liu Y, et al. Dietary biotin deficiency decreased growth performance and impaired the immune function of the head kidney, spleen and skin in on-growing grass carp (Ctenopharyngodon idella). Fish Shellfish Immunol. (2020) 97:216–34. doi: 10.1016/j.fsi.2019.12.033
21. Ni PJ, Jiang WD, Wu P, Liu Y, Kuang SY, Tang L, et al. Dietary low or excess levels of lipids reduced growth performance, and impaired immune function and structure of head kidney, spleen and skin in young grass carp (Ctenopharyngodon idella) under the infection of aeromonas hydrophila. Fish Shellfish Immunol. (2016) 55:28–47. doi: 10.1016/j.fsi.2016.03.163
22. Khan MA, Jafri AK, Chadha NK. Growth, reproductive performance, muscle and egg composition in grass carp, Ctenopharyngodon idella (Valenciennes), fed hydrilla or formulated diets with varying protein levels. Aquaculture Res. (2004) 35:1277–85. doi: 10.1111/j.1365-2109.2004.01150.x
23. Wu P, Feng L, Kuang, S.-Y., Liu Y, Jiang J, Hu, K., et al. Effect of dietary choline on growth, intestinal enzyme activities and relative expressions of target of rapamycin and eIF4E-binding protein2 gene in muscle, hepatopancreas and intestine of juvenile Jian carp (Cyprinus carpio var. Jian). Aquaculture. (2011) 317:107–16. doi: 10.1016/j.aquaculture.2011.03.042
24. Zeng YY, Jiang WD, Liu Y, Wu P, Zhao J, Jiang J, et al. Optimal dietary alpha-linolenic acid/linoleic acid ratio improved digestive and absorptive capacities and target of rapamycin gene expression of juvenile grass carp (Ctenopharyngodon idellus). Aquac Nutr. (2016) 22. doi: 10.1111/anu.12337
25. Ding Y, Mou S. Determination of choline chloride and trimethylamine in feedstuff by ion chromatography. Se Pu. (2004) 22:174.
26. Feng L, Xiao WW, Liu Y, Jiang J, Hu K, Jiang WD, et al. Methionine hydroxy analogue prevents oxidative damage and improves antioxidant status of intestine and hepatopancreas for juvenile Jian carp (Cyprinus carpio var. Jian). Aquaculture Nutri. (2011) 17:595–604. doi: 10.1111/j.1365-2095.2011.00853.x
27. Hong Y, Jiang W, Kuang S, Hu K, Tang L, Liu Y, et al. Growth, digestive and absorptive capacity and antioxidant status in intestine and hepatopancreas of sub-adult grass carp Ctenopharyngodon idella fed graded levels of dietary threonine. J Anim Sci Biotechnol. (2015) 6:34. doi: 10.1186/s40104-015-0032-1
28. Zhong JR, Feng L, Jiang WD, Wu P, Liu Y, Jiang J, et al. Phytic acid disrupted intestinal immune status and suppressed growth performance in on-growing grass carp (Ctenopharyngodon idella). Fish Shellfish Immunol. (2019) 92:536–551. doi: 10.1016/j.fsi.2019.06.045
29. Takemura A. Changes in an immunoglobulin M (IgM)-like protein during larval stages in tilapia, Oreochromis mossambicus. Aquaculture. (1993) 115:233–41. doi: 10.1016/0044-8486(93)90139-P
30. Huang C, Feng L, Jiang WD, Wu P, Liu Y, Zeng YY, et al. Deoxynivalenol decreased intestinal immune function related to NF-kappaB and TOR signalling in juvenile grass carp (Ctenopharyngodon idella). Fish Shellfish Immunol. (2019) 84:470–84. doi: 10.1016/j.fsi.2018.10.039
31. Yao J, Chen P, Apraku A, Zhang G, Huang Z, Hua X. Hydrolysable tannin supplementation alters digestibility and utilization of dietary protein, lipid, and carbohydrate in grass carp (Ctenopharyngodon idellus). Front Nutr. (2019) 6:183. doi: 10.3389/fnut.2019.00183
32. Zhang YL, Duan XD, Jiang WD, Feng L, Wu P, Liu Y, et al. Soybean glycinin decreased growth performance, impaired intestinal health, and amino acid absorption capacity of juvenile grass carp (Ctenopharyngodon idella). Fish Physiol Biochem. (2019) 45:1589–602. doi: 10.1007/s10695-019-00648-z
33. Zhang YL, Duan XD, Feng L, Jiang WD, Wu P, Liu Y, et al. Soybean glycinin impaired immune function and caused inflammation associated with PKC-zeta/NF-kappab and mTORC1 signaling in the intestine of juvenile grass carp (Ctenopharyngodon idella). Fish Shellfish Immunol. (2020) 106:393–403. doi: 10.1016/j.fsi.2020.08.008
34. Yuan Z, Feng L, Jiang W, Wu P, Liu Y, Kuang S, et al. Dietary choline deficiency aggravated the intestinal apoptosis in association with the MAPK signalling pathways of juvenile grass carp (Ctenopharyngodon idella). Aquaculture. (2021) 532:736046. doi: 10.1016/j.aquaculture.2020.736046
35. Renna M. Evaluation of the suitability of a partially defatted black soldier fly (Hermetia illucens L.) larvae meal as ingredient for rainbow trout (Oncorhynchus mykiss Walbaum) diets. J Anim Sci Biotechnol. (2017) 8:57. doi: 10.1186/s40104-017-0191-3
36. Ji Y, Luo X, Yang Y, Dai Z, Wu G, Wu Z. Endoplasmic reticulum stress-induced apoptosis in intestinal epithelial cells: a feed-back regulation by mechanistic target of rapamycin complex 1 (mTORC1). J Anim Sci Biotechnol. (2018) 9:38. doi: 10.1186/s40104-018-0253-1
37. Song P, Rekow SS, Singleton C, Sekhon HS, Dissen GA, Zhou M, et al. Choline transporter-like protein 4 (CTL4) links to non-neuronal acetylcholine synthesis. J Neurochem. (2013) 126:451–61. doi: 10.1111/jnc.12298
38. Pan FY, Feng L, Jiang WD, Jiang J, Wu P, Kuang SY, et al. Methionine hydroxy analogue enhanced fish immunity via modulation of NF-kappa B, TOR, MLCK, MAPKs and Nrf2 signaling in young grass carp (Ctenopharyngodon idella). Fish Shellfish Immunol. (2016) 56:208–28. doi: 10.1016/j.fsi.2016.07.020
39. Li M, Feng L, Jiang WD, Wu P, Liu Y, Jiang J, et al. Condensed tannins decreased the growth performance and impaired intestinal immune function in on-growing grass carp (Ctenopharyngodon idella). Br J Nutr. (2020) 123:737–55. doi: 10.1017/S0007114519003295
40. Liu HX, Zhou XQ, Jiang WD, Wu P, Liu Y, Zeng YY, et al. Optimal alpha-lipoic acid strengthen immunity of young grass carp (Ctenopharyngodon idella) by enhancing immune function of head kidney, spleen and skin. Fish Shellfish Immunol. (2018) 80:600–17. doi: 10.1016/j.fsi.2018.06.057
41. Giacomin PR, Moy RH, Noti M, Osborne LC, Siracusa MC, Alenghat T, et al. Epithelial-intrinsic IKKα expression regulates group 3 innate lymphoid cell responses and antibacterial immunity. J Experi Med. (2015) 212:1513–28. doi: 10.1084/jem.20141831
42. Monte MM, Zou J, Wang T, Carrington A, Secombes CJ. Cloning, expression analysis and bioactivity studies of rainbow trout (Oncorhynchus mykiss) interleukin-22. Cytokine. (2011) 55:62–73. doi: 10.1016/j.cyto.2011.03.015
43. Morimoto T, Biswas G, Kono T, Sakai M, Hikima J. Immune responses in the Japanese pufferfish (Takifugu rubripes) head kidney cells stimulated with particulate silica. Fish Shellfish Immunol. (2016) 49:84–90. doi: 10.1016/j.fsi.2015.12.023
44. Wang T, Husain M. The expanding repertoire of the IL-12 cytokine family in teleost fish: Identification of three paralogues each of the p35 and p40 genes in salmonids, and comparative analysis of their expression and modulation in Atlantic salmon Salmo salar. Dev Comp Immunol. (2014) 46:194–207. doi: 10.1016/j.dci.2014.04.008
45. Kolodziej L. Systemic metabolism of tryptophan and its catabolites, kynurenine and 3-HAA, in mice with inflammatory arthritis. Gene. (2013) 512:23–7. doi: 10.1016/j.gene.2012.09.122
46. Chandler TL, White HM. Choline and methionine differentially alter methyl carbon metabolism in bovine neonatal hepatocytes. PLoS ONE. (2017) 12:e171080. doi: 10.1371/journal.pone.0171080
47. Su YN, Wu P, Feng L, Jiang WD, Jiang J, Zhang YA, et al. The improved growth performance and enhanced immune function by DL-methionyl-DL-methionine are associated with NF-kappaB and TOR signalling in intestine of juvenile grass carp (Ctenopharyngodon idella). Fish Shellfish Immunol. (2018) 74:101–18. doi: 10.1016/j.fsi.2017.12.051
48. Sun H, Jiang WD, Wu P, Liu Y, Jiang J, Yang QH, et al. Betaine supplementations enhance the intestinal immunity of on-growing grass carp (Ctenopharyngodon idella): partly related to TOR and NF-κB signaling pathways. Aquaculture. (2020) 518:734846. doi: 10.1016/j.aquaculture.2019.734846
49. Shih VF, Tsui R, Caldwell A, Hoffmann A. A single NFkappaB system for both canonical and non-canonical signaling. Cell Res. (2011) 21:86–102. doi: 10.1038/cr.2010.161
50. Vallabhapurapu S, Karin M. Regulation and function of NF-κB transcription factors in the immune system. Annu Rev Immunol. (2009) 27:693–733. doi: 10.1146/annurev.immunol.021908.132641
51. Won S, Lee S, Hamidoghli A, Lee S, Bai SC. Dietary choline requirement of juvenile olive flounder (Paralichthys olivaceus). Aquaculture Nutri. (2019) 25:1281–8. doi: 10.1111/anu.12948
52. De Jonge WJ, Der Zanden EPV, Bijlsma MF, Van Westerloo DJ, Bennink RJ, Berthoud H, et al. Stimulation of the vagus nerve attenuates macrophage activation by activating the Jak2-STAT3 signaling pathway. Nat Immunol. (2005) 6:844–51. doi: 10.1038/ni1229
53. Melendez AJ, Harnett MM, Allen JM. Differentiation-dependent switch in protein kinase C isoenzyme activation by FccRI, the human high-affinity receptor for immunoglobulin G. Immunology. (1999) 6:457–64. doi: 10.1046/j.1365-2567.1999.00689.x
54. Peng Y, Sigua CA, Gallagher SF, Murr MM. Protein kinase C-zeta is critical in pancreatitis-induced apoptosis of Kupffer cells. J Gastrointest Surg. (2007) 11:1253–61. doi: 10.1007/s11605-007-0193-0
55. Arup Chakraborty SMW, Schaefer TS, Ball ED, Dyer KF, Tweardy DJ. Granulocyte colony-stimulating factor activation of Stat3a and Stat3P in immature normal and leukemic human myeloid cells. Rapid Commun. (1996) 88:2442–9. doi: 10.1182/blood.V88.7.2442.bloodjournal8872442
Keywords: juvenile grass carp (Ctenopharyngodon idella), immune function, choline, skin, JAK/STAT3, NF-κB signaling
Citation: Yuan Z-H, Feng L, Jiang W-d, Wu P, Liu Y, Jiang J, Kuang S-y, Tang L and Zhou X-q (2021) Dietary Choline-Enhanced Skin Immune Response of Juvenile Grass Carp Might Be Related to the STAT3 and NF-kB Signaling Pathway (Ctenopharyngodon idella). Front. Nutr. 8:652767. doi: 10.3389/fnut.2021.652767
Received: 13 January 2021; Accepted: 29 March 2021;
Published: 20 May 2021.
Edited by:
Reinaldo B. Oria, Federal University of Ceara, BrazilCopyright © 2021 Yuan, Feng, Jiang, Wu, Liu, Jiang, Kuang, Tang and Zhou. This is an open-access article distributed under the terms of the Creative Commons Attribution License (CC BY). The use, distribution or reproduction in other forums is permitted, provided the original author(s) and the copyright owner(s) are credited and that the original publication in this journal is cited, in accordance with accepted academic practice. No use, distribution or reproduction is permitted which does not comply with these terms.
*Correspondence: Xiao-qiu Zhou, xqzhouqq@tom.com; zhouxq@sicau.edu.cn