Imaging the neural correlates of tinnitus: a comparison between animal models and human studies
- 1Department of Otolaryngology, University of Pittsburgh, Pittsburgh, PA, USA
- 2Department of Neurobiology, University of Pittsburgh, Pittsburgh, PA, USA
- 3Center for the Neural Basis of Cognition, University of Pittsburgh, Pittsburgh, PA, USA
Tinnitus is the perception of a sound, a so-called “phantom sound,” in the absence of a physical sound. The phantom perception persists after transection of the auditory nerve, indicating that the site of tinnitus manifestation is in the central nervous system. Imaging studies in tinnitus sufferers have revealed increased neuronal activity—hyperactivity—in subcortical and cortical auditory centers. These studies have demonstrated that non-auditory brain areas, such as the limbic system, are involved in the neural basis of tinnitus, Finally human imaging studies have led to novel hypotheses for the generation of tinnitus, such as the thalamocortical dysrhythmia hypothesis. Imaging in animal models of tinnitus exhibit similarities to results from human studies and have revealed hyperexcitability of auditory brain centers as a neural correlate of tinnitus. We propose that the comparison between animal model and human studies will aid in the design of appropriate experimental paradigms aimed at elucidating the cellular and circuit mechanisms underlying tinnitus.
Introduction
Tinnitus sufferers perceive sounds in the absence of any physical auditory stimulus. Tinnitus can be caused by head and neck injuries (Chan and Reade, 1994), chemotherapy (Bokemeyer et al., 1998), ototoxic drugs such as salicylate (Stypulkowski, 1990) but, most frequently, tinnitus is caused by intense sound exposure (Passchier-Vermeer and Passchier, 2000). The various causes of tinnitus generate a diverse range of tinnitus percepts (Eggermont and Roberts, 2004). Some patients with chronic tinnitus are constantly aware of the phantom perception, but cope very effectively with this disturbance. For some patients, however, tinnitus is more than just a minor annoyance—these patients report that tinnitus causes extreme feelings of desperation and in some cases results in suicidal thoughts (Dobie, 2003). Despite the increasing numbers of tinnitus sufferers—due to increasing risks from occupational and recreational sources as well as the increased awareness of the disease—a basic understanding of the neural basis of tinnitus is lacking.
Mechanistically, tinnitus had been considered for many years as a peripheral disorder; a disorder of the external ear. This view has changed as sectioning of the eighth cranial nerve was an ineffective surgical treatment of tinnitus (House and Brackmann, 1981; Barrs and Brackmann, 1984) and collateral sectioning of the auditory nerve with tumor removal surgery even caused tinnitus in some patients (Berliner et al., 1992; Baguley et al., 2006). These results indicate that it is the auditory central nervous system—and not the periphery—that maintains the percept of tinnitus.
Recently developed animal models of noise-induced tinnitus have provided a unique opportunity for determining the neural mechanisms underlying the induction and the expression mechanisms of tinnitus (Eggermont and Roberts, 2004). These studies have uncovered many forms of aberrant plasticity that result in marked changes in the cellular and molecular properties of the auditory system of animals with tinnitus compared to healthy animals. An emerging hypothesis for the cellular mechanisms underlying tinnitus involves reduced activity of the auditory nerve—as a result of the noise-exposure or other injury (Liberman and Kiang, 1978; Mulheran, 1999; Muller et al., 2003)—and subsequent development of hyperactivity of neurons in central auditory brain centers. This hyperactivity is thought to arise from a central down regulation of inhibition to compensate for reduced peripheral afferent drive (Suneja et al., 1998a,b; Wang et al., 2009; Middleton et al., 2011). An alternative hypothesis suggests the up-regulation of excitatory inputs as a contributor to tinnitus-related hyperactivity (Dehmel et al., 2012). Tinnitus-related hyperexcitability has been observed in several auditory centers throughout the ascending auditory pathway. (Jastreboff and Sasaki, 1986; Chen and Jastreboff, 1995; Ochi and Eggermont, 1997; Eggermont and Komiya, 2000; Kaltenbach and Afman, 2000; Kaltenbach et al., 2000; Brozoski et al., 2002; Chang et al., 2002; Norena and Eggermont, 2003). While the establishment of hyperactivity neural activity as a correlate of tinnitus has advanced the understanding of the mechanistic basis for tinnitus, the features of neural activity that specifically underlie the perception of tinnitus remain elusive. One hypothesis proposes that aberrant thalamocortical rhythms—thalamocortical dysrhythmia—emerge as a plastic maladaptation to peripheral injury (Llinas et al., 2005). These rhythms may act to promote coherent high-frequency (gamma) oscillations and thus generate the conscious perception of tinnitus.
Imaging studies in humans have sought to pinpoint the neural correlates of tinnitus, however, they do not allow for the dissection of the cellular mechanisms underlying tinnitus. On the other hand, imaging studies in animal models of tinnitus offer a reduced, better-controlled experimental environment that will facilitate the discovery of the underlying physiological remodeling that leads to tinnitus. Here we will review and compare studies that have employed different imaging modalities to examine the neural correlates of tinnitus in animal models and in humans. We will also discuss how imaging in animal models may help address specific hypotheses about the persistent basis of tinnitus in the central nervous system. Further experimental and conceptual linking between human studies and studies in animal models of tinnitus will facilitate the discovery of the etiology and the cure of tinnitus.
Imaging Techniques
Traditional methods of recording electrophysiological brain activity present significant problems that prohibit their usage in human studies. The most important issue being that extracellular electrode recordings require craniotomies, which are untenable for non-critical patients. Imaging techniques offer an alternative means of accessing physiological parameters in a non-invasive manner. Imaging of human patients has gained widespread usage in both clinical and research settings. In particular, positron emission tomography (PET) and functional magnetic resonance imaging (fMRI) are now commonly used to study the physiological basis of tinnitus in human sufferers.
Neural imaging involves monitoring changes in the level of cerebral blood flow or glucose metabolism in areas of the central nervous system. Subjects in PET studies are injected with radioactive tracer isotopes attached to a biologically active compound. These compounds are introduced to the bloodstream and, depending on the biological compound tethered to the isotope, can be used for detecting changes in blood flow, oxygen metabolism or glucose metabolism as a result of brain activity (Fox et al., 1986). fMRI can be used to image the oxygenation state of cerebral blood, and thus provides an indirect measure of neural activity. Hemoglobin in the oxygenated state is more susceptible to the brief magnetic pulses produced by fMRI scanners, thus enabling this technique to specifically measure the contrast of blood oxygenation level dependence (BOLD) (Ogawa et al., 1990). fMRI typically has a finer spatial resolution and faster temporal resolution than PET imaging. However, since the BOLD signal also arises from non-neural sources, baseline neural activity cannot be inferred using fMRI. Instead, relative changes in the BOLD signal must be used as a secondary indicator of changes in neural activity. Both imaging methods described, PET and fMRI, have limitations compared to traditional recording techniques: (1) they indicate blood flow or metabolic state and thus are only indirectly linked to neural activity, (2) their temporal resolution is slower than recording techniques that directly measure neural activity (i.e., electrical recordings, voltage, or calcium-sensitive dyes), and (3) their spatial resolution is limited to the scale of millimeters. This last point is the factor that most limits the usage of these techniques in animal studies of tinnitus. In particular, it becomes difficult to resolve signals from auditory brainstem nuclei in rodent models of tinnitus since their volume is typically smaller than a cubic millimeter.
Other non-invasive recording techniques, such as electroencephalograms (EEGs) and magentoencephalograms (MEGs), provide alternates to PET and fMRI imaging. The electric fields measured by EEGs are the result of extracellular currents that are oriented perpendicular to the cortical surface from synchronously firing neurons (Nunez and Srinivasan, 1981). MEGs likely arise from the synaptic currents that are generated by apical dendrites across synchronously activated neurons (Hillebrand and Barnes, 2002). The skull and scalp attenuate EEGs, but are transparent to magnetic fields, thus MEGs offer better spatial resolution than EEGs. The spatial resolution of MEGs is only limited by the number of neurons that must be synchronously active to generate sufficiently strong signals for detection.
Human Imaging Studies
In this section, we will briefly review several imaging studies that show patterns of activation that are consistent with physiological studies of animal models and some results that point to the involvement of non-auditory brain centers in tinnitus. PET imaging has revealed increased regional cerebral blood flow (rCBF) in the contralateral auditory cortex of patients with tinnitus in one ear (Lockwood et al., 2002). Some patients have the ability to modulate the experience of perceived tinnitus with orofacial manipulations (Lockwood et al., 1998). In the cases where manipulations decreased tinnitus perception, there was a significant reduction of rCBF in the auditory cortex and also in the contralateral hippocampus. This further supports the hypothesis that the basis of tinnitus is centrally located and that internal perceptions can be modulated by the top-down influence of voluntary orofacial movements.
Measuring changes in hemodynamics in tinnitus patients with fMRI has revealed increased activation of inferior colliculus (IC) to sound stimuli compared to control subjects (Figure 1) (Melcher et al., 2009). This study is consistent with animal studies showing increased activation of IC in different animal models of tinnitus (Bauer et al., 2008) (see next section). Another fMRI study shows that auditory cortex hyperexcitability correlates with tinnitus while hyperexcitability of subcortical brainstem regions is more directly linked with the occurrence of hyperacusis, or diminished sound level tolerance (Gu et al., 2010).
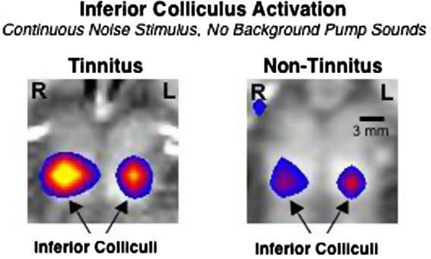
Figure 1. An fMRI imaging study reveals larger sound-evoked activation of the inferior colliculus in patients with tinnitus (left) compared to control subjects (right). The color scale indicates the significance of the difference of activation between on and off stimulus periods. Figure adapted from Melcher et al. (2009).
One recent discovery emerging from human imaging studies is the involvement of the limbic system in tinnitus. Tinnitus-related functional and structural changes are observed in the hippocampus and in the amygdala, (Lockwood et al., 1998; De Ridder et al., 2006; Landgrebe et al., 2009). More recently, strong correlation was shown between structural and functional changes in the auditory system and limbic system in tinnitus patients (Leaver et al., 2011). Specifically, fMRI revealed hyperactivity in the nucleus accumbens (NAc) and primary auditory cortex when tinnitus patients were presented acoustic stimuli matched to their perceived tinnitus frequency (Figure 2A). No functional changes were observed in the prefrontal cortex, however, structural changes were uncovered using voxel-based morphometry (VBM) on high resolution structural MRI. The ventromedial prefrontal cortex (vmPFC) of tinnitus patients had increased gray matter and decreased white matter concentrations. The degree of structural changes in vmPFC was significantly correlated with functional changes in NAc and auditory cortex (Figure 2B). Because the limbic system is involved in modulating and maintaining emotional state it has previously been hypothesized to play a role in the neural basis of tinnitus (Rauschecker et al., 2010). However, it remains unclear whether the limbic dysfunction is involved in the establishment of tinnitus or whether it is a secondary consequence of the emotional distress tinnitus caused by tinnitus. Furthermore, the recent finding of correlated structural and functional changes in the limbic and auditory systems suggest a widespread alteration in the limbic corticostriatal pathway, which is thought to assess stimulus relevance and filter out undesirable stimuli (Leaver et al., 2011). The compromise of the limbic system may play a role in gating the persistent representation of phantom stimuli in the auditory cortex.
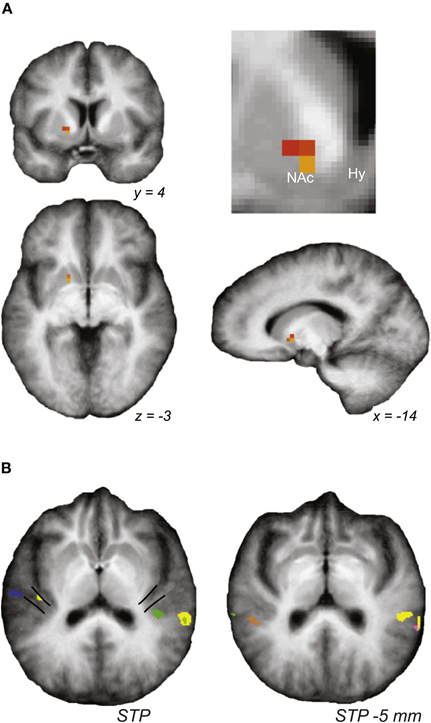
Figure 2. Auditory and limbic brain centers exhibit sound-evoked hyperactivity. Stimuli matched for perceived tinnitus frequency evoked significantly higher activity, as measured by fMRI, in (A) the nucleus accumbens (NAc) and (B) medial Hershel's gyrus (mHG), the presumed site of primary auditory cortex. Figure adapted from Leaver et al. (2011).
BOLD signals reveal auditory stimulus-induced activity relative to baseline. It is not clear whether the perception of tinnitus is linked to stimulus-induced activity or properties of spontaneous neural activity alone, however, there is evidence that auditory stimulus related changes in the cortical BOLD signal are specifically correlated with the presence of tinnitus (Gu et al., 2010). While the intrinsic, synaptic and circuit mechanisms underlying tinnitus-related hyperactivity or tinnitus-related enhanced evoked activity are not well understood (Tzounopoulos, 2008), it is likely that similar mechanisms may mediate both spontaneous and stimulus-evoked changes.
MEG recordings from tinnitus sufferers have also contributed important insights into the neurobiology of tinnitus. MEG recordings from human sufferers of tinnitus demonstrated a redistribution of the tonotopic axis in A1 in human tinnitus patients (Muhlnickel et al., 1998). MEG signals for the presumed tinnitus frequency occupied more cortical space at the expense of other frequencies. Animal models of noise-induced tinnitus corroborate these findings as they demonstrate tonotopic reorganization (Norena and Eggermont, 2005, 2006). This reorganization can be prevented by enriched acoustic environments (Norena and Eggermont, 2005, 2006) and even reversed by pairing vagus nerve stimulation with pure tone stimulation (Engineer et al., 2011). Despite the evidence that tonotopic reorganization can result from noise-induced tinnitus, human fMRI studies suggest that tonotopic reorganization may not be necessary for the conscious perception of tinnitus (Langers et al., 2012). The interpretation that tinnitus does not require tonotopic reorganization is further supported by the possibility that increased MEG signals may also reflect changes in neural synchrony.
An alternative hypothesis for the neural basis of tinnitus proposes that reduced thalamic input—due to sound-exposure driven deafferentation—leads to thalamocortical dysrhythmia (Llinas et al., 1999). MEG recordings of spontaneous neural activity from tinnitus patient's exhibit pronounced cortical activity peaks in the theta range (4–8 Hz; Figure 3). Rhythms in this range were reduced when the patients were presented masking auditory stimuli (Llinas et al., 2005). Tinnitus patients also exhibit MEG activity that has broad-spectrum gamma rhythms with elevated power compared to MEGs from control subjects. Cortical gamma rhythms are hypothesized to bind together activity of neural populations to form the substrate of conscious perception (Fries et al., 2007). A potential causal link between aberrant theta rhythms and aberrant gamma rhythms has been suggested by in vitro experiments (Llinas et al., 2005). It was shown that 40 Hz (gamma) stimulation of cortical tissue evokes focal responses while stimulation with 4 Hz (theta) evoked more widespread responses. Furthermore, mixing of theta and gamma-evoked responses promotes the spread of gamma oscillatory activity into neighboring cortical regions. This so-called edge effect provides an attractive mechanistic explanation for the basis of certain forms of tinnitus where patients exhibit abnormally high theta rhythms in MEG signals.
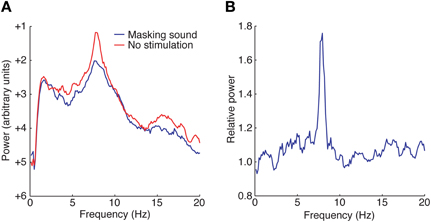
Figure 3. Magnetoencephalograms (MEGs) reveal abnormal rhythmicity in the auditory cortex of tinnitus patients. (A) MEGs of spontaneous neural activity from tinnitus patients show a distinct peak in the theta range (red) that is suppressed when a masking sound is presented (blue). (B) The relative power of these two conditions reveals a strong coherent theta rhythm under spontaneous conditions. Figure adapted from Llinas et al. (2005).
Gamma oscillations have been linked with behaviorally relevant perceptual tasks. In humans and monkeys, the latency of detection of visual stimulus changes is reduced when gamma power in visual cortex is increased (Womelsdorf et al., 2006; Hoogenboom et al., 2010). Correlated activity between different brain regions that is associated with perception can arise through rhythmic gamma activity (Womelsdorf et al., 2007). Thus, noise-induced intracortical gamma-mediated signaling, arising from thalamocortical dysrhythmia, could contribute to the perception of tinnitus.
Tinnitus generation via increases of gamma-band activity at the edge frequency may not be relevant for all forms of tinnitus. The edge effect that we described above may arise from the functional lesion of a very small range of frequencies; such a lesion may lead to increased gamma activity and to subsequent tinnitus perception at the predicted “edge area.” However, functional damage of a broader range of cochlear frequencies is expected to lead to a more global cortical reorganization, thus leading to tinnitus that involves perception of broader spectrum of frequencies.
The underlying cellular and molecular causes of aberrant thalamocortical dysrhythmia are unclear, but the relevance of this model is supported by a clinical study showing that a group of patients with therapy-resistant tinnitus exhibited theta frequency bursting of neurons in the medial thalamus (Jeanmonod et al., 1996). This type of bursting pattern is associated with the de-inactivation of low-threshold calcium currents (Llinas and Jahnsen, 1982). Surgical removal of the medial thalamus has suggested a reduced perception of phantom noises associated with tinnitus (Jeanmonod et al., 1996). Despite the appeal of the dysrhythmia hypothesis the mechanism underlying the initiation of pathological bursting in the thalamus remains poorly understood.
Animal Imaging Studies: Forming a Link Between Animal Model Physiology and Human Imaging Studies
Electrophysiological studies in animal models of tinnitus have contributed significantly to the understanding of the neural basis of tinnitus. These studies have uncovered physiological signatures of tinnitus in different processing centers of the auditory system. In the dorsal cochlear nucleus (DCN) elevated firing rates are observed after exposure to intense sound (Kaltenbach et al., 1998; Brozoski et al., 2002; Chang et al., 2002). In the IC increased firing rates are observed after application of large doses of salicylate (Jastreboff and Sasaki, 1986; Chen and Jastreboff, 1995). Salicylate also results in long lasting increases in firing rates of neurons in cat auditory cortex (Zhang et al., 2011). Noise trauma results in hyperactivity at the auditory cortex (Eggermont and Komiya, 2000; Seki and Eggermont, 2003). The trauma induces increases in both spontaneous firing rates and in peak neuronal cross-correlations (Norena and Eggermont, 2003, 2006). How the neural correlates of tinnitus observed in animal studies relate to the neural correlates of tinnitus revealed by imaging brain activity in human sufferers is not entirely clear. Recent studies that involve imaging techniques applied to animal models of tinnitus have begun to provide these missing connections.
Relatively few animal studies have imaged the neural correlates of tinnitus in vivo. One notable study employed the use of micro positron emission tomography (μPET) to image baseline spontaneous activity in rat auditory nuclei (Paul et al., 2009). Rats were injected with a radioactive fluorescent tracer fluorine-18 fluoro-2-deoxyglucose (FDG) and serial reconstructions of relative metabolic activity were made from measurements using a PET scanner. This study showed that metabolic activity signals were higher in IC and auditory cortex, but not significantly increased in the thalamus of rats with salycilate-induced tinnitus. An important aspect of this study is the reproducibility of response magnitudes in successive imaging sessions of control subjects. This allows for the measurement of an absolute baseline signal enabling the assessment of both spontaneous and evoked responses. Altogether, this paper—in agreement with electrophysiological studies—demonstrates that in vivo imaging techniques reveal hyperactivity as a neural correlate of tinnitus.
Plastic maladaptive changes in inhibitory neurotransmission in different auditory nuclei are thought to play a role in establishing the neural basis of tinnitus. GABAergic and glycinergic release are altered in auditory brainstem nuclei after cochlear ablation (Suneja et al., 1998b; Wang et al., 2009); GABAergic activity is altered in the IC in a salicylate model of tinnitus (Bauer et al., 2000). Importantly, pharmaceutical treatments that enhance the action of GABAergic systems restore the physiological function of the auditory system in animals with behavioral evidence of tinnitus (Bauer and Brozoski, 2001; Brozoski et al., 2007).
A recent imaging study revealed the role of different excitatory and inhibitory neurotransmitter systems in mediating the observed tinnitus-related hyperactivity. In this study a novel imaging paradigm has been applied; flavoprotein autofluorescence (FA) imaging (Middleton et al., 2011). FA imaging was used to monitor stimulus-evoked activity in DCN brain slices. Flavoproteins are mitochondrial proteins that become oxidized and reduced in the electron transport chain of cellular metabolism (Shibuki et al., 2003; Reinert et al., 2007). In the oxidized state, flavoproteins absorb blue light (∼480 nm) and emit green light (∼540 nm). Using FA imaging in DCN slices, electrical stimulation of the molecular cell layer of the DCN (Figure 4A) reveals that the spread of the FA signal is proximal to the stimulation site in control mice (Figure 4B1). Similar stimulation in mice with behavioral evidence of tinnitus revealed FA signals that extend over a much broader region of the DCN (Figure 4B2). Analysis of the center (stimulation site) and surround regions of the DCN slice show that the surround/center ratio is significantly larger in the DCN from tinnitus mice (Figure 4C). To determine which neurotransmitter system mediates the increased FA signal in tinnitus mice, the impact of a series of neurotransmitter receptor antagonists on FA signals was assessed (Figures 4D,E). Application of SR-95531 (GABAergic inhibition antagonist) caused a larger increase in the surround signal of control slices in tinnitus slices (Figure 4E). Successive application of blockers of excitation caused similar reductions in the relative FA response in control and tinnitus DCN slices. These results indicate that GABAergic inhibition plays a role in maintaining focal responses in normally functioning DCN. Moreover these results indicate that decreased GABAergic inhibition leads to hyperactive DCN circuits in mice with behavioral evidence of tinnitus. The impairment of GABAergic inhibition provides a mechanism for the neural basis of tinnitus-related hyperexcitability in the DCN in addition to the known role of impaired glycinergic inhibition after cochlear injury (Suneja et al., 1998a,b; Wang et al., 2009), Together, these studies demonstrate the applicability of imaging techniques—that are analogous to human imaging studies—to study the underlying mechanisms of tinnitus neural correlates.
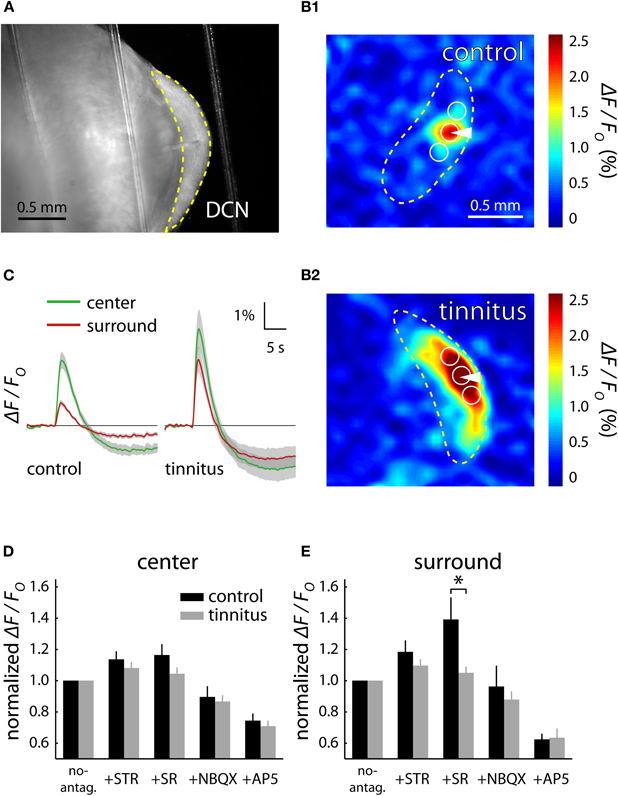
Figure 4. Flavoprotein autofluorescent (FA) imaging reveals GABAergic related hyperactivity in the dorsal cochlear nucleus (DCN) of mice with behavioral evidence of tinnitus. Electrical stimulation of the DCN brain slices (A) leads to a spatially concise excitatory response in control animals (B1) and a spatially extended response in tinnitus animals (B2). The center region is defined as the area at the stimulation electrode while the surround regions flank the center on either side along the fusiform cell layer. The size of surround responses relative to the stimulation center response is significantly larger in tinnitus brain slices (C). Pharmacological blockade of inhibitory and excitatory neurotransmitters has similar effects on the center signal of control and tinnitus mice (D) while the GABAergic antagonist SR causes a larger relative increase in the surround of control brain slices (E). Figure adapted from Middleton et al. (2011).
Discussion
The growing prevalence of tinnitus has made it imperative to better understand the mechanistic development of the neurological basis of tinnitus. Animal studies have contributed greatly to this understanding. However, the connection between the underlying physiology in animal models and the functional imaging patterns in human studies remains unclear.
The imaging methods discussed have their own respective advantages and disadvantages. The methods directly related to cellular metabolism, and thus indirectly related to neural activity (PET and fMRI) are somewhat limited in their ability to measure single cell activity and specific microcircuit activation. Metabolic signals may be used to develop models describing the dynamics of neural populations and how they change with tinnitus-related plasticity. However, any such model would be under constrained as there are multiple cell-types contributing to the metabolic signal, and many different combinations of subpopulation activity could lead to the same observable signal.
In order to understand how cellular and circuit based plasticity underlies altered BOLD responses in tinnitus patients one must be able to assess cellular and circuit properties underlying the bold response. A better understanding of the mechanistic link between circuit-based plasticity and the altered BOLD responses in tinnitus patients may be furthered through combined imaging and physiological studies in animal models of tinnitus. One technique that may allow for this powerful combination involves the use c-fos/green fluorescent protein (GFP) constructs to identify subsets of neurons that have been activated by sensory stimulation (Barth et al., 2004). C-fos is an early immediate gene whose expression is dependent on neural activity (Gall et al., 1998). When used in animal models, it is an ideal marker of neural populations that have been recently active in in vivo contexts. In a previous immunohistochemical study, levels of c-fos were visualized several hours after animals were exposed to loud narrowband noise or given salycilate injections (Mahlke and Wallhausser-Franke, 2004). In this study, immunoreactive neurons were found in the auditory cortex as well as the amygdala. A previous study showed differences in c-fos activation in the DCN: c-fos expression was increased with noise exposure but not salycilate injections (Wallhausser-Franke et al., 2003). Both of these findings have interesting implications for the different modes of tinnitus induction.
In order to understand the circuit-based mechanisms underlying the BOLD signal or other in vivo imaging signals, imaging experiments could be performed on c-fos/EGFP mice that have been given tinnitus-inducing manipulations. These mice could be taken immediately after imaging sessions so that brain slices may be prepared for in vitro recording experiments. What this technique will potentially reveal is the identities of the neurons that previously contributed to increased metabolic signals from in vivo imaging experiments. These fluorescent neurons may then be targeted for the characterization of changes in intrinsic physiological characteristics allowing for an increased understanding of the cellular and network basis for altered functional imaging signals in sufferers of tinnitus.
One interesting finding from imaging studies in tinnitus sufferers is the involvement of the limbic system in the neural basis of tinnitus (Lockwood et al., 1998; Mahlke and Wallhausser-Franke, 2004; Leaver et al., 2011). These studies revealed hyperactivity in the hippocampus (Lockwood et al., 1998), amygdala (Mahlke and Wallhausser-Franke, 2004) and prefrontal cortex (Leaver et al., 2011). These findings point to the need for increased focus on the cellular and circuit properties of neurons in affected limbic areas in animal models of tinnitus. The role of the structural changes in prefrontal cortex and/or other limbic structures could be assessed by stimulating these regions while performing concurrent imaging or electrophysiological recordings of auditory cortical regions in intact animal models of tinnitus.
Human MEG studies support the idea that a low frequency thalamocortical dysrhythmia may form the neurological substrate for long lasting tinnitus in the cortex. This theory hinges on the assumption that thalamic relay neurons are excessively hyperpolarized as a result of partial de-afferentation. Local hyperpolarization disinhibits T-type, burst-promoting calcium channels. The aberrant opening of these channels at the frequency areas where de-afferentation occurs would promote increased cortical gamma band activity in nearby frequency bands: abnormal silence in one area promotes sound generation in a neighboring area (edge effect, Llinas et al., 2005). This is an attractive model for explaining tonal tinnitus. In vitro imaging and electrophysiological studies, especially in T-type channel knockout mice will be essential in proving or disproving this hypothesized tinnitus generation mechanism.
While frequency-specific deafferentation at the level of the thalamus would account for burst promoting activation of T-type calcium channels, much evidence from animal studies points to hyperactivity in subthalamic auditory centers (Eggermont and Roberts, 2004). We propose an alternative hypothesis that involves the reciprocally connected excitatory-inhibitory loop between thalamus and the thalamic reticular nucleus (TRN). Sensory nuclei in the thalamus send excitatory collaterals to neurons in TRN, which in turn project inhibitory connections back to the thalamus (Crabtree, 1999). If subthalamic hyperactivity acts to strengthen the feedforward excitatory pathway (MGN → TRN) during early stages of tinnitus, the feedback inhibitory pathway (TRN → MGN) may be upregulated to maintain physiological levels of corticothalamic activity. If subthalamic hyperactivity subsides during later stages of tinnitus, the residual inhibition from TRN may persist thus providing a physiological mechanism for hyperpolarization-activated theta bursting in thalamus. This putative maladaptive TRN inhibition would thus promote thalamocortical dysrhythmia. Longitudinal animal imaging studies are necessary to test this hypothesis to verify whether there are time-dependent changes in hyperexcitability of subthalamic auditory nuclei. Additionally, joint recordings of neurons from MGN and TRN would help support or refute this hypothesis.
Anatomical studies have demonstrated top-down connections from layers 5 and 6 of auditory cortex to the IC, the olivary complex and the cochlear nucleus (Doucet et al., 2003). Activation of top-down pathways in a specific frequency region of auditory cortex modulates the response properties and the number of brainstem neurons preferentially responding to similar frequencies (Yan and Ehret, 2002; Yan et al., 2005; Luo et al., 2008). Therefore, noised-induced modification of top-down modulatory inputs could also play a role in the induction of tinnitus. This hypothesis could be addressed by ablating corticofugal neurons (Bajo et al., 2010) or electrically stimulating them in rodent models of tinnitus while measuring the resulting changes in brainstem neuron receptive field properties.
Another alternative theory, consistent with the discovery of limbic involvement is that NAc activity, which is elevated in tinnitus patients (Leaver et al., 2011), drives the TRN via serotonergic synapses (O'Donnell et al., 1997), and would thus indirectly increase the inhibition to MGN. The resulting increased inhibitory drive that TRN provides to auditory thalamus would provide the necessary hyperpolarization to activate T-type calcium currents and consequently promote aberrant thalamocortical rhythms as described above.
Ultimately, we believe that imaging studies, both in tinnitus sufferers and in animal models of tinnitus, are invaluable for their ability to connect non-invasively measured neural correlates and compare those to similarly measured quantities in animal models. Together with behavioral diagnostic techniques and in vitro intrinsic and network characterization of neurons that contributed to increased metabolic activity in vivo, imaging studies will increase the understanding of induction and maintenance of tinnitus and aid in the development of therapeutic strategies to improve hearing deficits for human sufferers of tinnitus.
Conflict of Interest Statement
The authors declare that the research was conducted in the absence of any commercial or financial relationships that could be construed as a potential conflict of interest.
References
Baguley, D. M., Phillips, J., Humphriss, R. L., Jones, S., Axon, P. R., and Moffat, D. A. (2006). The prevalence and onset of gaze modulation of tinnitus and increased sensitivity to noise after translabyrinthine vestibular schwannoma excision. Otol. Neurotol. 27, 220–224.
Bajo, V. M., Nodal, F. R., Moore, D. R., and King, A. J. (2010). The descending corticocollicular pathway mediates learning-induced auditory plasticity. Nat. Neurosci. 13, 253–260.
Barrs, D., and Brackmann, D. (1984). Translabyrinthine nerve section: effect on tinnitus. J. Laryngol. Otol. Suppl. 9, 287–293.
Barth, A. L., Gerkin, R. C., and Dean, K. L. (2004). Alteration of neuronal firing properties after in vivo experience in a FosGFP transgenic mouse. J. Neurosci. 24, 6466–6475.
Bauer, C. A., and Brozoski, T. J. (2001). Assessing tinnitus and prospective tinnitus therapeutics using a psychophysical animal model. J. Assoc. Res. Otolaryngol. 2, 54–64.
Bauer, C. A., Brozoski, T. J., Holder, T. M., and Caspary, D. M. (2000). Effects of chronic salicylate on GABAergic activity in rat inferior colliculus. Hear. Res. 147, 175–182.
Bauer, C. A., Turner, J. G., Caspary, D. M., Myers, K. S., and Brozoski, T. J. (2008). Tinnitus and inferior colliculus activity in chinchillas related to three distinct patterns of cochlear trauma. J. Neurosci. Res. 86, 2564–2578.
Berliner, K. I., Shelton, C., Hitselberger, W. E., and Luxford, W. M. (1992). Acoustic tumors: effect of surgical removal on tinnitus. Am. J. Otol. 13, 13–17.
Bokemeyer, C., Berger, C. C., Hartmann, J. T., Kollmannsberger, C., Schmoll, H. J., Kuczyk, M. A., and Kanz, L. (1998). Analysis of risk factors for cisplatin-induced ototoxicity in patients with testicular cancer. Br. J. Cancer 77, 1355–1362.
Brozoski, T. J., Bauer, C. A., and Caspary, D. M. (2002). Elevated fusiform cell activity in the dorsal cochlear nucleus of chinchillas with psychophysical evidence of tinnitus. J. Neurosci. 22, 2383–2390.
Brozoski, T. J., Spires, T. J., and Bauer, C. A. (2007). Vigabatrin, a GABA transaminase inhibitor, reversibly eliminates tinnitus in an animal model. J. Assoc. Res. Otolaryngol. 8, 105–118.
Chan, S. W., and Reade, P. C. (1994). Tinnitus and temporomandibular pain-dysfunction disorder. Clin. Otolaryngol. Allied Sci. 19, 370–380.
Chang, H., Chen, K., Kaltenbach, J. A., Zhang, J., and Godfrey, D. A. (2002). Effects of acoustic trauma on dorsal cochlear nucleus neuron activity in slices. Hear. Res. 164, 59–68.
Chen, G. D., and Jastreboff, P. J. (1995). Salicylate-induced abnormal activity in the inferior colliculus of rats. Hear. Res. 82, 158–178.
Crabtree, J. W. (1999). Intrathalamic sensory connections mediated by the thalamic reticular nucleus. Cell. Mol. Life Sci. 56, 683–700.
De Ridder, D., Fransen, H., Francois, O., Sunaert, S., Kovacs, S., and van de Heyning, P. (2006). Amygdalohippocampal involvement in tinnitus and auditory memory. Acta. Otolaryngol. Suppl. 566, 50–53.
Dehmel, S., Pradhan, S., Koehler, S., Bledsoe, S., and Shore, S. (2012). Noise overexposure alters long-term somatosensory-auditory processing in the dorsal cochlear nucleus–possible basis for tinnitus-related hyperactivity? J. Neurosci. 32, 1660–1671.
Doucet, J. R., Molavi, D. L., and Ryugo, D. K. (2003). The source of corticocollicular and corticobulbar projections in area Te1 of the rat. Exp. Brain Res. 153, 461–466.
Eggermont, J. J., and Komiya, H. (2000). Moderate noise trauma in juvenile cats results in profound cortical topographic map changes in adulthood. Hear. Res. 142, 89–101.
Eggermont, J. J., and Roberts, L. E. (2004). The neuroscience of tinnitus. Trends Neurosci. 27, 676–682.
Engineer, N. D., Riley, J. R., Seale, J. D., Vrana, W. A., Shetake, J. A., Sudanagunta, S. P., Borland, M. S., and Kilgard, M. P. (2011). Reversing pathological neural activity using targeted plasticity. Nature 470, 101–104.
Fox, P. T., Mintun, M. A., Raichle, M. E., Miezin, F. M., Allman, J. M., and van Essen, D. C. (1986). Mapping human visual cortex with positron emission tomography. Nature 323, 806–809.
Fries, P. (2009). Neuronal gamma-band synchronization as a fundamental process in cortical computation. Annu. Rev. Neurosci. 32, 209–224.
Gall, C. M., Hess, U. S., and Lynch, G. (1998). Mapping brain networks engaged by, and changed by, learning. Neurobiol. Learn. Mem. 70, 14–36.
Gu, J. W., Halpin, C. F., Nam, E. C., Levine, R. A., and Melcher, J. R. (2010). Tinnitus, diminished sound-level tolerance, and elevated auditory activity in humans with clinically normal hearing sensitivity. J. Neurophysiol. 104, 3361–3370.
Hillebrand, A., and Barnes, G. R. (2002). A quantitative assessment of the sensitivity of whole-head MEG to activity in the adult human cortex. Neuroimage 16, 638–650.
Hoogenboom, N., Schoffelen, J. M., Oostenveld, R., and Fries, P. (2010). Visually induced gamma-band activity predicts speed of change detection in humans. Neuroimage 51, 1162–1167.
House, J. W., and Brackmann, D. E. (1981). Tinnitus: surgical treatment. Ciba Found. Symp. 85, 204–216.
Jastreboff, P. J., and Sasaki, C. T. (1986). Salicylate-induced changes in spontaneous activity of single units in the inferior colliculus of the guinea pig. J. Acoust. Soc. Am. 80, 1384–1391.
Jeanmonod, D., Magnin, M., and Morel, A. (1996). Low-threshold calcium spike bursts in the human thalamus. Common physiopathology for sensory, motor and limbic positive symptoms. Brain 119(Pt 2), 363–375.
Kaltenbach, J. A., and Afman, C. E. (2000). Hyperactivity in the dorsal cochlear nucleus after intense sound exposure and its resemblance to tone-evoked activity: a physiological model for tinnitus. Hear. Res. 140, 165–172.
Kaltenbach, J. A., Godfrey, D. A., Neumann, J. B., McCaslin, D. L., Afman, C. E., and Zhang, J. (1998). Changes in spontaneous neural activity in the dorsal cochlear nucleus following exposure to intense sound: relation to threshold shift. Hear. Res. 124, 78–84.
Kaltenbach, J. A., Zhang, J., and Afman, C. E. (2000). Plasticity of spontaneous neural activity in the dorsal cochlear nucleus after intense sound exposure. Hear. Res. 147, 282–292.
Landgrebe, M., Langguth, B., Rosengarth, K., Braun, S., Koch, A., Kleinjung, T., May, A., de Ridder, D., and Hajak, G. (2009). Structural brain changes in tinnitus: grey matter decrease in auditory and non-auditory brain areas. Neuroimage 46, 213–218.
Langers, D. R., de Kleine, E., and van Dijk, P. (2012). Tinnitus does not require macroscopic tonotopic map reorganization. Front. Syst. Neurosci. 6:2. doi: 10.3389/fnsys.2012.00002
Leaver, A. M., Renier, L., Chevillet, M. A., Morgan, S., Kim, H. J., and Rauschecker, J. P. (2011). Dysregulation of limbic and auditory networks in tinnitus. Neuron 69, 33–43.
Liberman, M. C., and Kiang, N. Y. (1978). Acoustic trauma in cats. Cochlear pathology and auditory-nerve activity. Acta. Otolaryngol. Suppl. 358, 1–63.
Llinas, R., and Jahnsen, H. (1982). Electrophysiology of mammalian thalamic neurones in vitro. Nature 297, 406–408.
Llinas, R. R., Ribary, U., Jeanmonod, D., Kronberg, E., and Mitra, P. P. (1999). Thalamocortical dysrhythmia: a neurological and neuropsychiatric syndrome characterized by magnetoencephalography. Proc. Natl. Acad. Sci. U.S.A. 96, 15222–15227.
Llinas, R., Urbano, F. J., Leznik, E., Ramirez, R. R., and van Marle, H. J. (2005). Rhythmic and dysrhythmic thalamocortical dynamics: GABA systems and the edge effect. Trends Neurosci. 28, 325–333.
Lockwood, A. H., Salvi, R. J., Coad, M. L., Towsley, M. L., Wack, D. S., and Murphy, B. W. (1998). The functional neuroanatomy of tinnitus: evidence for limbic system links and neural plasticity. Neurology 50, 114–120.
Luo, F., Wang, Q., Kashani, A., and Yan, J. (2008). Corticofugal modulation of initial sound processing in the brain. J. Neurosci. 28, 11615–11621.
Mahlke, C., and Wallhausser-Franke, E. (2004). Evidence for tinnitus-related plasticity in the auditory and limbic system, demonstrated by arg3.1 and c-fos immunocytochemistry. Hear. Res. 195, 17–34.
Melcher, J. R., Levine, R. A., Bergevin, C., and Norris, B. (2009). The auditory midbrain of people with tinnitus: abnormal sound-evoked activity revisited. Hear. Res. 257, 63–74.
Middleton, J. W., Kiritani, T., Pedersen, C., Turner, J. G., Shepherd, G. M., and Tzounopoulos, T. (2011). Mice with behavioral evidence of tinnitus exhibit dorsal cochlear nucleus hyperactivity because of decreased GABAergic inhibition. Proc. Natl. Acad. Sci. U.S.A. 108, 7601–7606.
Muhlnickel, W., Elbert, T., Taub, E., and Flor, H. (1998). Reorganization of auditory cortex in tinnitus. Proc. Natl. Acad. Sci. U.S.A. 95, 10340–10343.
Mulheran, M. (1999). The effects of quinine on cochlear nerve fibre activity in the guinea pig. Hear. Res. 134, 145–152.
Muller, M., Klinke, R., Arnold, W., and Oestreicher, E. (2003). Auditory nerve fibre responses to salicylate revisited. Hear. Res. 183, 37–43.
Norena, A. J., and Eggermont, J. J. (2003). Changes in spontaneous neural activity immediately after an acoustic trauma: implications for neural correlates of tinnitus. Hear. Res. 183, 137–153.
Norena, A. J., and Eggermont, J. J. (2005). Enriched acoustic environment after noise trauma reduces hearing loss and prevents cortical map reorganization. J. Neurosci. 25, 699–705.
Norena, A. J., and Eggermont, J. J. (2006). Enriched acoustic environment after noise trauma abolishes neural signs of tinnitus. Neuroreport 17, 559–563.
Nunez, P. L., and Srinivasan, R. (1981). Electric Fields of the Brain: The Neurophysics of EEG. Oxford: Oxford University Press.
O'Donnell, P., Lavin, A., Enquist, L. W., Grace, A. A., and Card, J. P. (1997). Interconnected parallel circuits between rat nucleus accumbens and thalamus revealed by retrograde transynaptic transport of pseudorabies virus. J. Neurosci. 17, 2143–2167.
Ochi, K., and Eggermont, J. J. (1997). Effects of quinine on neural activity in cat primary auditory cortex. Hear. Res. 105, 105–118.
Ogawa, S., Lee, T. M., Kay, A. R., and Tank, D. W. (1990). Brain magnetic resonance imaging with contrast dependent on blood oxygenation. Proc. Natl. Acad. Sci. U.S.A. 87, 9868–9872.
Passchier-Vermeer, W., and Passchier, W. F. (2000). Noise exposure and public health. Environ. Health Perspect. 108, 123–131.
Paul, A. K., Lobarinas, E., Simmons, R., Wack, D., Luisi, J. C., Spernyak, J., Mazurchuk, R., Abdel-Nabi, H., and Salvi, R. (2009). Metabolic imaging of rat brain during pharmacologically-induced tinnitus. Neuroimage 44, 312–318.
Rauschecker, J. P., Leaver, A. M., and Muhlau, M. (2010). Tuning out the noise: limbic-auditory interactions in tinnitus. Neuron 66, 819–826.
Reinert, K. C., Gao, W., Chen, G., and Ebner, T. J. (2007). Flavoprotein autofluorescence imaging in the cerebellar cortex in vivo. J. Neurosci. Res. 85, 3221–3232.
Seki, S., and Eggermont, J. J. (2003). Changes in spontaneous firing rate and neural synchrony in cat primary auditory cortex after localized tone-induced hearing loss. Hear. Res. 180, 28–38.
Shibuki, K., Hishida, R., Murakami, H., Kudoh, M., Kawaguchi, T., Watanabe, M., Watanabe, S., Kouuchi, T., and Tanaka, R. (2003). Dynamic imaging of somatosensory cortical activity in the rat visualized by flavoprotein autofluorescence. J. Physiol. 549, 919–927.
Suneja, S. K., Benson, C. G., and Potashner, S. J. (1998a). Glycine receptors in adult guinea pig brain stem auditory nuclei: regulation after unilateral cochlear ablation. Exp. Neurol. 154, 473–488.
Suneja, S. K., Potashner, S. J., and Benson, C. G. (1998b). Plastic changes in glycine and GABA release and uptake in adult brain stem auditory nuclei after unilateral middle ear ossicle removal and cochlear ablation. Exp. Neurol. 151, 273–288.
Tzounopoulos, T. (2008). Mechanisms of synaptic plasticity in the dorsal cochlear nucleus: plasticity-induced changes that could underlie tinnitus. Am. J. Audiol. 17, S170–S175.
Wallhausser-Franke, E., Mahlke, C., Oliva, R., Braun, S., Wenz, G., and Langner, G. (2003). Expression of c-fos in auditory and non-auditory brain regions of the gerbil after manipulations that induce tinnitus. Exp. Brain Res. 153, 649–654.
Wang, H., Brozoski, T. J., Turner, J. G., Ling, L., Parrish, J. L., Hughes, L. F., and Caspary, D. M. (2009). Plasticity at glycinergic synapses in dorsal cochlear nucleus of rats with behavioral evidence of tinnitus. Neuroscience 164, 747–759.
Wespatat, V., Tennigkeit, F., and Singer, W. (2004). Phase sensitivity of synaptic modifications in oscillating cells of rat visual cortex. J. Neurosci. 24, 9067–9075.
Womelsdorf, T., Fries, P., Mitra, P. P., and Desimone, R. (2006). Gamma-band synchronization in visual cortex predicts speed of change detection. Nature 439, 733–736.
Womelsdorf, T., Schoffelen, J. M., Oostenveld, R., Singer, W., Desimone, R., Engel, A. K., and Fries, P. (2007). Modulation of neuronal interactions through neuronal synchronization. Science 316, 1609–1612.
Yan, J., and Ehret, G. (2002). Corticofugal modulation of midbrain sound processing in the house mouse. Eur. J. Neurosci. 16, 119–128.
Yan, J., Zhang, Y., and Ehret, G. (2005). Corticofugal shaping of frequency tuning curves in the central nucleus of the inferior colliculus of mice. J. Neurophysiol. 93, 71–83.
Keywords: tinnitus, imaging, fMRI, PET, flavoproteins autofluorescence, auditory, limbic
Citation: Middleton JW and Tzounopoulos T (2012) Imaging the neural correlates of tinnitus: a comparison between animal models and human studies. Front. Syst. Neurosci. 6:35. doi: 10.3389/fnsys.2012.00035
Received: 12 February 2012; Accepted: 17 April 2012;
Published online: 04 May 2012.
Edited by:
Jos J. Eggermont, University of Calgary, CanadaReviewed by:
Jos J. Eggermont, University of Calgary, CanadaSusan Shore, University of Michigan, USA
Copyright: © 2012 Middleton and Tzounopoulos. This is an open-access article distributed under the terms of the Creative Commons Attribution Non Commercial License, which permits non-commercial use, distribution, and reproduction in other forums, provided the original authors and source are credited.
*Correspondence: Jason W. Middleton, Department of Otolaryngology, University of Pittsburgh, Pittsburgh, PA 15213, USA. e-mail: jmiddlet@pitt.edu