Bone Marrow-Derived Cells and Wound Age Estimation
- Department of Forensic Medicine, Wakayama Medical University, Wakayama, Japan
Appropriate technology as well as specific target cells and molecules are key factors for determination of wound vitality or wound age in forensic practice. Wound examination is one of the most important tasks for forensic pathologists and is indispensable to distinguish antemortem wounds from postmortem damage. For vital wounds, estimating the age of the wound is also essential in determining how the wound is associated with the cause of death. We investigated bone marrow-derived cells as promising markers and their potential usefulness in forensic applications. Although examination of a single marker cannot provide high reliability and objectivity in estimating wound age, evaluating the appearance combination of bone marrow-derived cells and the other markers may allow for a more objective and accurate estimation of wound age.
Introduction
Wound healing is a dynamic process in which numerous cells and extracellular matrix structures are involved. These cellular and molecular events are highly regulated. Wound healing is an ordered and controlled progression that matures through artificially defined phases of hemostasis (coagulation), inflammation (infiltration of granulocytes and mononuclear cells), proliferation (epithelization, fibroplasia, and angiogenesis), and maturation (collagen deposition and formation of scarring tissue) (1–5) (Table 1).
In the first step, platelet activation and the coagulation cascade play a major role, with fibrin strands adhering in the first few seconds, subsequently forming blood clots and trapping platelets in the wound area. The inflammatory phase is triggered by the recruitment of inflammatory cells to the wound site and attempts to eliminate damaged cells. Leukocyte recruitment is a hallmark of the inflammatory phase. In the first event, neutrophils infiltrate the wound site for the sterilization, followed by the accumulation of monocytes and lymphocytes. These leukocytes secrete various bioactive molecules, such as cytokines, chemokines, enzymes, and growth factors (6). Cytokines and chemokines are involved in the wound healing process by recruiting leukocytes. To date, several candidates, including IL-1, IL-6, IL-8, and TNF-α, have been identified for wound age determination in the early phase after injury (7–9).
The main objective of the proliferative phase is to cover and fill the wound. The margins of the wound start contacting with fibroblasts that are activated and differentiate into myofibroblasts. Thereafter, the re-epithelialization process also begins. This stage mainly results from extracellular matrix (ECM) deposition of collagen (10–12). Finally, during maturation, collagen fibers are reorganized from collagen type III to type I, tissue is restructured, and strength and flexibility are gained by promoting epithelialization and angiogenesis (13–15). In forensic pathology, growth factors capable of stimulating cell proliferation and cellular differentiation, such as TGF-α and TGF-β1, and some types of collagens have been shown to be available for wound age estimation (16, 17).
Recently, several lines of accumulating studies have shown that bone marrow (BM)-derived cells (BMDCs) may contribute to tissue repair and/or regeneration of damaged tissue including the skin (18–21). After tissue injury, hematopoietic and multipotent progenitor cells are mobilized from the BM into a pool of circulating cells, which migrate to the site of injury and regulate the proliferation and migration of epithelial and dermal mesenchymal cells in the early inflammatory phase (22). The contribution of BMDCs to inflammatory cells in the acute response to injury is well-established, and the long-term role of BMDCs in the healing of skin wounds is being elucidated.
In this review, we assess the characteristics and key functions of BMDCs at each step of the wound healing process and whether they can be useful markers for forensic diagnosis of wound age.
History of Wound Age Estimation
Raekallio first introduced the application of a new method of enzymatic histochemistry and presented some new data for estimating wound age (23). A few years later, an important biochemical technique was reported that involved the detection of serotonin and histamine at the wound edge (24, 25). Over the next decade, significant progress has been made in the scientific research on immunology and immunohistochemistry. The application of immunohistochemical techniques has paved the way for a new field of wound age research by forensic pathologists (26). In the following decade, knowledge of basic immunological principles and application of immunohistochemical methods have led to significant scientific development (7, 8, 17, 27–44). The history of clinical medicine has been correlated with advances in basic research. Since forensic medicine is applied medicine, it is always necessary to apply the latest basic research knowledge to practice forensic medicine.
BM-Derived Hematotoietic Stem Cells
BM-Derived Hematopoietic Stem Cells (BM-HSCs) in Wound Healing
Hematopoietic stem cells (HSCs) constitute a relatively large fraction of BM mononuclear cells (45). Differentiation of HSCs into macrophages is one of the most important events during wound healing (46). There exist two major sources of wound macrophages: resident and BM; the latter accounts for a larger proportion and plays a dominant role in wound healing (47–49). When attracted to the wound, monocytes differentiate into macrophages, which can engage in multiple activities with many possible phenotypes (50, 51). Early in the healing process, macrophages produce multiple cytokines and chemokines that stimulate the inflammatory response (52, 53). Wound macrophages actively phagocytose, removing microbes, dying cells, and necrotic material (54). Several studies have suggested that macrophage phagocytosis of senescent neutrophils causes a switch from a pro-inflammatory to a growth-promoting phenotype (55).
BM-derived monocytes from the circulation are classified as either inflammatory monocytes, which are CD14+CD16− that can differentiate into M1 macrophages, or anti-inflammatory monocytes, which are CD14lowCD16+ that give rise to M2 macrophages (56). In a mouse model of wound healing, circulating monocytes can also be divided into two groups: CX3CR1lowCCR2+Ly6C+, which produces inflammatory cytokines and enters the wound first, and CX3CR1highCCR2−Ly6C− which enters later (57). M1 macrophages play an important role in protection from pathogens by producing high levels of iNOS and inflammatory cytokines such as TNF-α, IL-1b, IL-6, and IL-12, and initiate a Th1 immune response (58). M2 macrophages have anti-inflammatory properties and are characterized by high IL-10 secretion and high arginase-1 expression (58, 59) (Figure 1).
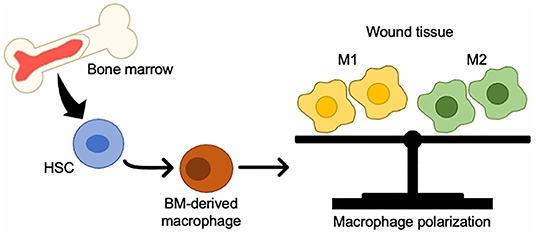
Figure 1. Changes in BMDCs to macrophages in wound tissue. BM-HSCs toward M1/M2 macrophages in injured tissue.
The potential of BM-HSCs in skin regeneration is derived from their high plasticity and involvement in the angiogenesis (60, 61). In addition, they also affect ECM during wound healing by secreting collagen and downregulating MMP expression (62). Moreover, they stimulate the proliferation of keratinocytes and fibroblasts, significantly accelerating wound closure (63).
Macrophages in Wound Age Estimation
Macrophages are mononuclear phagocytes that are recruited from the BM under inflammatory conditions, such as tissue repair (64). Macrophages are involved in host defense, the initiation and resolution of inflammation, growth factor production, phagocytosis, and tissue restoration in wounds (65). During inflammation, macrophages are recruited to the wound site to develop classical and alternative activation phenotypic polarization mediated by cytokines, oxidants, lipids, and growth factors released by macrophages (57, 64, 66). These cells regulate the response to changing wound environments and participate in multiple overlapping wound healing phases.
During early wound healing, macrophages help to clear the wound of contaminating microbes and apoptotic neutrophils and debris via phagocytosis (67–69). In addition, macrophages regulate the activity of other wound cells through the production and release of cytokines, chemokines, and growth factors. Early after injury, macrophages release numerous inflammatory cytokines and chemokines, including IL-1β, IL-6, TNF-α, and CCL2, to amplify the inflammatory response (70). Topical application of CCL2 could promote skin wound healing in diabetic mice, and these effects may be mediated by the action of CCL2 on macrophages (71). Indeed, immunohistochemical studies on the time-dependent expression of chemokines in human skin wounds have shown that inflammatory macrophages are positive for anti-CCL2 antibodies; moreover, a positive rate of > 30% for CCL2 indicates a wound age of at least 1 day (8).
Cyclooxygenase (COX) is an enzyme that is responsible for the formation of prostanoids, including thromboxane and prostaglandins such as prostacyclin, from arachidonic acid (72). COX-1 is constitutively expressed under physiological conditions, and COX-2 is expressed for increased production of prostanoids that occur at the site of disease and inflammation. Therefore, COX-2 may be involved in the inflammatory phase of wound healing. In human skin wound specimens, neutrophils are the main COX-2 expressing cells; however some macrophages also express COX-2 (73). In addition, the number of MMP-2+ and MMP-9+ macrophages significantly increase with wound age (74). These observations indicate that immunohistochemical detection of increased number of MMP-2+ and MMP-9+ macrophages in skin wounds, in combination with other markers such as COX-2, further enhances the reliability of wound age estimation.
Wound macrophages are also an important source of growth factors such as VEGF, which is important for angiogenesis (75, 76). Moreover, macrophages have been shown to be involved in collagen degradation during the tissue remodeling phase of wound healing (77, 78). In human wound specimens with wound ages of >7 days, granulation tissue and angiogenesis were observed with the migration of VEGF+ macrophages (79).
Mast Cells in Wound Age Estimation
Mast cells (MCs), that are one of the immune cells involved in allergy and anaphylaxis, play pivotal roles in skin wound healing thorough the release of chemical mediators such as histamine and the production of cytokines and chemokines (80). From the aspects of wound age estimation, there are several immunohistochemical studies on the dynamics of MCs with focusing on triptase and chimase (81, 82). The number of MCs immediately increased after wounding, eventually reaching a peak at 1–3 h later followed by decreasing within 6 h (81, 82). The post-mortem release of proteins from MCs is known as an influence factor on the data interpretation, which should be taken into consideration in the forensic practices (83). In order to avoid the influences of the postmortem release from MCs, the stem cell factor (SCF) and the Kit receptor, which involved in the survival, growth, migration, and activation of MCs, are investigated. Actually, SCF+ cells rapidly increased in the dermis by day 1 after injury, whereas the Kit receptor elevated more gradually, with a peak on day 14 (84). On the contrary, Oehmichen et al. (85) investigated the loss of MC enzymatic activity at the wound margin, and found the loss of naphtol AS-D chloroacetate esterase (NASDCAE) activity at wound margins in injuries of <60 min (85).
Dendritic Cells (DCs) in Wound Age Estimation
DCs are mononuclear and antigen presenting immune cells. DCs have an ultimate origin in HSCs from the BM (86). Intermediate precursors of DCs lack a lineage-specific marker (lin−) and can be sought among BM cells that have not yet expressed DC markers such as CD11c and surface MHC class II molecules. Later, DC precursors can be found in BM cells that already express the DC marker CD11c, but that still lack cell surface expression of MHC class II molecules (86).
Several studies have indicated that dermal DC recruitment may be involved in the repair process of damaged tissue (87–90). CD11c and HLA-DR are considered specific markers for dermal DCs (91). Kuninaka et al. performed a double-color immunofluorescence analyses with anti-CD11c and anti-HLA-DRα antibodies to detect DCs in human skin wounds from autopsies (92). DCs were rarely detected in wounds aged <1 day, whereas DC accumulation increased over time in wounds aged 3–14 days. These findings suggest that DCs could be a useful cellular marker for determining wound age.
There is a specific DC population in the human epidermis, and those epidermal DCs express CD1a and CD207/langerin, and is called Langerhans cells (93). However, there is only one forensic study exploring the dynamics of dermal DCs after wounding. Bacci et al. (94) investigated the behavior of epidermal DCs/Langerhans cells in relation with wound ages. Both MHC-II+ cells and CD1a+ cells rapidly increased in number within the first hour after injury. Especially, CD1a+ cells, as well-differentiated Langerhans cells, increased earlier and for a shorter time period than MHC-II+ cells. These observations implied that the behavior of epidermal DCs/Langerhans could give a useful information to differentiate antemortem skin lesions from postmortem damage especially in neck compression cases.
BM-Derived Mesenchymal Stem Cells
History of BM-Derived Mesenchymal Stem Cells (BM-MSCs)
BM contains HSCs and MSCs. MSCs were first observed in the BM by Cohnheim in 1867 (95). Cohnheim discovered that these cells could be a source of fibroblasts involved in wound repair. Subsequently, these cells were isolated and cultured by Friedenstein (96). While culturing cells from rat BM, Friedenstein discovered that these cells were a population of non-hematopoietic cells that were morphologically similar to fibroblasts attached to the plastic of the culture flask. The term “mesenchymal stem cells” was presented by Caplan in 1991 after conducting human BM studies (97). To date, it is a hot topic of research that is being explored for multiple purposes.
BM-MSCs in Wound Healing
With the expansion of MSC research, its potential role in skin wound healing has been elucidated. BM-MSCs can accelerate wound healing by regulating the function of inflammatory cells such as neutrophils, macrophages and lymphocytes to provoke an anti-inflammatory response (98). In addition, BM-MSCs can be directed to differentiate into multiple skin cell lineages, including keratinocytes and endothelial cells, and secrete various cytokines to promote wound re-epithelialization and limit excessive scarring (98–103). In addition, BM-MSCs can be recruited to the wound site to induce neovascularization and to increase cell migration and proliferation (104, 105).
Several studies have revealed the underlying mechanisms of BM-MSC recruitment to the wounds. BM-MSCs express CCR7, a receptor of CCL21, which was found to be the main factor responsible for enhanced BM-MSC migration to the wounds in mice (106). Intradermal injection of CCL21 increased the recruitment of BM-MSCs to the wound, resulting in accelerated repair (106). Moreover, serum levels of HMGB1 are increased by skin grafting, and intravenously administered HMGB1 augment the accumulation of PDGFRα+ MSCs in the skin graft by enhancing the expression of the SDF-1 receptor CXCR4 in these cells (107, 108).
The inflammatory phase is important for the wound healing process because it leads to the recruitment of immune cells to remove pathogens and clear the wound. MSCs can suppress the inflammatory responses in several ways. It is generally recognized that infiltrative M2 macrophages play an important role in the progression of wound healing, the promotion of angiogenesis, and the suppression of inflammation (109–111). MSCs promote macrophage polarization to the M2-like functional phenotype, which reduces inflammation and immunosuppressive function (112). Zhao et al. revealed that IL1RA from BM-derived MCSs inhibits the production and activity of IL-1 and TNF-α (113). These studies suggest that MSCs exhibit anti-inflammatory potential through the regulation of macrophage polarization and expression of anti-inflammatory cytokines.
In the proliferative phase, macrophages release growth factors such as EGF and TGF-α to stimulate keratinocyte migration and proliferation (114). Smith et al. revealed that BM-MSCs are a source of soluble signals that regulate dermal fibroblast migration and proliferation (115). MSCs can also contribute to angiogenesis at the wound site. In the wound area, MSCs secrete growth factors such as VEGF, PDGF, bFGF, and angiopoietin-1 to promote angiogenesis and wound healing (116, 117). In addition, SDF-1 secreted by MSCs induces endothelial cell survival, vascular branching, and pericyte recruitment (118). These paracrine mechanisms of MSCs play important roles in angiogenesis. The wounds treated with MSC-seeded hydrogels showed a significant enhancement of angiogenesis, which was associated with elevated VEGF levels within the wound (119). Qiu et al. demonstrated that educated MSC exosomes significantly increase wound healing by inducing angiogenesis (120).
MSCs have also been shown to contribute to the production and remodeling of ECM during the wound healing process. BM-MSCs secrete high levels of TIMPs, which stabilize vessels and protect the vascular basement membrane, forming MMP-induced degradation (121). This ECM production and remodeling function of MSCs may be associated with the promotion of angiogenesis and the formation of granulation tissue.
BM-MSCs may be involved in the regeneration of mesenchymal and other embryonic tissues, including the skin (106). In animal models of wound healing, intravenously transplanted MSCs can differentiate into cells of resident tissue, including fibroblasts, myofibroblasts, vascular endothelial cells, pericytes, and keratinocytes in the wound area (106, 116). In addition, MSCs injected into mouse wounds transdifferentiate into keratin-14+ keratinocytes in vivo (106, 116). Labeled MSCs were observed in the hair follicles, sebaceous glands, and blood vessels in full-thickness wounds in an animal model (122). BM-MSC-engineered skin (EGF loaded) has been found to repair sweat glands and improve skin wound healing (123).
These studies indicate that BM-derived MSCs can differentiate into tissue-specific cells, secrete a wide range of paracrine factors, and regulate the immune response and the local tissue microenvironment (Figure 2).
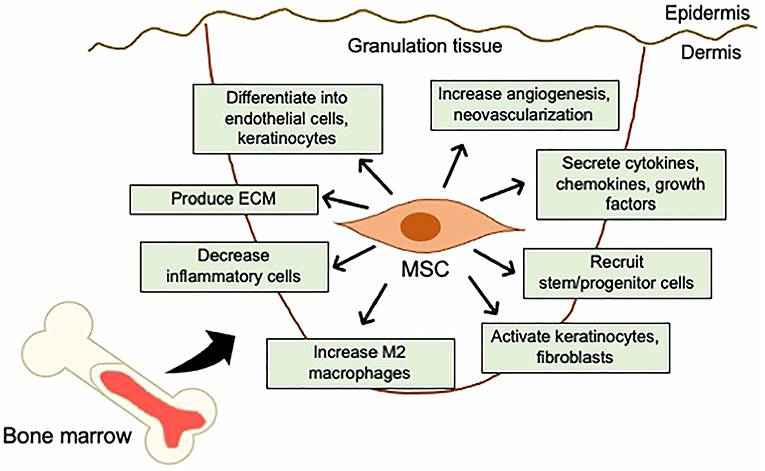
Figure 2. Mechanistic roles of MSCs in the skin wound healing. Mechanisms of acceleration of wound healing by MSCs; (i) activation of keratinocytes and fibroblasts, (ii) increase in angiogenesis and neovascularization, (iii) increase in M2 macrophages infiltration, (iv) recruitment of stem/progenitor cells, (v) secretion of cytokines and growth factors, (vi) production of ECM, (vii) decrease in inflammatory cytokine levels by immunosuppressive effects, and (viii) differentiation into endothelial cells, fibroblasts, and keratinocytes.
Fibrocytes in Wound Age Estimation
In 1994, a distinct population of blood-borne fibroblast-like cells that rapidly entered sites of tissue injury was described (124). These cells, named “fibrocytes,” comprise 0.1–0.5% of the non-erythrocyte cells in the peripheral blood and show an adherent, spindle-shaped morphology when cultured in vitro. Cultured fibrocytes express the fibroblast products including type I collagen I (Col I), type III collagen (Col III), and fibronectin, CD45RO, CD13, and CD34. Additionally, fibrocytes express MHC class II and costimulatory molecules (CD80 and CD86) and can present antigens in vitro and in vivo (125, 126). Fibrocytes differ from monocytes/macrophages, dendritic cells, and other antigen-presenting cells in their morphology, growth properties, and cell surface markers. In addition, fibrocytes isolated from peripheral blood and cultured ex vivo secrete cytokines, growth factors, and chemokines (127). TGF-β functions as a fibrocyte maturation factor during differentiation (128, 129).
There is an increasing evidence that fibrocytes contribute to new fibroblast and myofibroblast populations during wound healing. Prior to differentiation, immature fibrocytes secrete ECM-degrading enzymes, including MMP-2, -7, -8, and -9 which promote the migration of fibrocytes into granulation tissue and endothelial cell invasion (130, 131). CCL21 acts as a potent stimulus for fibrocyte chemotaxis in vitro and for the migration of injected fibrocytes to sites of skin wound site in vivo (128). In addition, exogenous TGF-β1 stimulates in vitro differentiation and synthetic activity of cultured human fibrocytes into mature fibroblasts or myofibroblasts (128). Moreover, fibrocyte differentiation can occur in conditions where serum amyloid P (SAP) and aggregated IgG levels are low, such as during the resolution phase of inflammation (132, 133). The main fibrocyte secreting cytokines include TGF-β1 and CTGF (134). Moreover, fibrocytes indirectly regulate resident fibroblast activity during wound healing (132).
Although the role of fibrocytes in wound healing has been postulated based on their accumulation at the wound sites (124), the molecular signals that mediate the migration of fibrocytes to the wounds have not been investigated. Abe et al. demonstrated that fibrocytes express several chemokine receptors, such as CCR3, CCR5, CCR7, and CXCR4 (128). Furthermore, Ishida et al. showed that Ccl3−/− and Ccr5−/− mice exhibit reduced bleomycin (BLM)-induced fibrosis and the number of CCR5+ fibrocytes in the lungs compared to wild-type mice (135). This finding indicates that the CCL3-CCR5 axis can mediate the migration of BLM-induced fibrocyte to the lungs. In addition, fibrocytes also express CX3CR1, and their population increases in the lungs of mice with BLM-induced pulmonary fibrosis (136). These findings suggests that the CX3CL1-CX3CR1 axis is essential for the development of BLM-induced pulmonary fibrosis by regulating fibrocytes capable of exerting fibrosis-promoting activity. Therefore, some chemokine systems may be involved in the migration of fibrocytes to damaged and fibrotic tissues.
Ishida et al. performed a double-color immunofluorescence analyses using anti-CD45 and anti-Col I antibodies to examine the time-dependent appearance of fibrocytes in human skin wounds of different age groups (137). The appearance of fibrocytes in human skin wounds occurs at least a 4-days post infliction; therefore, detection of fibrocytes could be a useful marker for wound age determination.
Endothelial Progenitor Cells (EPCs) in Wound Age Estimation
EPCs are cells that act as endothelial precursors and help promote angiogenesis to improve tissue perfusion. EPCs were first described in 1997 as a population of postnatal mononuclear blood cells that have been shown to promote angiogenesis following recruitment from the BM (138, 139). EPCs are positive for the following cell surface markers: CD31, CD45, CD14, CD105, CD146, VEGFR-2, CD144, and von Willebrand factor (vWF). Morphologically they appear spindle-shaped (140, 141), and the presence of CD14 and CD45 on these cells indicates that they are hematopoietic rather than of endothelial origin. In addition, markers such as CD31, CD144, VEGFR-2, vWF, and eNOS are not necessarily endothelium-specific (142). There is no single marker that defines EPCs, and a combination of markers has been used to identify them within a heterogeneous population. EPCs are mobilized from the BM through a complex process involving enzymes, growth factors and cell surface receptors. The first step in the EPC mobilization is MMP-9 activation (143). VEGF plays an important role in the activation of MMP-9 and can increase the recruitment of EPCs from the BM (144). Interestingly, fibrocytes can produce angiogenic factors, including MMP-9 and VEGF, as demonstrated in vivo (131).
EPCs are known to be sensitive to hypoxia because they respond to HIF-1-induced SDF-1 under conditions of oxygen deprivation (145). They contribute to angiogenesis and are promising targets for the treatment of chronic wounds such as diabetic ulcers (146–148). Transplanted BM-MSCs induce the recruitment of endogenous EPCs to the wound site from the BM or circulation via growth factors such as VEGF, PDGF, HGF, and insulin-like growth factor and the SDF-1-CXCR4 axis (149–153). Transplantation of human EPCs into a mouse skin wound model has been shown to accelerate wound closure and increase angiogenesis (154). EPC transplantation accelerated wound re-epithelialization in a mouse skin excision wound model compared to that in control mice (155). EPCs produce several chemoattractants of monocytes and macrophages which are known to play important roles in the early stages of wound healing. In addition, EPCs migrate to the wound and are incorporated directly into the newly formed capillaries in the granulation tissue (155). Thus, EPCs have the potential to differente into the endothelium, recruit other cells to the wound site, and secrete growth factors and cytokines; these factors explain the effects on wound healing.
Ishida et al. demonstrated that topical application of CCL2, a potent macrophage chemoattractant, can promote neovascularization, collagen accumulation, and eventual cutaneous wound healing in mice with diabetes (71). The effects of CCL2 may be mediated by EPCs and macrophages, which are critically involved in angiogenesis and collagen production, respectively, and their effects on steps essential to the wound healing process. In addition, the CCL5-CCR5 axis is essential for EPC recruitment (156). In a mouse model of skin wounds, gene expression of the Ccl5 and Ccr5 genes was upregulated at the wound sites and CCR5 protein was detected in endothelial cells. Ccr5−/− mice showed delayed wound healing with diminished neovascularization. The CCR5+ EPCs were directly incorporated into the vasculature at the wound sites. Moreover, EPCs produce growth factors such as TGF-β and VEGF, which are important for skin wound healing (157, 158). These observations suggest that EPCs may contribute to skin wound healing as a source of endothelial cell origin and growth factors.
The accumulation of EPCs in wound sites increases over time after injury; this finding indicates that EPC accumulation may help estimate wound age (156, 159). In forensic practice, examining only a single marker does not provide forensic safety; therefore, some markers need to be investigated in wound samples for a more accurate estimation of wound age. For example, detection of both EPCs and VEGF (79) provides more reliable information for estimating wound age, especially during the proliferative phase, as their collaboration synergistically promotes angiogenesis.
Conclusion and Future Perspectives
Over the last few decades, numerous studies have elucidated the role of BMDCs in skin wound healing (Table 2). It is clear that BMDCs have great potential for skin tissue regeneration as they not only regenerate lost tissue, but also promote wound repair in a paracrine manner. Several cell types, including HSCs and MSCs, are currently being investigated. Recent data on BM cell therapy in skin repair show great promise as therapeutic agents in clinical practice. Further investigation into experimental and clinical applications is required to identify the most effective cell migration system for BM cells at the wound sites. However, it is evident that BMDCs contribute to skin wound healing; therefore, these cells can serve as candidates for wound age estimation in forensic practice. New molecular biomarkers and innovative devices and technologies are constantly being sought to correctly diagnose the cause of death, postmortem interval, wound age, and more.
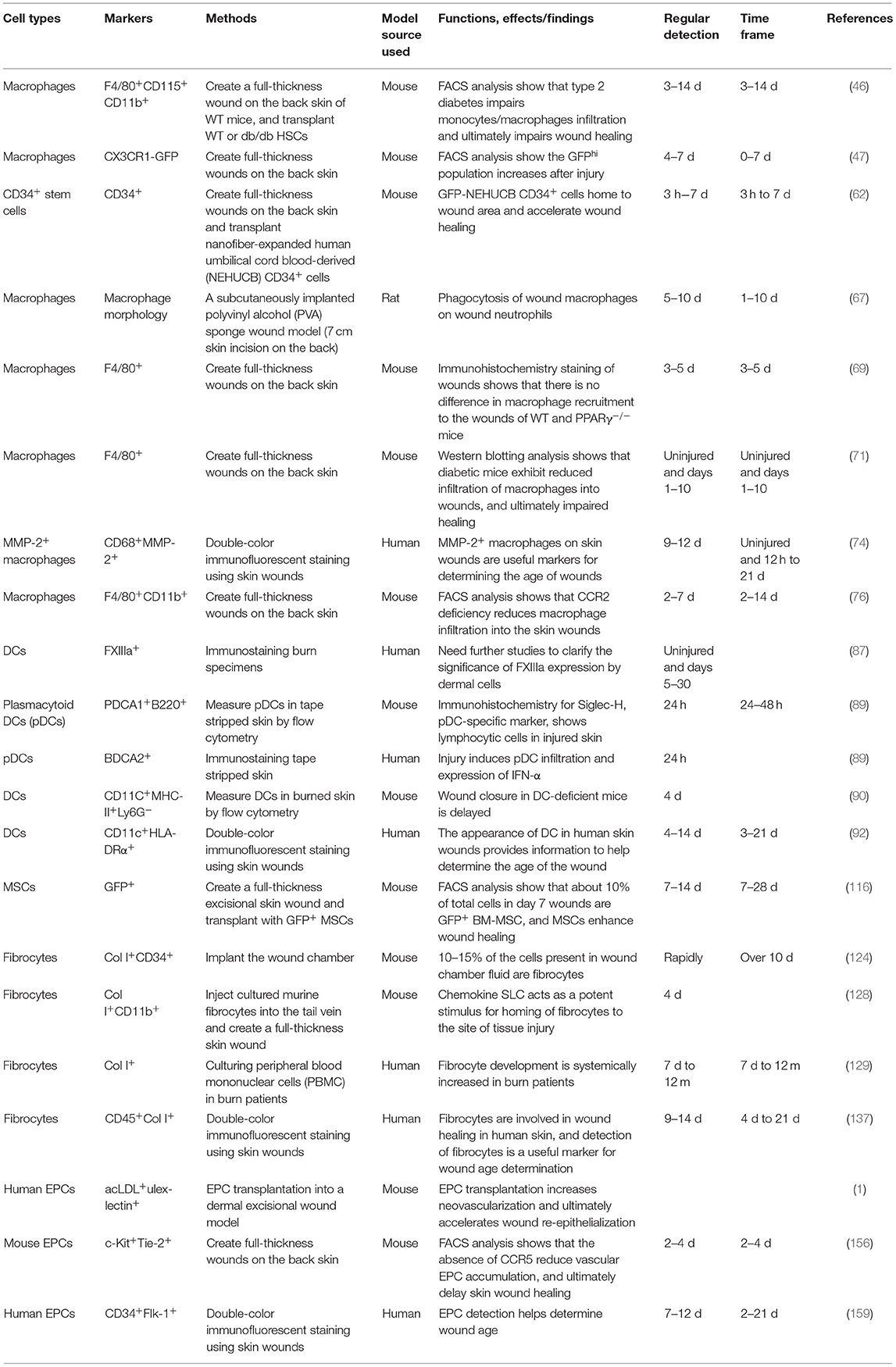
Table 2. Summary of related studies on the appearance and effects of BMDCs on the skin wound healing process.
Recent studies have stimulated us to recognize the importance of BMDCs in the skin; however, many questions remain. For example, BMDCs contribute not only to inflammatory and mesenchymal cells of the dermis, but also to keratinocytes of the epidermis. In addition, it is not yet known whether BM-derived cells are essential to contribute to the cells that make up the normal skin. Furthermore, the specific types of BMDCs playing a role in these processes are unidentified. We believe that answers to these questions will help us understand skin homeostasis and the wound healing process as well as develop new techniques for future skin wound age estimation in future.
Finally, there is a limitation of wound age estimation as the forensic evidence. From the aspects of forensic pathology, the purpose of wound age estimation is to present the objective evidence in court. Cell types, enzymes and chemical mediators are experimentally and practically applied to wound age estimation as the marker (160). Actually, when only a single marker is investigated, contradictory results are often obtained, eventually making confusion the interpretation of data. It is needless to say that various populations of BMCs and BMC-derived enzymes as well as chemical mediators should be investigated. Moreover, among multiple forensic institutes, the accumulation of practical evidence using different types and sizes of wound samples with known post-injured intervals is necessary.
Author Contributions
YI and TK contributed to conception and design of the review, drafted the original manuscript and drew the figures, and provided the main funding for the manuscript. MN edited various versions of the manuscript. All authors read and approved the final version of the review.
Funding
This study was financially supported in part by Grants-in-Aid for Scientific Research (B, 20H03957, YI) and (B, 18H03067, TK) from JSPS.
Conflict of Interest
The authors declare that the research was conducted in the absence of any commercial or financial relationships that could be construed as a potential conflict of interest.
Publisher's Note
All claims expressed in this article are solely those of the authors and do not necessarily represent those of their affiliated organizations, or those of the publisher, the editors and the reviewers. Any product that may be evaluated in this article, or claim that may be made by its manufacturer, is not guaranteed or endorsed by the publisher.
Acknowledgments
We thank my colleagues for their scientific support and appreciate Ms. Mariko Kawaguchi for her excellent assistance in the preparation of this manuscript.
References
1. Stadelmann WK, Digenis AG, Tobin GR. Physiology and healing dynamics of chronic cutaneous wounds. Am J Surg. (1998) 176(2A Suppl.):26S−38. doi: 10.1016/S0002-9610(98)00183-4
2. Midwood KS, Williams LV, Schwarzbauer JE. Tissue repair and the dynamics of the extracellular matrix. Int J Biochem Cell Biol. (2004) 36:1031–7. doi: 10.1016/j.biocel.2003.12.003
3. Theoret CL. The pathophysiology of wound repair. Vet Clin North Am Equine Pract. (2005) 21:1–13. doi: 10.1016/j.cveq.2004.11.001
4. Furie B, Furie BC. Mechanisms of thrombus formation. N Engl J Med. (2008) 359:938–49. doi: 10.1056/NEJMra0801082
5. Velnar T, Bailey T, Smrkolj V. The wound healing process: an overview of the cellular and molecular mechanisms. J Int Med Res. (2009) 37:1528–42. doi: 10.1177/147323000903700531
6. Landén NX, Li D, Ståhle M. Transition from inflammation to proliferation: a critical step during wound healing. Cell Mol Life Sci. (2016) 73:3861–85. doi: 10.1007/s00018-016-2268-0
7. Kondo T, Ohshima T, Eisenmenger W. Immunohistochemical and morphometrical study on the temporal expression of interleukin-1α (IL-1α) in human skin wounds for forensic wound age determination. Int J Legal Med. (1999)112:249–52. doi: 10.1007/s004140050244
8. Kondo T, Ohshima T, Mori R, Guan DW, Ohshima K, Eisenmenger W. Immunohistochemical detection of chemokines in human skin wounds and its application to wound age determination. Int J Legal Med. (2002) 116:87–91. doi: 10.1007/s004140100260
9. Grellner W. Time-dependent immunohistochemical detection of proinflammatory cytokines (IL-1β, IL-6, TNF-α) in human skin wounds. Forensic Sci Int. (2002) 130:90–6. doi: 10.1016/S0379-0738(02)00342-0
10. Caley MP, Martins VLC, O'Toole EA. Metalloproteinases and wound healing. Adv Wound Care. (2015) 4:225–234. doi: 10.1089/wound.2014.0581
11. Kim BC, Kim HT, Park SH, Cha JS, Yufit T, Kim SJ, et al. Fibroblasts from chronic wounds show altered TGF-β-signaling and decreased TGF-β Type II receptor expression. J Cell Physiol. (2003) 195:331–6. doi: 10.1002/jcp.10301
12. Pastar I, Stojadinovic O, Yin NC, Ramirez H, Nusbaum AG, Sawaya A, et al. Epithelialization in Wound Healing: A Comprehensive Review. Adv Wound Care (New Rochelle). (2014) 3:445–464. doi: 10.1089/wound.2013.0473
13. Leavitt T, Hu MS, Marshall CD, Barnes LA, Lorenz HP, Longaker MT. Scarless wound healing: finding the right cells and signals. Cell Tissue Res. (2016) 365:483–93. doi: 10.1007/s00441-016-2424-8
14. Baron JM, Glatz M, Proksch E. Optimal support of wound healing: new insights. Dermatology. (2020) 236:593–600. doi: 10.1159/000505291
15. Pakyari M, Farrokhi A, Maharlooei MK, Ghahary A. Critical role of transforming growth factor beta in different phases of wound healing. Adv Wound Care. (2013) 2:215–24. doi: 10.1089/wound.2012.0406
16. Grellner W, Vieler S, Madea B. Transforming growth factors (TGF-α and TGF-β1) in the determination of vitality and wound age: immunohistochemical study on human skin wounds. Forensic Sci Int. (2005) 153:174–80. doi: 10.1016/j.forsciint.2004.08.021
17. Betz P. Immunohistochemical parameters for the age estimation of human skin wounds. A review. Am J Forensic Med Pathol. (1995) 16:203–9. doi: 10.1097/00000433-199509000-00003
18. Kataoka K, Medina RJ, Kageyama T, Miyazaki M, Yoshino T, Makino T, et al. Participation of adult mouse bone marrow cells in reconstitution of skin. Am J Pathol. (2003) 163:1227–31. doi: 10.1016/S0002-9440(10)63482-7
19. Nakagawa H, Akita S, Fukui M, Fujii T, Akino K. Human mesenchymal stem cells successfully improve skin-substitute wound healing. Br J Dermatol. (2005) 153:29–36. doi: 10.1111/j.1365-2133.2005.06554.x
20. Devine SM, Cobbs C, Jennings M, Bartholomew A, Ron Hoffman R. Mesenchymal stem cells distribute to a wide range of tissues following systemic infusion into nonhuman primates. Blood. (2003) 101:2999–3001. doi: 10.1182/blood-2002-06-1830
21. Kawada H, Fujita J, Kinjo K, Matsuzaki Y, Tsuma M, Miyatake H, et al. Nonhematopoietic mesenchymal stem cells can be mobilized and differentiate into cardiomyocytes after myocardial infarction. Blood. (2004) 104:3581–7. doi: 10.1182/blood-2004-04-1488
22. Singer AJ, Clark RA. Cutaneous wound healing. N Engl J Med. (1999) 341:738–46. doi: 10.1056/NEJM199909023411006
23. Raekallio J. Determination of the age of wounds by histochemical and biochemical methods. Forensic Sci. (1972) 1:3–16. doi: 10.1016/0300-9432(72)90144-6
24. Berg S, Ditt J, Friedrich D, Bonte W. Possibilities of biochemical wound age determination. Dtsch Z Gesamte Gerichtl Med. (1968) 63:183–98. doi: 10.1007/BF00592087
25. Berg S, Ditt J, Kunze P, Garbe G. Histamine content and histidine-decarboxylase-activity in dermal injuries. Z Rechtsmed. (1971) 69:26–40. doi: 10.1007/BF02092634
26. Eisenmenger W, Nerlich A, Glück G. The significance of collagen in determining the age of a wound. Z Rechtsmed. (1988) 100:79–100. doi: 10.1007/BF00200749
27. Fieguth A, Kleemann WJ, Tröger HD. Immunohistochemical examination of skin wounds with antibodies against α-1-antichymotrypsin, α-2-macroglobulin and lysozyme. Int J Legal Med. (1994) 107:29–33. doi: 10.1007/BF01247271
28. Dressler J, Bachmann L, Kasper M, Hauck JG, Müller E. Time dependence of the expression of ICAM-1 (CD 54) in human skin wounds. Int J Legal Med. (1997) 110:299–304. doi: 10.1007/s004140050092
29. Dressler J, Bachmann L, Koch R, Müller E. Estimation of wound age and VCAM-1 in human skin. Int J Legal Med. (1999) 112:159–62. doi: 10.1007/s004140050223
30. Dressler J, Bachmann L, Strejc P, Koch R, Müller E. Expression of adhesion molecules in skin wounds: diagnostic value in legal medicine. Forensic Sci Int. (2000) 113:173–6. doi: 10.1016/S0379-0738(00)00258-9
31. Grellner W, Dimmeler S, Madea B. Immunohistochemical detection of fibronectin in postmortem incised wounds of porcine skin. Forensic Sci Int. (1998) 97:109–16. doi: 10.1016/S0379-0738(98)00147-9
32. Fieguth A, Feldbrügge H, Gerich T, Kleemann WJ, Tröger HD. The time-dependent expression of fibronectin, MRP8, MRP14 and defensin in surgically treated human skin wounds. Forensic Sci Int. (2003) 131:156–61. doi: 10.1016/S0379-0738(02)00428-0
33. Hernández-Cueto C, Girela E, Sweet DJ. Advances in the diagnosis of wound vitality: a review. Am J Forensic Med Pathol. (2000) 21:21–31. doi: 10.1097/00000433-200003000-00004
34. Hernández-Cueto C, Vieira DN, Girela E, Marques E, Villanueva E, Sá FO. Diagnostic ability of D-dimer in the establishment of the vitality of wounds. Forensic Sci Int. (1995) 76:141–9. doi: 10.1016/0379-0738(95)01808-5
35. Ortiz-Rey JA, Suárez-Peñaranda JM, Muñoz-Barús JI, Álvarez C, San Miguel P, Rodríguez-Calvo MS, et al. Expression of fibronectin and tenascin as a demonstration of vital reaction in rat skin and muscle. Int J Legal Med. (2003) 117:356–60. doi: 10.1007/s00414-003-0403-6
36. Kondo T, Tanaka J, Ishida Y, Mori R, Takayasu T, Ohshima T. Ubiquitin expression in skin wounds and its application to forensic wound age determination. Int J Legal Med. (2002) 116:267–72. doi: 10.1007/s00414-002-0322-y
37. Kondo T, Ohshima T, Sato Y, Mayama T, Eisenmenger W. Immunohistochemical study on the expression of c-Fos and c-Jun in human skin wounds. Histochem J. (2000) 32:509–14. doi: 10.1023/A:1004164905041
38. Kondo T, Ohshima T. The dynamics of inflammatory cytokines in the healing process of mouse skin wound: a preliminary study for possible wound age determination. Int J Legal Med. (1996) 108:231–6. doi: 10.1007/BF01369816
39. Betz P, Nerlich A, Wilske J, Wiest I, Penning R, Eiseumenger W. Comparison of the solophenyl-red polarization method and the immunohistochemical analysis for collagen type III. Int J Legal Med. (1992) 105:27–9. doi: 10.1007/BF01371233
40. Betz P, Nerlich A, Wilskel J, Tübel J, Wiest I, Penning R, et al. Immunohistochemical localization of fibronectin as a tool for the age determination of human skin wounds. Int J Legal Med. (1992) 105:21–6. doi: 10.1007/BF01371232
41. Betz P, Nerlich A, Wilske J, Tübel J, Wiest I, Penning R, et al. The time-dependent rearrangement of the epithelial basement membrane in human skin wounds -immunohistochemical localization of Collagen IV and VII. Int J Legal Med. (1992) 105:93–7. doi: 10.1007/BF02340831
42. Hausmann R, Nerlich A, Betz P. The time-related expression of p53 protein in human skin wounds - a quantitative immunohistochemical analysis. Int J Legal Med. (1998) 111:169–72. doi: 10.1007/s004140050142
43. Betz P, Tübel J, Eisenmenger W. Immunohistochemical analysis of markers for different macrophage phenotypes and their use for a forensic wound age estimation. Int J Legal Med. (1995) 107:197–200. doi: 10.1007/BF01428405
44. Oehmichen M, Cröpelin A. Temporal course of intravital and postmortem proliferation of epidermal cells after mechanical injury - an immunohistochemical study using bromodeoxyuridine in rats. Int J Legal Med. (1995) 107:257–62. doi: 10.1007/BF01245484
45. Li H, Ghazanfari R, Zacharaki D, Lim HC, Scheding S. Isolation and characterization of primary bone marrow mesenchymal stromal cells. Ann N Y Acad Sci. (2016) 1370:109–18. doi: 10.1111/nyas.13102
46. Yan J, Tie G, Wang S, Tutto A, Demarco N, Khair L, et al. Diabetes impairs wound healing by Dnmt1-dependent dysregulation of hematopoietic stem cells differentiation towards macrophages. Nat Commun. (2018) 9:33. doi: 10.1038/s41467-017-02425-z
47. Burgess M, Wicks K, Gardasevic M, Mace KA. Cx3CR1 expression identifies distinct macrophage populations that contribute differentially to inflammation and repair. ImmunoHorizons. (2019) 3:262–73. doi: 10.4049/immunohorizons.1900038
48. Davies LC, Jenkins SJ, Allen JE, Taylor PR. Tissue-resident macrophages. Nat Immunol. (2013) 14:986–95. doi: 10.1038/ni.2705
49. Malissen B, Tamoutounour S, Henri S. The origins and functions of dendritic cells and macrophages in the skin. Nat Rev Immunol. (2014) 14:417–28. doi: 10.1038/nri3683
50. Daley JM, Reichner JS, Mahoney EJ, Manfield L, Henry WL, Mastrofrancesco B, et al. Modulation of macrophage phenotype by soluble product(s) released from neutrophils. J Immunol. (2005) 174:2265–72. doi: 10.4049/jimmunol.174.4.2265
51. Novak ML, Koh TJ. Phenotypic transitions of macrophages orchestrate tissue repair. Am J Pathol. (2013) 183:1352–63. doi: 10.1016/j.ajpath.2013.06.034
52. DiPietro LA, Burdick M, Low QE, Kunkel SL, Strieter RM. Mip-1α as a critical macrophage chemoattractant in murine wound repair. J Clin Invest. (1998) 101:1693–8. doi: 10.1172/JCI1020
53. DiPietro LA, Reintjes MG, Low QEH, Levi B, Gamelli RL. Modulation of macrophage recruitment into wounds by monocyte chemoattractant protein-1. Wound Repair Regenerat. (2001) 9:28–33. doi: 10.1046/j.1524-475x.2001.00028.x
54. Greenlee-Wacker MC. Clearance of apoptotic neutrophils and resolution of inflammation. Immunol Rev. (2016) 273:357–70. doi: 10.1111/imr.12453
55. Khanna S, Biswas S, Shang Y, Collard E, Azad A, Kauh C, et al. Macrophage dysfunction impairs resolution of inflammation in the wounds of diabetic mice. PLoS ONE. (2010) 5:e9539. doi: 10.1371/journal.pone.0009539
56. Raziyeva K, Kim Y, Zharkinbekov Z, Kassymbek K, Jimi S, Saparov A. Immunology of acute and chronic wound healing. Biomolecules. (2021) 11:700. doi: 10.3390/biom11050700
57. Brancato SK, Albina JE. Wound macrophages as key regulators of repair: origin, phenotype, and function. Am J Pathol. (2011) 178:19–25. doi: 10.1016/j.ajpath.2010.08.003
58. Martinez FO, Sica A, Mantovani A, Locati M. Macrophage activation and polarization. Front Biosci. (2008) 13:453–61. doi: 10.2741/2692
59. Naito Y, Takagi T, Higashimura Y. Heme oxygenase-1 and anti-inflammatory M2 macrophages. Arch Biochem Biophys. (2014) 564:83–8. doi: 10.1016/j.abb.2014.09.005
60. Kamolz LP, Kolbus A, Wick N, Mazal PR, Eisenbock B, Burjak S, et al. Cultured human epithelium: human umbilical cord blood stem cells differentiate into keratinocytes under in vitro conditions. Burns. (2006) 32:16–9. doi: 10.1016/j.burns.2005.08.020
61. Sackstein R. The bone marrow is akin to skin: HCELL and the biology of hematopoietic stem cell homing. J Invest Dermatol. (2004) 122:1061–9. doi: 10.1111/j.0022-202X.2004.09301.x
62. Kanji S, Das M, Aggarwal R, Lu J, Joseph M, Basu S, et al. Nanofiber-expanded human umbilical cord blood-derived CD34+ cell therapy accelerates murine cutaneous wound closure by attenuating pro-inflammatory factors and secreting IL-10. Stem Cell Res. (2014) 12:275–88. doi: 10.1016/j.scr.2013.11.005
63. Kim JY, Song SH, Kim KL, Ko JJ, Im JE, Yie SW, et al. Human cord blood-derived endothelial progenitor cells and their conditioned media exhibit therapeutic equivalence for diabetic wound healing. Cell Transplant. (2010) 19:1635–44. doi: 10.3727/096368910X516637
64. Laskin DL, Sunil VR, Gardner CR, Laskin JD. Macrophages and tissue injury: agents of defense or destruction? Annu Rev Pharmacol Toxicol. (2011) 51:267–88. doi: 10.1146/annurev.pharmtox.010909.105812
65. Snyder RJ, Lantis J, Kirsner RS, Shah V, Molyneaux M, Carter MJ. Macrophages: a review of their role in wound healing and their therapeutic use. Wound Repair Regenerat. (2016) 24:613–29. doi: 10.1111/wrr.12444
66. Delavary BM, van der Veer WM, van Egmond M, Niessen FB, Beelen RHJ. Macrophages in skin injury and repair. Immunobiology. (2011) 216:753–62. doi: 10.1016/j.imbio.2011.01.001
67. Meszaros AJ, Reichner JS, Albina JE. Macrophage phagocytosis of wound neutrophils. J Leukoc Biol. (1999) 65:35–42. doi: 10.1002/jlb.65.1.35
68. Soehnlein O, Lindbom L. Phagocyte partnership during the onset and resolution of inflammation. Nat Rev Immunol. (2010) 10:427–39. doi: 10.1038/nri2779
69. Chen H, Shi R, Luo B, Yang X, Qiu L, Xiong J, et al. Macrophage peroxisome proliferator-activated receptor B deficiency delays skin wound healing through impairing apoptotic cell clearance in mice. Cell Death Dis. (2015) 6:e1597. doi: 10.1038/cddis.2014.544
70. Barrientos S, Stojadinovic O, Golinko MS, Brem H, Tomic-Canic M. Growth factors and cytokines in wound healing. Wound Repair Regenerat. (2008) 16:585–601. doi: 10.1111/j.1524-475X.2008.00410.x
71. Ishida Y, Kuninaka Y, Nosaka M, Furuta M, Kimura A, Taruya A, et al. CCL2-mediated reversal of impaired skin wound healing in diabetic mice by normalization of neovascularization and collagen accumulation. J Invest Dermatol. (2019) 139:2517–27.e5. doi: 10.1016/j.jid.2019.05.022
72. Dubois RN, Abramson SB, Crofford L, Gupta RA, Simon LS, van de Putte LB, et al. Cyclooxygenase in biology and disease. FASEB J. (1998) 12:1063–73. doi: 10.1096/fasebj.12.12.1063
73. Ishida Y, Kimura A, Nosaka M, Kuninaka Y, Takayasu T, Eisenmenger W, et al. Immunohistochemical analysis on cyclooxygenase-2 for wound age determination. Int J Legal Med. (2012) 126:435–40. doi: 10.1007/s00414-012-0685-7
74. Ishida Y, Kuninaka Y, Nosaka M, Kimura A, Kawaguchi T, Hama M, et al. Immunohistochemical analysis on MMP-2 and MMP-9 for wound age determination. Int J Legal Med. (2015) 129:1043–8. doi: 10.1007/s00414-015-1167-5
75. Stockmann C, Kirmse S, Helfrich I, Weidemann A, Takeda N, Doedens A, et al. A wound size-dependent effect of myeloid cell-derived vascular endothelial growth factor on wound healing. J Invest Dermatol. (2011) 131:797–801. doi: 10.1038/jid.2010.345
76. Willenborg S, Lucas T, van Loo G, Knipper JA, Krieg T, Haase I, et al. CCR2 recruits an inflammatory macrophage subpopulation critical for angiogenesis in tissue repair. Blood. (2012) 120:613–25. doi: 10.1182/blood-2012-01-403386
77. Madsen DH, Leonard D, Masedunskas A, Moyer A, Jürgensen HJ, Peters DE, et al. M2-like macrophages are responsible for collagen degradation through a mannose receptor-mediated pathway. J Cell Biol. (2013) 202:951–66. doi: 10.1083/jcb.201301081
78. Roch T, Akymenko O, Krüger A, Jung F, Ma N, Lendlein A. Expression pattern analysis and activity determination of matrix metalloproteinase derived from human macrophage subsets. Clin Hemorheol Microcirc. (2014) 58:147–58. doi: 10.3233/CH-141885
79. Hayashi T, Ishida Y, Kimura A, Takayasu T, Eisenmenger W, Kondo T. Forensic application of VEGF expression to skin wound age determination. Int J Legal Med. (2004) 118:320–5. doi: 10.1007/s00414-004-0468-x
80. Trautmann A, Toksoy A, Engelhardt E, Bröcker EB, Gillitzer R. Mast cell involvement in normal human skin wound healing: expression of monocyte chemoattractant protein-1 is correlated with recruitment of mast cells which synthesize interleukin-4 in vivo. J Pathol. (2000) 190:100–6. doi: 10.1002/(SICI)1096-9896(200001)190:1<100::AID-PATH496>3.0.CO;2-Q
81. Bonelli A, Bacci S, Vannelli B, Norelli A. Immunohistochemical localization of mast cells as a tool for the discrimination of vital and postmortem lesions. Int J Legal Med. (2003) 117:14–8. doi: 10.1007/s00414-002-0304-0
82. Bonelli A, Bacci S, Norelli G. A. Affinity cytochemistry analysis of mast cells in skin lesions: a possible tool to assess the timing of lesions after death. Int J Legal Med. (2003) 117:331–4. doi: 10.1007/s00414-003-0396-1
83. Betz P. Histological and enzyme histochemical parameters for the age estimation of human skin wounds. Int J Legal Med. (1994) 107:60–8. doi: 10.1007/BF01225491
84. Huttunen M, Naukkarinen A, Horsmanheimo M, Harvima IT. Transient production of stem cell factor in dermal cells but increasing expression of Kit receptor in mast cells during normal wound healing. Arch Dermatol Res. (2002) 294:324–30. doi: 10.1007/s00403-002-0331-1
85. Oehmichen M, Gronki T, Meissner C, Anlauf M, Schwark T. Mast cell reactivity at the margin of human skin wounds: an early cell marker of wound survival? Forensic Sci Int. (2009) 191:1–5. doi: 10.1016/j.forsciint.2009.05.020
86. Shortman K, Naik SH. Steady-state and inflammatory dendritic-cell development. Nat Rev Immunol. (2007) 7:19–30. doi: 10.1038/nri1996
87. Gibran NS, Heimbach DM, Holbrook KA. Immunolocalization of FXIIIa+ dendritic cells in human burn wounds. J Surg Res. (1995) 59:378–86. doi: 10.1006/jsre.1995.1179
88. Gao N, Yin J, Yoon GS, Mi QS, Yu FSX. Dendritic cell epithelium interplay is a determinant factor for corneal epithelial wound repair. Am J Pathol. (2011) 179:2243–53. doi: 10.1016/j.ajpath.2011.07.050
89. Gregorio J, Meller S, Conrad C, di Nardo A, Homey B, Lauerma A, et al. Plasmacytoid dendritic cells sense skin injury and promote wound healing through type I interferons. J Exp Med. (2010) 207:2921–30. doi: 10.1084/jem.20101102
90. Vinish M, Cui W, Stafford E, Bae L, Hawkins H, Cox R, et al. Dendritic cells modulate burn wound healing by enhancing early proliferation. Wound Repair Regenerat. (2016) 24:6–13. doi: 10.1111/wrr.12388
91. Meunier L, Gonzalez-Ramos A, Cooper KD. Heterogeneous populations of class II MHC+ cells in human dermal cell suspensions. Identification of a small subset responsible for potent dermal antigen-presenting cell activity with features analogous to Langerhans cells. J Immunol. (1993) 151:4067–80.
92. Kuninaka Y, Ishida Y, Nosaka M, Shimada E, Kimura A, Ozaki M, et al. Forensic pathological study on temporal appearance of dendritic cells in skin wounds. Int J Legal Med. (2020) 134:597–601. doi: 10.1007/s00414-019-02185-z
93. Mizumoto N, Takashima A. CD1a and langerin: acting as more than Langerhans cell markers. J Clin Invest. (2004) 113:658–60. doi: 10.1172/JCI200421140
94. Bacci S, Defraia B, Cinci L, Calosi L, Guasti D, Pieri L, et al. Immunohistochemical analysis of dendritic cells in skin lesions: correlations with survival time. Forensic Sci Int. (2014) 244:179–85. doi: 10.1016/j.forsciint.2014.08.024
95. Chamberlain G, Fox J, Ashton B, Middleton J. Concise review: mesenchymal stem cells: their phenotype, differentiation capacity, immunological features, and potential for homing. Stem Cells. (2007) 25:2739–49. doi: 10.1634/stemcells.2007-0197
96. Friedenstein AJ, Petrakova K, Kurolesova AI, Frolova GP. Heterotopic of bone marrow. Analysis of precursor cells for osteogenic and hematopoietic tissues. Transplantation. (1968) 6:230–47. doi: 10.1097/00007890-196803000-00009
98. Nuschke A. Activity of mesenchymal stem cells in therapies for chronic skin wound healing. Organogenesis. (2014) 10:29–37. doi: 10.4161/org.27405
99. Pǎunescu V, Deak E, Herman D, Siska IR, Tǎnasie G, Bunu C, et al. In vitro differentiation of human mesenchymal stem cells to epithelial lineage. J Cell Mol Med. (2007) 11:502–8. doi: 10.1111/j.1582-4934.2007.00041.x
100. Oswald J, Boxberger S, Joergensen B, Bornhaeuser M, Ehninger G, Werner C. Mesenchymal Stem Cells (MSC) can be differentiated into endothelial cells in vitro. Transactions. (2004) 22:377–84. doi: 10.1634/stemcells.22-3-377
101. Wu Y, Huang S, Enhe J, Ma K, Yang S, Sun T, et al. Bone marrow-derived mesenchymal stem cell attenuates skin fibrosis development in mice. Int Wound J. (2014) 11:701–10. doi: 10.1111/iwj.12034
102. Ward CL, Sanchez CJ, Pollot BE, Romano DR, Hardy SK, Becerra SC, et al. Soluble factors from biofilms of wound pathogens modulate human bone marrow-derived stromal cell differentiation, migration, angiogenesis, and cytokine secretion Microbe-host interactions and microbial pathogenicity. BMC Microbiol. (2015) 15:75. doi: 10.1186/s12866-015-0412-x
103. Zou JP, Huang S, Peng Y, Liu HW, Cheng B, Fu XB, et al. Mesenchymal stem cells/multipotent mesenchymal stromal cells (MSCs): potential role in healing cutaneous chronic wounds. Int J Lower Extremity Wounds. (2012) 11:244–53. doi: 10.1177/1534734612463935
104. Wu Y, Zhao RCH, Tredget EE. Concise review: bone marrow-derived stem/progenitor cells in cutaneous repair and regeneration. Stem Cells. (2010) 28:905–15. doi: 10.1002/stem.420
105. Shabbir A, Cox A, Rodriguez-Menocal L, Salgado M, van Badiavas E. Mesenchymal stem cell exosomes induce proliferation and migration of normal and chronic wound fibroblasts, and enhance angiogenesis in vitro. Stem Cells Dev. (2015) 24:1635–47. doi: 10.1089/scd.2014.0316
106. Sasaki M, Abe R, Fujita Y, Ando S, Inokuma D, Shimizu H. Mesenchymal stem cells are recruited into wounded skin and contribute to wound repair by transdifferentiation into multiple skin cell type. J Immunol. (2008) 180:2581–7. doi: 10.4049/jimmunol.180.4.2581
107. Tamai K, Yamazaki T, Chino T, Ishii M, Otsuru S, Kikuchi Y, et al. PDGFRα-positive cells in bone marrow are mobilized by high mobility group box 1 (HMGB1) to regenerate injured epithelia. Proc Natl Acad Sci USA. (2011) 108:6609–14. doi: 10.1073/pnas.1016753108
108. Aikawa E, Fujita R, Kikuchi Y, Kaneda Y, Tamai K. Systemic high-mobility group box 1 administration suppresses skin inflammation by inducing an accumulation of PDGFRα+ mesenchymal cells from bone marrow. Sci Rep. (2015) 5:11008. doi: 10.1038/srep11008
109. Ferrante CJ, Leibovich SJ. Regulation of macrophage polarization and wound healing. Adv Wound Care. (2012) 1:10–6. doi: 10.1089/wound.2011.0307
110. Jia XH, Feng GW, Wang ZL, Du Y, Shen C, Hui H, et al. Activation of mesenchymal stem cells by macrophages promotes tumor progression through immune suppressive effects. Oncotarget. (2016) 7:20934–44. doi: 10.18632/oncotarget.8064
111. Zhang QZ, Su WR, Shi SH, Wilder-Smith P, Xiang AP, Wong A, et al. Human gingiva-derived mesenchymal stem cells elicit polarization of M2 macrophages and enhance cutaneous wound healing. Stem Cells. (2010) 28:1856–68. doi: 10.1002/stem.503
112. Chiossone L, Conte R, Spaggiari GM, Serra M, Romei C, Bellora F, et al. Mesenchymal stromal cells induce peculiar alternatively activated macrophages capable of dampening both innate and adaptive immune responses. Stem Cells. (2016) 34:1909–21. doi: 10.1002/stem.2369
113. Zhao MM, Cui JZ, Cui Y, Li R, Tian YX, Song SX, et al. Therapeutic effect of exogenous bone marrow-derived mesenchymal stem cell transplantation on silicosis via paracrine mechanisms in rats. Mol Med Rep. (2013) 8:741–6. doi: 10.3892/mmr.2013.1580
114. Ellis S, Lin EJ, Tartar D. Immunology of wound healing. Curr Dermatol Rep. (2018) 7:350–8. doi: 10.1007/s13671-018-0234-9
115. Smith AN, Willis E, Chan VT, Muffley LA, Isik FF, Gibran NS, et al. Mesenchymal stem cells induce dermal fibroblast responses to injury. Exp Cell Res. (2010) 316:48–54. doi: 10.1016/j.yexcr.2009.08.001
116. Wu Y, Chen L, Scott PG, Tredget EE. Mesenchymal stem cells enhance wound healing through differentiation and angiogenesis. Stem Cells. (2007) 25:2648–59. doi: 10.1634/stemcells.2007-0226
117. Volarevic V, Arsenijevic N, Lukic ML, Stojkovic M. Concise review: mesenchymal stem cell treatment of the complications of diabetes mellitus. Stem Cells. (2011) 29:5–10. doi: 10.1002/stem.556
118. Galiano RD, Tepper OM, Pelo CR, Bhatt KA, Callaghan M, Bastidas N, et al. Topical vascular endothelial growth factor accelerates diabetic wound healing through increased angiogenesis and by mobilizing and recruiting bone marrow-derived cells. Am J Pathol. (2004) 164:1935–47. doi: 10.1016/S0002-9440(10)63754-6
119. Rustad KC, Wong VW, Sorkin M, Glotzbach JP, Major MR, Rajadas J, et al. Enhancement of mesenchymal stem cell angiogenic capacity and stemness by a biomimetic hydrogel scaffold. Biomaterials. (2012) 33:80–90. doi: 10.1016/j.biomaterials.2011.09.041
120. Qiu X, Liu J, Zheng C, Su Y, Bao L, Zhu B, et al. Exosomes released from educated mesenchymal stem cells accelerate cutaneous wound healing via promoting angiogenesis. Cell Prolifer. (2020) 53:e12830. doi: 10.1111/cpr.12830
121. Lozito TP, Tuan RS. Mesenchymal stem cells inhibit both endogenous and exogenous MMPs via secreted TIMPs. J Cell Physiol. (2011) 226:385–96. doi: 10.1002/jcp.22344
122. Li H, Fu X, Ouyang Y, Cai C, Wang J, Sun T. Adult bone-marrow-derived mesenchymal stem cells contribute to wound healing of skin appendages. Cell Tissue Res. (2006) 326:725–36. doi: 10.1007/s00441-006-0270-9
123. Huang S, Lu G, Wu Y, Jirigala E, Xu Y, Ma K, et al. Mesenchymal stem cells delivered in a microsphere-based engineered skin contribute to cutaneous wound healing and sweat gland repair. J Dermatol Sci. (2012) 66:29–36. doi: 10.1016/j.jdermsci.2012.02.002
124. Bucala R, Spiegel LA, Chesney J, Hogan M, Cerami A. Circulating fibrocytes define a new leukocyte subpopulation that mediates tissue repair. Mol Med. (1994) 1:71–81. doi: 10.1007/BF03403533
125. Chesney J, Bacher M, Bender A, Bucala R. The peripheral blood fibrocyte is a potent antigen-presenting cell capable of priming naive T cells in situ. Proc Natl Acad Sci USA. (1997) 94:6307–12. doi: 10.1073/pnas.94.12.6307
126. Grab DJ, Lanners HN, Martin LN, Chesney J, Cai C, Adkisson HD, et al. Interaction of Borrelia burgdorferi with peripheral blood fibrocytes, antigen-presenting cells with the potential for connective tissue targeting. Mol Med. (1999) 5:46–54. doi: 10.1007/BF03402138
127. Chesney J, Metz C, Stavitsky AB, Bacher M, Bucala R. Regulated production of type I collagen and inflammatory cytokines by peripheral blood fibrocytes. J Immunol. (1998) 160:419–25.
128. Abe R, Donnelly SC, Peng T, Bucala R, Metz CN. Peripheral blood fibrocytes: differentiation pathway and migration to wound sites. J Immunol. (2001) 166:7556–62. doi: 10.4049/jimmunol.166.12.7556
129. Yang L, Scott PG, Giuffre J, Shankowsky HA, Ghahary A, Tredget EE. Peripheral blood fibrocytes from burn patients: identification and quantification of fibrocytes in adherent cells cultured from peripheral blood mononuclear cells. Lab Invest. (2002) 82:1183–92. doi: 10.1097/01.LAB.0000027841.50269.61
130. García-de-Alba C, Becerril C, Ruiz V, González Y, Reyes S, García-Alvarez J, et al. Expression of matrix metalloproteases by fibrocytes: possible role in migration and homing. Am J Respir Crit Care Med. (2010) 182:1144–52. doi: 10.1164/rccm.201001-0028OC
131. Hartlapp I, Abe R, Saeed RW, Peng T, Voelter W, Bucala R, et al. Fibrocytes induce an angiogenic phenotype in cultured endothelial cells and promote angiogenesis in vivo. FASEB J. (2001) 15:2215–24. doi: 10.1096/fj.01-0049com
132. Pilling D, Buckley CD, Salmon M, Gomer RH. Inhibition of fibrocyte differentiation by serum amyloid P. J Immunol. (2003) 171:5537–46. doi: 10.4049/jimmunol.171.10.5537
133. Pilling D. Aggregated IgG inhibits the differentiation of human fibrocytes. J Leukoc Biol. (2006) 79:1242–51. doi: 10.1189/jlb.0805456
134. Wang JF, Jiao H, Stewart TL, Shankowsky HA, Scott PG, Tredget EE. Fibrocytes from burn patients regulate the activities of fibroblasts. Wound Repair Regenerat. (2007) 15:113–21. doi: 10.1111/j.1524-475X.2006.00192.x
135. Ishida Y, Kimura A, Kondo T, Hayashi T, Ueno M, Takakura N, et al. Essential roles of the CC chemokine ligand 3-CC chemokine receptor 5 axis in bleomycin-induced pulmonary fibrosis through regulation of macrophage and fibrocyte infiltration. Am J Pathol. (2007) 170:843–54. doi: 10.2353/ajpath.2007.051213
136. Ishida Y, Kimura A, Nosaka M, Kuninaka Y, Hemmi H, Sasaki I, et al. Essential involvement of the CX3CL1-CX3CR1 axis in bleomycin-induced pulmonary fibrosis via regulation of fibrocyte and M2 macrophage migration. Sci Rep. (2017) 7:16833. doi: 10.1038/s41598-017-17007-8
137. Ishida Y, Kimura A, Takayasu T, Eisenmenger W, Kondo T. Detection of fibrocytes in human skin wounds and its application for wound age determination. Int J Legal Med. (2009) 123:299–304. doi: 10.1007/s00414-009-0320-4
138. Asahara T, Murohara T, Sullivan A, Silver M, van der Zee R, Li T, et al. Isolation of putative progenitor endothelial cells for angiogenesis. Science. (1997) 275:964–7. doi: 10.1126/science.275.5302.964
139. Asahara T, Takahashi T, Masuda H, Kalka C, Chen D, Iwaguro H, et al. VEGF contributes to postnatal neovascularization by mobilizing bone marrow-derived endothelial progenitor cells. EMBO J. (1999) 18:3964–72. doi: 10.1093/emboj/18.14.3964
140. Mukai N, Akahori T, Komaki M, Li Q, Kanayasu-Toyoda T, Ishii-Watabe A, et al. A comparison of the tube forming potentials of early and late endothelial progenitor cells. Exp Cell Res. (2008) 314:430–40. doi: 10.1016/j.yexcr.2007.11.016
141. Yoder MC, Mead LE, Prater D, Krier TR, Mroueh KN, Li F, et al. Redefining endothelial progenitor cells via clonal analysis and hematopoietic stem/progenitor cell principals. Blood. (2007) 109:1801–9. doi: 10.1182/blood-2006-08-043471
142. Watt SM, Athanassopoulos A, Harris AL, Tsaknakis G. Human endothelial stem/progenitor cells, angiogenic factors and vascular repair. J R Soc Interface. (2010) 7(Suppl. 6):S731–51. doi: 10.1098/rsif.2010.0377.focus
143. Hristov M, Weber C. Endothelial progenitor cells: characterization, pathophysiology, and possible clinical relevance. J Cell Mol Med. (2004) 8:498–508. doi: 10.1111/j.1582-4934.2004.tb00474.x
144. Heissig B, Hattori K, Dias S, Friedrich M, Ferris B, Hackett NR, et al. Recruitment of stem and progenitor cells from the bone marrow niche requires MMP-9 mediated release of Kit-ligand. Cell. (2002) 109:625–37. doi: 10.1016/S0092-8674(02)00754-7
145. Ceradini DJ, Kulkarni AR, Callaghan MJ, Tepper OM, Bastidas N, Kleinman ME, et al. Progenitor cell trafficking is regulated by hypoxic gradients through HIF-1 induction of SDF-1. Nat Med. (2004) 10:858–64. doi: 10.1038/nm1075
146. Capla JM, Grogan RH, Callaghan MJ, Galiano RD, Tepper OM, Ceradini DJ, et al. Diabetes impairs endothelial progenitor cell-mediated blood vessel formation in response to hypoxia. Plastic Reconstruct Surg. (2007) 119:59–70. doi: 10.1097/01.prs.0000244830.16906.3f
147. Grenier G, Scimè A, le Grand F, Asakura A, Perez-Iratxeta C, Andrade-Navarro MA, et al. Resident endothelial precursors in muscle, adipose, and dermis contribute to postnatal vasculogenesis. Stem Cells. (2007) 25:3101–10. doi: 10.1634/stemcells.2006-0795
148. Loomans CJM, de Koning EJP, Staal FJT, Rookmaaker MB, Verseyden C, de Boer HC, et al. Endothelial progenitor cell dysfunction: a novel concept in the pathogenesis of vascular complications of type 1 diabetes. Diabetes. (2004) 53:195–9. doi: 10.2337/diabetes.53.1.195
149. Seebach C, Henrich D, Wilhelm K, Barker JH, Marzi I. Endothelial progenitor cells improve directly and indirectly early vascularization of mesenchymal stem cell-driven bone regeneration in a critical bone defect in rats. Cell Transplant. (2012) 21:1667–77. doi: 10.3727/096368912X638937
150. Forte G, Minieri M, Cossa P, Antenucci D, Sala M, Gnocchi V, et al. Hepatocyte growth factor effects on mesenchymal stem cells: proliferation, migration, and differentiation. Stem Cells. (2006) 24:23–33. doi: 10.1634/stemcells.2004-0176
151. Fiedler J, Röderer G, Günther KP, Brenner RE. BMP-2, BMP-4, and PDGF-bb stimulate chemotactic migration of primary human mesenchymal progenitor cells. J Cell Biochem. (2002) 87:305–12. doi: 10.1002/jcb.10309
152. Fiedler J, Brill C, Blum WF, Brenner RE. IGF-I and IGF-II stimulate directed cell migration of bone-marrow-derived human mesenchymal progenitor cells. Biochem Biophys Res Commun. (2006) 345:1177–83. doi: 10.1016/j.bbrc.2006.05.034
153. Kitaori T, Ito H, Schwarz EM, Tsutsumi R, Yoshitomi H, Oishi S, et al. Stromal cell-derived factor 1/CXCR4 signaling is critical for the recruitment of mesenchymal stem cells to the fracture site during skeletal repair in a mouse model. Arthritis Rheum. (2009) 60:813–23. doi: 10.1002/art.24330
154. Suh W, Kim KL, Kim J-M, Shin I-S, Lee Y-S, Lee J-Y, et al. Transplantation of endothelial progenitor cells accelerates dermal wound healing with increased recruitment of monocytes/macrophages and neovascularization. Stem Cells. (2005) 23:1571–8. doi: 10.1634/stemcells.2004-0340
155. Flisiak R, Jaroszewicz J, Łapiński TW, Flisiak I, Rogalska M, Prokopowicz D. Plasma transforming growth factor β1, metalloproteinase-1 and tissue inhibitor of metalloproteinases-1 in acute viral hepatitis type B. Regul Peptides. (2005) 131:54–8. doi: 10.1016/j.regpep.2005.06.002
156. Ishida Y, Kimura A, Kuninaka Y, Inui M, Matsushima K, Mukaida N, et al. Pivotal role of the CCL5/CCR5 interaction for recruitment of endothelial progenitor cells in mouse wound healing. J Clin Invest. (2012) 122:711–21. doi: 10.1172/JCI43027
157. Gao D, Nolan D, McDonnell K, Vahdat L, Benezra R, Altorki N, et al. Bone marrow-derived endothelial progenitor cells contribute to the angiogenic switch in tumor growth and metastatic progression. Biochim Biophys Acta Rev Cancer. (2009) 1796:33–40. doi: 10.1016/j.bbcan.2009.05.001
158. Rehman J, Li J, Orschell CM, March KL. Peripheral blood “endothelial progenitor cells” are derived from monocyte/macrophages and secrete angiogenic growth factors. Circulation. (2003) 107:1164–9 doi: 10.1161/01.CIR.0000058702.69484.A0
159. Ishida Y, Kimura A, Nosaka M, Kuninaka Y, Shimada E, Yamamoto H, et al. Detection of endothelial progenitor cells in human skin wounds and its application for wound age determination. Int J Legal Med. (2015) 129:1049–54. doi: 10.1007/s00414-015-1181-7
Keywords: bone marrow-derived cells, hematopoietic stem cells, mesenchymal stem cells, skin wound, wound healing, wound age
Citation: Ishida Y, Nosaka M and Kondo T (2022) Bone Marrow-Derived Cells and Wound Age Estimation. Front. Med. 9:822572. doi: 10.3389/fmed.2022.822572
Received: 25 November 2021; Accepted: 03 January 2022;
Published: 27 January 2022.
Edited by:
Burkhard Madea, University of Bonn, GermanyReviewed by:
Julian Prangenberg, University Hospital Bonn, GermanyStefano Bacci, University of Florence, Italy
Copyright © 2022 Ishida, Nosaka and Kondo. This is an open-access article distributed under the terms of the Creative Commons Attribution License (CC BY). The use, distribution or reproduction in other forums is permitted, provided the original author(s) and the copyright owner(s) are credited and that the original publication in this journal is cited, in accordance with accepted academic practice. No use, distribution or reproduction is permitted which does not comply with these terms.
*Correspondence: Yuko Ishida, iyuko@wakayama-med.ac.jp; orcid.org/0000-0001-6104-7599; Toshikazu Kondo, kondot@wakayama-med.ac.jp; orcid.org/0000-0002-5059-8309