A Comparative Study of the Microbiological Efficacy of Polymyxin B on Different Carbapenem-Resistant Gram-Negative Bacteria Infections
- 1Department of Pharmacy, The Second Xiangya Hospital, Central South University, Institute of Clinical Pharmacy, Central South University, Changsha, China
- 2Department of Pharmacy, Hunan Provincial Corps Hospital of Chinese People's Armed Police Force, Changsha, China
- 3Department of Pharmacy, Xiangya Hospital, Central South University, Changsha, China
- 4Department of Pharmacy, The First Hospital of Changsha, Changsha, China
Objective: The emergence of carbapenem-resistant gram-negative bacteria (CR-GNB) has brought great challenges to clinical anti-infection treatment around the world. Polymyxins are often considered as the last line of defense in the treatment of CR-GNB infections. In this study, we explored the microbiological efficacy of Polymyxin B (PMB) on different CR-GNB infections as well as the factors influencing microbiological efficacy.
Methods: CR-GNB infected patients with PMB-based regimens were enrolled. Clinical and microbiological data were collected from the medical electronic record system of the Second Xiangya hospital. The efficacy of PMB on different CR-GNB was evaluated by the clearance rate at 7-days and within the course of treatment, as well as the 30-day mortality rate.
Results: A total of 294 CR-GNB infected patients were enrolled: 154 CR-Acinetobacter baumannii (CRAB), 55 CR-Klebsiella pneumoniae (CRKP), and 85 CR-Pseudomonas aeruginosa (CRPA). The CRAB group had the highest 7-day bacterial clearance rate [(CRAB: 39.0%) vs. (CRKP: 29.4%) vs. (CRPA: 14.5%), P = 0.003] and total bacterial clearance rate [(CRAB: 49.0%) vs. (CRKP: 39.8%) vs. (CRPA: 18.2%), P < 0.001] among the three groups, while the bacterial clearance rate of the CRPA group was the lowest. Multivariate logistic regression showed that the differences among the three groups were multiple CR-GNB infections (P = 0.004), respiratory infections (P = 0.001), PMB resistance (P < 0.001), and the combination of tigecycline (P < 0.001). Binary logistic regression showed that multiple CR-GNB infection [(7-day bacterial clearance: P = 0.004) & (total bacterial clearance: P = 0.011)] and bacterial species [(7-day bacterial clearance: P < 0.001) & (total bacterial clearance: P < 0.001)] were independent risk factors for microbiological efficacy.
Conclusion: PMB exhibited differential microbiological efficacy on different types of CR-GNB infections; it had the best effect on CRAB, followed by CRKP and CRPA. Multiple CR-GNB infections and bacterial species were independent risk factors for microbiological efficacy.
Introduction
Carbapenem-resistant gram-negative bacteria (CR-GNB) infections are an urgent global public health threat with high mortality rate (1). According to the standard of Clinical and Laboratory Standards Institute (CLSI), minimum inhibitory concentrations (MICs) of both imipenem and meropenem ≥8 mg/L means carbapenem-resistance, and MIC ≥4 mg/L means intermediate susceptibility (2). However, in the new guide of the European Committee on Antimicrobial Susceptibility Testing (EUCAST), the breakpoints of imipenem and meropenem are 4 and 8mg/L, respectively (3). Carbapenem-resistant Enterobacteriaceae (CRE), Pseudomonas, and Acinetobacter species are the three most common CR-GNB (4, 5). The emergence of these bacteria has brought great challenges to clinical anti-infection treatment around the world, and rational selection of antibacterial drugs has become an important way to prevent and treat CR-GNB infections (6). There are many clinical studies and summaries regarding anti-CR-GNB drugs such as polymyxins (7), tigecycline (8), and ceftazidime-avibactam (9).
Polymyxins, a kind of polypeptide antibiotic, are often regarded as the last line of defense in the treatment of CR-GNB infections, and polymyxin B (PMB) and colistin are the two drugs under heated discussion. A system review and meta-analysis showed that being elderly, high daily dose, having underlying diseases such as diabetes, and use of concomitant nephrotoxic drugs were independent predictors of polymyxin-induced nephrotoxicity (10). A recent cohort study also showed that high-dose PMB was associated with increased nephrotoxicity (11). Therefore, therapeutic drug monitoring should be done to outweigh the potential benefits of polymyxin therapy from its risk (10).
Some studies have explored the efficacy of PMB on a specific bacterial infection of CR-GNB (either CRAB or CRKP), but no studies have compared the efficacy of PMB for three different types of CR-GNB infection. PMB has been shown to have a strong bactericidal effect on carbapenem-resistant Acinetobacter baumannii (CRAB) in vitro (12). Sun et al. indicated that even with salvage use of PMB, the tigecycline based medications did not significantly increase the 28-day mortality rate of patients infected with carbapenem-resistant Klebsiella pneumoniae (CRKP) (13). There is also evidence to suggest that early use of PMB can reduce the mortality of CRKP bloodstream infections (BSIs) (14). Moreover, the combination of PMB and imipenem presented synergistic antibacterial effects against carbapenem-resistant Pseudomonas aeruginosa (CRPA) in vitro (15). Although PMB has curative effects on many types of CR-GNB infections, its differential efficacy with respect to various bacteria has not been clearly discussed. In this study, we explored the efficacy of PMB on different CR-GNBs and factor influencing efficacy.
Patients and Methods
Ethical Approval
This research was approved by the Ethics Committees of the Second Xiangya Hospital of Central South University in Changsha, China (LYF-2020021). The implementation of this study was in line with the Declaration of Helsinki and its amendments. Informed consent was waived because this study was non-interventional in nature.
Patients
Data were collected concerning patients with PMB medication histories in the Second Xiangya Hospital of Central South University from January 1, 2018 to March 31, 2020. The inclusion criteria were as follows: (1) Patients treated with PMB (Shanghai Number 1 Biochemical & Pharmaceuticals, Shanghai, China) for CR-GNB infection; (2) The duration of PMB treatment was not <72 h; (3) CR-GNB infection was confirmed by culture results and drug sensitivity test results were available. The exclusion criteria were as follows: (1) The number of patients infected with certain types of CR-GNB was too small to perform inferential analyses; (2) Patients with malignant tumors or severe liver/kidney dysfunction before treatment; (3) Incomplete clinical data.
Collection of Clinical Data
We collected the relevant information of patients that may be related to the efficacy of PMB, including basic demographic information, medication (treatment duration, dosage, frequency, and combined drugs), diagnoses, pathogenic bacteria (species, sensitivity, and site of infection), Acute Physiology and Chronic Health Evaluation (APACHE) II scores, efficacy evaluation indices and prognoses. “Single CR-GNB” represents infection caused by one CR-GNB; “multiple CR-GNB” represents infection caused by two or more CR-GNB.
Research Design
According to the types of CR-GNB infection, the patients were divided into three groups: CRAB, CRKP, and CRPA infections. Patients infected with multiple CR-GNB before and during the treatment course were split into different cases according to the type of bacteria, and then assigned to the appropriate group. The microbiological efficacy of PMB on different bacteria was evaluated by the bacterial clearance rate after the course of treatment (total bacterial clearance rate) and the 7-day bacterial clearance rate. Mortality was defined as deaths in hospitals or the discontinuation of treatment in severe cases due to poor outcomes.
Microbiology
The sensitivity of bacteria to antibacterial drugs was tested by the broth-microdilution method using analytical instruments. MIC was determined by a VITEK®2 system (bioMérieux, Marcy-l'Étoile, France) based on the recommendations of the EUCAST. “Carbapenem resistance” was defined as the MIC of bacteria to imipenem and meropenem ≥4 mg/L. Meanwhile, according to the European Committee on Antimicrobial Susceptibility Testing (EUCAST, v8.0, 2018), “MIC >2 mg/L” represented bacterial resistance to tigecycline and PMB (16).
Statistical Analysis
Before the start of the study, the appropriate sample size was estimated by the following equation: N = Z2P (1 – P)/E2 (Z = 1.96; P = 0.5; E = 10%), and the result showed that the minimum sample size is 96. Data were analyzed using SPSS v21.0 (IBM, Armonk, NY, USA). Data conforming to the normal distribution were analyzed by t-tests or analysis of variance (ANOVA) and expressed as mean ± standard deviation. Non-normally distributed data were analyzed by nonparametric tests and expressed by median and interquartile range (IQR). Count data were analyzed by the chi-square tests and expressed by quantity and percentage. In multiple groups analysis, measurement data were analyzed by one-way analysis of variance (ANOVA) or non-parametric test, and the post-hoc test (LSD) was further used for pairwise comparisons; count data were analyzed by chi-square test for pairwise comparisons. The binary logistic regression used the entry method, and P < 0.05 was considered statistically significant. In addition, multiple logistic regression was used to analyze data with multiple endpoints. Cox-regression analysis was applied to compare the 30-day mortality of the three types of CR-GNB infected patients and influencing factors, and P < 0.05 was considered statistically significant.
Results
Characteristics
According to the inclusion and exclusion criteria, 294 CR-GNB infected patients were enrolled: 154 (52.4%) CRAB strains, 55 (18.4%) CRKP strains, and 85 CRPA strains. Nine patients were infected with carbapenem-resistant Escherichia coli and Enterobacter cloacae, and were excluded due to insufficient numbers. The clinical characteristics are shown in Table 1. The average age of enrolled patients was 57.32 ± 19.29 years and the majority (72.4%) were male. Patients with mechanical ventilation and vasoactive agents accounted for 73.1 and 52.7% of the sample, respectively. The ICU admission rate was 71.4%. The most common infection site was the respiratory tract (88.4%), and 86.0% of patients suffered from the respiratory system diseases. The average dose of PMB was 0.86 (0.83–1.00) mg/kg/q12h and the average course of treatment was 11.75 (7.50–15.88) days. 5.1% of pathogenic bacteria were PMB resistant, and the total bacterial clearance rate was 40.4%, while the 7-day bacterial clearance rate was 31.6%. Tigecycline was the most frequently used drug in the combination with PMB (33.4%). 28.2% of patients died in hospital or discontinued treatment due to poor efficacy.
Microbiological Efficacy in Different CR-GNB Infection Contexts
We compared the characteristics and clinical parameters among the three groups. The results showed that patients in the CRPA group had a higher average age than the other two groups [(CRAB: 56.83 ± 19.60 years) vs. (CRKP: 54.09 ± 19.93 years) vs. (CRPA: 63.67 ± 15.94 years), P = 0.014]. In the CRKP group, the proportions of patients who were male [(CRAB: 77.3%) vs. (CRKP: 62.4%) vs. (CRPA: 74.5%), P = 0.044] and exhibited respiratory tract infection [(CRAB: 96.8%) vs. (CRKP: 70.6%) vs. (CRPA: 92.7%), P < 0.001] were lower than the other two groups.
There were statistically significant differences in the resistance of the three bacteria to antimicrobial drugs: CRPA was naturally resistant to tigecycline, while CRAB was more resistant to tigecycline than CRKP [(CRAB: 94.7%) vs. (CRKP: 84.1%), P = 0.007]. In addition, CRPA was more resistant to PMB (P < 0.001). Furthermore, compared with the other two groups, tigecycline was used less frequently to treat CRPA infection [(CRAB: 33.4%) vs. (CRKP: 44.0%) vs. (CRPA: 14.5%), P = 0.001]. More CRPA infections were accompanied by other CR-GNB [(CRAB: 33.1%) vs. (CRKP: 44.7%) vs. (CRPA: 54.5%), P = 0.013].
The CRAB group had the highest 7-day bacterial clearance rate [(CRAB: 39.0%) vs. (CRKP: 29.4%) vs. (CRPA: 14.5%), P = 0.003] and total bacterial clearance rate [(CRAB: 49.0%) vs. (CRKP: 39.8%) vs. (CRPA: 18.2%), P < 0.001] among the three groups, while the bacterial clearance rate of the CRPA group was the lowest. There was no statistical difference among the three groups in terms of treatment duration and mortality rate (Table 2 and Figure 1A). Next, we performed multiple logistic regression on the three CR-GNB in order to control for the interference of confounding factors, and the results showed that the differences among them were multiple CR-GNB infection (P = 0.004), respiratory infection (P = 0.001), PMB resistance (P < 0.001), and the combination of tigecycline (P < 0.001) (Table 3 and Figures 1B–D).
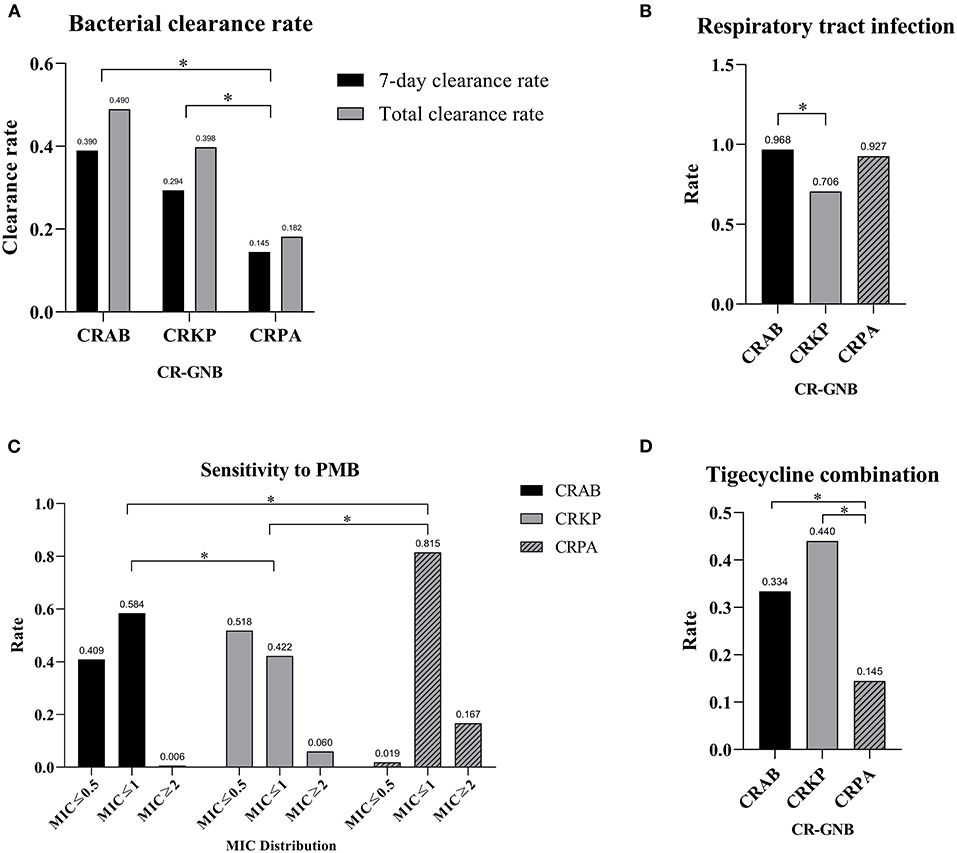
Figure 1. Divergences among different CR-GNB groups. (A) Divergence in clearance rates. (B) Divergence in respiratory tract infection rates. (C) Divergence in PMB resistance rates. (D) Divergence in the tigecycline combination rate. *p < 0.05.
Factors Influencing Microbiological Efficacy
The above results indicated that age, gender, multiple CR-GNB infection, respiratory tract infection, sensitivity to PMB, and the combination of tigecycline may be associated with the different clearance rates for different CR-GNB. However, whether these factors can cause differences in bacterial clearance rates still needs to be verified. Therefore, we performed a binary logistic regression and found that multiple CR-GNB infection [(7-day bacterial clearance: P = 0.004) & (total bacterial clearance: P = 0.011)] and bacterial species [(7-day bacterial clearance: P < 0.001) & (total bacterial clearance: P < 0.001)] were independent risk factors for bacterial clearance (Table 4).
In order to clarify the effect of multiple CR-GNB infection on bacterial clearance, we divided the cases into two groups “Single CR-GNB” and “Multiple CR-GNB,” and compared the treatment information and outcomes of the two groups. The results showed that the medication information between the two groups was well-matched, and cases of multiple CR-GNB infection did not cause a statistical difference in clearance rate (Supplementary Table 1). Furthermore, we compared the clinical data of patients with and without bacterial clearance at 7 days and at the end of the PMB treatment course. The results showed that the above factors did not significantly affect the respective clearance rates (Supplementary Table 2).
Cox-Regression Survival Analysis for 30-Day Mortality
Finally, in order to compare the 30-day mortality rate and survival time among the three CR-GNB groups, we performed Cox-regression survival analysis and found that none of the above factors significantly affected the 30-day mortality or survival time statistically (Table 5 and Figure 2).
Discussion
CR-GNB infections pose great challenges to clinical treatment (17). PMB is an important drug for the treatment of CR-GNB infections, but its microbiological efficacy against different pathogens still needs to be elucidated. In this study, we explored the microbiological efficacy of PMB on CRAB, CRKP, and CRPA. We also explored the factors influencing PMB microbiological efficacy and 30-days mortality. The results showed that PMB has different microbiological efficacy among CR-GNBs, with the best in CRAB, followed by CRKP and CRPA. Moreover, multivariate logistic regression showed that the differences among the three groups were multiple CR-GNB infection, respiratory infection, PMB resistance, and the combination of tigecycline. Multiple CR-GNB infection and bacterial species may be risk factors for the microbiological efficacy of PMB-based regimens.
We found that it was easier to eliminate CRAB by PMB compared to CRKP and CRPA. Previous studies have also shown that PMB has good efficacy on respiratory tract infections caused by Acinetobacter baumannii, Pseudomonas aeruginosa, and multidrug-resistant Gram-negative bacteria (18). Another study which carried out a Cox regression with 30-day mortality as the endpoint showed that the PMB-based regimen is beneficial to lower respiratory tract infections and Acinetobacter baumannii infections compared with Pseudomonas aeruginosa infections, which is consistent with our results (19). In terms of PMB combinations, a checkerboard assay study found that PMB plus sulbactam exhibited the highest synergistic effect at a rate of 82.35% in CRAB infections (20). Further research is needed to explore which combinations are the most effective against CRAB infection.
In our study, CRPA was naturally resistant to tigecycline and exhibited the highest resistance to PMB, which may be attributed to the production of broad-spectrum β-lactamase and metallo-β-lactamase (21). CRPA is inherently resistant to a variety of antibacterial drugs; it can develop resistance easily through multiple mechanisms (22). The PMB resistance rate is <5% globally, but it is increasing gradually and even approaches 50% in Singapore (23). These characteristics make it difficult for PMB to clear CRPA successfully (17). Because CRPA is naturally resistant to tigecycline, combining tigecycline, and PMB in this context exhibited the lowest efficacy; therefore, the purpose of such a combination may be to treat coexisting CR-GNB infections. Since PMB monotherapy commonly leads to the occurrence of drug resistance, it is necessary to select the appropriate combination drugs. Studies have shown that PMB combined with tigecycline shows promising rapid and long-lasting bactericidal effects in CRAB and CRE including CRKP, both in vivo and in vitro (24–26). Treatment with 100 mg PMB q12h plus 200 mg tigecycline q12h significantly reduced bacterial density (27). Finding ways to improve the clearance rate and efficacy of CRPA infection is an urgent clinical problem. An in vitro study indicated that the combination of PMB and enrofloxacin can work synergistically against CRPA (28). Moreover, PMB and usnic acid have also been shown to exhibit a synergistic effect against CRPA infection (29). These existing findings may provide an important benchmark for finding more effective PMB combinations.
A single-center retrospective study showed that receiving PMB-based therapy provided a survival benefit compared with tigecycline-based therapy in the context of CRKP bloodstream infection (30). Time-lapse microscopy and time-kill experiments evaluated PMB in combination with 13 other antibiotics against CRKP and found that PMB in combination with minocycline, rifampicin, or fosfomycin could be of potential clinical interest (31). The 7-days bacterial clearance rate and total bacterial clearance of CRKP in our study were 29.4 and 39.8%, respectively; the microbiological efficacy of PMB in CRKP is lower than that in CRAB and higher than that in CRPA.
In vitro and in vivo experiments of different types of CR-GNB with different combinations based on PMB are worthy of further study. Different combination regimens may have different clinical and microbial effects on different bacteria. Therefore, joint drug sensitivity experiments are a useful tool for investigating the combined bactericidal effects of various PMB combined regimens in vitro. Because of our limited sample size, we did not carry out combination regimens subgroups analyses. Other research has investigated this important issue (32, 33). One study evaluated the activity of ceftazidime-avibactam and PMB in combination against CRKP in a tandem in vitro time-kill/in vivo Galleria mellonella survival model assay and found no improvement in in vitro bactericidal activity or in vivo efficacy using this combination (33). Another investigation focused on 82 KPC-KP BSIs and found that PMB plus amikacin showed a survival benefit compared with other regimens (32). Moreover, with the development of new technologies, methods such as machine learning brings forth a possible avenue to optimize treatment regimens beyond the use of the “traditional” indices of antibiotic action (34). This methodology leverages in vitro experimental data, a mathematical pharmacodynamic model, and population pharmacokinetics to optimize antibiotic combinations (34). For precise treatment, therapeutic drug monitoring (TDM) guided medication of PMB has also been used in the clinical practice to ensure efficiency and safety (35).
Beyond the individual types of CR-GNB, multiple CR-GNB infection and bacterial species may be risk factors for microbiological efficacy of PMB-based regimens. While in the separate analysis of CR-GNB and the binary logistic regression analysis, no exact factors were found that may lead to different clearance rates, and the divergences in clearance rates failed to lead to differences in survival time according to the results from Cox regression. These results also suggested that there may be internal factors associated with different CR-GNB that make clearance difficult.
There were some limitations to our study. First, this single center retrospective study with a limited sample size needs more cases to drawn robust conclusions. Second, the clinical data on patients were not detailed enough, and the dosage information of combined drugs was not comprehensive. In addition, the number of CR-GNB subgroups is small.
In conclusion, our study firstly explored the microbiological efficacy of PMB on different CR-GNB infections and the factors influencing microbiological efficacy. The results showed that PMB was an effective antibiotic in the treatment of CR-GNB infection, with differential efficacy depending on the type of CR-GNB. The best effect occurred with respect to CRAB, followed by CRKP and CRPA. Multiple CR-GNB infection and bacterial species were independent risk factors for microbiological efficacy. Large-sample multicenter studies are needed to find precise strategies to optimize the microbiological efficacy of PMB on CR-GNB.
Data Availability Statement
The original contributions presented in the study are included in the article/Supplementary Material, further inquiries can be directed to the corresponding author.
Ethics Statement
The studies involving human participants were reviewed and approved by the Ethics Committees of the Second Xiangya Hospital of Central South University in Changsha, China (LYF-2020021). Written informed consent for participation was not required for this study in accordance with the national legislation and the institutional requirements.
Author Contributions
JQ and QL conceptualized and designed the study. H-HZ, G-HL, T-TQ, L-JY, X-QT, QQ, and G-FH collected data. G-HL and T-TQ analyzed the data. G-HL, JQ, and QL drafted the manuscript. H-HZ modified relevant contents including language and initial draft responding to reviewers. All authors contributed to the revision and approved the final version of the manuscript.
Funding
This work was supported by the National Natural Science Foundation of China (No. 81503166), Hunan Provincial Natural Science Foundation of China (2017JJ2285, 2018JJ3718), WU JIEPING Medical Foundation (320.6750.19090-11), and the Hunan Provincial Department of Finance Grant (No. 2019-93, No. 2018-92).
Conflict of Interest
The authors declare that the research was conducted in the absence of any commercial or financial relationships that could be construed as a potential conflict of interest.
Supplementary Material
The Supplementary Material for this article can be found online at: https://www.frontiersin.org/articles/10.3389/fmed.2021.620885/full#supplementary-material
References
1. Lalaoui R, Javelle E, Bakour S, Ubeda C, Rolain JM. Infections due to carbapenem-resistant bacteria in patients with hematologic malignancies. Front. Microbiol. (2020) 11:1422. doi: 10.3389/fmicb.2020.01422
2. CLSI. Performance Standards for Antimicrobial Susceptibility Testing; 27th Informational Supplement. CLSI Document M100-S27. Wayne, PA: Clinical and Laboratory Standards Institute (2017).
3. EUCAST. Clinical Breakpoints - Bacteria (v 11.0) Consultation 4 Dec - 18 Dec, 2020 (2020). Available online at: https://www.eucast.org/clinical_breakpoints/ (accessed January 25, 2020).
4. Carbapenem-resistant organisms (CRO): information for healthcare workers. J. Hosp. Infect. (2016) 92:99–101. doi: 10.1016/j.jhin.2015.08.012
5. Pannala R, Baldwin B, Aluru V, Grys TE, Holmes J, Miller LJ, et al. Prospective study of the feasibility of point-of-care testing strategy for carbapenem-resistant organism detection. Endosc. Int. Open. (2018) 6:E58–63. doi: 10.1055/s-0043-122141
6. Wilson AP, Livermore DM, Otter JA, Warren RE, Jenks P, Enoch DA, et al. Prevention and control of multi-drug-resistant gram-negative bacteria: recommendations from a joint working party. J. Hosp. Infect. (2016) 92(Suppl. 1):S1–44. doi: 10.1016/j.jhin.2015.08.007
7. Thomas R, Velaphi S, Ellis S, Walker AS, Standing JF, Heath P, et al. The use of polymyxins to treat carbapenem resistant infections in neonates and children. Expert Opin. Pharmacother. (2019) 20:415–22. doi: 10.1080/14656566.2018.1559817
8. Shankar C, Nabarro LEB, Anandan S, Veeraraghavan B. Minocycline and tigecycline: what is their role in the treatment of carbapenem-resistant gram-negative organisms? Microb. Drug Resist. (2017) 23:437–46. doi: 10.1089/mdr.2016.0043
9. Temkin E, Torre-Cisneros J, Beovic B, Benito N, Giannella M, Gilarranz R, et al. Ceftazidime-avibactam as salvage therapy for infections caused by carbapenem-resistant organisms. Antimicrob. Agents Chemother. (2017) 61:e01964-16. doi: 10.1128/AAC.01964-16
10. Sisay M, Hagos B, Edessa D, Tadiwos Y, Mekuria AN. Polymyxin-induced nephrotoxicity and its predictors: a systematic review and meta-analysis of studies conducted using RIFLE criteria of acute kidney injury. Pharmacol. Res. (2020) 163:105328. doi: 10.1016/j.phrs.2020.105328
11. Cai Y, Leck H, Tan RW, Teo JQ, Lim TP, Lee W, et al. Clinical experience with high-dose polymyxin B against carbapenem-resistant gram-negative bacterial infections-a cohort study. Antibiotics. (2020) 9:451. doi: 10.3390/antibiotics9080451
12. Thamlikitkul V, Tiengrim S, Seenama C. In vitro activity of polymyxin B against carbapenem-resistant Acinetobacter baumannii. J. Med. Assoc. Thai. (2014) 97:1254–8. doi: 10.1016/j.ijantimicag.2013.05.014
13. Sun WM, Zhou H, Shen LS, Yang Q, Ma WJ, Zhou JY. The efficacy and safety of different antimicrobial regimens in carbapenem-resistant Klebsiella pneumoniae bloodstream infections. Zhonghua Nei Ke Za Zhi. (2019) 58:566–71. doi: 10.3760/cma.j.issn.0578-1426.2019.08.004
14. Liang Q, Huang M, Xu Z. Early use of polymyxin B reduces the mortality of carbapenem-resistant Klebsiella pneumoniae bloodstream infection. Braz. J. Infect. Dis. (2019) 23:60–5. doi: 10.1016/j.bjid.2018.12.004
15. Wilhelm CM, Nunes LS, Martins AF, Barth AL. In vitro antimicrobial activity of imipenem plus amikacin or polymyxin B against carbapenem-resistant Pseudomonas aeruginosa isolates. Diagn. Microbiol. Infect. Dis. (2018) 92:152–4. doi: 10.1016/j.diagmicrobio.2018.05.004
16. Dalyan CB, Topac T, Agca H, Saglam S, Efe K, Ener B. Comparison of Clinical Laboratory Standards Institute (CLSI) and European Committee on Antimicrobial Susceptibility Testing (EUCAST) broth microdilution methods for determining the susceptibilities of Candida isolates. Mikrobiyol. Bul. (2018) 52:35–48. doi: 10.5578/mb.63991
17. Dubrovskaya Y, Chen TY, Scipione MR, Esaian D, Phillips MS, Papadopoulos J, et al. Risk factors for treatment failure of polymyxin B monotherapy for carbapenem-resistant Klebsiella pneumoniae infections. Antimicrob. Agents Chemother. (2013) 57:5394–7. doi: 10.1128/AAC.00510-13
18. Sobieszczyk ME, Furuya EY, Hay CM, Pancholi P, Della-Latta P, Hammer SM, et al. Combination therapy with polymyxin B for the treatment of multidrug-resistant Gram-negative respiratory tract infections. J. Antimicrob. Chemother. (2004) 54:566–9. doi: 10.1093/jac/dkh369
19. Rigatto MH, Vieira FJ, Antochevis LC, Behle TF, Lopes NT, Zavascki AP. Polymyxin B in combination with antimicrobials lacking in vitro activity versus polymyxin B in monotherapy in critically Ill patients with Acinetobacter baumannii or Pseudomonas aeruginosa infections. Antimicrob. Agents Chemother. 59:6575–80. doi: 10.1128/AAC.00494-15
20. Qu J, Yu R, Wang Q, Feng C, Lv X. Synergistic antibacterial activity of combined antimicrobials and the clinical outcome of patients with carbapenemase-producing Acinetobacter baumannii Infection. Front. Microbiol. (2020) 11:541423. doi: 10.3389/fmicb.2020.541423
21. Farhan SM, Ibrahim RA, Mahran KM, Hetta HF, Abd El-Baky RM. Antimicrobial resistance pattern and molecular genetic distribution of metallo-β-lactamases producing Pseudomonas aeruginosa isolated from hospitals in Minia, Egypt. Infect. Drug Resist. (2019) 12:2125–33. doi: 10.2147/IDR.S198373
22. Potron A, Poirel L, Nordmann P. Emerging broad-spectrum resistance in Pseudomonas aeruginosa and Acinetobacter baumannii: mechanisms and epidemiology. Int. J. Antimicrob. Agents. (2015) 45:568–85. doi: 10.1016/j.ijantimicag.2015.03.001
23. Lim TP, Lee W, Tan TY, Sasikala S, Teo J, Hsu LY, et al. Effective antibiotics in combination against extreme drug-resistant Pseudomonas aeruginosa with decreased susceptibility to polymyxin B. PLoS ONE. (2011) 6:e28177. doi: 10.1371/journal.pone.0028177
24. Lim TP, Tan TY, Lee W, Sasikala S, Tan TT, Hsu LY, et al. In-vitro activity of polymyxin B, rifampicin, tigecycline alone and in combination against carbapenem-resistant Acinetobacter baumannii in Singapore. PLoS ONE. (2011) 6:e18485. doi: 10.1371/journal.pone.0018485
25. Cai Y, Lim TP, Teo JQ, Sasikala S, Chan EC, Hong YJ, et al. Evaluating polymyxin B-based combinations against carbapenem-resistant Escherichia coli in time-kill studies and in a hollow-fiber infection model. Antimicrob. Agents Chemother. (2017) 61:e01509-16. doi: 10.1128/AAC.01509-16
26. Alves PH, Boff RT, Barth AL, Martins AF. Synergy of polymyxin B, tigecycline and meropenem against carbapenem-resistant Enterobacter cloacae complex isolates. Diagn. Microbiol. Infect. Dis. (2019) 94:81–5. doi: 10.1016/j.diagmicrobio.2018.11.011
27. Hagihara M, Housman ST, Nicolau DP, Kuti JL. In vitro pharmacodynamics of polymyxin B and tigecycline alone and in combination against carbapenem-resistant Acinetobacter baumannii. Antimicrob. Agents Chemother. (2014) 58:874–9. doi: 10.1128/AAC.01624-13
28. Lin YW, Han ML, Zhao J, Zhu Y, Rao G, Forrest A, et al. Synergistic combination of polymyxin B and enrofloxacin induced metabolic perturbations in extensive drug-resistant Pseudomonas aeruginosa. Front. Pharmacol. (2019) 10:1146. doi: 10.3389/fphar.2019.01146
29. da Costa Junior SD, da Silva WRC, da Silva A, Maciel MAV, Cavalcanti IMF. Synergistic effect between usnic acid and polymyxin B against resistant clinical isolates of Pseudomonas aeruginosa. Evid. Based Complement Alternat. Med. (2020) 2020:9852145. doi: 10.1155/2020/9852145
30. Shen L, Lian C, Zhu B, Yao Y, Yang Q, Zhou J, et al. Bloodstream Infections due to carbapenem-resistant klebsiella pneumoniae: a single-center retrospective study on risk factors and therapy options. Microb. Drug Resist. (2020). doi: 10.1089/mdr.2019.0455. [Epub ahead of print].
31. Wistrand-Yuen P, Olsson A, Skarp KP, Friberg LE, Nielsen EI, Lagerback P, et al. Evaluation of polymyxin B in combination with 13 other antibiotics against carbapenemase-producing Klebsiella pneumoniae in time-lapse microscopy and time-kill experiments. Clin. Microbiol. Infect. (2020) 26:1214–21. doi: 10.1016/j.cmi.2020.03.007
32. Medeiros GS, Rigatto MH, Falci DR, Zavascki AP. Combination therapy with polymyxin B for carbapenemase-producing Klebsiella pneumoniae bloodstream infection. Int. J. Antimicrob. Agents. (2019) 53:152–7. doi: 10.1016/j.ijantimicag.2018.10.010
33. Borjan J, Meyer KA, Shields RK, Wenzler E. Activity of ceftazidime-avibactam alone and in combination with polymyxin B against carbapenem-resistant Klebsiella pneumoniae in a tandem in vitro time-kill/in vivo Galleria mellonella survival model analysis. Int. J. Antimicrob. Agents. (2020) 55:105852. doi: 10.1016/j.ijantimicag.2019.11.009
34. Smith NM, Lenhard JR, Boissonneault KR, Landersdorfer CB, Bulitta JB, Holden PN, et al. Using machine learning to optimize antibiotic combinations: dosing strategies for meropenem and polymyxin B against carbapenem-resistant Acinetobacter baumannii. Clin. Microbiol. Infect. (2020) 26:1207–13. doi: 10.1016/j.cmi.2020.02.004
Keywords: polymyxin B, carbapenem-resistant organisms, tigecycline, microbiological efficacy, bacteria clearance
Citation: Lu Q, Zhu H-H, Li G-H, Qi T-T, Ye L-J, Teng X-Q, Qu Q, He G-F and Qu J (2021) A Comparative Study of the Microbiological Efficacy of Polymyxin B on Different Carbapenem-Resistant Gram-Negative Bacteria Infections. Front. Med. 8:620885. doi: 10.3389/fmed.2021.620885
Received: 10 November 2020; Accepted: 15 January 2021;
Published: 09 February 2021.
Edited by:
Marwan Osman, Lebanese University, LebanonReviewed by:
Fatima Bachir Halimeh, Lebanese University, LebanonMohsin Khurshid, Government College University, Faisalabad, Pakistan
Yan Zhu, Monash University, Australia
Copyright © 2021 Lu, Zhu, Li, Qi, Ye, Teng, Qu, He and Qu. This is an open-access article distributed under the terms of the Creative Commons Attribution License (CC BY). The use, distribution or reproduction in other forums is permitted, provided the original author(s) and the copyright owner(s) are credited and that the original publication in this journal is cited, in accordance with accepted academic practice. No use, distribution or reproduction is permitted which does not comply with these terms.
*Correspondence: Jian Qu, qujianstanley@csu.edu.cn