Feeding ecology of Antarctic toothfish, Dissostichus mawsoni in the subarea 88.3 (Bellingshausen Sea and eastern Amundsen Sea) of the Southern Ocean
- 1Department of Marine Biology & Aquaculture/Department of Aquaculture Science/Institute of Marine Industry, College of Marine Science, Gyeongsang National University, Tongyeong, Republic of Korea
- 2Distant Water Fisheries Resources Research Division, National Institute of Fisheries Science, Busan, Republic of Korea
Understanding feeding ecology is essential for ecosystem-based management. As dietary data can show differences depending on the spatiotemporal and ontogenetic aspects of the sample, the results should be derived based on various size classes and samples over a wide spatiotemporal range. Morphological analysis of the stomach contents of Dissostichus mawsoni, a piscivore, collected at depths of 603–2,113 m in subarea 88.3 during the Antarctic summer fishing seasons from 2016 to 2020 was used to assess the dietary composition according to the research block, fishing season, depth, and size. Here, we used 1,639 D. mawsoni specimens with a total length range of 44.5–189.0 cm. The dietary composition of D. mawsoni did not show significant differences by fishing season but differed with research block, depth, and size. Macrouridae dominated research blocks 88.3_1 and 88.3_3; Nototheniidae dominated research blocks 88.3_2 and 88.3_6; and Channichthyidae dominated research blocks 88.3_4 and 88.3_5. The proportion of Nototheniidae decreased as depth increased, whereas the consumption of Channichthyidae increased gradually with depth in research blocks 88.3_3 and 88.3_4. The proportion of Channichthyidae decreased as body size increased, whereas the consumption of Macrouridae increased gradually with body size in both blocks 88.3_3 and 88.3_4. During the study period, the dietary composition of D. mawsoni in both blocks 88.3_3 and 88.3_4 did not show significant temporal differences, suggesting that these data could be used as a baseline for identifying future environmental changes in the region. This study provides information on the relationships between various species and benthic fish fauna, which are essential for ecosystem-based management.
1 Introduction
Dissostichus spp. are commercially valuable marine fish. Dissostichus mawsoni is mainly distributed south of the polar front. Antifreeze proteins allow them to survive low water temperatures. Their range differs from Dissostichus eleginoides, with some exceptions (Gon and Heemstra, 1990; Eastman and Hubold, 1999; Goldsworthy et al., 2002). Dissostichus mawsoni primarily feeds on fish and cephalopods. They represent a high ecological niche as apex predators and scavengers and serve as food sources for seals, cetaceans, and giant squids (Fenaughty et al., 2003; Stevens et al., 2014). Furthermore, based on ontogenetic changes, this species inhabits shallow waters during the early stages of growth and then moves to deeper waters up to 2,200 m during the adult stage (Hanchet et al., 2015); thus, its ecological role in the Southern Ocean ecosystem is critical throughout its life cycle (Mormede et al., 2014). However, there are uncertainties of information on the biology and life cycle of D. mawsoni has been primarily obtained from fishery samples (Mormede et al., 2014; Ainley et al., 2016).
The geographical distance and inaccessible natural environment of the Southern Ocean make it challenging to systematically study species richness and biomass within its waters (Caccavo et al., 2021). Antarctic toothfish fisheries occur only in convention areas of the Commission for the Conservation of Antarctic Marine Living Resources (CCAMLR) and are managed through ecosystem-based resource management and a precautionary approach. Most of the data used were collected by scientific observers onboard vessels, and sampling followed strict regulations of the CCAMLR (e.g., random sampling). Antarctic toothfish catches averaged 4,157 metric tons per year during the 2011–2020 fishing season, with the highest average being 2,871 metric tons per year in subarea 88.1 (Ross Sea). The study area, subarea 88.3, is a CCAMLR convention area located in the Bellingshausen Sea and eastern Amundsen Sea. The Antarctic toothfish fishery in subarea 88.3 began in 1998 and was conducted intermittently until 2012, when exploratory fisheries were reinstated in 2016 to obtain information on biological and ecological species relationships for ecosystem-based management, with an average annual catch of 85 metric tons by 2020. There is also a lack of information on the environmental characteristics, species composition of the ecosystem, and trophic interactions of Antarctic toothfish in subarea 88.3.
Traditional single-species management methods must be reconsidered as the sole methods for preserving and managing fishery resources worldwide. Recently, an ecosystem-based resource management method has been developed that considers the relationships between various species as well as the interactions between target species and the environment (Slocombe, 1993; Berkes, 2012; Kadin et al., 2019; Cucuzza et al., 2021). A precautionary approach is used for risk management to address situations with incomplete data (Karim et al., 2020). Feeding ecology studies can help identify predators-prey relationships within ecosystems, assess the ecological status and role of target species, and predict how environmental changes caused by fishing and climate change affect ecosystems (Link, 2002; Roessig et al., 2004; Melnychuk et al., 2017). Such studies can also estimate the biomass and abundance of prey species (Sallaberry-Pincheira et al., 2018; Ng et al., 2021). Similarly, it is important to understand the function of the target species in the food web structure through dietary studies, such as exploring the effects of population structure changes and population decline of prey species through fisheries. However, diet composition data can differ depending on the temporal and spatial factors related to the sample and size class (Boyd, 1996; Holt et al., 2019). Diet composition studies can be conducted using various size classes and samples with a wide spatiotemporal range, which helps mitigate limitations, and specific trophic interactions within the target area can be better understood. A long-term dietary study of D. mawsoni can reveal its specific ecosystem functions in the Southern Ocean. Furthermore, it can help inform ecosystem-based management and precautionary approaches to better predict resource fluctuations caused by fisheries and changes in the marine environment.
Among the studies of D. mawsoni diets in area 88 of the Southern Ocean (Pacific Ocean sector), Kokorin (2010) analyzed differences in the composition of prey organisms based on the growth and habitat of D. mawsoni collected in the Ross and Amundsen Seas. In addition, Stevens et al. (2014) analyzed the differences in diet composition based on the growth, region, and fishing season of D. mawsoni collected in the Ross Sea. Both studies have demonstrated that the diet of D. mawsoni differs depending on its growth and area (Kokorin, 2010; Stevens et al., 2014). There was no difference in the dietary composition of D. mawsoni living in the Ross Sea between 2003 and 2010 (Stevens et al., 2014). Similarly, Queirós et al. (2022) reported differences in diet composition by region via morphological and isotopic analyses of D. mawsoni collected from the Amundsen Sea and Dumont D’Urville Sea. Yoon et al. (2017) and Lee et al. (2022) used metabarcoding to compare the diets of D. mawsoni collected in subareas 88.3 and 58.4 and identified various prey species that could not be accurately classified morphologically post-digestion. However, these authors could not analyze specific dietary ecology based on factors such as water depth and size class.
The present study analyzed the contents of D. mawsoni stomach samples collected over five years from 2016 to 2020 in the subarea 88.3 of the Southern Ocean. The study aimed to analyze feeding ecology in detail regarding temporal and spatial factors and size variations. We conducted quantitative analyses of differences in diet composition among research blocks and fishing seasons within subarea 88.3 and changes in D. mawsoni diet composition over time (fishing season), space (depth), and size class at each site (88.3_3 and 88.3_4) during each year of the study. This study will help improve our understanding of the feeding ecology, ecological niche, and functional role of D. mawsoni by identifying the main prey by area and the pattern of dietary changes in subareas of 88.3 of the Southern Ocean. In addition, this study provides ecological information for the sustainable use and management of D. mawsoni resources by predicting the impacts of fishing and environmental change.
2 Materials and methods
2.1 Sampling
The D. mawsoni samples used in this study were collected from the Korean commercial bottom longline vessel Greenstar from February to April during the 2015/16, 2016/17, 2017/18, 2018/19, and 2019/20 fishing seasons (e.g., 2015/16 fishing season: December 2015-November 2016) in subarea 88.3 of the Southern Ocean (Figure 1). Trotlines were used as fishing gears, and Humboldt squid (Dosidicus gigas) and Pacific herring (Clupea pallasii) were used as baits. The onboard scientific observer measured the total length (TL) and wet body weight of D. mawsoni with an accuracy of 0.1 cm and 0.1 kg, respectively. The sampled stomachs were preserved by freezing immediately after extraction and then transported to the laboratory using a ship and refrigerator truck.
2.2 Analysis of stomach
The frozen stomachs of each individual were thawed and dissected. Prey remnants were identified using taxonomic guides at the species level if the specimen in whole or parts remained intact and at the family or order level when digestion was advanced and identification was difficult (Gon and Heemstra, 1990; McMillan et al., 2012). The status of prey digestion was categorized as fresh, slightly digested, and digested, and the beaks of cephalopods and otoliths of fishes were included in subsequent analysis. We counted the number of prey organisms per species, measured their total length, and weighed them with an accuracy of 0.1 g. Plastics and stones were considered incidental ingestion and were included in the prey composition table but not in the subsequent dietary analysis. To quantitatively determine the composition of prey species in the study area, the frequency (%F), number (%N), and weight (%W) of each prey species were calculated using the following equations.
where Ai is the number of fish preying on species i, N is the total number of fish examined (excluding individuals with empty stomachs), Ni (Wi) is the number (wet weight) of prey species i, and Ntotal (Wtotal) is the total number (wet weight) of prey. Then the index of relative importance (IRI; Pinkas et al., 1971) was calculated for each prey type as follows:
and expressed as a percentage (%IRI):
The trophic level (TLk) to identify the ecological niche was obtained using the formula of Cortés (1999).
where Pi is the index of the relative importance of prey species i (IRI as in Pinkas et al., 1971), and TLi is the trophic level of prey species i. Trophic levels of prey taxa were analyzed by Pauly et al. (1998); Cortés (1999), and Ebert and Bizzarro (2007) were used as the average value.
To determine the differences in diet composition between areas, we compared the diet composition of D. mawsoni for each of the six research blocks (88.3_1, 88.3_2, 88.3_3, 88.3_4, 88.3_5, and 88.3_6) in sub-area 88.3. To identify changes in the diet composition according to the fishing season for each research block, data from 2015/16, 2016/17, 2017/18, 2018/19, and 2019/20 fishing seasons were used in this study in 2016, 2017, 2018, 2019, and 2020 respectively, and annual changes in diet composition during the Southern Ocean summer were analyzed. We also examined changes in prey composition with depth by dividing into three ranges (<1,200 m, 1,200–1,600 m, ≥1,600 m) and three size classes (<120 cm, 120–160 cm, ≥160 cm).
2.3 Statistical analysis
The weight dietary data of each prey taxa accurately determine the relative importance of each prey organism when feeding on prey of various sizes (or weights) (Anderson et al., 2008; Clarke and Gorley, 2015). Therefore, in this study, metric multidimensional scaling (mMDS), permutational multivariate analysis of variance (PERMANOVA), canonical analysis of principal coordinates (CAP), distance-based linear model (DISTLM), and similarity percentages (SIMPER) were performed using the weight ratio of the dominant prey organisms, excluding unidentified fish, to statistically analyze dietary changes according to each factor, and PRIMER v7 (Primer-e, Auckland, New Zealand) was used for all analyses.
To examine the dietary differences between research blocks with respect to fishing season, depth, and size class, dietary data for the species were randomly sorted into subgroups that included three to five individuals within each research block, fishing season, depth, and size class, and the average percentage weight of each prey taxon was determined for each of the resultant groups (Platell and Potter, 2001; Sommerville et al., 2011). Thus, random grouping of gravimetric data increased the effectiveness of multivariate analysis by reducing the number of prey items in samples with zero values (Lek et al., 2011; Park et al., 2021). We also performed a square-root transformation of the mean weight ratios of the prey species to reduce the bias of the dominant prey. These were analyzed using Bray-Curtis similarity/dissimilarity matrices (Platell and Potter, 2001; Anderson et al., 2008; Park et al., 2021).
First, a two-way PERMANOVA was conducted to determine the statistical significance of the differences in diet composition according to the factors of the research block (six levels) and fishing season (five levels) in subarea 88.3. Following the significance test results (PERMANOVA), post hoc tests using pairwise comparisons were performed to identify differences between means. In addition, CAP analysis was performed, which employed the mean weight percentage of prey taxa for the research blocks and fishing seasons. The relative contribution of prey taxa to the differences between the research blocks and fishing seasons was assessed using correlation coefficients. The correlation of prey taxa >0.4 was used as an arbitrary limit for a strong relationship between the dietary composition of each group and prey taxa on the principal coordinate axes. In addition, a distance-based linear model (DistLM) analysis was used to identify potential predictors that explained most of the dietary variability. The DistLM is a nonparametric procedure that performs distance-based multivariate multiple regression to model the relationship between multivariate data and one or more predictor variables. The potential predictors were research block, fishing season, depth, total length, and body weight. Depth, total length, and body weight were considered continuous variables, whereas other predictors were categorical. The most significant predictors in the conditional tests were selected using the “forward” selection method, using the Akaike Information Criterion (AIC).
Second, in each research block (88.3_3 and 88.3_4), where samples were collected for all five fishing seasons during the study period, the Bray-Curtis similarity matrix was visualized to assess the effects of fishing season (five levels), depth (three levels), and size class (three levels) using an mMDS ordination technique. In addition, a three-way PERMANOVA was conducted to determine the statistical significance of the differences in diet composition according to each factor (fishing season, depth, and size class). Pairwise comparisons were conducted for post hoc analysis when significant differences were found in specific factors or their interactions. CAP and SIMPER analyses were performed to identify the prey that explained most of the dissimilarities among the factors.
3 Results
3.1 Overall diet composition
Of the 1,639 stomachs examined, 606 (37.0%) were empty. Nine taxa were identified in the remaining 1,033 stomachs. In all research blocks (1–6), fishes were the most important prey item for D. mawsoni, with an IRI of 90.4% or higher (Table 1; Supplementary Table 1). Cephalopods were the second-most important prey item in the research blocks, except for 88.3_2, and crustaceans were the second-most important prey item in 88.3_2. Therefore, a trophic level of 4.20 or higher was demonstrated in all six research blocks. Macrourus spp. (Macrouridae) was the dominant taxon collected from research blocks 88.3_1 and 88.3_3. Nototheniidae was the dominant taxon in research blocks 88.3_2 and 88.3_6. Chionobathyscus dewitti (Channichthyidae) was the dominant taxon in research blocks 88.3_4 and 88.3_5. The second largest dietary component for D. mawsoni was C. dewitti which was dominant in research blocks 88.3_1, 88.3_3, and 88.3_6, while Bathydraco marri (Bathydraconidae), Macrourus spp., and Arctozenus risso (Paralepididae) were the second largest prey components for D. mawsoni collected at research blocks 88.3_2, 88.3_4, and 88.3_5, respectively.
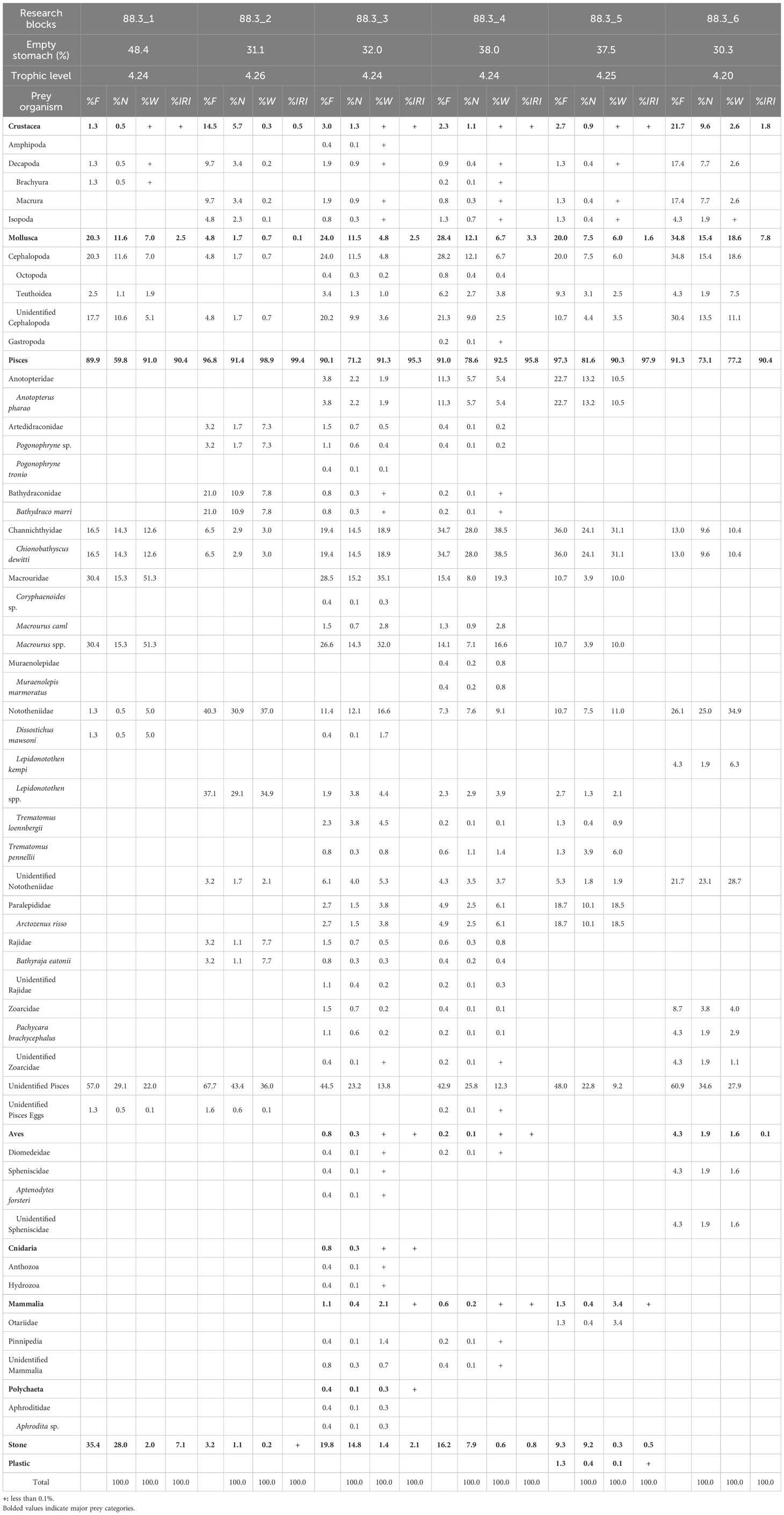
Table 1 Composition of the stomach contents of Dissostichus mawsoni caught in each research block in subarea 88.3 of Southern Ocean by frequency of occurrence (%F), number (%N), weight (%W), and index of relative importance (%IRI).
3.2 Temporal variability in diet composition
Fish were the most important prey in all research blocks during all fishing seasons in subarea 88.3, accounting for 77.2–99.6% of the total biomass. Cephalopods were consumed in all research blocks and during all fishing seasons (except 88.3_2 in 2017), accounting for 0.8–18.6% of the total biomass, as the second most important prey item in all research blocks and fishing seasons (except 88.3_3 in 2016 and 88.3_5 in 2018). We also analyzed the dominant prey for each research block and fishing season (Table 2), excluding unidentified fish. In research block 88.3_1, Macrouridae was the dominant prey during 2016 and 2019. In research block 88.3_2, Channichthyidae was the dominant prey in 2017, whereas Nototheniidae was the dominant prey in 2019. In research block 88.3_3, Macrouridae was the dominant prey taxon in all fishing seasons. In research block 88.3_4, Channichthyidae was the dominant prey organism in 2016, 2018, 2019, and 2020, whereas Macrouridae was the dominant prey taxon in 2017. In research block 88.3_5, Channichthyidae was the dominant prey organism in 2017, 2018, and 2019, whereas Anotopteridae was the dominant prey taxon in 2016. In research block 88.3_6, Nototheniidae was the dominant prey taxon in 2020.
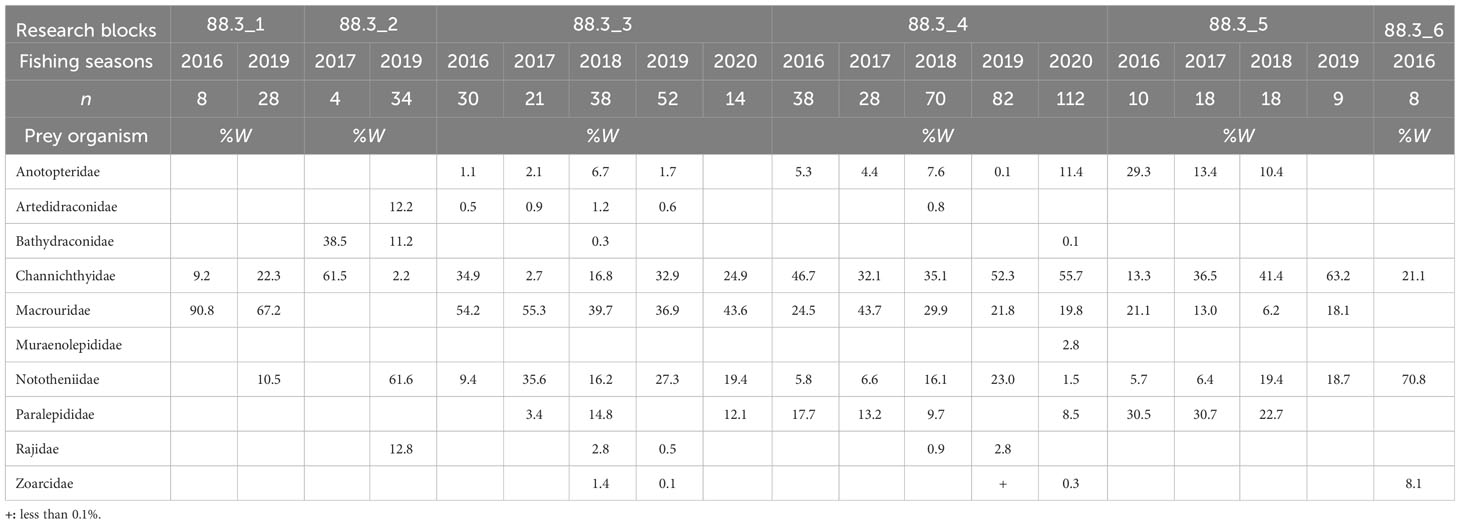
Table 2 Diet composition of the dominant prey item (fishes) of Dissostichus mawsoni caught in each research block (in subarea 88.3) and fishing season based on the weight (%W).
Two-way PERMANOVA (Supplementary Table 2A) indicated that the research block (PERMANOVA, p = 0.005) and the interaction between the research block and fishing season (PERMANOVA, p = 0.001) significantly affected the diet composition of D. mawsoni. Pairwise comparisons (Supplementary Table 2B) indicated significant differences in diet composition between research blocks 88.3_3 and 88.3_4 during all fishing seasons except 2018 and between research blocks 88.3_3 and 88.3_5 in 2016, 2017, and 2018. The canonical analysis of the principal (CAP) analysis plot (Figure 2) for the research block-fishing season interaction shows separation among research blocks, although the separation among fishing seasons is small. Among the prey taxa that contributed to differences among the research blocks, the contributions of Macrouridae and Channichthyidae were relatively high in research blocks 88.3_3, 88.3_4, and 88.3_5. In addition, in 2019, the contribution of Nototheniidae was relatively high at research block 88.3_4. The DistLM analysis (Supplementary Table 3) indicated that the diet composition of D. mawsoni was not significantly affected by body weight (p = 0.151). However, the research block (p = 0.001), fishing season (p = 0.001), depth (p = 0.001), and total length (p = 0.006) significantly affected D. mawsoni diet. The most parsimonious conditional model for diet composition included four predictors–research block, fishing season, depth, and total length–all of which exhibited significant effects. However, the conditional test model explained only 12.2% of the variability in the diet composition data, indicating that the variability in the diet of D. mawsoni cannot be explained by these predictors alone.
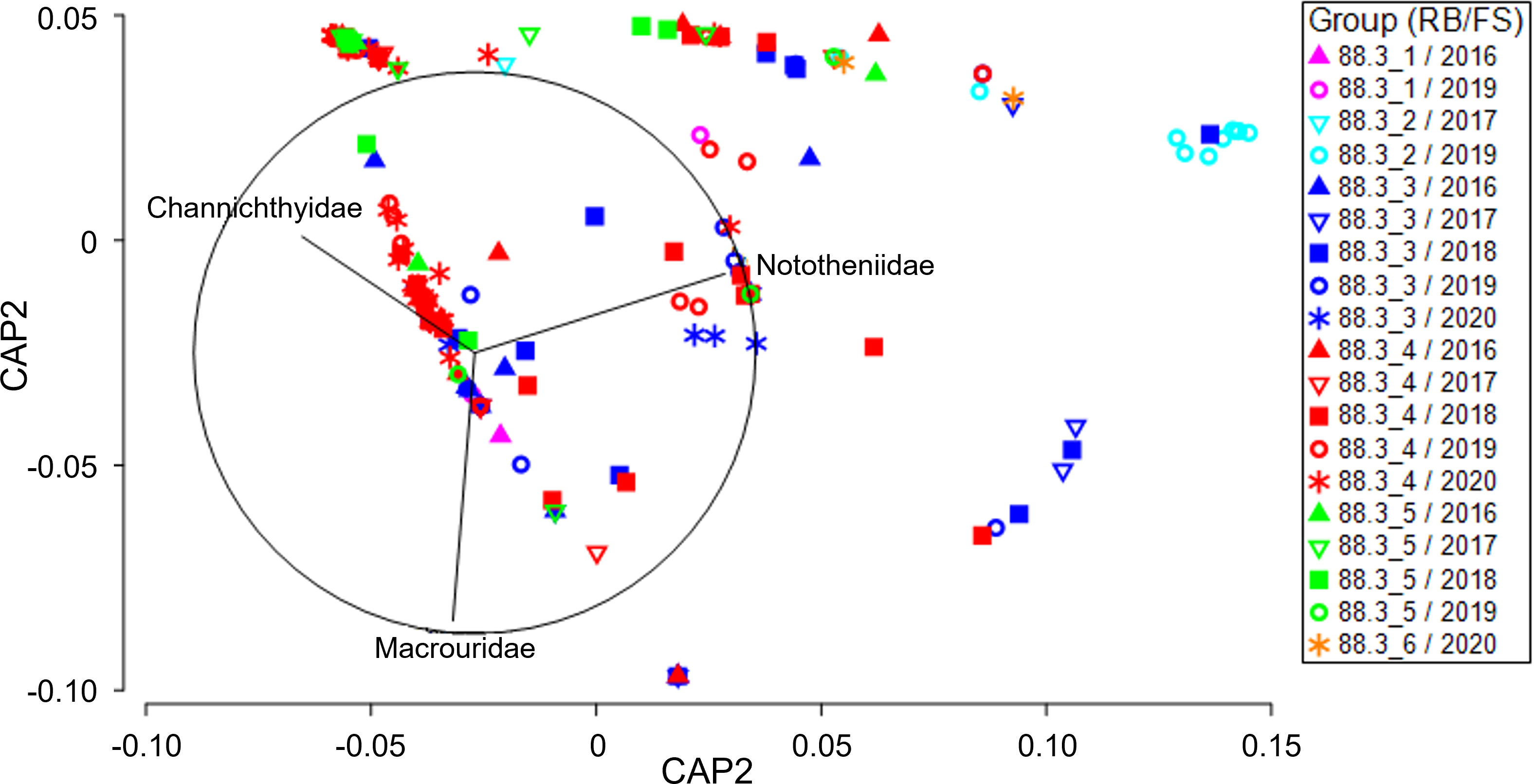
Figure 2 Canonical analysis of principal coordinates (CAP) ordination plot for the diet composition of a dominant prey item (fishes) of Dissostichus mawsoni caught in the subarea 88.3 of the Southern Ocean to assess differences between research block and fishing season. FS, Fishing season; RB, Research block.
3.3 Spatial and size-related variability in diet composition
Analyzing the variation in Antarctic toothfish emptying rates across depth ranges and size classes in research blocks 88.3_3 and 88.3_4 (Figure 3), we found that overall emptying rates decreased with increasing depth and decreased with increasing size class.
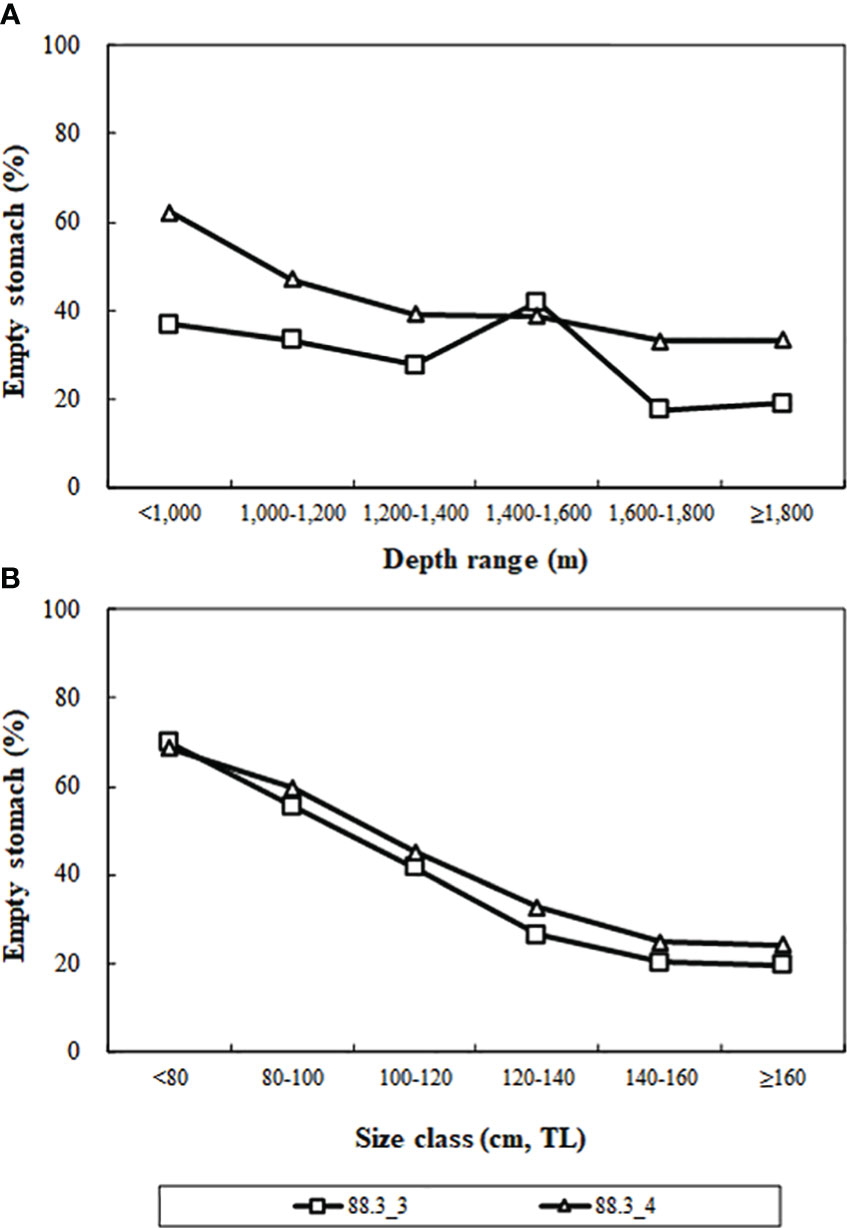
Figure 3 Percentage of empty stomachs by depth (A) and size classes (B) of Dissostichus mawsoni caught in the research block 88.3_3 and 88.3_4 in the Southern Ocean.
Fishes were the most important prey at depths of< 1,200, 1,200–1,600 m, and ≥ 1,600 m, accounting for 86.8%, 94.0%, and 95.3% of total biomass, respectively, in research block 88.3_3, and 98.4%, 93.5%, and 91.6%, respectively, in research block 88.3_4. We also examined changes in the diet composition of D. mawsoni with depth in research blocks 88.3_3 and 88.3_4 for dominant prey, excluding unidentified fish (Table 3). We found that at depths of< 1,200 m, Nototheniidae was the most dominant prey taxon in research blocks 88.3_3 and 88.3_4, whereas Macrouridae accounted for the second largest fraction of the diet in research blocks 88.3_3 and 88.3_4. At depths of 1,200–1,600 m and ≥ 1,600 m in research block 88.3_3, Macrouridae was the most dominant prey taxon, followed by Channichthyidae. However, at depths of 1,200–1,600 m and ≥ 1,600 m in research block 88.3_4, Channichthyidae was the most dominant prey taxon, followed by Macrouridae.
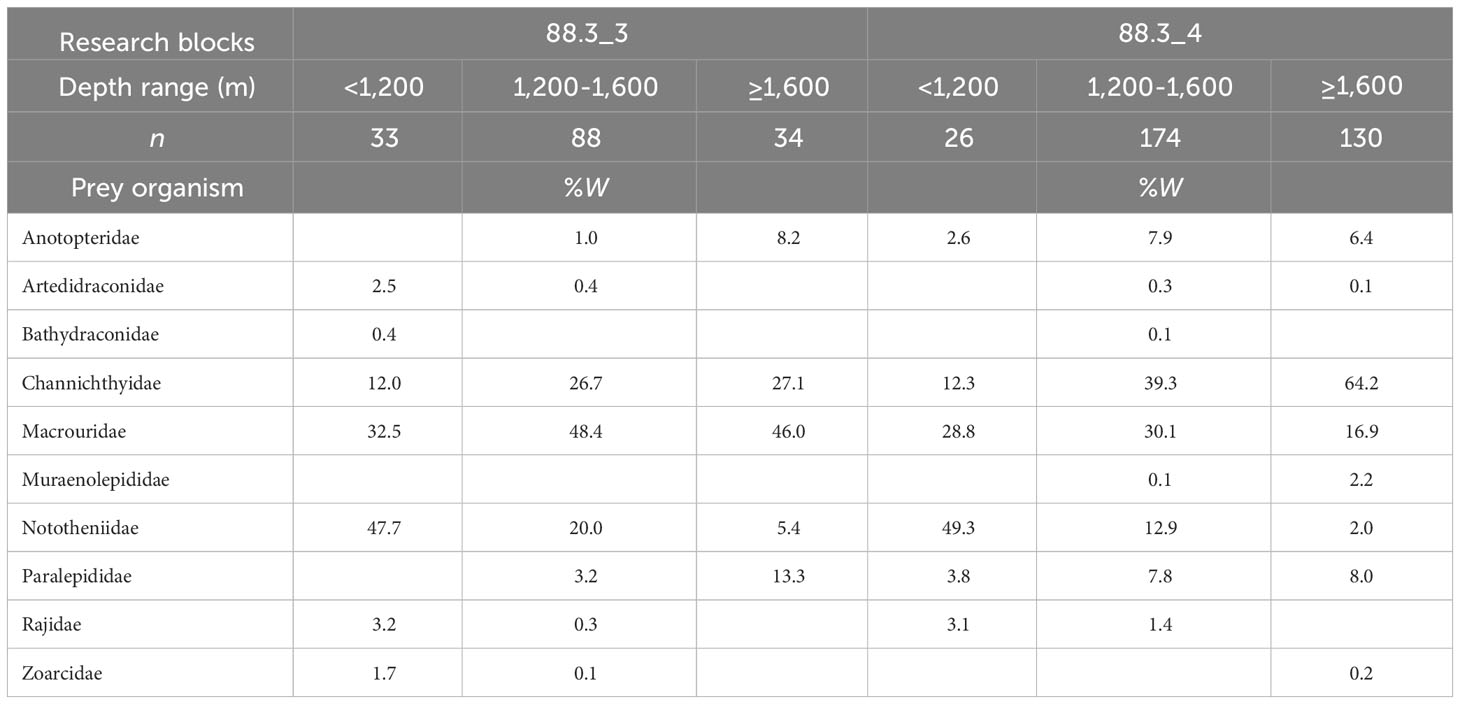
Table 3 Diet composition of the dominant prey item (fishes) of Dissostichus mawsoni caught in the research block 88.3_3 and 88.3_4 of the Southern Ocean based on the weight (%W) by depth.
Fishes were the most important prey for D. mawsoni, with size classes of< 120 cm, 120–160 cm, and ≥ 160 cm, accounting for 91.3%, 92.8%, and 92.7% of total biomass in research block 88.3_3, respectively, and 91.3%, 92.2%, and 97.1% in research block 88.3_4, respectively. In research block 88.3_3, the proportion of Macrouridae increased with D. mawsoni size (Table 4). The proportion of Channichthyidae decreased with an increase D. mawsoni size. In addition, in research block 88.3_4, Channichthyidae was the most dominant prey taxon in all size classes, and the proportion of Channichthyidae decreased with increasing D. mawsoni size. The proportion of Macrouridae increased slightly with an increase D. mawsoni size.
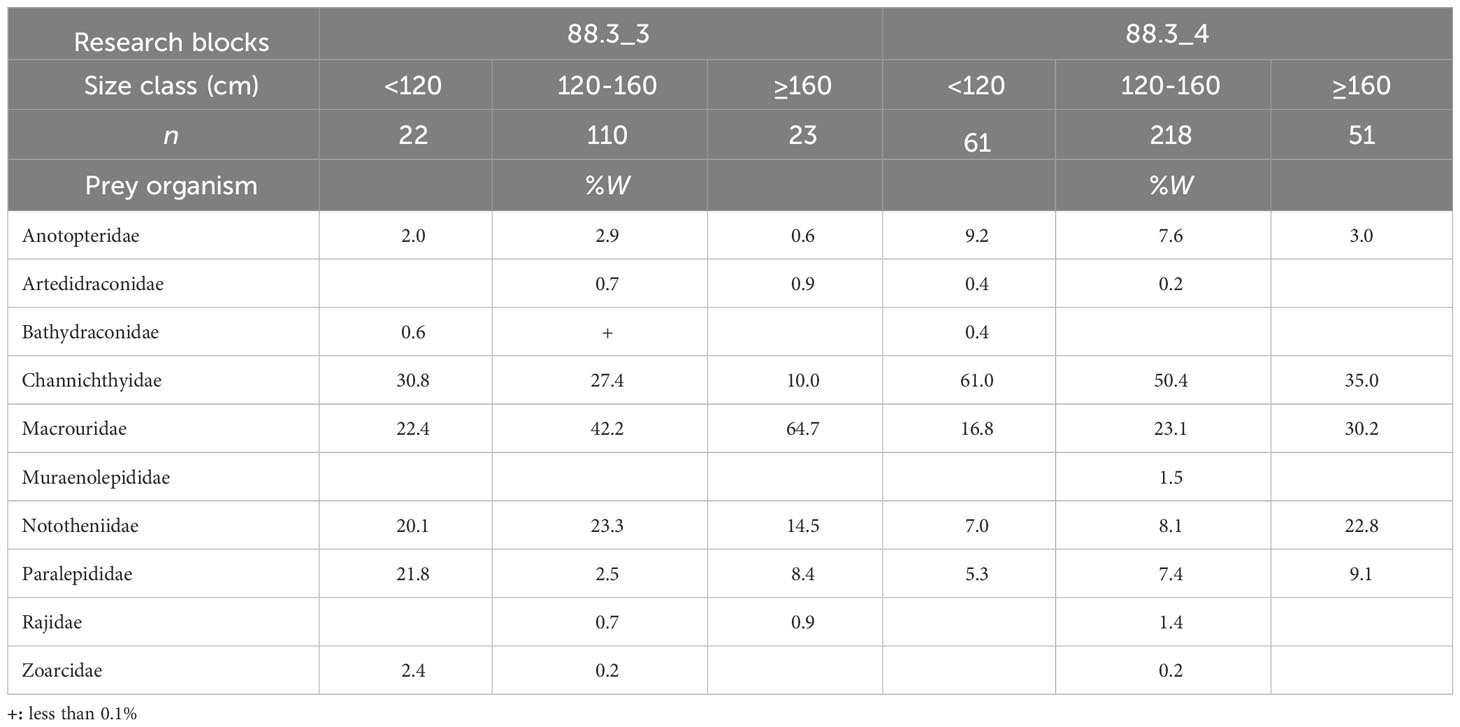
Table 4 Diet composition of the dominant prey item (fishes) of Dissostichus mawsoni caught in the research block 88.3_3 and 88.3_4 of the Southern Ocean based on the weight (%W) among size classes.
The metric MDS ordination of the mean dietary composition in research block 88.3_3 showed a near-complete overlap of multivariate dispersions among the fishing seasons (Figure 4A). In contrast, in research block 88.3_4 in 2019, the metric MDS ordination of the mean dietary composition showed little overlap with the other fishing seasons (Figure 4D). The ordination illustrates a near-complete overlap for all fishing seasons. In research blocks 88.3_3 and 88.3_4, diet composition at depths of< 1,200 and ≥ 1,600 m was clearly divided along the x-axis (Figures 4B, E). Furthermore, in research blocks 88.3_3 and 88.3_4, the 120–160 cm size class did not separate from the< 120 cm and ≥160 cm size classes, whereas clear separation along the x-axis occurred between the latter two size classes (Figures 4C, F).
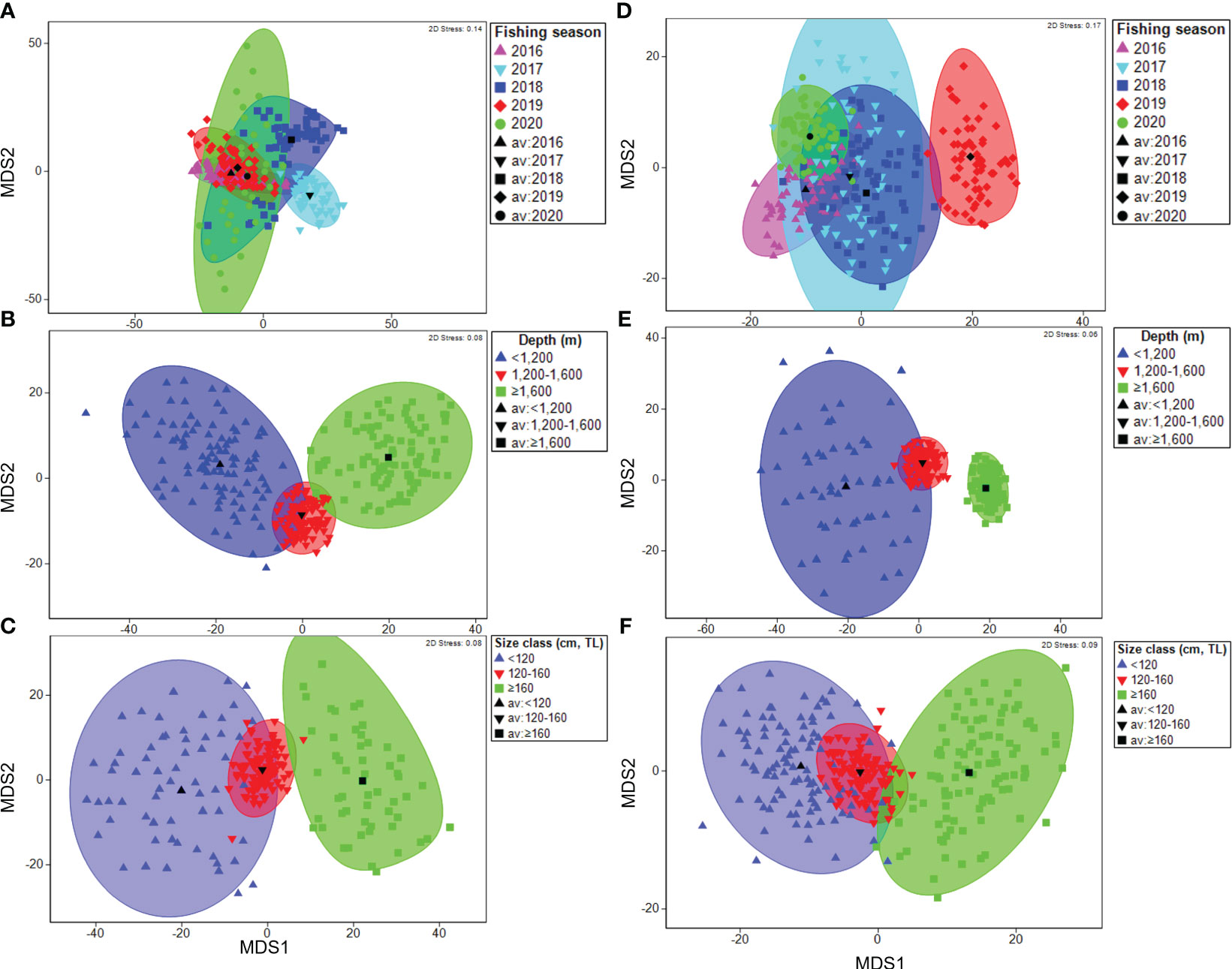
Figure 4 Metric multidimensional scaling (mMDS) ordinations of bootstrap averages for the diet composition of a dominant prey item (fishes) of Dissostichus mawsoni caught in the research block 88.3_3 and 88.3_4 of Southern Ocean from the five fishing season (A, D), three sampling depth classes (B, E), and three size classes (C, F).
Three-way permutational analysis of variance (PERMANOVA) indicated significant differences in the diet composition of D. mawsoni with depth (p = 0.004) in research block 88.3_3 (Supplementary Table 4A). However, the differences in all the other factors (p > 0.050) were not statistically significant. Pairwise comparisons in research block 88.3_3 (Supplementary Table 4B) indicated significant differences in the diet composition at all depths. The SIMPER analysis indicated that Nototheniidae had the highest contribution to diet composition between depths of < 1,200 m and 1,200–1,600 m and< 1,200 and ≥ 1,600 m. Between depths of 1,200–1,600 and ≥ 1,600 m, Channichthyidae had the highest contribution.
A three-way PERMANOVA analysis of toothfish diet composition in research block 88.3_4 (Supplementary Table 5A) indicated significant differences based on depth (PERMANOVA, p = 0.003), size class (PERMANOVA, p = 0.012), and the interaction between depth and size class (PERMANOVA, p = 0.008). Pairwise comparisons of sampling depth and size class in research block 88.3_4 showed significant differences in diet composition among sampling depths within each size class, and between ≥160 cm size class and other size classes within depths of ≥ 1,600 m (Supplementary Table 5B). A CAP analysis plot (Figure 5) of the depth-size class interaction illustrated group separation among depths, although the separation among size classes was small. Among the prey taxa contributing to differences among depths, the contribution of Nototheniidae was relatively high at< 1,200 m, while the contribution of Channichthyidae was high at ≥ 1,600 m. In addition, significant size-related differences at a depth of ≥ 1,600 m were characterized by Anotopteridae and Macrouridae high contributions from< 120 cm and ≥ 160 cm size classes, respectively.
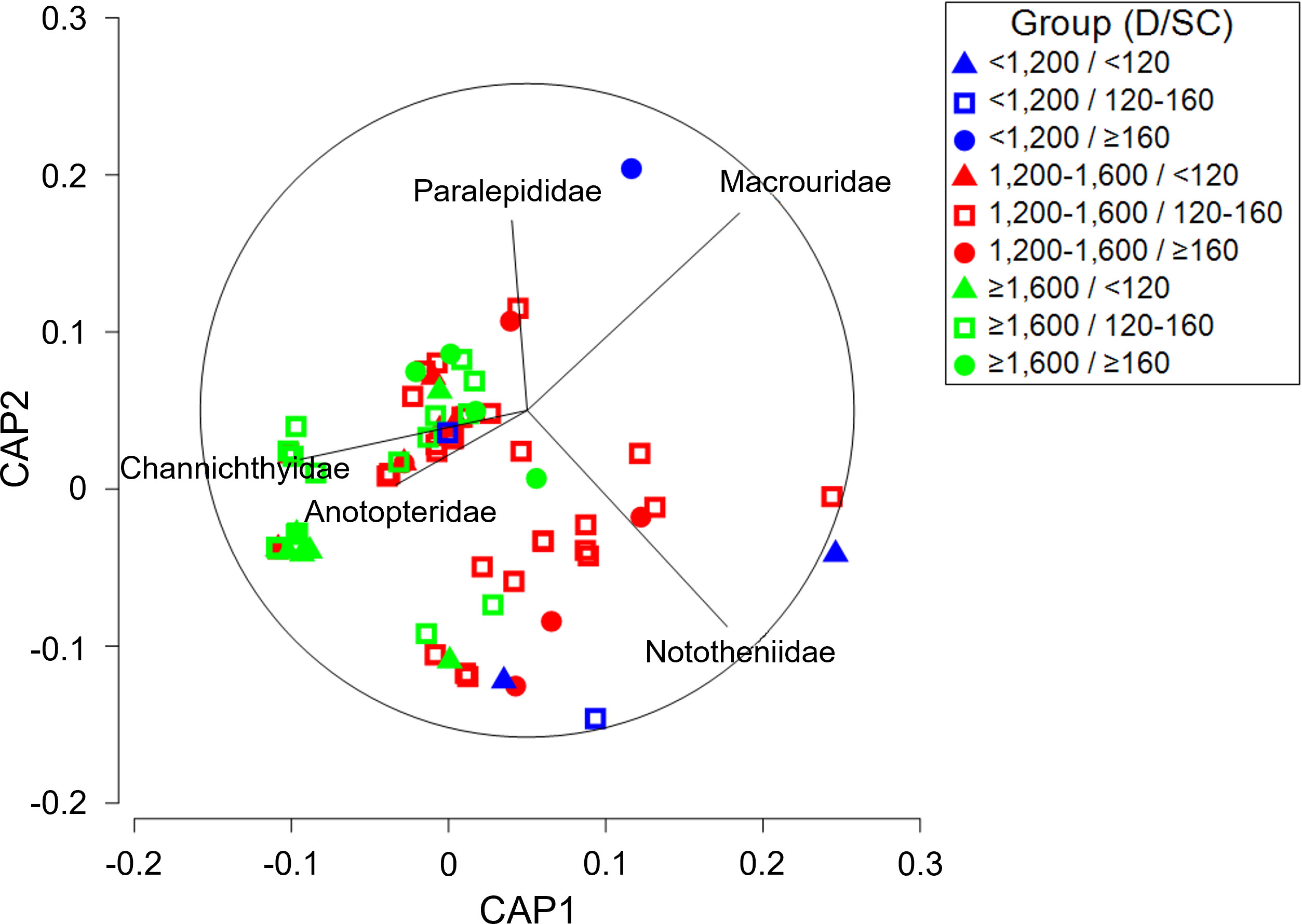
Figure 5 Canonical analysis of principal coordinates (CAP) ordination plot for the diet composition of a dominant prey item (fishes) of Dissostichus mawsoni caught in the research block 88.3_4 of Southern Ocean to assess differences between sampling depth class and size class. D, Depth; SC, Size class.
4 Discussion
It is presumed that C. dewitti, Macrourus spp., and Nototheniidae were the dominant prey species in this study because they are similar to D. mawsoni in their depth ranges and appear abundantly in the study area. Chionobathyscus dewitti, which appeared predominantly in this study, is the deepest among the 16 species belonging to Channichthyidae and has been reported to live at depths of up to 2,025 m (Kock, 2005; Balushkin and Prut’ko, 2006; Eastman et al., 2013). Macrourus caml occurred at depths of 350–2,080 m and was previously identified as M. whitsoni; however, McMillan et al. (2012) suggested the existence of two species. Lee et al. (2022) identified M. caml and M. whitsoni in the stomach contents of D. mawsoni from subarea 88.3 through metabarcoding. It is assumed that these two species are sympatric in subarea 88.3. Lepidonotothen spp. were assumed to be Lepidonotothen squamifrons based on comparisons with stomach content metabarcoding analysis by Lee et al. (2022). Lepidonotothen squamifrons, T. loennbergii, and T. pennellii are all known demersal fish, and they inhabit depths of 5–651 m (Koubbi et al., 2000; Eastman et al., 2013), 65–1,191 m (Gon and Heemstra, 1990; Eastman and Hubold, 1999), and 63–675 m (Hanchet et al., 2013) respectively. In addition, L. squamifrons, Macrourus spp., and C. dewitti are demersal fish predominantly observed in the western Southern Ocean (Amsler et al., 2016) and are the main bycatch species among the toothfish fisheries in subarea 88.3 (Arana and Vega, 1999).
The differences in diet composition among the research blocks resulted from a combination of factors, including habitat type, prey abundance, and water depth. Fish feeding differs markedly according to spatial factors, such as habitat type (Platell et al., 2007; Lek et al., 2011), differences in food resources (Mittelbach et al., 1992; Francis and Schindler, 2009), temporal factors, such as seasonal and annual changes in prey species (Hovde et al., 2002; Ginter et al., 2012), and ontogenetic changes (Werner and Gilliam, 1984; Park et al., 2021). Toothfish are top predators that feed on fish and cephalopods. They have been reported to show differences in diet composition depending on area, depth, and body size (Arkhipkin et al., 2003; Roberts et al., 2011; Stevens et al., 2014). The main prey of D. mawsoni are Channichthyidae and cephalopods in the Lazarev Sea (Petrov and Tatarnikov, 2011), and Macrouridae and cephalopods in the South Sandwich Islands (Roberts et al., 2011). In addition, Macrouridae dominates the diet composition of D. mawsoni in the Weddell Sea (Petrov and Gordeev, 2015) and subarea 58.4 (Seong et al., 2021). Furthermore, D. mawsoni, which inhabits the continental slopes of the Ross Sea, feeds mainly on Channichthyidae and Macrourus spp. However, in habitats such as ridges, hills, and seamounts, they mainly feed on Macrourus spp. (Stevens et al., 2014). D. mawsoni localized in the continental slope of the Amundsen Sea mainly feed on cephalopods, Macrourus spp., and Trematomus spp.; however, in the seamounts, they mainly feed on cephalopods, Macrourus spp., and Antimora rostrata (Queirós et al., 2022). Therefore, it is assumed that the food web structure in the Southern Ocean ecosystem differs depending on the local environment. Additional studies are required to understand the structure of the food webs in each area.
Fish cannibalism is affected by many factors, including the quantity and quality of prey and population density (Pereira et al., 2017). Cannibalism regulates population density (Mammel et al., 2022), and individuals grow faster than in populations without cannibalism (Berg et al., 2010; Huss et al., 2010). Toothfish are voracious predators that feed on their offspring. In this study, cannibalism of D. mawsoni was observed, with 163.8 cm and 158.8 cm individuals feeding on 58.1 cm and 54.3 cm individuals, respectively. Cannibalism of D. mawsoni in the past was reported by Queirós et al. (2022), and D. eleginoides was reported by Arkhipkin et al. (2003) and Troccoli et al. (2020).
Stomach emptiness in fishes is influenced by a variety of factors, including feeding ecology, environmental conditions, and sampling procedures (Vinson and Angradi, 2011). At higher trophic levels, piscivorous fishes consume larger, more energy-dense prey and have higher empty rates, on average, than omnivores, invertivores, planktivores, and herbivores (Malmquist et al., 1992; Arrington et al., 2002). Previous studies on Antarctic toothfish diets have reported emptying rates ranging from 9.8–69.1%, with an average of approximately 32% (Fenaughty et al., 2003; Kokorin, 2010; Petrov and Tatarnikov, 2011; Roberts et al., 2011; Stevens et al., 2014; Seong et al., 2021; Queirós et al., 2022), and these high emptying rates are similar to our results. Moreover, both Antarctic and Patagonian toothfish from South Sandwich Island have been shown to have decreased empty rates with increasing depth and size classes (Roberts et al., 2011), which is similar to our findings. These results are contrary to the hypotheses that deeper depths are more likely to cause stress-induced prey regurgitation (Pilling et al., 2001) and that relatively large fish should have higher empty rates because they feed on larger, more energy-dense prey (Vinson and Angradi, 2011). Therefore, the variation in emptying rates among Antarctic toothfish by depth and size group is likely the result of factors such as feeding ecology, physiological characteristics, and environmental conditions; however, further research is needed to clarify this.
Changes in the Antarctic toothfish prey composition with depth were closely linked to the depth range and abundance of each prey species. At shallow depths below 550 m, Nototheniidae such as Trematomus scotti and L. squamifrons are predominantly observed in the Bellingshausen Sea (Matallanas and Olaso, 2007). However, with increasing depth, the abundance of Nototheniidae decreased significantly, and Zoarcidae, Macrouridae, and Muraenolepidae dominated at depths > 550 m. In addition, Nototheniidae, such as L. squamifrons and T. loennbergii were dominant at depths of 400–599 m on Anvers Island and the Western Antarctic Peninsula, with a significant decrease in abundance observed at depths deeper than 599 m (Amsler et al., 2016). Macrourus spp. and C. dewitti were abundant at depths of 700–1,499 m and 900–1,499 m, respectively. In Marguerite Bay, Macrourus spp. and C. dewitti proportions were 5% and 6%, respectively, at depths of< 600 m and 30% and 12% at depths of 600–1,000 m, respectively. At depths > 1,000 m, the Macrourus spp. and C. dewitti proportions were 65% and 82%, respectively (Eastman et al., 2013).
In general, fish show variations in diet composition as they grow because they improve their swimming ability, increase their mouth size, become more food-demanding, and can consume various prey species (Barnes et al., 2010; Holt et al., 2019), and we believe that the changes in diet composition among size classes in this study are related to this. Differences in prey composition with growth rate are associated with an increased ability of larger individuals to consume a broader range of prey species and are known to reduce within-species competition for food (Park et al., 2022). Dissostichus mawsoni consumed Channichthyidae, Nototheniidae, Anotopteridae, and cephalopods in the<100 cm size class, whereas in the ≥100 cm size class, the diet was dominated by Macrouridae (Stevens et al., 2014; Seong et al., 2021; Pérez-Pezoa et al., 2023). This may be because D. mawsoni migrates to deeper depths due to ontogenetic shifts, thus feeding primarily on Macrouridae, which are abundant in deeper waters. Nevertheless, the observed differences in preferred prey sources among size classes in this study, even within the same depth range, suggest that they coexist in a way that avoids competition for food among the same species.
Data on most fish and cephalopod species in the Southern Ocean are inherently biased owing to fishery-dependent sampling (Caccavo et al., 2021). Similarly, data on the diet of D. mawsoni are largely fishery-dependent, which means that relatively large individuals are likely to be collected as a feature of fishing gear (Ashford et al., 2005), and most are caught on continental slopes at depths greater than 600 m in accessible summer sea ice-free areas, limiting their ability to understand the diet of D. mawsoni which inhabits a wide range of depths owing to ontogenetic variation. In this regard, at depths of 300-350 m in the Cosmonaut Sea, D. mawsoni fed primarily on fishes such as Trematomus eulepidotus and Chaenodraco wilsoni (Pakhomov and Tseytlin, 1992), in the McMurdo Sound sea ice region at depths of 500-600 m they fed primarily on Pleuragramma antarcticum; and on the continental shelf of the Ross Sea, D. mawsoni fed primarily on Trematomus spp. and Channichthyidae (Parker et al., 2019). Therefore, if a study is conducted through systematic sampling in space and time, it will be possible to collect specific life cycle characteristics, such as the feeding ecology of D. mawsoni according to seasonal and ontogenetic changes, which is important for ecosystem-based management.
Antarctic toothfish play an important role in the ecosystem as apex predators by influencing the structure of prey species populations and regulating the abundance of organisms at lower trophic levels. However, in recent years, many changes in the marine ecosystem have been observed in the Southern Ocean due to the direct and indirect effects of climate change and fisheries, and in the face of these challenges, CCAMLR is working to manage the species through an ecosystem-based, precautionary approach. As generalist feeders and opportunistic predators that feed on various fish and cephalopods, depending on prey availability in the area, we expect that Antarctic toothfish will flexibly adapt to changes in food abundance that may result from future environmental changes. However, Antarctic toothfish have been reported to respond negatively to future increases in water temperature due to their low tolerance to water temperatures above 2°C (Cheung et al., 2008; Reid, 2019), and little information is available on life cycle characteristics such as reproductive ecology and early life history stages; therefore, the impact of future climate change on Antarctic toothfish stocks remains unclear (Pérez-Pezoa et al., 2023). Therefore, to preserve marine ecosystems and conduct fisheries that consider species diversity and trophic interactions, it is necessary to continue dietary studies to obtain detailed information on the food web structure by area. In this study, we determined the ecological niche and role of the Antarctic toothfish in the Bellingshausen Sea and the eastern Amundsen Sea, where ecological data on this species are scarce. This allowed us to predict future changes in ecosystems, such as the trophic cascade of prey species (Channichthyidae, Macrouridae, and Nototheniidae) in response to changes in Antarctic toothfish abundance. In addition, no significant temporal differences in the diet composition of Antarctic toothfish were observed between 2016 and 2020 in each research block in subarea 88.3, suggesting that these data could be used as a baseline for identifying future environmental changes in the region. However, these results may be subject to inherent bias due to fishery-dependent summer opportunistic sampling, and further research is needed on the food web structure of the entire region for ecosystem-based precautionary management.
Data availability statement
The original contributions presented in the study are included in the article/Supplementary Material. Further inquiries can be directed to the corresponding author.
Ethics statement
Ethical approval was not required for the study involving animals in accordance with the local legislation and institutional requirements because The Antarctic toothfish individuals were collected during exploratory fisheries, which does not violate animal ethics because the stomachs of dead animals were used for the dietary analysis.
Author contributions
GB and SC designed this study. GS, D-GK, DK, SJ, and GB performed the experiments. GS and GB wrote the manuscript and prepared the figures and tables. GS and GB did the final editing of the manuscript. All authors discussed, read, and approved the final manuscript.
Funding
The author(s) declare financial support was received for the research, authorship, and/or publication of this article. This research was supported by the distant-water fisheries resources assessment and management of the National Institute of Fisheries Science, South Korea (R2023003).
Acknowledgments
We thank the captain and crew of the commercial fishing vessel Greenstar for the sample collection. We appreciate chief editor and reviewers for their fruitful comments that greatly improved the paper.
Conflict of interest
The authors declare that the research was conducted in the absence of any commercial or financial relationships that could be construed as a potential conflict of interest.
Publisher’s note
All claims expressed in this article are solely those of the authors and do not necessarily represent those of their affiliated organizations, or those of the publisher, the editors and the reviewers. Any product that may be evaluated in this article, or claim that may be made by its manufacturer, is not guaranteed or endorsed by the publisher.
Supplementary material
The Supplementary Material for this article can be found online at: https://www.frontiersin.org/articles/10.3389/fmars.2023.1240569/full#supplementary-material
Supplementary Table 1 | Range, mean and standard deviation (SD) of the depth of catch, total length and body weight of Dissostichus mawsoni caught in each research block (in subarea 88.3) and fishing season.
Supplementary Table 2 | Results of the two-way PERMANOVA (Permutational multivariate analysis of variance) analysis and p-values of pairwise comparisons on the diet composition of a dominant prey item (fishes) of Dissostichus mawsoni caught in the subarea 88.3 of Southern Ocean, considering the factors research blocks and fishing seasons.
Supplementary Table 3 | Results of distance-based linear models (DISTLM) analyzing the diet composition of a dominant prey item (fishes) of Dissostichus mawsoni concerning research block, fishing season, sampling depth, total length, and body weight individually (marginal test) and sequentially (conditional test) for a population in subarea 88.3 of Southern Ocean.
Supplementary Table 4 | Results of the three-way PERMANOVA (Permutational multivariate analysis of variance) analysis, pairwise comparisons, and SIMPER (Similarity percentages) on the diet composition of a dominant prey item (fishes) of Dissostichus mawsoni caught in the research block 88.3_3 of Southern Ocean, considering the factors fishing seasons, sampling depth classes, and size classes.
Supplementary Table 5 | Results of the three-way PERMANOVA (Permutational multivariate analysis of variance) analysis and p-values of pairwise comparisons on the diet composition of a dominant prey item (fishes) of Dissostichus mawsoni caught in the research block 88.3_4 of Southern Ocean, considering the factors fishing seasons, sampling depth classes, and size classes.
References
Ainley D. G., Eastman J. T., Brooks C. M. (2016). Comments on “The Antarctic toothfish (Dissostichus mawsoni): biology, ecology, and life history in the Ross Sea region,” by Hanchet S., et al. Hydrobiologia 771, 1–7. doi: 10.1007/s10750-015-2607-4
Amsler M. O., Eastman J. T., Smith K. E., Mcclintock J. B., Singh H., Thatje S., et al. (2016). Zonation of demersal fishes off Anvers Island, western Antarctic Peninsula. Antarct. Sci. 28 (1), 44–50. doi: 10.1017/S0954102015000462
Anderson M., Gorley R., Clarke K. (2008). PERMANOVA+ for PRIMER: Guide to Software and Statistical Methods (Plymouth: PRIMER-E).
Arana P. M., Vega R. (1999). Exploratory fishing for Dissostichus spp. in the Antarctic region (subareas 48.1, 48.2 and 88.3). CCAMLR Sci. 6 (1), 1–17.
Arkhipkin A., Brickle P., Laptikhovsky V. (2003). Variation in the diet of the Patagonian toothfish with size, depth and season around the Falkland Islands. J. Fish Biol. 63 (2), 428–441. doi: 10.1046/j.1095-8649.2003.00164.x
Arrington D. A., Winemiller K. O., Loftus W. F., Akin S. (2002). How often do fishes “run on empty”? Ecology 83 (8), 2145–2151. doi: 10.1890/0012-9658(2002)083[2145:HODFRO]2.0.CO;2
Ashford J. R., Jones C. M., Hofmann E., Everson I., Moreno C., Duhamel G. (2005). Can otolith elemental signatures record the capture site of Patagonian toothfish (Dissostichus eleginoides), a fully marine fish in the Southern Ocean> Can. J. Fish. Aquat. Sci. 62, 2832–2840. doi: 10.1139/f05-191
Balushkin A. V., Prut’ko V. G. (2006). On occurrences of Chionobathyscus dewitti (Notothenioidei Channichthyidae) in the Ross Sea. J. Ichthyol. 46 (3), 271–273. doi: 10.1134/S0032945206030076
Barnes C., Maxwell D., Reuman D. C., Jennings S. (2010). Global patterns in predator–prey size relationships reveal size dependency of trophic transfer efficiency. Ecology 91 (1), 222–232. doi: 10.1890/08-2061.1
Berg O. K., Finstad A. G., Olsen P. H., Arnekleiv J. V., Nilssen K. (2010). Dwarfs and cannibals in the Arctic: production of Arctic char (Salvelinus alpinus (L.)) at two trophic levels. Hydrobiologia 652 (1), 337–347. doi: 10.1007/s10750-010-0366-9
Berkes F. (2012). Implementing ecosystem-based management: Evolution or revolution? Fish Fish. 13 (4), 465–476. doi: 10.1111/j.1467-2979.2011.00452.x
Boyd I. L. (1996). Temporal scales of foraging in a marine predator. Ecology 77, 426–434. doi: 10.2307/2265619
Caccavo J. A., Christiansen H., Constable A. J., Ghigliotti L., Trebilco R., Brooks C. M., et al. (2021). Productivity and change in fish and squid in the Southern Ocean. Front. Ecol. Evol. 351. doi: 10.3389/fevo.2021.624918
Cheung W. W., Lam V. W., Pauly D. (2008). Modelling present and climate-shifted distribution of marine fishes and invertebrates. Fish. Cent. Res. Rep. 16 (3), 1–72.
Cortés E. (1999). Standardized diet compositions and trophic levels of sharks. ICES J. Mar. Sci. 56, 707–717. doi: 10.1006/jmsc.1999.0489
Cucuzza M., Stoll J. S., Leslie H. M. (2021). Evaluating the theoretical and practical linkages between ecosystem-based fisheries management and fisheries co-management. Mar. Policy 126, 104390. doi: 10.1016/j.marpol.2020.104390
Eastman J. T., Amsler M. O., Aronson R. B., Thatje S., McClintock J. B., Vos S. C., et al. (2013). Photographic survey of benthos provides insights into the Antarctic fish fauna from the Marguerite Bay slope and the Amundsen Sea. Antarct. Sci. 25 (1), 31–43. doi: 10.1017/S0954102012000697
Eastman J. T., Hubold G. (1999). The fish fauna of the Ross Sea, Antarctica. Antarct. Sci. 11 (3), 293–304. doi: 10.1017/S0954102099000383
Ebert D. A., Bizzarro J. J. (2007). Standardized diet compositions and trophic levels of skates (Chondrichthyes: Rajiformes: Rajoidei). Environ. Biol. Fish. 80, 221–237. doi: 10.1007/978-1-4020-9703-4_8
Fenaughty J. M., Stevens D. W., Hanchet S. M. (2003). Diet of the Antarctic toothfish (Dissostichus mawsoni) from the Ross Sea, Antarctica (subarea 88.1). CCAMLR Sci. 10, 113–123.
Francis T. B., Schindler D. E. (2009). Shoreline urbanization reduces terrestrial insect subsidies to fishes in North American lakes. Oikos 118 (12), 1872–1882. doi: 10.1111/j.1600-0706.2009.17723.x
Ginter K., Kangur K., Kangur A., Kangur P., Haldna M. (2012). Shifts in prey selection and growth of juvenile pikeperch (Sander lucioperca) over half a century in a changing lake Võrtsjärv. J. Appl. Sci. 2 (03), 168. doi: 10.4236/ojapps.2012.23024
Goldsworthy S. D., Lewis M., Williams R., He X., Young J. W., Van Den Hoff J. (2002). Diet of Patagonian toothfish (Dissostichus eleginoides) around Macquarie Island, South Pacific Ocean. Mar. Freshw. Res. 53, 49–57. doi: 10.1071/mf00075
Gon O., Heemstra P. C. (1990). Fishes of the Southern Ocean (Grahamstown, South Africa: J.L.B. Smith Institute of Ichthyology).
Hanchet S. M., Dunn A., Parker S., Horn P., Stevens D., Mormede S. (2015). The Antarctic toothfish (Dissostichus mawsoni): biology, ecology, and life history in the Ross Sea region. Hydrobiologia 761, 397–414. doi: 10.1007/s10750-015-2435-6
Hanchet S. M., Stewart A. L., McMillan P. J., Clark M. R., O'Driscoll R. L., Stevenson M. L. (2013). Diversity, relative abundance, new locality records, and updated fish fauna of the Ross Sea region. Antarct. Sci. 25 (5), 619–636. doi: 10.1017/S0954102012001265
Holt R. E., Bogstad B., Durant J. M., Dolgov A. V., Ottersen G. (2019). Barents Sea cod (Gadus morhua) diet composition: long-term interannual, seasonal, and ontogenetic patterns. ICES J. Mar. Sci. 76 (6), 1641–1652. doi: 10.1093/icesjms/fsz082
Hovde S. C., Albert O. T., Nilssen E. M. (2002). Spatial, seasonal and ontogenetic variation in diet of Northeast Arctic Greenland halibut (Reinhardtius hippoglossoides). ICES J. Mar. Sci. 59 (2), 421–437. doi: 10.1006/jmsc.2002.1171
Huss M., Van Kooten T., Persson L. (2010). Intra-cohort cannibalism and size bimodality: a balance between hatching synchrony and resource feedbacks. Oikos 119 (12), 2000–2011. doi: 10.1111/j.1600-0706.2010.18454.x
Kadin M., Blenckner T., Casini M., Gårdmark A., Torres M. A., Otto S. A. (2019). Trophic interactions, management trade-offs and climate change: The need for adaptive thresholds to operationalize ecosystem indicators. Front. Mar. Sci. 6. doi: 10.3389/fmars.2019.00249
Karim M. S., Techera E., Al Arif A. (2020). Ecosystem-based fisheries management and the precautionary approach in the Indian Ocean regional fisheries management organisations. Mar. pollut. Bull. 159, 111438. doi: 10.1016/j.marpolbul.2020.111438
Kock K. H. (2005). Antarctic icefishes (Channichthyidae): a unique family of fishes. A review, Part I. Polar Biol. 28 (11), 862–895. doi: 10.1007/s00300-005-0019-z
Kokorin N. V. (2010). The analysis of activeness and composition of food items of the Antarctic toothfish Dissostichus mawsoni (Nototheniidae) in Ross and Amundsen Seas during the 2006-2007 fishing season. Problems Fisheries 11, 48–59.
Koubbi P., Duhamel G., Hebert C. (2000). Role of bay, fjord and seamount on the early life history of Lepidonotothen squamifrons from the Kerguelen Islands. Polar Biol. 23 (7), 459–465. doi: 10.1007/s003009900106
Lee S. R., Choi S. G., Chung S., Kim D. N., Kang C. K., Kim H. W. (2022). Geographical differences in the diet of Dissostichus mawsoni revealed by metabarcoding. Front. Mar. Sci. 1499. doi: 10.3389/fmars.2022.888167
Lek E., Fairclough D. V., Platell M. E., Clarke K. R., Tweedley J. R., Potter I. C. (2011). To what extent are the dietary compositions of three abundant, co-occurring labrid species different and related to latitude, habitat, body size and season? J. Fish Biol. 78 (7), 1913–1943. doi: 10.1111/j.1095-8649.2011.02961.x
Link J. (2002). Does food web theory work for marine ecosystems? Mar. Ecol. Prog. Ser. 230, 1–9. doi: 10.3354/meps230001
Malmquist H. J., Snorrason S. S., Skúlason S., Jonsson B., Sandlund O. T., Jónasson P. M. (1992). Diet differentiation in polymorphic Arctic char in Thingvallavatn, Iceland. J. Anim. Ecol. 61, 21–35. doi: 10.2307/5505
Mammel M., Wang Y. C., Lan Y. C., Hsu C. M., Lee M. A., Liao C. H. (2022). Ontogenetic diet shifts and feeding dynamics of Trichiurus japonicus Temminck & Schlegel 1844, off Guishan Island, Southern East China Sea. Reg. Stud. Mar. Sci. 49, 102104. doi: 10.1016/j.rsma.2021.102104
Matallanas J., Olaso I. (2007). Fishes of the Bellingshausen sea and Peter I island. Polar Biol. 30 (3), 333–341. doi: 10.1007/s00300-006-0189-3
McMillan P., Iwamoto T., Stewart A., Smith P. J. (2012). A new species of grenadier, genus Macrourus (Teleostei, Gadiformes, Macrouridae) from the southern hemisphere and a revision of the genus. Zootaxa 3165 (1), 1–24. doi: 10.11646/zootaxa.3165.1.1
Melnychuk M. C., Peterson E., Elliott M., Hilborn R. (2017). Fisheries management impacts on target species status. Pro. Nat. Acad. Sci. 114 (1), 178–183. doi: 10.1073/pnas.1609915114
Mittelbach G. G., Osenberg C. W., Wainwright P. C. (1992). Variation in resource abundance affects diet and feeding morphology in the pumpkinseed sunfish (Lepomis gibbosus). Oecologia 90 (1), 8–13. doi: 10.1007/BF00317802
Mormede S., Dunn A., Hanchet S. M. (2014). A stock assessment model of Antarctic toothfish (Dissostichus mawsoni) in the Ross Sea region incorporating multi-year mark-recapture data. CCAMLR Sci. 21, 39–62.
Ng E. L., Deroba J. J., Essington T. E., Grüss A., Smith B. E., Thorson J. T. (2021). Predator stomach contents can provide accurate indices of prey biomass. ICES J. Mar. Sci. 78 (3), 1146–1159. doi: 10.1093/icesjms/fsab026
Pakhomov E. A., Tseytlin V. B. (1992). Diet of seven species of Antarctic fishes and estimation of their daily rations. J. Ichthyol. 32, 31–41.
Park J. M., Jung H. K., Lee C. I. (2021). Factors influencing dietary changes of walleye pollock, Gadus chalcogrammus, inhabiting the East Sea off the Korean coast. J. Mar. Sci. Eng. 9 (11), 1154. doi: 10.3390/jmse9111154
Park J. M., Jung H. K., Lee C. I., Park H. J. (2022). Winter food resource partitioning between sympatric Gadus macrocephalus and G. chalcogrammus in the northern coast of East Sea, South Korea inferred from stomach contents and stable isotopes analyses. Korean J. Ichthyol. 11, 191–197. doi: 10.35399/ISK.34.2.4
Parker S. J., Mormede S., Hanchet S. M., Devries A., Canese S., Ghigliotti L. (2019). Monitoring Antarctic toothfish in McMurdo Sound to evaluate the Ross Sea region marine protected area. Antarct. Sci. 31 (4), 195–207. doi: 10.1017/S0954102019000245
Pauly D., Trites A. W., Capuli E., Christensen V. (1998). Diet composition and trophic levels of marine mammals. ICES J. M. Sci. 55 (3), 467–481. doi: 10.1006/jmsc.1997.0280
Pereira L. S., Agostinho A. A., Winemiller K. O. (2017). Revisiting cannibalism in fishes. Rev. Fish Biol. Fish. 27 (3), 499–513. doi: 10.1007/s11160-017-9469-y
Pérez-Pezoa K., Cárdenas C. A., González-Aravena M., Gallardo P., Rivero A., Arriagada V., et al. (2023). Trophodynamics of the Antarctic toothfish (Dissostichus mawsoni) in the Antarctic Peninsula: Ontogenetic changes in diet composition and prey fatty acid profiles. PloS One 18 (10), e0287376. doi: 10.1371/journal.pone.0287376
Petrov A. F., Gordeev I.I. (2015). Distribution and biological characteristics of Antarctic toothfish Dissostichus mawsoni in the Weddell Sea. J. Ichthyol. 55 (2), 210–216. doi: 10.1134/S0032945215020137
Petrov A. F., Tatarnikov V. A. (2011). Results of investigation of the diet of Antarctic toothfish Dissostichus mawsoni (Nototheniidae) in the Lazarev Sea. J. Ichthyol. 51, 131–135. doi: 10.1134/S0032945210051017
Pilling G. M., Purves M. G., Daw T. M., Agnew D. A., Xavier J. C. (2001). The stomach contents of Patagonian toothfish around South Georgia (South Atlantic). J. Fish Biol. 59, 1370–1384. doi: 10.1111/j.1095-8649.2001.tb00198.x
Pinkas L., Oliphant M. S., Iverson I. L. K. (1971). Food habits of albacore, bluefin tuna and bonito in California waters. Fish Bull. 152, 1–105.
Platell M. E., Ang H. P., Hesp S. A., Potter I. C. (2007). Comparisons between the influences of habitat, body size and season on the dietary composition of the sparid Acanthopagrus latus in a large marine embayment. Estuar. Coast. Shelf Sci. 72 (4), 626–634. doi: 10.1016/j.ecss.2006.11.026
Platell M. E., Potter I. C. (2001). Partitioning of food resources amongst 18 abundant benthic carnivorous fish species in marine waters on the lower west coast of Australia. J. Exp. Mar. Biol. Ecol. 261 (1), 31–54. doi: 10.1016/S0022-0981(01)00257-X
Queirós J. P., Stevens D. W., Pinkerton M. H., Rosa R., Duarte B., Baeta A., et al. (2022). Feeding and trophic ecology of Antarctic toothfish Dissostichus mawsoni in the Amundsen and Dumont D’Urville Seas (Antarctica). Hydrobiologia 849 (10), 2317–2333. doi: 10.1007/s10750-022-04871-3
Reid K. (2019). “Climate change impacts, vulnerabilities and adaptations: Southern Ocean marine fisheries,” in Impacts of climate change on fisheries and aquaculture: Synthesis of current knowledge, adaptation and mitigation options. Eds. Barange M., Bahri T., Beveridge M. C. M., Cochrane K. L., Funge-Smith S., Poulain F. (Rome: FAO Fisheries and Aquaculture).
Roberts J., Xavier J. C., Agnew D. J. (2011). The diet of toothfish species Dissostichus eleginoides and Dissostichus mawsoni with overlapping distributions. J. Fish Biol. 79, 138–154. doi: 10.1111/j.1095-8649.2011.03005.x
Roessig J. M., Woodley C. M., Cech J. J., Hansen L. J. (2004). Effects of global climate change on marine and estuarine fishes and fisheries. Rev. Fish Biol. Fish. 14 (2), 251–275. doi: 10.1007/s11160-004-6749-0
Sallaberry-Pincheira P., Galvez P., Molina-Burgos B. E., Fernandoy F., Melendez R., Klarian S. A. (2018). Diet and food consumption of the Patagonian toothfish (Dissostichus eleginoides) in South Pacific Antarctic waters. Polar Biol. 41 (11), 2379–2385. doi: 10.1007/s00300-018-2360-z
Seong G. C., Choi S. G., Chung S., An D. H., Kim H. W., Baeck G. W. (2021). Morphological dietary composition of Antarctic toothfish (Dissostichus mawsoni) along the East Antarctic continental slope. Polar Biol. 44 (3), 499–508. doi: 10.1007/s00300-021-02820-9
Slocombe D. S. (1993). Implementing ecosystem-based management. Bioscience 43, 612–622. doi: 10.2307/1312148
Sommerville E., Platell M. E., White W. T., Jones A. A., Potter I. C. (2011). Partitioning of food resources by four abundant, co-occurring elasmobranch species: relationships between diet and both body size and season. Mar. Freshw. Res. 62 (1), 54–65. doi: 10.1071/MF10164
Stevens D. W., Dunn M. R., Pinkerton M. H., Forman J. S. (2014). Diet of Antarctic toothfish (Dissostichus mawsoni) from the continental slope and oceanic features of the Ross Sea region, Antarctica. Antarct. Sci. 26, 502–512. doi: 10.1017/S095410201300093X
Troccoli G. H., Aguilar E., Martínez P. A., Belleggia M. (2020). The diet of the Patagonian toothfish Dissostichus eleginoides, a deep-sea top predator off Southwest Atlantic Ocean. Polar Biol. 43 (10), 1595–1604. doi: 10.1007/s00300-020-02730-2
Vinson M. R., Angradi T. R. (2011). Stomach emptiness in fishes: sources of variation and study design implications. Rev. Fish. Sci. 19, 63–73. doi: 10.1080/10641262.2010.536856
Werner E. E., Gilliam J. F. (1984). The ontogenetic niche and species interactions in size-structured populations. Annu. Rev. Ecol. Syst. 15, 393–425. doi: 10.1146/annurev.es.15.110184.002141
Keywords: Antarctic toothfish, feeding ecology, Southern Ocean, CCAMLR, food web
Citation: Seong GC, Chung S, Kim D-G, Kang DY, Jin S and Baeck GW (2023) Feeding ecology of Antarctic toothfish, Dissostichus mawsoni in the subarea 88.3 (Bellingshausen Sea and eastern Amundsen Sea) of the Southern Ocean. Front. Mar. Sci. 10:1240569. doi: 10.3389/fmars.2023.1240569
Received: 15 June 2023; Accepted: 27 November 2023;
Published: 12 December 2023.
Edited by:
Chiara Papetti, University of Padua, ItalyReviewed by:
David Ainley, H.T. Harvey & Associates, United StatesCassandra Brooks, University of Colorado Boulder, United States
Copyright © 2023 Seong, Chung, Kim, Kang, Jin and Baeck. This is an open-access article distributed under the terms of the Creative Commons Attribution License (CC BY). The use, distribution or reproduction in other forums is permitted, provided the original author(s) and the copyright owner(s) are credited and that the original publication in this journal is cited, in accordance with accepted academic practice. No use, distribution or reproduction is permitted which does not comply with these terms.
*Correspondence: Gun Wook Baeck, gwbaeck@gnu.ac.kr