- 1College of Bioscience and Biotechnology, Hunan Agricultural University, Changsha, China
- 2Pharmaceutical College, Changsha Health Vocational College, Changsha, China
- 3Department of Gastroenterology, Changsha Hospital of Traditional Chinese Medicine, Changsha, China
Background: Chlorogenic acid (CGA), a natural bioactive polyphenol, exerts anti-inflammatory, antioxidant, and antibacterial effects that support the maintenance of intestinal health. However, the influence of CGA on gut microbiota and their metabolites, as well as its potential effects and mechanism of action in inflammatory bowel disease, remain to be elucidated.
Methods: First, an oral gavage was used to administer CGA to indomethacin-treated mice. Then, fecal microbiota transplantation was performed to explore the role of intestinal microbiota in indomethacin-induced inflammation.
Results: CGA treatment protected against body weight loss, damage to intestinal morphology and integrity, inflammation, and alteration of microbiota composition in indomethacin-treated mice. Interestingly, CGA failed to inhibit inflammation or protect intestine integrity in mice treated with antibiotics. Notably, mice who had been colonized with intestinal microbiota from CGA-treated or CGA-and-indomethacin-treated mice, through the fecal microbiota transplantation program, were protected from indomethacin-induced inflammation, growth of Bacteroides, and the accumulation of Bacteroides-derived LPS, in congruence with those who had been treated with CGA.
Conclusion: The results suggest that CGA may protect intestine integrity and alleviate inflammatory responses, primarily by inhibiting the growth of Bacteroides and the accumulation of Bacteroides-derived LPS, in indomethacin-induced colitis. This newly identified mechanism broadens our knowledge of how CGA exerts protective effects on intestinal inflammation and provides strategies for the prevention of gastrointestinal mucosal damage in patients treated with indomethacin.
Introduction
Chlorogenic acid (CGA), one of the most common and abundant polyphenols, is present in a wide variety of beverages and foods. This natural bioactive substance has been attracting the attention of researchers due to its strong anti-inflammatory and anti-oxidant properties, which are hypothesized to contribute to CGA's therapeutic effects on many diseases, including diabetes (1), cardiovascular diseases (2), cancer (3) and inflammatory bowel disease (4). However, although the biological function of CGA has been explored in detail, a paucity of reports exists on the specific mechanisms underlying the effects of CGA on inflammatory responses and mucosa damage in colitis.
Recently, studies have focused on the importance of microbiota to intestinal health, and have proven it to be critical for the maintenance of the intestinal immune system and the prevention of epithelial mucosa (5). Importantly, the microbiota is suggested to mediate the beneficial effects of dietary factors on colitis. CGA has been proven to modulate intestinal microbiota, through methods such as selectively increasing the relative abundance of the Bifidobacterium spp. and Clostridium coccoides-Eubacterium rectale groups in humans (6), Akkermansia in rodents (7), and lactobacillus in pigs (8). However, the mechanism by which changes in certain bacteria mediate CGA's effects on intestinal function and inflammation remains to be elucidated.
It is suggested that dietary CGA is only absorbed at a rate of approximately 33% in the small intestine, and as a result, most dietary CGA is able to reach the large intestine (9). In the present study, mice were treated with indomethacin, which induced ulceration in the small intestine and colon (10); this allowed for a focus on the effects of CGA on the colon. The changes in microbiota composition that may contribute to the beneficial effects of CGA on inflammatory response and intestinal function were identified. Through microbiota transplantation and LPS analyses, this study revealed that CGA protects against indomethacin-induced inflammation and mucosa damage by decreasing Bacteroides-derived LPS.
Materials and Methods
Animal Experiments
Experiment 1: Thirty-two C57BL/6J mice (7 weeks old) were purchased from Hunan SJA Laboratory Animal Co., Ltd (Changsha, China). After acclimating for 1 week, they were each assigned to one of four treatment groups: (1) Control group (CON); (2) Indomethacin group (IND); (3) Chlorogenic acid group (CA); (4) Indomethacin and chlorogenic acid group (IND-CA) (Figure 1A). Mice in the IND group were gavaged with PBS for the first 7 days and then with 5 mg/kg indomethacin (Selleck Chemicals, Houston, TX, USA) for the last 5 days; Mice in the CA group were gavaged with 50 mg/kg chlorogenic acid (Sigma, St. Louis, MO, USA) for the whole 12-day experiment; Mice in the IND-CA group were gavaged with chlorogenic acid for the whole period and with indomethacin for the last 5 days. Body weight was recorded daily for the last 6 days. Experiment 2: Sixteen mice (7 weeks old) were allowed to acclimate for 1 week, and then assigned to either the IND-CA or IND-CAA group (n = 8) (Figure 1B). Mice in the IND-CA group were treated with the same protocol as in Experiment 1 with regular drinking water, while mice in the IND-CAA group were treated as did in the IND-CA group and further treated with antibiotic water (1 g/L each of gentamicin and streptomycin, and 0.5 g/L each of ampicillin and vancomycin; Meilun Bio, Dalian, China) from Day 8 and onward. All animals were maintained in plastic cages under standard conditions and had free access to feed and water.
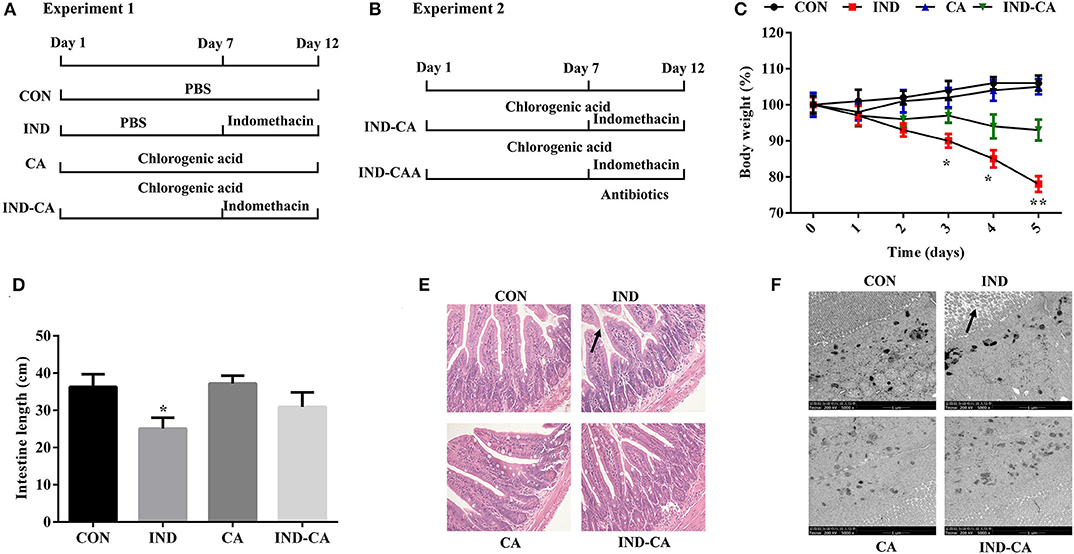
Figure 1. Chlorogenic acid prevented morphological damage to intestines of indomethacin-treated mice. A timeline with treatment in Experiment 1 (A) and Experiment 2 (B); (C) Body weight changes after the mice were gavaged with indomethacin; (D) Intestinal length; (E) HE staining of colon morphology (200×); (F) Ultrastructural observation of microvilli in colon by TEM (5000×). The morphology changes were marked with black arrows in (E,F). Values are expressed as mean ± SEM, n = 8. *P < 0.05; **P < 0.01.
The experimental protocol was approved by the Institutional Animal Care and Use Committee of Hunan Agricultural University (20190501) and mice were treated according to the animal care guidelines of Hunan Agricultural University (Changsha, China).
Gut Microbiota Transplantation
After treating with the antibiotic water for 10 days, the microbiota-depleted mice were given transplants of donor microbiota. Fecal samples (100 mg) were obtained from mice in the CON, CA, and IND-CA groups. The collected fecal samples were re-suspended in 1.0 mL sterile PBS and then centrifuged at 3000 g. The antibiotic-treated mice were gavaged with 0.1 mL/day microbiota supernatant for 5 days. After microbiota transplantation, the mice who received feces from these three groups were designated as MT-CON, MT-CA, and MT-IND-CA, respectively. Finally, these mice were further gavaged with 5 mg/kg indomethacin for another 5 days.
Sample Collection
At the end of the experiments, all animals were sacrificed and intestine length was measured. Then, colonic samples were collected and immediately frozen in liquid nitrogen and stored at −80°C until analysis of gene and protein expression was conducted. Additionally, colonic samples were either fixed in 10% neutral formaldehyde or in 2.5% glutaraldehyde for histological examination. Finally, cecum contents were collected for the analysis of microbiota composition.
Histological Analyses
The samples (1.0–1.5 cm proximal to the anus) fixed in formaldehyde were embedded in paraffin and 8-μm sections were stained with hematoxylin and eosin (HE) for the observation of histological morphology as previously described (11). In addition, pre-treatment of colonic samples and transmission electron microscopy (TEM) were performed. Briefly, the samples fixed in glutaraldehyde were rinsed in PBS twice and then post-fixed in osmium tetroxide. Then, the samples were dehydrated in 30, 50, 70, 90, and 100% acetones solutions, respectively. Finally, the dehydrated samples were embedded in araldite, and then stained with uranyl acetate and lead citrate for observation under a transmission electron microscope (Zeiss, Thornwood, NY, United States).
RT-qPCR Analysis
RT-qPCR analysis was performed as previously described (12). Briefly, a TRIZOL kit (Takara, Dalian, China) was used for the extraction of total RNA from colonic samples (1.5–2.0 cm proximal to the anus), which was reverse transcribed using a cDNA Reverse Transcription Kit (Takara). Then, RT-qPCR was performed with SYBR Green mix (Takara) according to the manufacturer's instructions. The primers are listed in Table 1.
Protein Qualification by the Wes Simple Western System
Protein qualification was performed using the Wes Simple Western System (ProteinSimple, San Jose, CA, USA) as previously described (13). Proteins extracted from colonic samples (2.0–3.0 cm proximal to the anus) were mixed with dithiothreitol, Master Mix, Simple Western Sample Buffer, and fluorescent standards (ProteinSimple) and loaded into Wes 25-well plates. Thereafter, primary antibodies (phosphorylated NFκB (pNFκB) and NFκB, Abcam, Cambridge, MA, USA), secondary antibodies, stacking gel matrix, luminol-peroxide mixture, and separation gel matrix were added. Results were collected using the “gel view” function of the Protein Simple software.
Immunofluorescence Assay for Tight Junction Proteins
Colonic samples embedded in paraffin were sliced into 8-μm sections and incubated with primary antibodies (occludin and claudin-1) overnight at 4°C. Subsequently, the slices were further incubated with the secondary antibodies at 37°C for 1 h. They were then mounted on a slide with DAPI (Sigma) and representative pictures were taken using a Zeiss LSM880 confocal microscope (Shanghai, China).
FITC-Dextran Intestinal Permeability Assay
Oral gavage of FITC-dextran (Sigma) was performed to determine intestinal permeability. Animals were gavaged with 600 mg/kg FITC-dextran. Serum samples were collected 4 h later, and FITC-dextran concentrations were assayed by fluorometry.
Determination of Myeloperoxidase, Eosinophil Peroxidase and LPS Contents
Myeloperoxidase (MPO), eosinophil peroxidase (EPO), and LPS concentrations were determined using ELISA quantitative kits (Meimian, Nanjing, China) according to the manufacturer's instructions.
Gut Microbiota Profiling
Samples of cecum contents were collected and homogenized, and then used for DNA extraction using the QIAamp DNA stool Mini Kit (Qiagen, Shanghai, China). The V3–V4 regions of bacterial 16S rRNA gene sequences were amplified. PCR was performed in a solution consisting of 12.5 mL of Phusion High-Fidelity PCR Master Mix (Beverly, MA, United States), 50 ng of template DNA, and 1 mL of forward and reverse primer, respectively. The PCR products (400–450 bp) were purified for MiSeq Illumina sequencing, which was performed using the Illumina HiSeq2500 platform (San Diego, CA, United States). Then, the merged reads were assigned to each sample based on their unique barcodes. Based on 97% sequence similarity, OTUs were obtained by clustering the high-quality clean tags. Analysis was performed with representative OTUs using the Greengenes database with the RDP algorithm.
Statistical Analysis
Data were collected for statistical analysis performed using the t-test or one-way ANOVA with SPSS 18.0 (Chicago, IL, USA). All data were expressed as means ± standard error (SEM) and a P-value of <0.05 was considered statistically significant.
Results
Chlorogenic Acid Prevented Morphological Damage to Intestines of Indomethacin-Treated Mice
The indomethacin-treated group showed significantly decreased body weight at the third day of treatment, while no changes were observed in the mice of the other three groups (Figure 1C). Intestinal length was significantly decreased in the indomethacin-treated group, while this change was prevented when chlorogenic acid was added to the treatment protocol (Figure 1D). HE staining results showed partial loss and sloughing of colonic villi in mice treated with indomethacin, while no changes were observed in the villi shapes of the other three groups (Figure 1E). Moreover, TEM results showed that the microvilli were irregular as a result of indomethacin treatment, while no such change was observed in the other groups (Figure 1F).
Chlorogenic Acid Prevented Inflammation in the Colons of Indomethacin-Treated Mice
As shown in Figure 2, indomethacin treatment gave rise to significant increases in the gene expression of TNFα, IL1β, and IL8 in the colon, while chlorogenic acid prevented these increases. No significant change in the gene expression of IL6 was observed among the four treatment groups. In addition, pNFκB protein expression in the colon was significantly increased due to indomethacin treatment, while no change was observed in the mice treated with chlorogenic acid.
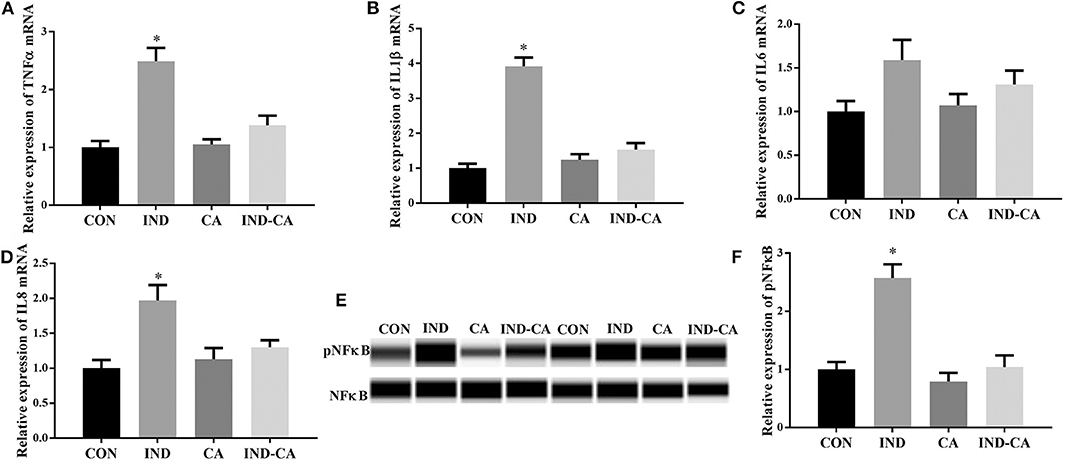
Figure 2. Chlorogenic acid prevented inflammation in the colons of indomethacin-treated mice. Relative mRNA expression of TNFα (A), IL1β (B), IL6 (C), and IL8 (D); (E) pNFκB expression determined by the WES Simple Western System. A full image of panel (E) shown in Supplementary Figure 1; (F) Relative abundance of pNFκB, (F) is the quantification of the WB in E. Values are expressed as mean ± SEM, n = 8 for results of gene expression and n = 3 for results of protein expression. *P < 0.05.
Chlorogenic Acid Prevented Intestinal Barrier Dysfunction in Indomethacin-Treated Mice
Indomethacin treatment significantly decreased occludin and claudin-1 protein expression, while it failed to cause such changes in mice treated with chlorogenic acid (Figures 3A–C). The FITC fluorescence intensity and the EPO and MPO concentrations were significantly increased by indomethacin treatment, while no such changes were observed in mice treated with chlorogenic acid (Figures 3D–F).
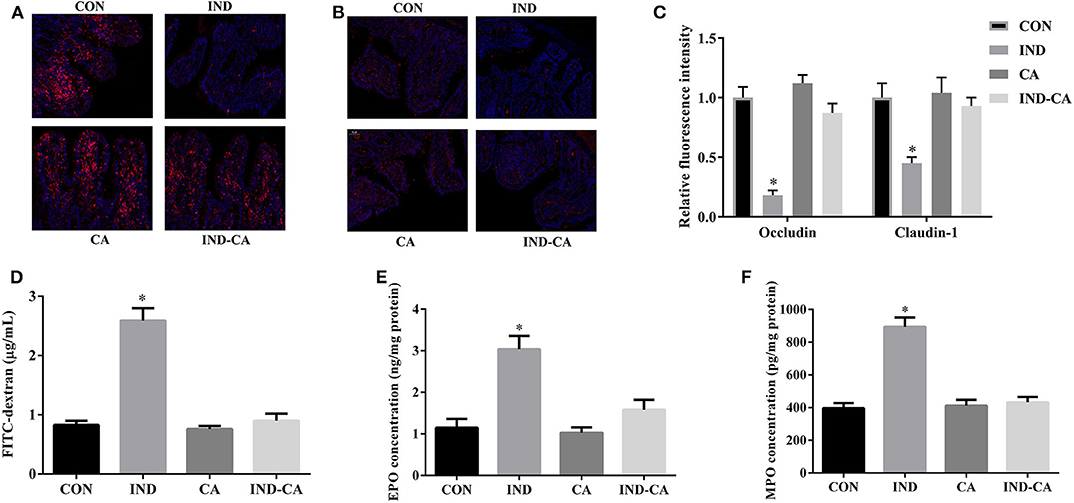
Figure 3. Chlorogenic acid prevented intestinal barrier dysfunction in indomethacin-treated mice. Occludin (A) and claudin-1 (B) expression in colon by immunofluorescence assay (Red, tight junction proteins; Blue, DAPI); (C) Relative fluorescence intensity of occluding and claudin-1 (n = 3); (D) Concentration of FITC-dextran in serum; EPO (E) and MPO (F) concentrations in colonic tissue. Values are expressed as mean ± SEM, n = 8. *P < 0.05.
Chlorogenic Acid Decreased Bacteroides Abundance and LPS Content in Indomethacin-Treated Mice
The microbiota of the ceca of different groups were analyzed. PCoA analysis highlighted the differences in the composition of microbiota between the IND group and the other three groups (Figure 4A). The Firmicutes/Bacteroidetes ratio was significantly lower in the IND group when compared with the ratios in the other three groups (Figure 4B). Additionally, the relative abundance of Bacteroides at the genus level and Bacteroidetes at the phylum level in the microbiota of the IND group was significantly higher than those of the other three groups (Figures 4C,D). Notably, LPS levels in the colonic tissue and cecum had significantly increased in the IND group, while no similar change was observed in the other three groups (Figures 4E,F).
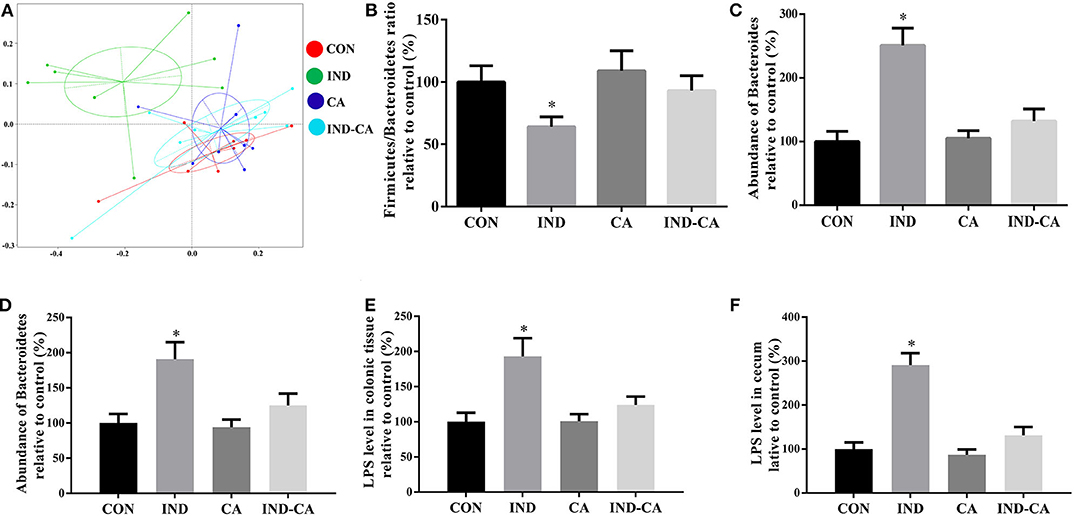
Figure 4. Chlorogenic acid decreased Bacteroides-derived LPS content in indomethacin-treated mice. (A) PCoA plot of the cecum microbiota; (B) Relative ratio of Firmicutes to Bacteroidetes; Relative abundance of Bacteroides at the genus level (C) and Bacteroidetes at the phylum level (D); Relative LPS content in the colonic tissue (E) and cecum (F). Values are expressed as mean ± SEM, n = 8. *P < 0.05.
Microbiota Depletion by Antibiotics Blocked the Anti-inflammatory Effect of Chlorogenic Acid in Indomethacin-Treated Mice
As shown in Figure 5, mice in the IND-CAA group had higher gene expression of TNFα, IL1β, IL6, and IL8 in colonic tissue than those in the IND-CA group. Additionally, mice in the IND-CAA group had higher concentrations of EPO and MPO in colonic tissue than did those in the IND-CA group.
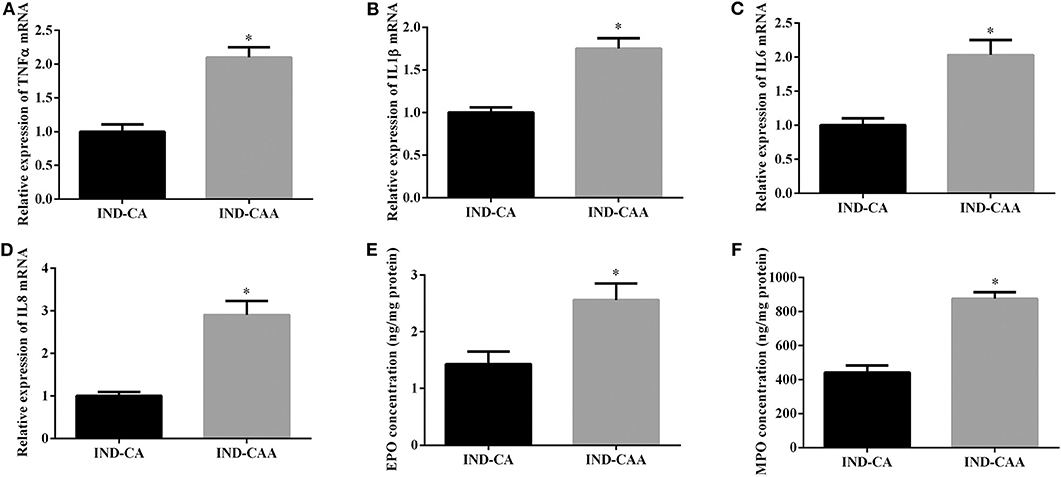
Figure 5. Microbiota depletion by antibiotics blocked the anti-inflammatory effect of chlorogenic acid in indomethacin-treated mice. Relative mRNA expression of TNFα (A), IL1β (B), IL6 (C), and IL8 (D); EPO (E) and MPO (F) concentrations in colonic tissue. Values are expressed as mean ± SEM, n = 8. *P < 0.05.
CGA-Originated Microbiota Prevented Intestinal Morphology Damage and Barrier Dysfunction Caused by Indomethacin
After the gut microbiota transplantation program, mice in the MT-CA and MT-IND-CA groups experienced less body weight loss than mice in the MT-CON group (Figure 6A). Compared with those in MT-CON group, mice in MT-CA and MT-IND-CA exhibited longer intestine length and lower FITC fluorescence intensity in serum (Figures 6B,C). HE staining results showed that mice in the MT-CON group had partial loss and sloughing of colonic villi, while no such changes were observed in mice of the MT-CA and MT-IND-CA groups (Figure 6D). In addition, results of an immunofluorescence assay showed that mice in the MT-CON group had a lower expression level of occludin and claudin-1 than did mice in MT-CA and MT-IND-CA (Figures 6E,F).
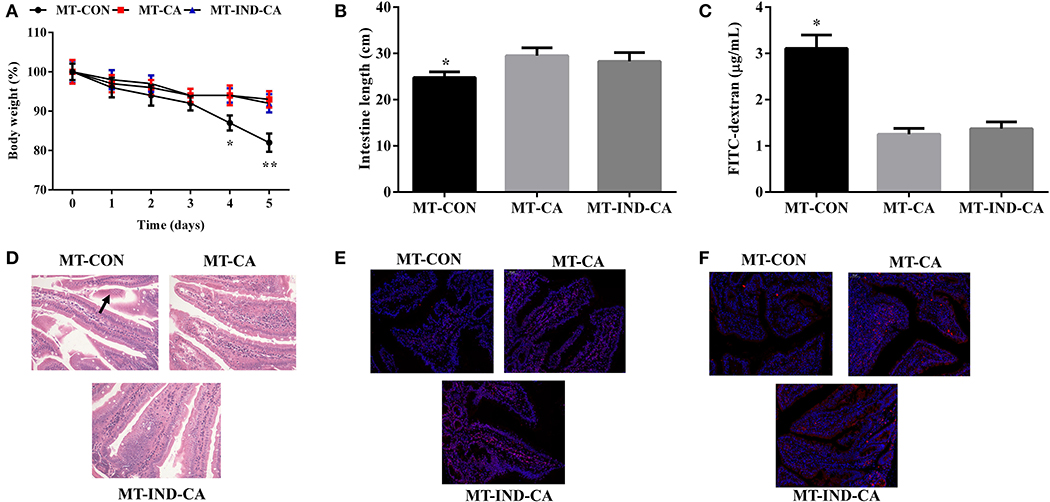
Figure 6. CGA-originated microbiota prevented intestinal morphology damage and barrier dysfunction caused by indomethacin. (A) Body weight changes after the mice were gavaged with indomethacin; (B) intestinal length; (C) Concentration of FITC-dextran in serum; (D) HE staining of colon morphology (200×), The morphology changes were marked with black arrows; Occludin (E) and claudin-1 (F) expression in colon by immunofluorescence assay (Red, tight junction proteins; Blue, DAPI). Values are expressed as mean ± SEM, n = 4. *P < 0.05; **P < 0.01.
CGA-Originated Microbiota Prevented Intestinal Inflammation and Inhibited the Growth of LPS-Producing Bacteroides Caused by Indomethacin
Mice in the MT-CON group had higher gene expression of TNFα, IL1β, IL6, and IL8 in colonic tissue than those in the MT-CA and MT-IND-CA groups (Figures 7A–D). Additionally, mice in the MT-CON group had a lower Firmicutes/Bacteroidetes ratio and more abundant Bacteroides and LPS levels when compared with those in the MT-CA and MT-IND-CA groups (Figures 7E–G).
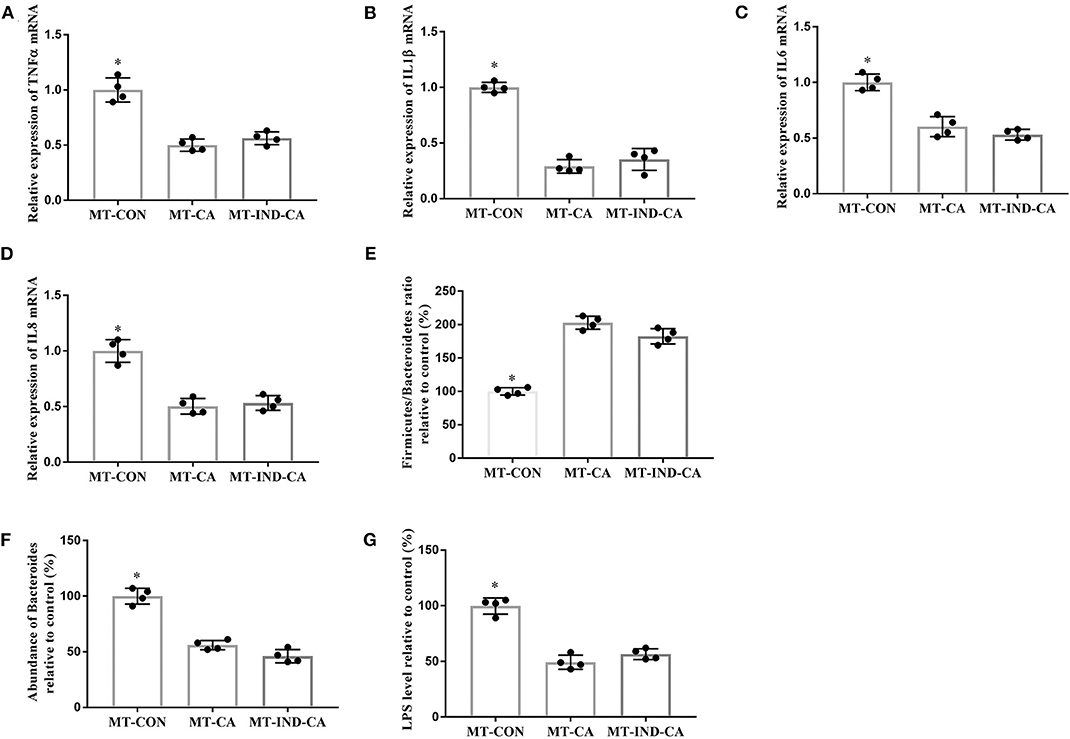
Figure 7. CGA-originated microbiota prevented intestinal inflammation and inhibited the growth of LPS-producing Bacteroides caused by indomethacin. Relative mRNA expression of TNFα (A), IL1β (B), IL6 (C), and IL8 (D); (E) Relative ratio of Firmicutes to Bacteroidetes; (F) Relative abundance of Bacteroides at the genus level; (G) Relative LPS content in the colonic tissue. Values are expressed as mean ± SEM, n = 4. *P < 0.05.
Discussion
The natural polyphenol CGA has numerous biological functions, and the protective effects of CGA on the gastrointestinal tract have been widely demonstrated in a variety of in vivo and in vitro models (4, 14, 15). Dietary CGA plays important roles in the maintenance of intestinal morphology, intestinal permeability, and barrier function via different mechanisms. Since intestinal permeability is positively associated with mitochondrial dysfunction, a previous study has suggested that CGA exerts its beneficial effects by decreasing mitochondrial lipid peroxidation and ameliorating mitochondrial respiratory chain dysfunction (15). The strength of the intestinal physical barrier is determined by its major structural basis, the tight junction. Moreover, the activation of myosin light chain kinase (MCK) is essential for intestinal barrier function, as it triggers changes in tight junctions by promoting the phosphorylation of myosin light chain. CGA has been proven to decrease MCK expression and improve the dynamic distribution of tight junction proteins in a rodent model of colitis (16), confirming its beneficial effects on intestinal barrier function. This study found that CGA prevents increases in intestinal leakage, as serum FITC-labeled dextran was found to decrease. Moreover, CGA increases the expression of tight junction proteins in the colon. These results suggest that CGA exerts the same effects on intestinal permeability and barrier function in indomethacin-induced colitis, which gives insight into the protective effects of CGA on the gastrointestinal tract in different models of colitis. Ulcerative colitis is a common form of inflammatory bowel disease (IBD) characterized by inflammation and colonic mucosa. It is important to alleviate the inflammatory response during IBD treatment. In vitro experiments have shown that CGA exerts strong anti-inflammatory effects via the activation of the Nrf2 or NFκB signaling pathway in Caco-2 cells challenged with a mixture of phorbol, 12-myristate, 13-acetate, and interferon γ or a mixture of TNFα and H2O2 (4, 14). Moreover, CGA prevents inflammatory cell infiltration and inhibits the over-accumulation of inflammatory cytokines in the rodent model of colitis induced by dextran sulfate sodium (4, 7). However, although the anti-inflammatory effects of CGA have been proven in this model of IBD, further evidence is needed to confirm these findings. This study found that CGA deceases the expression of inflammatory cytokines and inhibits the expression of NFκB in indomethacin-induced colitis, and these new findings allow for a broader understanding of the effects of CGA on the prevention of IBD.
Indomethacin, one of the most widely used non-steroidal anti-inflammatory drugs, exerts adverse effects on gastrointestinal mucosa (17). Indomethacin treatment results in extraintestinal lesions, inflammatory responses, and the inhibition of mucosal cell renewal. Consequently, it is a priority to find strategies to effectively and safely prevent gastrointestinal mucosal damage and inflammation in patients treated with indomethacin. Additionally, based on its adverse effects on intestine, indomethacin has been used to induce colonic ulceration in rodents, which has been suggested to be an appropriate animal model for IBD research (10). The model has some advantages, such as being easily induced and sharing some similarities with Crohn's disease. However, the detailed mechanism underlying the pathogenesis of indomethacin-induced colonic ulceration and inflammation remains to be elucidated. This study provides evidence that indomethacin treatment damages intestinal epithelial cell barrier integrity, increases gene expression of pro-inflammatory cytokines, increases protein expression of pNFκB, and affects microbiota composition. It is possible that indomethacin might affect the intestinal microflora and damage the epithelium, leading to the activation of inflammatory signaling pathways such as NFκB, which causes pro-inflammatory cytokines to be over-expressed and accumulate in the intestine. CGA might directly prevent indomethacin-induced activation of NFκB and its down-stream targets including certain cytokines, as numerous previous studies have demonstrated in certain rodent models of colitis (4, 7). Another possibility is that CGA could also indirectly affect the NFκB pathway via inhibition of certain upstream signals, such as changes in microbiota composition and related metabolites (7, 18).
Several studies have demonstrated the influence of CGA on gut microbiota composition. A previous study found that CGA increases the abundance of the phyla Firmicutes and Bacteroidetes in weaned piglets (19). The researchers suggested that the increased abundance of Bacteroidetes, which produces short-chain fatty acids (SCFAs) such as butyrate, would partially benefit intestinal function. Surprisingly, CGA decreased the abundance of the genus Bacteroides at the same time. The effects of CGA on the concentration of SCFAs align with another study on weaned piglets, which found that dietary CGA supplementation led to an increase in butyric acid in the intestine (8). However, a study on growing pigs did not show increased abundance of Firmicutes and Bacteroidetes (20). Unfortunately, the content of SCFAs was not determined, but they found that other metabolites such as 5-hydroxytryptamine increased, accompanied by changes in the microbiota composition of the colon. The effects of CGA on experimental colitis induced by dextran sulfate sodium were explored by another study, and microbiota was suggested to play a critical role in mediating the ameliorative effects of CGA (7). The proportions of Firmicutes and Bacteroidetes are decreased in colitis mice. Notably, a significantly increased proportion of Akkermansia, a mucin-degrading bacterium, is suggested to improve the mucus layer integrity. We found that dietary CGA increases the Firmicutes/Bacteroidetes ratio in indomethacin-treated mice, which is in accordance with the aforementioned results in several animal models of intestinal dysfunction. However, unlike previous studies, this study found that CGA affects Bacteroides abundance and decreases LPS content by decreasing the level of LPS-producing Bacteroides in the colon. The Bacteroides genus is sometimes associated with health conditions (21); some of its strains such as Bacteroides dorei and Bacteroides fragilis are suggested to promote the development of diseases such as diabetes and coeliac disease (22, 23). LPS is one of the major products of Bacteroides and may induce intestinal epithelial barrier dysfunction and inflammation (24). Notably, CGA had little effect on indomethacin-induced inflammation in the colons of antibiotic-treated mice, which suggests the prevention of intestinal inflammation by CGA could be largely influenced by the microbiota. Importantly, mice colonized with fecal microbiota from mice in the CA and CA-IND groups showed similar effects (intestine length, morphology and integrity, cecum Bacteroides abundance and LPS content, and colonic inflammation) as CGA did after indomethacin treatment. It can be inferred from these facts that the intestinal microbiota, primarily LPS-producing Bacteroides, mediate the effects of CGA on the prevention of indomethacin-induced inflammation.
In conclusion, it was found that CGA could protect against indomethacin-induced inflammation and mucosa damage. Importantly, the results also suggest that CGA may protect intestine integrity and alleviate inflammatory responses, primarily by inhibiting the growth of Bacteroides and the accumulation of Bacteroides-derived LPS. This newly identified mechanism broadens the base of knowledge on how CGA exerts its protective effects on intestinal damage, and may help to provide strategies for the prevention of gastrointestinal mucosal damage in patients treated with indomethacin.
Data Availability Statement
The raw data supporting the conclusion of this article will be made available by the authors, without undue reservation, to any qualified researchers.
Ethics Statement
The animal study was reviewed and approved by Institutional Animal Care and Use Committee of Hunan Agriculture University.
Author Contributions
YY and HY designed the experiment and drafted the manuscript. YY, XZ, and KG carried out the animal trials and sample analysis. FZ did data analysis work. HY was responsible for the integrity of the work as a whole. All authors reviewed and approved the final manuscript.
Funding
This work was supported by the Scientific Research Fund of the Hunan Provincial Education Department (No. 17C0123) and the Scientific Research Fund of the Hunan Provincial health and planning Business Committee (No. C2017010).
Conflict of Interest
The authors declare that the research was conducted in the absence of any commercial or financial relationships that could be construed as a potential conflict of interest.
Supplementary Material
The Supplementary Material for this article can be found online at: https://www.frontiersin.org/articles/10.3389/fimmu.2020.01125/full#supplementary-material
Supplementary Figure 1. A full image of Figure 2E.
References
1. Jin S, Chang C, Zhang L, Liu Y, Huang X, Chen Z. Chlorogenic acid improves late diabetes through adiponectin receptor signaling pathways in db/db mice. PLoS ONE. (2015) 10:e0120842. doi: 10.1371/journal.pone.0120842
2. Bhandarkar NS, Brown L, Panchal SK. Chlorogenic acid attenuates high-carbohydrate, high-fat diet-induced cardiovascular, liver, and metabolic changes in rats. Nutr Res. (2019) 62:78–88. doi: 10.1016/j.nutres.2018.11.002
3. Huang S, Wang LL, Xue NN, Li C, Guo HH, Ren TK, et al. Chlorogenic acid effectively treats cancers through induction of cancer cell differentiation. Theranostics. (2019) 9:6745–63. doi: 10.7150/thno.34674
4. Shin HS, Satsu H, Bae MJ, Zhao Z, Ogiwara H, Totsuka M, et al. Anti-inflammatory effect of chlorogenic acid on the IL-8 production in Caco-2 cells and the dextran sulphate sodium-induced colitis symptoms in C57BL/6 mice. Food Chem. (2015) 168:167–75. doi: 10.1016/j.foodchem.2014.06.100
5. O'Hara AM, Shanahan F. The gut flora as a forgotten organ. EMBO Rep. (2006) 7:688–93. doi: 10.1038/sj.embor.7400731
6. Mills CE, Tzounis X, Oruna-Concha MJ, Mottram DS, Gibson GR, Spencer JP. In vitro colonic metabolism of coffee and chlorogenic acid results in selective changes in human faecal microbiota growth. Br J Nutr. (2015) 113:1220–7. doi: 10.1017/S0007114514003948
7. Zhang Z, Wu X, Cao S, Cromie M, Shen Y, Feng Y, et al. Chlorogenic acid ameliorates experimental colitis by promoting growth of akkermansia in mice. Nutrients. (2017) 9:677. doi: 10.3390/nu9070677
8. Zhang Y, Wang Y, Chen D, Yu B, Zheng P, Mao X, et al. Dietary chlorogenic acid supplementation affects gut morphology, antioxidant capacity and intestinal selected bacterial populations in weaned piglets. Food Func. (2018) 9:4968–78. doi: 10.1039/C8FO01126E
9. Gonthier MP, Verny MA, Besson C, Remesy C, Scalbert A. Chlorogenic acid bioavailability largely depends on its metabolism by the gut microflora in rats. J Nutr. (2003) 133:1853–9. doi: 10.1093/jn/133.6.1853
10. Jurjus AR, Khoury NN, Reimund JM. Animal models of inflammatory bowel disease. J Pharmacol Toxicol Methods. (2004) 50:81–92. doi: 10.1016/j.vascn.2003.12.002
11. Wang K, Jin X, You M, Tian W, Le Leu RK, Topping DL, et al. Dietary propolis ameliorates dextran sulfate sodium-induced colitis and modulates the gut microbiota in rats fed a western diet. Nutrients. (2017) 9:875. doi: 10.3390/nu9080875
12. Li Q, Liang X, Zhao L, Zhang Z, Xue X, Wang K, et al. UPLC-Q-Exactive Orbitrap/MS-Based lipidomics approach to characterize lipid extracts from bee pollen and their in vitro anti-inflammatory properties. J Agric Food Chem. (2017) 65:6848–60. doi: 10.1021/acs.jafc.7b02285
13. He L, Long J, Zhou X, Liu Y, Li T, Wu X. Serine is required for the maintenance of redox balance and proliferation in the intestine under oxidative stress. FASEB J. (2020) 34:4702–17. doi: 10.1096/fj.201902690R
14. Liang N, Kitts DD. Amelioration of oxidative stress in caco-2 cells treated with pro-inflammatory proteins by chlorogenic acid isomers via activation of the Nrf2-Keap1-ARE-Signaling pathway. J Agric Food Chem. (2018) 66:11008–17. doi: 10.1021/acs.jafc.8b03983
15. Zhou Y, Ruan Z, Zhou L, Yang Y, Mi S, Deng Z, et al. Chlorogenic acid decreased intestinal permeability and ameliorated intestinal injury in rats via amelioration of mitochondrial respiratory chain dysfunction. Food Sci Biotechnol. (2016) 25:253–60. doi: 10.1007/s10068-016-0037-3
16. Ruan Z, Mi SM, Zhou LL, Zhou Y, Li J, Liu WH, et al. Chlorogenic acid enhances intestinal barrier by decreasing MLCK expression and promoting dynamic distribution of tight junction proteins in colitic rats. J Funct Foods. (2016) 26:698–708. doi: 10.1016/j.jff.2016.08.038
17. Maity P, Bindu S, Dey S, Goyal M, Alam A, Pal C, et al. Indomethacin, a non-steroidal anti-inflammatory drug, develops gastropathy by inducing reactive oxygen species-mediated mitochondrial pathology and associated apoptosis in gastric mucosa: a novel role of mitochondrial aconitase oxidation. J Biol Chem. (2009) 284:3058–68. doi: 10.1074/jbc.M805329200
18. Song J, Zhou N, Ma W, Gu X, Chen B, Zeng Y, et al. Modulation of gut microbiota by chlorogenic acid pretreatment on rats with adrenocorticotropic hormone induced depression-like behavior. Food Func. (2019) 10:2947–57. doi: 10.1039/C8FO02599A
19. Chen J, Yu B, Chen D, Zheng P, Luo Y, Huang Z, et al. Changes of porcine gut microbiota in response to dietary chlorogenic acid supplementation. Appl Microbiol Biotechnol. (2019) 103:8157–68. doi: 10.1007/s00253-019-10025-8
20. Wu Y, Liu W, Li Q, Li Y, Yan Y, Huang F, et al. Dietary chlorogenic acid regulates gut microbiota, serum-free amino acids and colonic serotonin levels in growing pigs. Int J Food Sci Nutr. (2018) 69:566–73. doi: 10.1080/09637486.2017.1394449
21. Ridaura VK, Faith JJ, Rey FE, Cheng J, Duncan AE, Kau AL, et al. Gut microbiota from twins discordant for obesity modulate metabolism in mice. Science. (2013) 341:1241214. doi: 10.1126/science.1241214
22. De Palma G, Nadal I, Medina M, Donat E, Ribes-Koninckx C, Calabuig M, et al. Intestinal dysbiosis and reduced immunoglobulin-coated bacteria associated with coeliac disease in children. BMC Microbiol. (2010) 10:63. doi: 10.1186/1471-2180-10-63
23. Davis-Richardson AG, Ardissone AN, Dias R, Simell V, Leonard MT, Kemppainen KM, et al. Bacteroides dorei dominates gut microbiome prior to autoimmunity in finnish children at high risk for type 1 diabetes. Front Microbiol. (2014) 5:678. doi: 10.3389/fmicb.2014.00678
Keywords: chlorogenic acid, indomethacin, inflammatory bowel disease, intestinal integrity, microbiota
Citation: Yan Y, Zhou X, Guo K, Zhou F and Yang H (2020) Chlorogenic Acid Protects Against Indomethacin-Induced Inflammation and Mucosa Damage by Decreasing Bacteroides-Derived LPS. Front. Immunol. 11:1125. doi: 10.3389/fimmu.2020.01125
Received: 21 March 2020; Accepted: 07 May 2020;
Published: 03 June 2020.
Edited by:
Kai Wang, Chinese Academy of Agricultural Sciences, ChinaReviewed by:
Deguang Song, Yale University School of Medicine, United StatesQingbiao Xu, Huazhong Agricultural University, China
Copyright © 2020 Yan, Zhou, Guo, Zhou and Yang. This is an open-access article distributed under the terms of the Creative Commons Attribution License (CC BY). The use, distribution or reproduction in other forums is permitted, provided the original author(s) and the copyright owner(s) are credited and that the original publication in this journal is cited, in accordance with accepted academic practice. No use, distribution or reproduction is permitted which does not comply with these terms.
*Correspondence: Hongqi Yang, csyhq@sina.com