- 1APHM, Service de Médecine Intensive et Réanimation, Réanimation Des Urgences, Hôpital la Timone, Marseille, France
- 2CEReSS - Center for Studies and Research on Health Services and Quality of Life EA3279, Aix-Marseille University, Marseille, France
- 3APHM, Hôpital de la Timone, Service d'Immunologie, Marseille Immunopôle, Marseille, France
- 4Aix Marseille Univ, CNRS, INSERM, CIML, Marseille, France
Background: Septic shock, a major cause of death in critical care, is the clinical translation of a cytokine storm in response to infection. It can be complicated by sepsis-induced immunosuppression, exemplified by blood lymphopenia, an excess of circulating Treg lymphocytes, and decreased HLA-DR expression on circulating monocytes. Such immunosuppression is associated with secondary infections, and higher mortality. The effect of these biological modifications on circulating innate lymphoid cells (ILCs) has been little studied.
Methods: We prospectively enrolled patients with septic shock (Sepsis-3 definition) in the intensive care unit (ICU) of Timone CHU Hospital. ICU controls (trauma, cardiac arrest, neurological dysfunction) were recruited at the same time (NCT03297203). We performed immunophenotyping of adaptive lymphocytes (CD3+ T cells, CD19+ B cells, CD4+CD25+FoxP3+ Treg lymphocytes), ILCs (CD3−CD56+ NK cells and helper ILCs – ILC1, ILC2, and ILC3), and monocytes by flow cytometry on fresh blood samples collected between 24 and 72 h after admission.
Results: We investigated adaptive and innate circulating lymphoid cells in the peripheral blood of 18 patients in septic shock, 15 ICU controls, and 30 healthy subjects. As expected, the peripheral blood lymphocytes of all ICU patients showed lymphopenia, which was not specific to sepsis, whereas those of the healthy volunteers did not. Circulating CD3+ T cells and CD3−CD56+ NK cells were mainly concerned. There was a tendency toward fewer Treg lymphocytes and lower HLA-DR expression on monocytes in ICU patients with sepsis. Although the ILC1 count was higher in septic patients than healthy subjects, ILC2, and ILC3 counts were lower in both ICU groups. However, ILC3s within the total ILCs were overrepresented in patients with septic shock. The depression of immune responses has been correlated with the occurrence of secondary infections. We did not find any differences in ILC distribution according to this criterion.
Conclusion: All ICU patients exhibit lymphopenia, regardless of the nature (septic or sterile) of the initial medical condition. Specific distribution of circulating ILCs, with an excess of ILC1, and a lack of ILC3, may characterize septic shock during the first 3 days of the disease.
Introduction
Sepsis and septic shock are major public health concerns. In the absence of therapeutic advances, septic shock is a leading cause of mortality in critically ill patients (1). Septic shock corresponds to an intense and uncontrolled systemic immune response to a bacterial or fungal infection (2). In the first hours, the activation of innate immunity leads to cytokine storm syndrome (CSS), which can lead to multiple organ failure and early death. In some survivors, secondary immune dysfunction can occur. Immunosuppression can lead to secondary infections, such as ventilator-associated pneumonia (VAP), which increases morbidity, and mortality (3). Immunosuppression is not specific to sepsis or septic shock in critical care. It involves both innate and adaptive immunity. The phagocytosis of peripheral blood neutrophils can be altered, leading to VAP (4), as reduced IFN-γ production by natural killer (NK) cells is associated with CMV reactivation (5). Activation markers, such as HLA-DR (MHC class II), on circulating monocytes are underexpressed in septic patients (6), especially in the later stages of the disease (> 72 h) (7), and this is associated with secondary infections, and mortality. Lymphopenia is also common in patients admitted for septic shock, with the loss of splenic CD4+ T cells (autopsy findings), and reduced levels of circulating B lymphocytes (8). Conversely, circulating regulatory T cells (Tregs) are always overrepresented 3 to 7 days after diagnosis of the infection (9), and promote anti-inflammatory responses during the second phase of sepsis (10).
Innate lymphoid cells (ILCs) are innate lymphocytes. ILC subgroups are defined according to their expression of key transcription factors and cytokine production, based on their similarity to T cells, and T helper (TH) cell subsets. Thus, ILCs can be classified as “cytotoxic” ILCs (bona fide NK cells) or as “helper” ILCs (ILC1, ILC2, and ILC3) (11, 12). Helper ILCs represent 0.1% of all circulating lymphocytes (13). The ILC1, ILC2, and ILC3 subsets are present mainly as sedentary cells in tissues, in which they can be maintained by self-renewal (14). Nevertheless, ILC subsets are detectable in human peripheral blood by flow cytometry by excluding lineage (Lin) positive cells (including CD3+ T cells, CD19+ B cells, CD94+ NK cells, and CD14+ monocyte/myeloid cells), and gating on cells that express the IL-7 receptor (CD127; Figure 3). Helper ILC1s require T-bet for development and produce IFN-γ as their main effector cytokine. ILC2s depend on GATA-3 and produce “Th2” cytokines (IL-4, IL-5, IL-9, and IL-13). They express CRTH2, a marker used in the gating strategy. ILC3s depend on RORγt, secrete “Th17” cytokines, such as IL-17, or “Th22” cytokines, such as IL-22, and express CD117 (gating strategy). Many studies have suggested an important role for helper ILCs in immunity, particularly in mouse models. A review on the role of ILCs in inflammatory diseases has been recently published (15). ILC1s are pro-inflammatory cells and may be involved in the pathogenesis of chronic obstructive pulmonary disease (16) and Crohn's disease (17). ILC2s may be involved in atopic diseases (18) and may promote fibrosis (19). ILC3s may be critical effector cells in psoriasis (20). ILC biology has never been well-studied in sepsis, even if they appear to be redundant for protective immunity in humans when T-cell, and B-cell functions are preserved (13). Here, we prospectively evaluated circulating immune cells by flow cytometry, especially circulating ILCs, and their three subsets during the early phase of septic shock, defined as the first 3 days after diagnosis of the infection.
Materials and Methods
Patients
Patients with septic shock in the Intensive Care Unit (ICU) of Timone CHU Hospital (Réanimation des Urgences, AP-HM Marseille, France) were prospectively enrolled between June and December 2017. According to the Sepsis-3 definition (21), septic shock was considered to be a bacterial infection responsible for arterial hypotension or hyperlactatemia without hypovolemia. All patients required norepinephrine infusion after adequate fluid resuscitation. Hydrocortisone was systemically added as symptomatic therapeutic support for these patients (200 mg/day) (22, 23). First, we compared septic patients to other patients hospitalized in the ICU for cardiac arrest, trauma, or neurological dysfunction (stroke, status epilepticus). These patients had no sign of infection on the day of inclusion. Exclusion criteria were age < 18 years, life expectancy < 48 h, and bone-marrow aplasia (no circulating lymphocytes). Thirty healthy volunteers were recruited in our laboratory to determine normal values of circulating ILCs. All patient data are shown in Table 1. We compared all ICU patients who could have severe tissue injuries vs. all ICU patients with less severe injuries to assess the impact of “aggression intensity” on the depression of immune biomarkers. We used the SOFA (Sepsis-related Organ Failure Assessment) score, which adds organ dysfunctions (hemodynamic respiratory, hepatic, renal, neurological, and hematopoietic dysfunctions), to define severe tissue injuries. We arbitrarily defined a SOFA score ≥ 8 as corresponding to severe tissue damage. Patient characteristics according to the SOFA score are shown in Table 2. Finally, we compared critically ill patients who had a secondary infection during their stay in the ICU vs. patients who did not (patient data in Table 3) to assess the role of “biomarkers depression” in the occurrence of secondary infections. The study protocol was approved by the Committee for the Protection of Persons North-West II—France and the trial was registered online before initiation (NCT03297203). Written informed consent was obtained from each patient.
Immunophenotyping by Flow Cytometry
Biological analyses were performed on fresh blood during the 6 h following blood-sample collection. Blood samples were all collected between 24 and 72 h after ICU admission for all ICU patients. For patients with septic shock, ICU admission corresponded to the diagnosis of infection and the initiation of antibiotics. The first 3 days were considered to be the early phase of the disease based on the clinical and biological systemic inflammatory response (cytokine storm, hemodynamic impairment, multiple organ failure) (24, 25). Lymphocyte populations (total lymphocytes, CD3+ T cells, CD4+ T cells, CD8+ T cells, CD19+ B cells, and CD3−CD56+ NK cells) were quantified using 6-Color BD Multitest and BD Trucount Technologies (Becton Dickinson, Le Pont de Claix, France), according to the manufacturer's instructions. We also determined the HLA-DR expression on circulating monocytes. Whole blood was incubated with BD Quantibrite PE Anti-HLA-DR (clone L243)/Anti-Monocyte Stain (clone MΦP9; Becton Dickinson, San Jose, CA, USA). The analysis was performed on a BD FACSCanto II™ cytometer using BD FACS DIVA Software. The amount of antibody bound per cell (Ab/cell) was calculated by standardizing the HLA-DR geometric mean fluorescence intensity (MFI) of monocytes to BD Quantibrite phycoerythrin (PE) beads (BD CellQuest™ Software). Peripheral blood mononuclear cells (PBMCs) were immediately collected by MSL (Eurobio) density centrifugation. PBMCs were used to identify regulatory T cells, considered to be CD4+CD25+FoxP3+ lymphocytes. The following antibodies were used: AmCyan anti-CD3 (Clone SK7), APC-H7 anti-CD4 (clone SK3), PerCP-Cy5.5 anti-CD8 (clone SK1), APC anti-CD25 (clone 2A3), Alexa Fluor 488 anti-FoxP3 (clone 259D/C7), and Alexa Fluor 488 anti-IgG1 (isotype-matched controls) (BD Biosciences). PBMCs were also used to characterize ILCs in peripheral blood. We used a panel of conventional lineage markers (Lin: CD3, CD19, CD14, TCRαβ, TCRγδ, CD94, CD16, FcεRI, CD34, CD123, and CD303) and cell-surface expression of CD127, CD117, and CRTH2 to identify the ILC1 subset as Lin− CD127+ CD117− CRTH2− cells, the ILC2 subset as Lin− CD127+ CRTH2+ cells, and the ILC3 subset as Lin− CD127+ CD117+ CRTH2− cells, among the circulating lymphocytes. The following antibodies were used: FITC anti-CD3 (clone UCTH1), FITC anti-CD19 (clone HIB19), FITC anti-CD14 (clone M5E2), FITC anti-TCRαβ (clone T10B9), FITC anti-TCRγδ (clone B1), FITC anti-CD94 (clone HP-3D9), FITC anti-CD16 (clone 3G8), FITC anti-CD34 (clone 581/CD34), FITC anti-CD123 (clone 7G3) (BD Pharmigen), FITC anti-CD303 (clone AC144) (Miltenyi Biotec), FITC anti-FcεRI (AER-37) (Ebioscience), PE-C7 anti-CD127 (HIL-7R-M21) (BD Pharmigen), Alexa Fluor 647 anti-CD294/CRTH2 (clone BM16) (BD Horizon), and PE-Cy5 anti-CD117 (clone YB5.B8) (BD Pharmigen). Data was acquired using a BD LSRFortessa™ cytometer and data analysis was performed using FlowJo 10.2 Software.
Statistical Analysis
The results are presented (text, tables, and figures) as the median [min, max]. For continuous variables, multiple group comparisons were analyzed using the Mann-Withney U-test for two groups and the Kruskal-Wallis test for more than two groups. For categorical variables, the Chi squared test was used. Statistical analyses were performed with Prism 6 (GraphPad Software, San Diego, CA, USA). Results were considered significant for a p < 0.05.
Results
Patient Characteristics (Tables 1−3)
Eighteen patients with septic shock were included (ICU Sepsis Group). The sources of infection and bacterial strains are detailed in Table 1. The ICU control group contained 15 patients (ICU Control Group): six cardiac arrests, five severe trauma, two strokes, one status epilepticus, and one voluntary overdose with benzodiazepine.
Patients with sepsis were slightly, but not significantly, older (57 years [29–77]) than the controls (50 years [21–80]; p = 0.12). Most patients were male (21/33), with no difference between the two groups (p = 0.74). The median SOFA score was higher for the Sepsis Group (8 [4–15]) than the ICU controls (6 [2–17]; p = 0.10). The same was true for the SAPSII (64 [28–76] vs. 40 [25–76], p = 0.22). According to the protocol for septic shock, all patients with sepsis received norepinephrine infusion with a median dosage of 3 mg/h [0.3–12], higher than for the controls, for whom norepinephrine infusion was from 1 mg/h [0–9] (p = 0.01). Only one patient in the ICU Sepsis Group required renal replacement therapy (RRT) vs. two in the control group (p = 0.44). Three quarters of the patients were mechanically ventilated (more in the sepsis group, 12 vs. 13, p = 0.18), with a median duration of mechanical ventilation of 3 days [0–40] in the sepsis group and 5 days [0–120] in the ICU control group (p = 0.13). Secondary infections in the ICU concerned seven patients with anterior septic shock and six ICU controls (p = 0.95). Secondary infections corresponded to 10 cases of VAP, one CRI, one case of cholecystitis, and one of C. difficile colitis. One patient with VAP also had CMV reactivation. These secondary infections occurred between day 2 and day 30 (median of seven days). Overall mortality at day 180 was 11/33 (33%) and was higher in the controls (6/15 vs. 5/18 deaths in the sepsis group, p = 0.46). The median length of stay in the ICU was 7 days and was nearly the same for the two groups (6 [2–38] for the sepsis group vs. 7 [2–20] for the control group, p = 0.72).
Immunological Analysis (Table 4)
Conventional Blood Lymphocyte Immunophenotyping
Most patients with septic shock develop lymphopenia (8, 26). Patients of the ICU groups developed lymphopenia, with no difference between the two groups (p = 0.90), whereas the lymphocyte counts of the healthy volunteers remained normal. The median lymphocyte count was 2,042/mm3 [708–3,606] for the healthy subjects vs. 992/mm3 [298–2,487] for the septic patients and 856/mm3 [298–2,246] for the ICU controls (p < 0.0001). Lymphopenia concerned all lymphocyte subsets, above all CD3+ T cells (TCD4+ and TCD8+), and CD3−CD56+ NK cells (Figure 1A). There was no statistical difference for the CD19+ B cell counts between the three groups. Patients with a secondary infection tended to have fewer circulating lymphocytes than those without, with no statistical difference (Table 4). The more extensive the tissue damage was (SOFA ≥ 8), the more pronounced was the deficit of circulating lymphocytes, with a correlation between a higher SOFA score, and lower lymphocyte counts (Rho (Spearman): −0.446 [IC 95%: −0.684, −0.121], p < 0.001). These results were only significant concerning CD4+ T cells and CD3−CD56+ NK cells (Figure 1B).
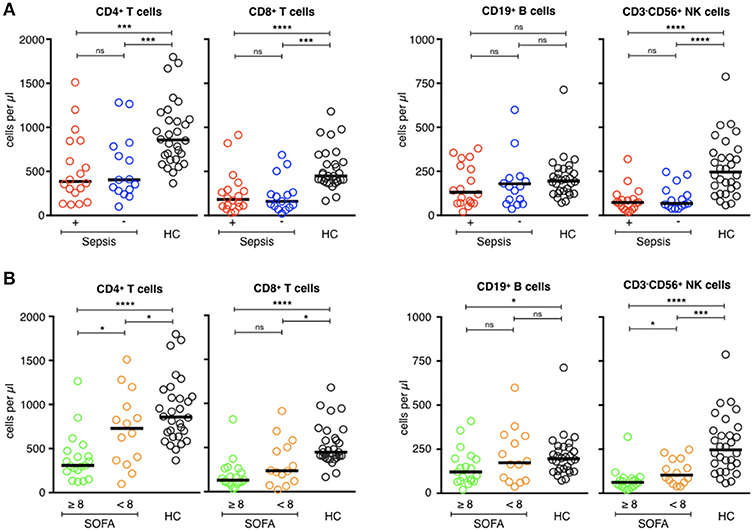
Figure 1. Phenotypic analysis of circulating lymphocyte subsets in ICU patients. (A) Comparison of helper T-cell, cytotoxic T-cell, B-cell, and NK-cell counts (cells/μl) of septic patients (red circles) with those of healthy controls (dark circles), and ICU patients without sepsis (blue circles). (B) Comparison of helper T-cell, cytotoxic T-cell, B-cell, and NK cell counts (cells/μl) of patients with severe tissue injuries (green circles) with those of healthy controls (dark circles), and patients with less severe lesions (orange circles). The bars show the median. Statistical analyses were performed using the Mann Whitney U-test. Differences were considered significant when P < 0.05: *P < 0.05, ***P < 0.001, ****P < 0.0001. ns: not significant.
Circulating Treg Lymphocytes
Treg lymphocytes are expected to be overrepresented in patients with septic shock and could promote the post-aggression anti-inflammatory response (9, 27). Here, the Treg counts were lower in patients with septic shock than the ICU controls (9/mm3 [1–80] vs. 17/mm3 [5–64], p = 0.04) (Figure 2A, top panel). There was no difference in the number of Treg cells in patients who developed secondary infections and those who did not (11/mm3 [3–38] and 14/mm3 [1–80], respectively, p = 0.67; Table 4). ICU patients who were more severely ill (SOFA ≥ 8) had fewer Treg lymphocytes than those who were less ill (SOFA < 8; 11/mm3 [3–34] vs. 17/mm3 [1–80], p = 0.04; Figure 2A, bottom panel).
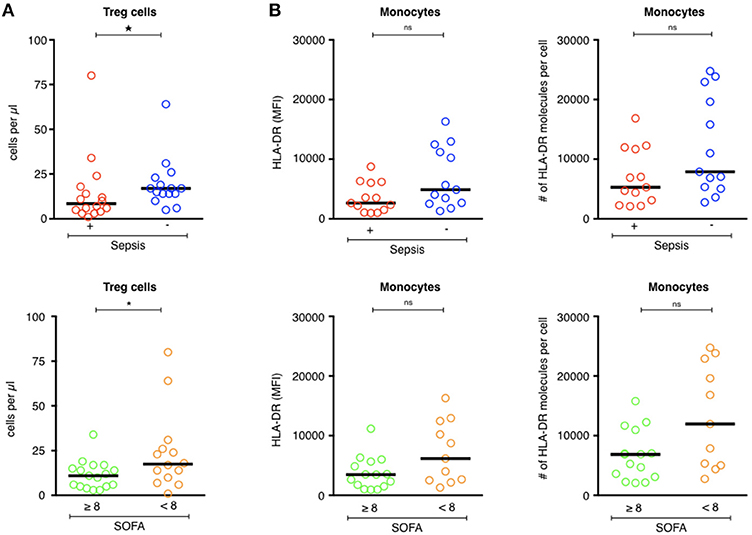
Figure 2. Phenotypic analysis of circulating Tregs and monocytes in ICU patients. (A, upper) Comparison of Treg cell counts (cells/μl) of septic patients (red circles) with those of ICU patients without sepsis (blue circles). (A, lower) Comparison of Treg cell counts (cells/μl) of patients with severe tissue injuries (green circles) with those of patients with less severe lesions (orange circles). (B, upper) Comparison of the MFI of HLA-DR expression and the number of HLA-DR molecules on monocytes of septic patients (red circles) with those of ICU patients without sepsis (blue circles). (B, lower) Comparison of the MFI of HLA-DR expression and the number of HLA-DR molecules on monocytes of patients with severe tissue injuries (green circles) with those of patients with less severe lesions (orange circles). The bars show the median. Statistical analyses were performed using the Mann Whitney U-test. Differences were considered significant when P < 0.05: *P < 0.05. ns: not significant.
HLA-DR Expression by Circulating Monocytes
HLA-DR expression on circulating monocytes is frequently used as a marker for the monitoring of immune alterations in critically ill patients, especially those with septic shock (6, 7, 28–31). As expected, HLA-DR expression was lower on circulating monocytes of patients with septic shock (MFI = 2,649 [959–8,752]—HLA-DR Ab/cell = 5,286 [2,089–16,832]) than those of the ICU controls (MFI = 4,882 [1,314–16,298]—HLA-DR Ab/cell = 7,882 [2,760–24,756]; p = 0.08; Figure 2B, top panel). There was no difference in HLA-DR expression between patients with secondary infections and those who were uninfected (MFI = 3,702 [959–12,941]—Ab/cell = 6,044 [2,089–24,756] for secondarily infected patients vs. MFI = 3,533 [1,048–16,298]—Ab/cell = 6,959 [2,257–23,846] for patients without secondary infection, p = 0.5; Table 4). Less ill patients (SOFA < 8) showed higher HLA-DR expression on their circulating monocytes than those who were more ill (SOFA > 8; Figure 2B, bottom panel), without statistical significance.
Circulating ILCs
Circulating ILCs have never been studied in patients with septic shock. As tissue-resident cells, they represent one of the first immune gates encountered by the infection and participate in the innate immune response through the production and release of pro-inflammatory cytokines (32–39). Total ILCs are defined as lineage-negative CD127+ lymphocytes (Figure 3). Among total ILCs, ILC subsets can be discriminated according to the expression of CD294 (CRTH2) and CD117 (cKit): ILC2s are Lin−CD127+CRTH2+ cells, ILC3 are Lin−CD127+CRTH2−CD117+ cells, and ILC1 are Lin−CD127+CRTH2−CD117− cells. ILCs in critically ill patients are not spared by the global deficit in circulating lymphocytes. Total ILC counts were slightly lower in both ICU groups than in healthy controls (1,293/mL [483–4,468], 1,242/mL [326–4,085], and 1,632/mL [505–5,846], respectively).
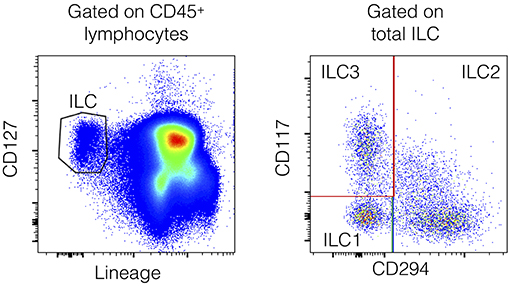
Figure 3. Flow cytometry gating strategy for the identification of human peripheral blood ILCs. ILCs are defined as Lin−CD127+ cells with a lineage cocktail containing antibodies directed against CD3, CD19, CD14, TCRαβ, TCRγδ, CD94, CD16, FcεRI, CD34, CD123, and CD303 (Left). Within the ILC gate, ILC2s are CD294+ cells, whereas ILC1s are CD294−CD117− cells and ILC3s are CD294−CD117+ cells (Right).
However, the ILC distribution was not the same in the two ICU groups (Figure 4A). Septic patients had more circulating ILC1s (931/mL [282–3,478]) than the ICU controls (512/mL [133–2,978]) and, above all, than the healthy volunteers (468/mL [178–1,980], p = 0.04). The proportion of ILC1s within total ILCs followed the same pattern: 66% [32–96] of circulating ILC1s in the sepsis group, 64% [27–83] in the ICU controls, and 30% [13-71] in the healthy controls (p < 0.0001). Balancing the excess of ILC1s, the ILC2, and ILC3 counts and percentages were significantly lower in both ICU groups than the healthy volunteers. The number of ILC2s in septic patients was 239/mL [29–1,592] (20% [3–58] of total ILC) vs. 147/mL [39–791] (14% [3–43] of total ILCs) in ICU controls and 584/mL [117–1,597] (28% [14–66] of total ILCs) in healthy subjects. The most significant finding for patients with septic shock was a severe deficit in the ILC3 subset (162/mL [41-469] or 13% [2–31]) relative to both the healthy subjects (513/mL [83–2,424], p < 0.0001 or 32% [12–55], p < 0.0001), and critically ill controls (258/mL [71–1,460], p = 0.2 or 25% [8–49] p = 0.04). Finally, we compared ILCs and ILC subsets between patients who had a secondary infection and those who did not (Table 4). There was no statistical difference between the two groups based on this criterion. Similarly, there was no difference according to the severity of tissue damage (SOFA ≥ 8 or SOFA < 8) (Figure 4B).
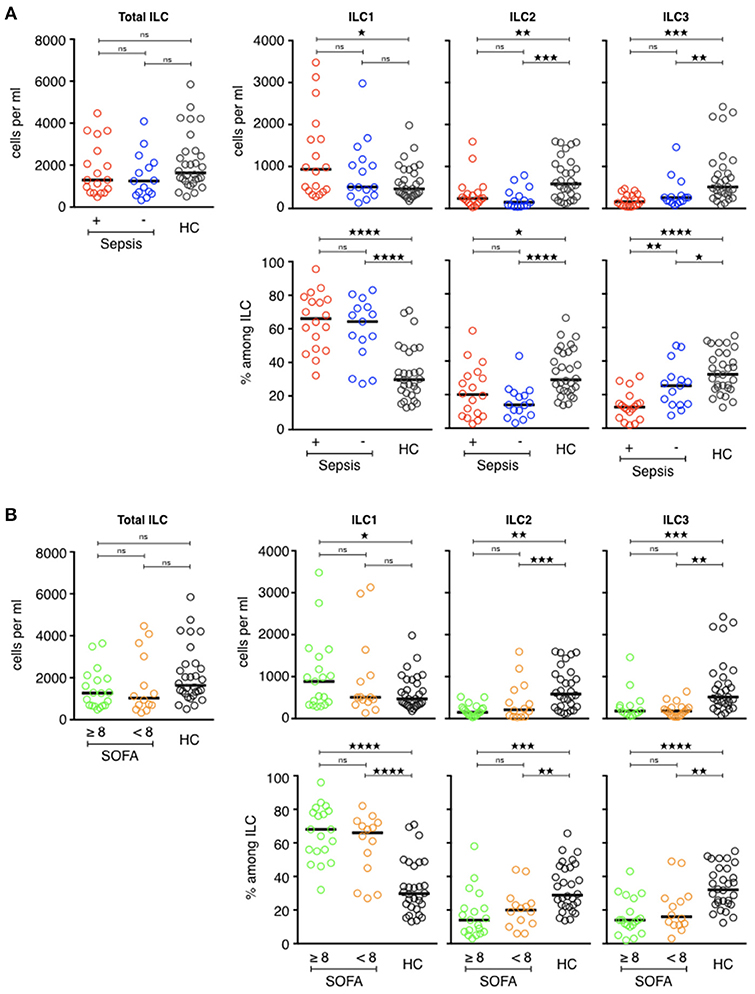
Figure 4. Phenotypic analysis of circulating ILCs in ICU patients. (A, upper) Comparison of total ILC, ILC1, ILC2, and ILC3 counts (cells/ml) of septic patients (red circles) with those of healthy controls (dark circles) and ICU patients without sepsis (blue circles). (A, lower) Distribution of each ILC subset among total ILCs. (B, upper) Comparison of total ILC, ILC1, ILC2, and ILC3 counts (cells/ml) of patients with severe tissue injuries (green circles) with those of healthy controls (dark circles) and patients with less severe lesions (orange circles). (B, lower) Distribution of each ILC subset among total ILCs. The bars show the median. Statistical analyses were performed using the Mann Whitney U-test. Differences were considered significant when P < 0.05: *P < 0.05, **P < 0.01, ***P < 0.001, ****P < 0.0001. ns: not significant.
Discussion
Lymphopenia is common in critically ill patients, especially when the reason for admission is septic shock. The immune deficit following sepsis is called sepsis-induced immunosuppression (25). Other pathological situations, such as trauma (40), or extensive burns (41), can induce the same biological modifications. These situations can all be complicated by “opportunistic” or, more precisely, secondary infections. Here, we confirmed that acute injuries (sepsis, trauma, cardiac arrest) are associated with circulating lymphopenia, which affected all lymphocyte subsets, above all circulating T cells, NK cells, and helper ILCs. Treg lymphocytes were also affected. This appears to contradict previous reports (9), but an examination of the results of Venet et al. show the absolute number of Treg lymphocytes to be lower in septic-ICU patients than in healthy subjects. No ICU controls were considered. Only the percentage of Treg lymphocytes among all CD4+ T cells was higher in patients with septic shock, although we did not find this result. The method to identify Treg cells was also not the same as in our study (reagents and samples, whole blood in their study vs. PBMCs in ours). In ICU patients, the deficit in ILCs (as Treg lymphocytes) could be explained by the global lymphopenia.
We also confirmed that lymphopenia is not specific to sepsis. Ischemia-reperfusion (after a cardiac arrest for example), traumatic injuries, or major surgical procedures (42–44) led to the same biological states. Our results are however limited by the small number of patients and our monocentric recruitment. Although “ICU immunosuppression” is not specific to sepsis, it appears to correlate with the severity of the tissue injuries. Comparison of patients with more severe tissue damage (arbitrarily defined by a SOFA score ≥ 8) with those with less severe lesions (arbitrarily defined by a SOFA score < 8) showed that the most ill patients showed a lower count of circulating lymphocytes and lower HLA-DR expression on circulating monocytes. However, there were no major differences in terms of circulating ILCs and their three subsets. It is possible that choosing a SOFA score ≥ 8 to define the severity of tissue injuries may have been somewhat arbitrary. However, the SOFA score adds all organ dysfunction and their intensity. Moreover, the prognosis of these two groups of patients was very different in terms of mortality (2/14 (14%) vs. 9/19 (47%), p = 0.046; Table 2). After a severe injury, lymphocyte apoptosis must occur to control inflammation. The initial cytokine storm is subsequent to overstimulation of the innate immune system (45). Pathogen-associated molecular patterns (PAMPs—microbial patterns) and damage-associated molecular patterns (DAMPs—“sterile” patterns) (46) bind to their Toll-like receptors (TLRs) to start an effective immune response to aggression. The resolution of inflammation requires negative feedback involving lymphocyte apoptosis and “tolerization” (47). Lymphocyte apoptosis is the main mechanism leading to lymphopenia in the ICU (40, 48, 49). The more severe the organ damages are, the greater is the deficit in lymphocytes and the resulting so-called “secondary immunosuppression.”
We also observed the absence of a correlation between the depression of biological immune markers (for example, circulating lymphocytes) and the occurrence of secondary infections. This observation contradicts published reports (50, 51). These contradictory findings can be explained by the timing of the blood sample collection. Our biological samples were collected relatively early after the onset of critical illness (24 to 72 h), whereas previous studies used samples taken later (>72 h after the initial infection) (7, 9). It is likely that the risk of secondary infection correlates more with the persistence of an immune deficit than the immune deficit itself, especially in the first hours of care. Even though immunosuppression may be a risk factor (52), the mechanism behind secondary infections appears to be more complex than a simple deficit of several biomarkers (53). In the ICU, comorbidities, length of stay, and the retention of invasive materials can lead to nosocomial infections. Mechanical ventilation can promote VAP and the retention of a central venous catheter can promote catheter-related infections (CRI). For example, in our study, the median length of stay was 18 days [2–60] for secondarily infected patients vs. 4 days [2–120] for uninfected subjects (p = 0.001). The length of stay may have been the consequence of secondary infections but an extended length of stay may have also promoted it.
Among all immune modifications, the distribution of circulating ILCs and their subsets appear to show some specificity for the critically ill patients with septic shock. These patients showed a greater proportion of ILC1s balanced by a lower proportion of ILC3s. This result for the ILC1 subset contradicts the recent findings of Cruz-Zárate et al. (49). However, there were differences between their study and ours. Our patients were all in septic shock (infusion of norepinephrine), whereas theirs were mainly in sepsis. Moreover, the distribution of ILCs in their healthy controls is surprising. Although an equal distribution between the ILC subsets has been reported (13, 54), ~85% of the ILCs in their study belonged to the ILC1 subset.
ILC1 is characterized by its ability to produce IFN- γ, which plays a role in the fight against bacterial infections. The ILC1 subset has already been shown to play a role in microbial infections, such as C. difficile or rodentium (33, 35) colitis and T gondii invasion (34) in mouse models. The lack of the ILC3 subset in sepsis suggests that these cells may represent a pool of future and effective ILC1s (ex-ILC3). Lim et al. recently showed (55) that cultured human peripheral blood CD7+CD127+CD117+ cells (ILC3) can give rise to both mature cytotoxic NK and helper ILC subsets, showing an important role for ILC3 in ILC-poiesis (56). ILCs are highly plastic cells (57, 58), which can change phenotype and function depending on their microenvironment. In animals, ILC2s and ILC3s exposed to IL-12 loose the expression of GATA-3 and RORγt, respectively, and acquire features of ILC1s, including T-bet expression and IFN-γ production (59). In the early phase of septic shock, the increase in the pool of ILC1s, originating from ILC3s (ILC1 precursors), may promote the pro-inflammatory response to eliminate the pathogen. Concerning the risk of secondary infections due to the lack of circulating ILCs, our study revealed no significant differences between secondarily infected and uninfected patients, although there was a trend toward a lower proportion of ILC1s and higher proportion of ILC2s in secondarily infected patients (Table 3). ILC2s have been shown to play a role in the resolution of inflammation in ischemic-reperfusion (60) and central nervous system inflammation (61) models. However, they are not “infection models.” In a mouse model of E. faecalis infection after burn injury (62), ILC2s had a detrimental role in sepsis and the use of an inhibitor of ILC2 development improved survival. In another study, ILC2s were protective against acute lung injury in a sepsis-induced acute lung inflammation model (63). Shifting the balance in favor of ILC2s (anti-inflammatory) vs. ILC1s (pro-inflammatory) would bear the risk of promoting “immunosuppression” and secondary infections in the ICU.
The study of ILCs in humans is made difficult because of their mostly being located in mucosal tissues (64). Circulating ILCs are considered to be immature relative to tissue-resident ILCs. In humans, the mechanisms by which ILCs circulate between peripheral blood and tissues are still unknown; it is unknown whether a deficit in circulating ILCs is associated with an equal deficiency in tissues. Nevertheless, peripheral blood is the main biological compartment available in humans to analyze the immune response and we show, for the first time, a disequilibrium in the distribution of ILC subsets in patients with septic shock, in which ILCs could participate in the pro-inflammatory immune response and may account for certain immunological post-injury modifications.
Data Availability
All datasets generated for this study are included in the manuscript/supplementary files.
Ethics Statement
This study protocol was approved by the Committee for the protection of persons West-North II - France - and the trial was registered online before initiation (NCT03297203).
Author Contributions
JC and FV devised and supervised the study, designed the research, and wrote the manuscript, with the help of the other co-authors. JC, CP, NB, CF, and CD designed the research, performed experiments, and analyzed the data. JB and MG provided key expertise and samples.
Funding
This work was supported by APHM, MSDAvenir, and institutional grants to the CIML (INSERM, CNRS, and Aix- Marseille University) and to Marseille Immunopole.
Conflict of Interest Statement
The authors declare that the research was conducted in the absence of any commercial or financial relationships that could be construed as a potential conflict of interest.
References
1. Besen BA, Romano TG, Nassar AP Jr, Taniguchi LU, Azevedo LC, Mendes PV, et al. Sepsis-3 definitions predict ICU mortality in a low-middle-income country. Ann Intens Care. (2016) 6:107. doi: 10.1186/s13613-016-0204-y
2. Mira JC, Gentile LF, Mathias BJ, Efron PA, Brakenridge SC, Mohr AM, et al. Sepsis pathophysiology, chronic critical illness, and persistent inflammation-immunosuppression and catabolism syndrome. Crit Care Med. (2017) 45:253–62. doi: 10.1097/CCM.0000000000002074
3. Pelekanou A, Tsangaris I, Kotsaki A, Karagianni V, Giamarellou H, Armaganidis A, et al. Decrease of CD4-lymphocytes and apoptosis of CD14-monocytes are characteristic alterations in sepsis caused by ventilator-associated pneumonia: results from an observational study. Crit Care. (2009) 13:R172. doi: 10.1186/cc8148
4. Conway Morris A, Kefala K, Wilkinson TS, Dhaliwal K, Farrell L, Walsh T, et al. C5a mediates peripheral blood neutrophil dysfunction in critically ill patients. Am J Respir Crit Care Med. (2009)180:19–28. doi: 10.1164/rccm.200812-1928OC
5. Chiche L, Forel JM, Thomas G, Farnarier C, Cognet C, Guervilly C, et al. Interferon-γ production by natural killer cells and cytomegalovirus in critically ill patients. Crit Care Med. (2012) 40:3162–9. doi: 10.1097/CCM.0b013e318260c90e
6. Drewry AM, Ablordeppey EA, Murray ET, Beiter ER, Walton AH, Hall MW, et al. Comparison of monocyte human leukocyte antigen-DR expression and stimulated tumor necrosis factor alpha production as outcome predictors in severe sepsis: a prospective observational study. Crit Care. (2016) 20:334. doi: 10.1186/s13054-016-1505-0
7. Landelle C, Lepape A, Voirin N, Tognet E, Venet F, Bohé J, et al. Low monocyte human leukocyte antigen-DR is independently associated with nosocomial infections after septic shock. Intens Care Med. (2010) 36:1859–66. doi: 10.1007/s00134-010-1962-x
8. Hotchkiss RS, Tinsley KW, Swanson PE, Schmieg RE Jr, Hui JJ, Chang KC, et al. Sepsis-induced apoptosis causes progressive profound depletion of B and CD4+ T lymphocytes in humans. J Immunol. (2001) 166:6952–63. doi: 10.4049/jimmunol.166.11.6952
9. Venet F, Chung CS, Kherouf H, Geeraert A, Malcus C, Poitevin F, et al. Increased circulating regulatory T cells (CD4(+)CD25 (+)CD127 (-)) contribute to lymphocyte anergy in septic shock patients. Intensive Care Med. (2009) 35:678–86. doi: 10.1007/s00134-008-1337-8
10. Faivre V, Lukaszewicz AC, Alves A, Charron D, Payen D, Haziot A. Human monocytes differentiate into dendritic cells subsets that induce anergic and regulatory T cells in sepsis. PLoS ONE. (2012) 7:e47209. doi: 10.1371/journal.pone.0047209
11. Spits H, Di Santo JP. The expanding family of innate lymphoid cells: regulators and effectors of immunity and tissue remodeling. Nat Immunol. (2011) 12:21–7. doi: 10.1038/ni.1962
12. Vivier E, Artis D, Colonna M, Diefenbach A, Di Santo JP, Eberl G, et al. Innate lymphoid cells: 10 years on. Cell. (2018) 174:1054–66. doi: 10.1016/j.cell.2018.07.017
13. Vély F, Barlogis V, Vallentin B, Neven B, Piperoglou C, Perchet T, et al. Evidence of innate lymphoid cell redundancy in humans. Nat Immunol. (2016) 17:1291–9. doi: 10.1038/ni.3553
14. Gasteiger G, Fan X, Dikiy S, Lee SY, Rudensky AY. Tissue residency of innate lymphoid cells in lymphoid and nonlymphoid organs. Science. (2015) 350:981–5. doi: 10.1126/science.aac9593
15. Ebbo M, Crinier A, Vély F, Vivier E. Innate lymphoid cells: major players in inflammatory diseases. Nat Rev Immunol. (2017) 17:665–78. doi: 10.1038/nri.2017.86
16. Silver JS, Kearley J, Copenhaver AM, Sanden C, Mori M, Yu L, et al. Inflammatory triggers associated with exacerbations of COPD orchestrate plasticity of group 2 innate lymphoid cells in the lungs. Nat Immunol. (2016) 17:626–35. doi: 10.1038/ni.3443
17. Bernink JH, Peters CP, Munneke M, te Velde AA, Meijer SL, Weijer K, et al. Human type 1 innate lymphoid cells accumulate in inflamed mucosal tissues. Nat Immunol. (2013) 14:221–9. doi: 10.1038/ni.2534
18. Smith SG, Chen R, Kjarsgaard M, Huang C, Oliveria JP, O'Byrne PM, et al. Increased numbers of activated group 2 innate lymphoid cells in the airways of patients with severe asthma and persistent airway eosinophilia. J Allergy Clin Immunol. (2016) 137:75–86.e8. doi: 10.1016/j.jaci.2015.05.037
19. Wohlfahrt T, Usherenko S, Englbrecht M, Dees C, Weber S, Beyer C, et al. Type 2 innate lymphoid cell counts are increased in patients with systemic sclerosis and correlate with the extent of fibrosis. Ann Rheum Dis. (2016) 75:623–6. doi: 10.1136/annrheumdis-2015-207388
20. Teunissen MBM, Munneke JM, Bernink JH, Spuls PI, Res PCM, Te Velde A, et al. Composition of innate lymphoid cell subsets in the human skin: enrichment of NCR(+) ILC3 in lesional skin and blood of psoriasis patients. J Invest Dermatol. (2014) 134:2351–60. doi: 10.1038/jid.2014.146
21. Shankar-Hari M, Phillips GS, Levy ML, Seymour CW, Liu VX, Deutschman CS, et al. Developing a new definition and assessing new clinical criteria for septic shock: for the third international consensus definitions for sepsis and septic shock (Sepsis-3). JAMA. (2016) 315:775–87. doi: 10.1001/jama.2016.0289
22. Annane D, Renault A, Brun-Buisson C, Megarbane B, Quenot JP, Siami S, et al. Hydrocortisone plus fludrocortisone for adults with septic shock. N Engl J Med. (2018) 378:809–18. doi: 10.1056/NEJMoa1705716
23. Venkatesh B, Finfer S, Cohen J, Rajbhandari D, Arabi Y, Bellomo R, et al. Adjunctive glucocorticoid therapy in patients with septic shock. N Engl J Med. (2018) 378:797–808. doi: 10.1056/NEJMoa1705835
24. Hotchkiss RS, Karl IE. The pathophysiology and treatment of sepsis. N Engl J Med. (2003). 348:138–50. doi: 10.1056/NEJMra021333
25. Hotchkiss RS, Monneret G, Payen D. Sepsis-induced immunosuppression: from cellular dysfunctions to immunotherapy. Nat Rev Immunol. (2013) 13:862–74. doi: 10.1038/nri3552
26. Boomer JS, To K, Chang KC, Takasu O, Osborne DF, Walton AH, et al. Immunosuppression in patients who die of sepsis and multiple organ failure. JAMA. (2011) 306:2594–605. doi: 10.1001/jama.2011.1829
27. Nascimento DC, Melo PH, Piñeros AR, Ferreira RG, Colón DF, Donate PB, et al. IL-33 contributes to sepsis-induced long-term immunosuppression by expanding the regulatory T cell population. Nat Commun. (2017) 8:14919. doi: 10.1038/ncomms14919
28. Meisel C, Schefold JC, Pschowski R, Baumann T, Hetzger K, Gregor J, et al. Granulocyte-macrophage colony-stimulating factor to reverse sepsis-associated immunosuppression: a double-blind, randomized, placebo-controlled multicenter trial. Am J Respir Crit Care Med. (2009) 180:640–8. doi: 10.1164/rccm.200903-0363OC
29. Cazalis MA, Friggeri A, Cavé L, Demaret J, Barbalat V, Cerrato E, et al. Decreased HLA-DR antigen-associated invariant chain (CD74) mRNA expression predicts mortality after septic shock. Crit Care. (2013) 17:R287. doi: 10.1186/cc13150
30. Monneret G, Venet F. Monocyte HLA-DR in sepsis: shall we stop following the flow? Crit Care. (2014) 18:102. doi: 10.1186/cc13179
31. Zorio V, Venet F, Delwarde B, Floccard B, Marcotte G, Textoris J, et al. Assessment of sepsis-induced immunosuppression at ICU discharge and 6 months after ICU discharge. Ann Intens Care. (2017) 7:80. doi: 10.1186/s13613-017-0304-3
32. Satoh-Takayama N, Vosshenrich CA, Lesjean-Pottier S, Sawa S, Lochner M, Rattis F, et al. Microbial flora drives interleukin 22 production in intestinal NKp46+ cells that provide innate mucosal immune defense. Immunity. (2008) 29:958–70. doi: 10.1016/j.immuni.2008.11.001
33. Satoh-Takayama N, Dumoutier L, Lesjean-Pottier S, Ribeiro VS, Mandelboim O, Renauld JC, et al. The natural cytotoxicity receptor NKp46 is dispensable for IL-22-mediated innate intestinal immunedefense against citrobacter rodentium. J Immunol. (2009) 183:6579–87. doi: 10.4049/jimmunol.0901935
34. Klose CS, Flach M, Möhle L, Rogell L, Hoyler T, Ebert K, et al. Differentiation of type 1 ILCs from a common progenitor to all helper-like innate lymphoid cell lineages. Cell. (2014) 157:340–56. doi: 10.1016/j.cell.2014.03.030
35. Abt MC, Lewis BB, Caballero S, Xiong H, Carter RA, Sušac B, et al. Innate immune defenses mediated by two ILC subsets are critical for protection against acute clostridium difficile infection. Cell Host Microbe. (2015) 18:27–37. doi: 10.1016/j.chom.2015.06.011
36. Sonnenberg GF, Artis D. Innate lymphoid cells in the initiation, regulation and resolution of inflammation. Nat Med. (2015) 21:698–708. doi: 10.1038/nm.3892
37. Quatrini L, Wieduwild E, Guia S, Bernat C, Glaichenhaus N, Vivier E, et al. Host resistance to endotoxic shock requires the neuroendocrine regulation of group 1 innate lymphoid cells. J Exp Med. (2017) 214:3531–41. doi: 10.1084/jem.20171048
38. Chun TT, Chung CS, Fallon EA, Hutchins NA, Clarke E, Rossi AL, et al. Group 2 innate lymphoid cells (ILC2s) are key mediators of the inflammatory response in polymicrobial sepsis. Am J Pathol. (2018) 188:2097–108. doi: 10.1016/j.ajpath.2018.05.009
39. Monticelli LA, Sonnenberg GF, Abt MC, Alenghat T, Ziegler CG, Doering TA, et al. Innate lymphoid cells promote lung-tissue homeostasis after infection with influenza virus. Nat Immunol. (2011) 12:1045–54. doi: 10.1038/ni.2131
40. Hotchkiss RS, Schmieg RE Jr, Swanson PE, Freeman BD, Tinsley KW, Cobb JP, et al. Rapid onset of intestinal epithelial and lymphocyte apoptotic cell death in patients with trauma and shock. Crit Care Med. (2000) 28:3207–17. doi: 10.1097/00003246-200009000-00016
41. Zhang F, Qiu XC, Wang JJ, Hong XD, Wang GY, Xia ZF. Burn-related dysregulation of inflammation and immunity in experimental and clinical studies. J Burn Care Res. (2017) 38:e892–9. doi: 10.1097/BCR.0000000000000511
42. Sasajima K, Inokuchi K, Onda M, Miyashita M, Okawa KI, Matsutani T, et al. Detection of T cell apoptosis after major operations. Eur J Surg. (1999) 165:1020–3. doi: 10.1080/110241599750007829
43. Nishiguchi K, Okuda J, Toyoda M, Tanaka K, Tanigawa N. Comparative evaluation of surgical stress of laparoscopic and open surgeries for colorectal carcinoma. Dis Colon Rectum. (2001) 44:223–30. doi: 10.1007/BF02234297
44. Edwards MR, Sultan P, del Arroyo AG, Whittle J, Karmali SN, Moonesinghe SR, et al. Metabolic dysfunction in lymphocytes promotes postoperative morbidity. Clin Sci. (2015) 129:423–37. doi: 10.1042/CS20150024
45. Chousterman BG, Swirski FK, Weber GF. Cytokine storm and sepsis disease pathogenesis. Semin Immunopathol. (2017) 39:517–28. doi: 10.1007/s00281-017-0639-8
46. Chen GY, Nuñez G. Sterile inflammation: sensing and reacting to damage. Nat Rev Immunol. (2010) 10:826–37. doi: 10.1038/nri2873
47. Seeley JJ, Baker RG, Mohamed G, Bruns T, Hayden MS, Deshmukh SD, et al. Induction of innate immune memory via microRNA targeting of chromatin remodelling factors. Nature. (2018) 559:114–9. doi: 10.1038/s41586-018-0253-5
48. Hotchkiss RS, Nicholson DW. Apoptosis and caspases regulate death and inflammation in sepsis. Nat Rev Immunol. (2006) 6:813–22. doi: 10.1038/nri1943
49. Cruz-Zárate D, Cabrera-Rivera GL, Ruiz-Sánchez BP, Serafín-López J, Chacón-Salinas R, López-Macías C, et al. Innate lymphoid cells have decreased HLA-DR expression but retain their responsiveness to TLR ligands during sepsis. J Immunol. (2018) 201:3401–10. doi: 10.4049/jimmunol.1800735
50. Monneret G, Lepape A, Voirin N, Bohé J, Venet F, Debard AL, et al. Persisting low monocyte human leukocyte antigen-DR expression predicts mortality in septic shock. Intensive Care Med. (2006) 32:1175–83. doi: 10.1007/s00134-006-0204-8
51. Grimaldi D, Louis S, Pène F, Sirgo G, Rousseau C, Claessens YE, et al. Profound and persistent decrease of circulating dendritic cells is associated with ICU-acquired infection in patients with septic shock. Intensive Care Med. (2011) 37:1438–46. doi: 10.1007/s00134-011-2306-1
52. van Vught LA, Wiewel MA, Hoogendijk AJ, Frencken JF, Scicluna BP, Klein Klouwenberg PMC, et al. The host response in patients with sepsis developing intensive care unit-acquired secondary infections. Am J Respir Crit Care Med. (2017) 196:458–70. doi: 10.1164/rccm.201606-1225OC
53. van Vught LA, Klein Klouwenberg PM, Spitoni C, Scicluna BP, Wiewel MA, Horn J, et al. Incidence, risk factors, and attributable mortality of secondary infections in the intensive care unit after admission for sepsis. JAMA. (2016) 315:1469–79. doi: 10.1001/jama.2016.2691
54. Hazenberg MD, Spits H. Human innate lymphoid cells. Blood. (2014) 124:700–9. doi: 10.1182/blood-2013-11-427781
55. Lim AI, Li Y, Lopez-Lastra S, Stadhouders R, Paul F, Casrouge A, et al. Systemic human ILC precursors provide a substrate for tissue ILC differentiation. Cell. (2017) 168:1086–100.e10. doi: 10.1016/j.cell.2017.02.021
56. Lim AI, Di Santo JP. ILC-poiesis: ensuring tissue ILC differentiation at the right place and time. Eur J Immunol. (2019) 49:11–8. doi: 10.1002/eji.201747294
57. Bonelli M, Shih HY, Hirahara K, Singelton K, Laurence A, Poholek A, et al. Helper T cell plasticity: impact of extrinsic and intrinsic signals on transcriptomes and epigenomes. Curr Top Microbiol Immunol. (2014) 381:279–326. doi: 10.1007/82_2014_371
58. Lim AI, Verrier T, Vosshenrich CA, Di Santo JP. Developmental options and functional plasticity of innate lymphoid cells. Curr Opin Immunol. (2017) 44:61–8. doi: 10.1016/j.coi.2017.03.010
59. Vonarbourg C, Mortha A, Bui VL, Hernandez PP, Kiss EA, Hoyler T, et al. Regulated expression of nuclear receptor RORγt confers distinct functional fates to NK cell receptor-expressing RORγt(+) innate lymphocytes. Immunity. (2010) 33:736–51. doi: 10.1016/j.immuni.2010.10.017
60. Huang Q, Niu Z, Tan J, Yang J, Liu Y, Ma H, et al. IL-25 Elicits innate lymphoid cells and multipotent progenitor type 2 cells that reduce renal ischemic/reperfusion injury. J Am Soc Nephrol. (2015) 26:2199–211. doi: 10.1681/ASN.2014050479
61. Russi AE, Walker-Caulfield ME, Ebel ME, Brown MA. Cutting edge: c-Kit signaling differentially regulates type 2 innate lymphoid cell accumulation and susceptibility to central nervous system demyelination in male and female SJL mice. J Immunol. (2015)194:5609–13. doi: 10.4049/jimmunol.1500068
62. Ito I, Bhopale KK, Kobayashi M, Finnerty CC, Herndon DN, Suzuki F. Lamina propria group 2 innate lymphoid cells impair the antibacterial defense of burned mice to enterococcal translocation. J Leukoc Biol. (2017) 102:1451–60. doi: 10.1189/jlb.4A0517-195R
63. Lai D, Tang J, Chen L, Fan EK, Scott MJ, Li Y, et al. Group 2 innate lymphoid cells protect lung endothelial cells from pyroptosis in sepsis. Cell Death Dis. (2018) 9:369. doi: 10.1038/s41419-018-0412-5
Keywords: sepsis, septic shock, immunosuppression, sepsis-induced immunosuppression, innate lymphoid cells (ILC), NK cells, lymphopenia
Citation: Carvelli J, Piperoglou C, Bourenne J, Farnarier C, Banzet N, Demerlé C, Gainnier M and Vély F (2019) Imbalance of Circulating Innate Lymphoid Cell Subpopulations in Patients With Septic Shock. Front. Immunol. 10:2179. doi: 10.3389/fimmu.2019.02179
Received: 18 February 2019; Accepted: 29 August 2019;
Published: 20 September 2019.
Edited by:
Massimo Vitale, Azienda Ospedaliera Universitaria San Martino (IRCCS), ItalyReviewed by:
Anja Fuchs, Washington University in St. Louis, United StatesVladimir Badovinac, The University of Iowa, United States
Copyright © 2019 Carvelli, Piperoglou, Bourenne, Farnarier, Banzet, Demerlé, Gainnier and Vély. This is an open-access article distributed under the terms of the Creative Commons Attribution License (CC BY). The use, distribution or reproduction in other forums is permitted, provided the original author(s) and the copyright owner(s) are credited and that the original publication in this journal is cited, in accordance with accepted academic practice. No use, distribution or reproduction is permitted which does not comply with these terms.
*Correspondence: Julien Carvelli, julien.carvelli@ap-hm.fr