Chemistry and Bioactivity of Marine-Derived Bisabolane Sesquiterpenoids: A Review
- Qingdao Hospital of Traditional Chinese Medicine (Qingdao Hiser Hospital), Qingdao, China
Natural products, characterized by intriguing scaffold diversity and structural complexity, as well as significant agricultural and medicinal activities, have been a valuable source of agrochemicals/drugs development and have historically made a huge contribution to pharmacotherapy. Structurally, bisabolanes are a family of naturally occurring sesquiterpenoids that featured a hexatomic ring core incorporating with eight continuous carbons, which cause high structural variability along the alkyl side chain to form abundant functionalities. Moreover, apart from their interesting structures, bisabolanes have shown multitudinous bioactivities. Bisabolanes are distributed in a variety of marine invertebrates, terrestrial plant, and microbial sources. Interestingly, bisabolanes characterized from marine environment possess unique characteristics both structurally and biologically. A total of 296 newly-discovered bisabolanes were searched. Among them, 94 members were isolated from marine organisms. This review particularly focuses on the new bisabolanes characterized from marine organisms (covering from 2000 to 2021), including marine-derived fungi, algae, soft corals, and sponges, with emphasis on the diversity of their chemical structures as well as the novelty and differences between terrestrial and marine sources. Moreover, a wide range of bioactivities of marine-derived bisabolanes, including antimicrobial, anti-inflammatory, enzyme inhibitory, and cytotoxic properties, are presented herein, which is considered to be a promising resource for the discovery of new drug leads and agrochemicals.
Introduction
Natural products (NPs) are secondary metabolites produced by plants, microorganisms, and animals through diversified biosynthetic pathways. NPs possess attractive structural diversity and complexity along with prominent biological properties, and hence have played an essential role in the discovery of lead compounds for new medicines (Butler, 2005). Historically, NPs and their derivatives have made a significant contribution to pharmacotherapy. The discovery of penicillin, the first broad-spectrum antibiotic, is a representative example, which, not only accelerated the course of pharmaceutical research but also saved thousands of lives (Zhang et al., 2020). NPs have been an inexhaustible reservoir for new drug development, which prove to be the cornerstone of modern pharmaceutical industry. It is estimated that approximately 60% of approved small-molecule based drugs between 1981 and 2019 are derived from NPs, indicating that there is still an urgent need to excavate more NPs with novel structures and promising bioactivities (Newman and Cragg, 2020).
Sesquiterpenoids represent structurally diverse NPs with extensive pharmacological activities, with more than 300 skeletons being reported up to now (Fraga, 2011). Bisabolanes are a family of sesquiterpenoids widely distributed in terrestrial plants, microorganisms, and marine organisms (algae, soft corals, and sponges) (Chen et al., 2021). Structurally, bisabolanes contain a hexatomic ring core that generally, but not always, features a 15-carbon skeleton. Bisabolanes appear to be among the simplest of all sesquiterpenoids, as most of them are monocyclic. However, they represent high complexity of cyclic sesquiterpenoids. These compounds incorporate an eight continuous carbons side chain, which cause high structural diversity to generate abundant functionalities including double bond, lactone, furan, pyran, and so on, by oxidation, reduction, esterification, and cyclization (Niu et al., 2020; Chen et al., 2021). Moreover, some unique members, such as norbisabolanes (Jian Li et al., 2015; Ge et al., 2021), dimers (Sun et al., 2012; Georgantea et al., 2013), glycosides (Lv et al., 2014), adducts (Xiao-Dong Li et al., 2015), have also been characterized. Chemically speaking, the findings of these fascinating molecules provide considerable chemical foundation in the course of new drugs and agricultural chemicals discovery. In addition, bisabolanes have been reported to possess extensive pharmacological activities, including but not limited to anti-inflammatory, insecticidal, antitumor, antimicrobial, and antiviral activities.
Marine environment has distinct ecological characteristics compared to terrestrial environment, such as high salinity, high pressure, extreme temperature, and low oxygen. To overcome those multiple extreme environmental stresses, marine organisms have evolved unique metabolic abilities to produce novel bioactive natural products. Marine natural products, therefore, possess unimaginable structural diversity and have brought immensely attention. A large amount of marine natural products have been discovered and reported, including polyketides, terpenoids, steroids, peptides, and nitrogen-containing compounds (Carroll et al., 2021). Compared to NPs isolated from terrestrial environment, marine natural products are often considered to be more diversiform and variable both structurally and biologically. For example, marine-derived heterocyclic alkaloids are challenging NPs possessing structurally unique skeletons arising from distinct amino acids (Zhang et al., 2020). Moreover, many of marine natural products are characterized as halogenated compounds, which are relatively few in terrestrial natural products. As for above-mentioned bisabolanes, it is believed that marine-derived bisabolanes may also have certain novelty and differences in contrast to terrestrial ones on structures and bioactivities. Therefore, to fully demonstrate the chemistry and bioactivity of newly-discovered marine-derived bisabolanes in recent years, we performed an in-depth investigation of these compounds, not only from marine sources but also from terrestrial sources. As a result, a total of 296 new bisabolanes and 93 references were searched, with 94 members isolated from marine organisms. They include 64 compounds (1–64) characterized from marine fungi, 6 compounds (65–70) from marine algae, 9 compounds (71–79) from soft corals, 15 compounds (80–94) from marine sponges. Moreover, another 27 compounds (95–122) from terrestrial fungi, 169 compounds (123–291) from terrestrial plants, and 5 compounds (292–296) from others were presented in Supplemental Materials (Supplementary Figures S1–S3). Pharmacological studies indicated the biological potential of these compounds, exhibiting antimicrobial, anti-inflammatory, enzyme inhibitory, cytotoxic, antimicroalgal, and antifouling properties. It should be pointed out that a review regarding to the chemistry and bioactivity of bisabolanes has been published very recently (Shu et al., 2021). The previous review by Shu et al. described 356 bisabolanes in total isolated from 24 families, primarily from Compositae, Zingiberaceae, Aspergillaceae, Halichondriidae, and Aplysiidae (Shu et al., 2021). Since the authors mixed up the marine and terrestrial bisabolanes for discussion, this review gave little information on marine bisabolanes, especially their structural features. In this study, this is the first attempt to summarize the chemical diversity of marine-derived bisabolanes, highlight their novelty and differences between terrestrial and marine sources, and provide a future perspective of their potential applications as lead compounds.
Chemical Diversity of the Newly-Discovered Marine-Derived Bisabolanes
Bisabolanes Characterized From Marine-Derived Fungi
As shown in Figure 1, Figure 2, Figure 3, Figure 4, a total of 64 new bisabolanes (1–64) were characterized from marine-derived filamentous fungi. Bioassay-guided isolation of a marine-derived fungus Aspergillus sp. obtained from a gorgonian coral yielded three new phenolic bisabolanes, (+)-methyl sydowate (1), 7-deoxy-7,14-didehydrosydonic acid (2), and 7-deoxy-7,8-didehydrosydonic acid 3) (Wei et al., 2010). 1 possessed a 2,2,6-trimethyltetrahydro-2H-pyran moiety, which was proved as a natural product, rather than an artifact during the isolation process. Chemical investigation of the mangrove-derived endophytic fungus Aspergillus sp. xy02 resulted in the isolation of seven new phenolic bisabolanes, namely (7R,10S)-7,10-epoxysydonic acid (4), (7S,10S)-7,10-epoxysydonic acid (5), (7R,11S)-7,12-epoxysydonic acid (6), (7S,11S)-7,12-epoxysydonic acid (7), 7-deoxy-7,14-didehydro-12-hydroxysydonic acid (8), (Z)-7-deoxy-7,8-didehydro-12-hydroxysydonic acid (9), and (E)-7-deoxy-7,8-didehydro-12-hydroxysydonic acid (10) (Wang et al., 2018). Compounds 4 and 5 were elucidated as a pair of isomers of the 7,10-epoxide of sydonic acid, while compounds 6 and 7 were found to form a 7,12-epoxide. Four new phenolic bisabolanes, (S)-(+)-11-dehydrosydonic acid 11) and (7S,11S)-(+)-12-acetoxysydonic acid (12), and expansols A 13) and B (14), were produced by the marine fungus Penicillium expansum 091,006, which was endogenous with the mangrove plant Excoecaria agallocha (Lu et al., 2010). Compounds 13 and 14 were the first examples of adducts containing both phenolic bisabolane sesquiterpenoid and diphenyl ether units.
Fourteen undescribed phenolic bisabolanes with diverse structures, asperbisabolanes A−N (15–28), were isolated from the deep sea sediment-derived fungus A. sydowii MCCC 3A00324 (Niu et al., 2020). 15 and 16 represented the first bisabolanes featuring a 6/6/6 tricyclic skeleton. 17 was characterized as a novel seco-bisabolane containing a rare dioxolane moiety, and 25 possessed a rare methylsulfonyl group. Four new bisabolanes, including aspergiterpenoid A (29), (−)-sydonol (30), (−)-sydonic acid (31), and (−)-5-(hydroxymethyl)-2-(2′,6′,6′-trimethyltetrahydro-2H-pyran-2-yl)phenol (32), were characterized from a marine sponge-sourced fungus Aspergillus sp. (Li et al., 2012). Two new phenolic bisabolanes, ent-aspergoterpenin C 33) and 7-O-methylhydroxysydonic acid (34), were obtained from the culture extracts of A. versicolor SD-330, a deep-sea sediment-sourced fungus (Li et al., 2019a). 33 and 34 were considered as natural products through HPLC analysis, rather than artifacts produced by esterification or methylation. Then from the same fungus, another new compound 12-hydroxysydowic acid 35) was isolated (Li et al., 2019b). Three new bisabolanes, (7S)-(+)-7-O-methylsydonol (36), (7S,11S)-(+)-12-hydroxysydonic acid (37), and 7-deoxy-7,14-didehydrosydonol (38), were isolated from the fungus A. sydowii by the addition of a DNA methyltransferase inhibitor 5-azacytidine (Chung et al., 2013).
Through chemical epigenetic manipulation strategy, the gorgonian-derived fungus A. versicolor XS-20090066 was found to produce a new bisabolane aspergillusene E (39) with a benzofuran moiety, by the addition of histone deacetylase inhibitor, suberoylanilide hydroxamic acid, and DNA methyltransferase inhibitor, 5-azacytidine (Wu et al., 2020). A pair of new S-containing norbisabolane enantiomers methylsulfinyl-1-hydroxyboivinianin A [(±)-40] were produced by the marine algal-derived endophytic fungus P. chrysogenum LD-201810 (Ge et al., 2021). 40 was proven to possess a rare methylsulfinyl substituent, and finally afforded individual enantiomers (+)-40 and (−)-40 by chiral HPLC. A new polychiral bisabolane with a 10,11-diol moiety named bisabolanoic acid A 41) was isolated from the mangrove-derived endophytic fungus Colletotrichum sp. SCSIO KcB3-2 (Li et al., 2021). A pair of new bisabolanes enantiomers (±)-flavilane A (42), as well as a new derivative flavilane B (43), were isolated from the seawater-derived fungus A. flavipes 297 (Chen et al., 2021). 42 and 43 represented uncommon cases of phenolic bisabolanes incorporating with a methylsulfinyl group. A new bisabolane bisabolan-1,10,11-triol 44) and a new norbisabolane 12-nor-11-acetoxybisabolen-3,6,7-triol 45) were isolated from a marine algal-derived endophytic fungus T. asperellum cf44-2 (Song et al., 2018). In a systemic phytochemical investigation on a marine red alga-epiphytic isolate of T. asperellum Y6−2, eight new bisabolanes, trichobisabolins A−H (46–53), were finally obtained (Shi et al., 2019). Compounds 49–53 were rare nor-sesquiterpenes.
Four new phenolic bisabolanes 54–57 were isolated from the culture of mangrove endophytic fungus A. flavus QQSG-3 (Wu et al., 2018). 54 was a phenol-bisabolane adduct linked via a methylene, while 56 was a dimeric analogue of sydonol. Three rare phenolic bisabolane dimers, disydonols A−C (58–60), were acquired by the extensive investigation on a marine-derived fungus Aspergillus sp. (Sun et al., 2012). 58–60 were the dehydration dimeric metabolites of (S)-(+)-sydonol. Four new phenolic bisabolanes, peniciaculins A 61) and B (62), (7S)-(−)-10-hydroxysydonic acid (63), and 1-hydroxyboivinianin A (64), were isolated from a deep sea sediment-derived fungus P. aculeatum SD-321 (Jian Li et al., 2015). 61 was a diphenyl ether-bisabolane adduct via an ether bond, whereas 62 represented the dimeric bisabolane with two monomers linking through an ester bond.
Bisabolanes Characterized From Marine Algae
Compounds 65–70 (Figure 5) were obtained from marine algae. Two new highly halogenated monocyclic bisabolanes, laurecomposins A 65) and B (66), were discovered from a phytochemical investigation on the red alga Laurencia composita Yamada (Hu et al., 2020). Phytochemical studies on L. okamurai, a marine red alga, yielded four new bisabolane sesquiterpenes, okamurenes A−D (67–70) (Liang et al., 2012). 67 and 68 were brominated isomers, and 69 and 70 were dehalogenated 6,9-epoxybisabola-2,7 (14),10-triene. In the sense of chemotaxonomy, 67 and 68 represented the first members of brominated bisabolanes possessing a phenyl moiety among sesquiterpenes isolated from algae belonging to Laurencia.
Bisabolanes Characterized From Soft Corals
Detailed chemical investigation of the tropical soft coral Pseudopterogorgia rigida led to the discovery of seven new bisabolanes 71–77 (Figure 6) (Georgantea et al., 2014). These obtained compounds belonged to phenolic bisabolanes, except for 73, which was elucidated as acetyl-β-bisabolol. Moreover, 71 and 72 were epimers of 1,9-epoxy-4-hydroxy-α-curcumene. This species also produced minor constituents, perezoperezone 78) and curcuperezone 79) (Georgantea et al., 2013). 78 possessed a non-symmetrical dimeric structure through the formation of a five-membered ring, whereas 79 was a rare dimer synthesized by perezone and α-curcumene to form a tricyclic core.
Bisabolanes Characterized From Marine Sponges
As shown in Figure 7, 15 bisabolanes were isolated and identified from marine sponges. Six new aromatic bisabolanes, plakordiols A−D (80–83), (7R, 10R)-hydroxycurcudiol (84), and (7R, 10S)-hydroxycurcudiol (85), were isolated from the marine sponge Plakortis simplex (Wang et al., 2021). Another four new aromatic bisabolanes, including 6-(3-hydroxy-6-methyl-1,5-heptadien-2-yl)-3-methylbenzene-1,4-diol (86), 4-hydroxy-3,7-dimethyl-7-(3-methylbut-2-en-1-yl)benzofuran-15-one (87), 6-(2-methoxy-5-methylhept-4-en-2-yl)-3-methylbenzene-1,4-diol (88), and 9-(3,3-dimethyloxiran-2-yl)-1,7-dimethyl-7-chromen-4-ol (89), were obtained from an organic extract of the marine sponge Myrmekioderma sp. (Costa et al., 2019). All of the isolated compounds represented cyclic bisabolanes bearing rich oxo functionality. Furthermore, chemical investigation of the Thai marine sponge Myrmekioderma sp. afforded two new aromatic bisabolanes, myrmekioperoxides A (90) and B 91) (Wongbundit et al., 2020). A new monocyclic bisabolane (E)-3-isocyanobisabolane-7,10-diene 92) containing an isonitrile group was isolated from the Okinawan sponge of the genus Axinyssa (Iwashima et al., 2002). Finally, two new rare epoxy-substituted nitrogenous bisabolanes, 3-formamido-7,8-epoxy-α-bisabolane 93) and 3-isocyano-7,8-epoxy-α-bisabolane (94), were produced by the Hainan sponge Axinyssa sp. (Sun et al., 2010). 93 and 94 were α-bisabolanes with an 7,8-epoxy ring, which also contained a formamide functional group in 93 and an isonitrile group in 94. It is worth mentioning that, although nitrogen-bearing bisabolanes are widely encountered in marine invertebrates, epoxy-substituted nitrogenous bisabolanes are rather rare.
Biological Activities of Bisabolanes
Antimicrobial Activity
Compound 1 exerted weak antibacterial efficacy against the Gram positive strain Staphylococcus aureus with an inhibition zone of 11 in diameter at the concentration of 100 μg/ml (Wei et al., 2010). Compounds 5, 6, 8, and 10 showed mild antibacterial effect on S. aureus with the IC50 values of 32.2, 36.0, 41.9, and 31.5 μM, respectively (Wang et al., 2018). Compound 30 was active against the pathogenic bacteria S. albus and Micrococcus tetragenus with the MICs of 5.00 and 1.25 μM, while 32 on S. albus and Bacillus subtilis with the MICs of 5.00 and 2.50 μM, respectively (Li et al., 2012). Compounds 33–35 exhibited high antibacterial activity against zoonotic pathogenic bacteria Escherichia coli, Edwardsiella tarda, Vibrio harveyi, and V. parahaemolyticus, with MICs no higher than 8.0 μg/ml (Li et al., 2019a; Li et al., 2019b). 39 possessed activity against S. epidermidis and S. aureus with MICs of 8 and 16 μg/ml. Moreover, 39 also showed antifungal activity toward Candida albicans and C. tropicalis with MICs of 64 and 32 μg/ml, respectively (Wu et al., 2020). 44 and 45 showed weak activity against four marine pathogenic bacteria, V. parahaemolyticus, V. anguillarum, V. harveyi, and V. splendidus, with the inhibitory zone diameters of 6.3–7.5 mm at 20 μg/disk (Song et al., 2018). Compound 61 demonstrated inhibitory activity against aquatic pathogens Micrococcus luteus and V. alginolyticus with MICs of 1.0 and 2.0 μg/ml, respectively (Xiao-Dong Li et al., 2015). Moreover, the dimer 62 and norbisabolane 64 showed selective activity against E. tarda and V. harveyi, with MICs of 8.0 and 4.0 μg/ml. The halogenated bisabolanes 65 and 66 exhibited not only potent antifungal activity against Microsporum gypseum, with MICs of 4 and 8 μg/ml, but also considerable antibacterial activity against S. aureus with MICs of 26.8 and 15.4 μg/ml, respectively (Hu et al., 2020).
Cytotoxic Activity
Compound 13, a new adduct of phenolic bisabolane and diphenyl ether, possessed moderate cytotoxicity against HL-60 tumor cells with an IC50 value of 15.7 μM, and 14 inhibited higher activity against A549 and HL-60 cells with IC50 values of 1.9 and 5.4 μM, respectively (Lu et al., 2010). The condensation between phenolic bisabolanes and diphenyl ethers appear to enhance cytotoxicity significantly. The sulfurated compounds 42 and 43 exhibited moderate cytotoxicity against MKN-45 and HepG2 cells, with the IC50 values ranging from 19.8 to 30.1 μg/ml, which indicated that the methylsulfinyl group could strengthen cytotoxicity (Chen et al., 2021). The dimmers 58 and 60 showed pronounced cytotoxic activities against HepG-2 and Caski cell lines with IC50 values of 2.91–12.40 μg/ml, whereas 59 was relatively noncytotoxic (IC50 > 100 μg/ml) (Sun et al., 2012).
Anti-Inflammatory Activity
Compounds 15–28 were evaluated inhibition effects against nitric oxide (NO) secretion in LPS-activated BV-2 microglia cells. 20 and 26 possessed the inhibition rates of 46.4 and 56.8% at a concentration of 10 μM. Structure–activity relationship analysis indicated that the double bond at Δ7,8 in bisabolane may strengthen the inhibition of NO secretion. Molecular mechanism study revealed that 26 inhibited the NF-κB-activated pathway in a dose-dependent manner (Niu et al., 2020).
Enzyme Inhibitory Activity
The polychiral bisabolane 41 displayed moderate inhibitory activity against acetylcholinesterase (AChE) with an IC50 value of 2.2 μM. In silico molecular docking analysis indicated that 41 may bind to the active site residue TYR121 by hydrogen bonds (Li et al., 2021). 54 and 56 showed strong α-glucosidase inhibitory effects with IC50 values of 4.5 and 3.1 μM, respectively (Wu et al., 2018).
Other Activities
Compound 32 not only showed selective antibacterial activity but also possessed significant antifouling activity. 32 drastically inhibited the larval settlement of the barnacle Balanus amphitrite at a concentration of 25.0 μg/ml (Li et al., 2012). 36 and 37 showed anti-diabetic activity, which increased medium glucose consumption of the differentiated 3T3-L1 adipocytes in comparison with the insulin treatment (Chung et al., 2013). 39 displayed antifouling activity against bryozoan larvae of Bugula neritina with the EC50 and LC50 values of 6.25 and 25 μg/ml, respectively (Wu et al., 2020). The norbisabolane 45 possessed antimicroalgal potency against the marine phytoplankton Heterosigma akashiwo with an IC50 value of 8.4 μg/ml (Song et al., 2018). Compounds 49–53 exhibited growth inhibition against four marine phytoplanktons (H. akashiwo, Prorocentrum donghaiense, Chattonella marina, and Karlodinium veneficum) with IC50s ranging from 2.1–85 μg/ml (Shi et al., 2019). It appeared that the acetoxy group at C-11 could significantly enhance inhibitory ability against marine phytoplanktons. Zebrafish Nile red fat metabolism assay revealed that compounds 86, 87, and 89 had significant lipid-reducing activity with IC50 values of 7.89, 12.61, and 1.22 μM, respectively (Costa et al., 2019). 92 containing an isonitrile group exerted strong brine shrimp lethal activity with an LC50 of 0.1 μg/ml (Iwashima et al., 2002).
Discussions and Conclusion
The Novelty of Bisabolanes Characterized From Fungi and Differences Between Terrestrial and Marine Sources
Bisabolanes, a unique family of naturally occurring sesquiterpenoids, have been a valuable reservoir for searching for new lead compounds with medical and/or agricultural potential. These compounds are widely distributed in terrestrial plants, microorganisms, and marine organisms (algae, soft corals, and sponges) (Figure 8A). This review summarizes 296 newly-discovered bisabolanes in the past few years. Among them, 92 compounds, accounting for 31.08% of all described bisabolanes, were characterized from fungi [including marine-derived 1–64 (Figure 1, Figure 2, Figure 3, Figure 4) and terrestrial 95–122 (Supplementary Figure S1 in Supplemental Materials], especially filamentous fungi. The filamentous fungi Aspergillus, Penicillium, and Trichoderma are dominant genera as producers of these compounds, with 44, 9, and 10 members described, respectively (Figure 8B). Structurally, bisabolanes characterized from fungi are mainly phenolic bisabolanes, which featured a para-alkylated benzene ring framework comprising a side chain with an eight consecutive carbons. Their structure variability is due to the transformation of the side chain, such as oxidation, reduction, esterification, and cyclization, to generate abundant functionalities including lactone (eg. compounds 6, 17, 22, 35), double bond (e.g., compounds 8–10), furan (e.g., compounds 4, 5, 39), pyran (e.g., compounds 1, 32, 43), and so on. Moreover, fungi also produce rare bisabolane dimers. Representative examples are compounds 13 and 14, 58–62, and 120–122. It should be pointed out that, the endophytic fungi, an ecological group generally resided in plants and symbiotic with their hosts, are considered as an important producer of bisabolanes. Endophytic fungi possess a complicated interaction with their host plants, thus they have evolved novel metabolic pathways to produce the same or similar secondary metabolites as the hosts. Therefore, it is a more talented pathway to isolate bisabolanes from fungal endophytes, rather than the medicinal plants.
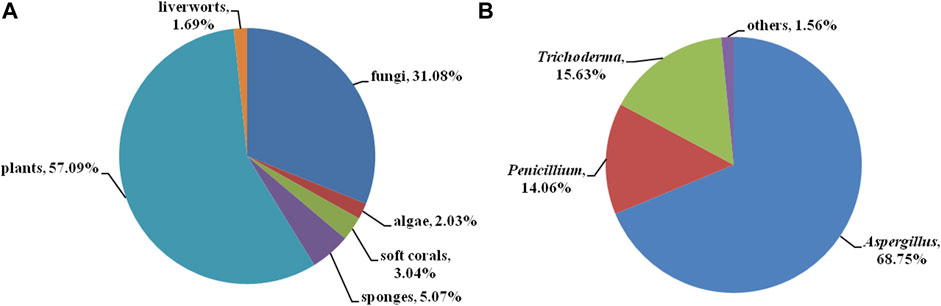
FIGURE 8. (A) Source categories of the described bisabolanes; (B) Categories of the marine fungi-derived bisabolanes.
Compared to bisabolanes isolated from terrestrial fungi, some of bisabolanes from marine-derived fungi possess unique functionalities. For example, the sulfurated bisabolanes were exclusively isolated from marine fungi, including 25 with a rare methylsulfonyl group isolated from the deep sea sediment-derived fungus A. sydowii MCCC 3A00324 (Niu et al., 2020), 40 and 42–43 incorporating with a rare methylsulfinyl group isolated from the marine algal-derived endophytic fungus P. chrysogenum LD-201810 (Ge et al., 2021) and the seawater-derived fungus A. flavipes 297 (Chen et al., 2021), respectively. 52 isolated from a marine red alga-epiphytic isolate of T. asperellum Y6−2, represented a rare example of nor-sesquiterpenes with a spiro system (Shi et al., 2019). It is believed that marine-derived fungi suffered more survival stress than terrestrial fungi, and thus evolved more flexible metabolic pathways by producing novel secondary metabolites.
Characterization of Terrestrial and Marine-Derived Bisabolanes
Apart from marine-derived fungi, a total of 30 compounds (65–94) were characterized from marine organisms, including marine plants algae and marine invertebrates, soft corals and sponges. It is well-known that marine organisms, which are suffered from high salinity, high pressure, extreme temperature, and low oxygen, possess unique metabolic pathways that are quite different from that of terrestrial organisms. Accordingly, marine-derived natural products tend to have unexpected structural types. For example, compounds 78 and 79, two unprecedented non-symmetrical tricyclic dimers were characterized from the tropical soft coral Pseudopterogorgia rigida (Georgantea et al., 2013). In addition, some highly halogenated bisabolanes, such as 65–68, were isolated from the marine algae belonging to Laurencia (Liang et al., 2012; Hu et al., 2020). With this consideration, it is believed that marine organisms, which have emerged as a valuable frontier for the discovery of new lead compounds, continue to be a rich source of structurally diverse bisabolanes.
Most of the described bisabolanes, apparently, are isolated from terrestrial plants. As shown in Figure 8A and Supplementary Figure S2, 169 compounds (123–291, accounting for 57.09% of all bisabolanes) are scattered across a variety of species belonging to the genera Ligularia, Parasenecio, Curcuma, and so on. The chemical types of plant-derived bisabolanes are relatively common. Most of them are featured as highly oxygenated monocyclic bisabolanes bearing a cyclohexane ring. Interestingly, some bisabolanes, such as 184, 187–190, 196, and 201, are uncommon chlorinated derivatives. It is generally considered that halogenated compounds can be exclusively isolated from the marine environment. The isolation of halogenated bisabolanes from terrestrial plants indicated that terrestrial plants may also possess great potentiality in finding novel halogenated compounds.
Bioactivity Diversity and Potential Applications
Bisabolanes are also attractive for their various and potent biological activities. As shown in Figure 9 and Table 1, the reported bisabolanes exhibited an extensive range of biological activities, including antimicrobial, anti-inflammatory, enzyme inhibitory, cytotoxic, antimicroalga, and other activities. Among the 94 described marine-derived bisabolanes, 43 compounds were found to possess moderate to potent biological activities. 18 compounds showed promising antibacterial or antifungal activity, while 6 compounds possessed various degrees of cytotoxic activity. Most importantly, some of them possessed significant bioactivities, which were higher than the positive controls. For example, 14 inhibited potent cytotoxic activity against A549 and HL-60 cells with IC50 values of 1.9 and 5.4 μM, respectively (Lu et al., 2010). 33–35 exhibited strong antibacterial activity against E. coli, E. tarda, V. harveyi, and V. parahaemolyticus, with MICs no higher than 8.0 μg/ml (Li et al., 2019a; Li et al., 2019b). In addition, bisabolanes characterized from terrestrial fungi and plants also possess promising activities. Compounds 118 and 119, two dicyclic bisabolanes produced by the fungus Pleurotus cystidiosus were found to have significant cytotoxicity at the nM level. Especially, 119 exhibited the strongest effect on two human prostate cancer DU-145 and C42B cells, with the IC50s of 28 and 52 nM. Further pharmacological studies indicated that 119 induced the apoptosis of DU-145 cells (Zheng et al., 2015). The dicyclic 141 obtained from the rhizomes of Curcuma longa displayed more potent anti-inflammatory activity against the LPS-activated production of NO (IC50, 25.5 μM), when compared with the positive control dexamethasone (IC50, 33.6 μM) (Cheng et al., 2019). The aromatic bisabolanes 182 and 183 potently inhibited α-glucosidase with the IC50 values of 14.9 and 19.4 μM, respectively, approximately 30 times stronger than the positive control acarbose (IC50, 496 μM) (Song et al., 2021). The attractive bioactivities make many of these compounds suitable lead compounds or even candidates for the development of new medicines and agrochemicals and may trigger further pharmacological and synthesis studies.
In conclusion, bisabolane-type sesquiterpenoids, especially those isolated from marine organisms are considered to be nonnegligible natural products both structurally and biologically. This review systematically summarizes 296 newly reported bisabolanes characterized from fungi, marine algae, soft corals, marine sponges, terrestrial plants, and the others. Among them, 94 members were isolated from marine organisms. The following pharmacological studies indicated the biological potential of these compounds, which exhibited antimicrobial, anti-inflammatory, enzyme inhibitory, cytotoxic, antimicroalgal, and antifouling properties. This is the first attempt to focus on the chemistry and bioactivity of marine-derived bisabolanes, aiming to summarize the chemical diversity of marine-derived bisabolanes, highlight their novelty and differences between terrestrial and marine sources, and provide a future perspective of their potential application prospect as lead compounds. It is believed that in the near future, the research on these fascinating compounds will be increasingly abundant and conducive to the development of new drugs and agrochemicals.
Author Contributions
Conceptualization, C-SL; Writing—original draft preparation, C-SL and L-TL; Writing—review and editing, LY, XD, and JL. All authors have read and approved the final manuscript.
Funding
This research was supported by the Qingdao Science and Technology Project of TCM (No. 2021-zyyq05).
Conflict of Interest
The authors declare that the research was conducted in the absence of any commercial or financial relationships that could be construed as a potential conflict of interest.
Publisher’s Note
All claims expressed in this article are solely those of the authors and do not necessarily represent those of their affiliated organizations, or those of the publisher, the editors and the reviewers. Any product that may be evaluated in this article, or claim that may be made by its manufacturer, is not guaranteed or endorsed by the publisher.
Supplementary Material
The Supplementary Material for this article can be found online at: https://www.frontiersin.org/articles/10.3389/fchem.2022.881767/full#supplementary-material
References
Butler, M. S. (2005). Natural Products to Drugs: Natural Product Derived Compounds in Clinical Trials. Nat. Prod. Rep. 22, 162–195. doi:10.1039/b402985m
Carroll, A. R., Copp, B. R., Davis, R. A., Keyzers, R. A., and Prinsep, M. R. (2021). Marine Natural Products. Nat. Prod. Rep. 38, 362–413. doi:10.1039/d0np00089b
Chen, Y., Zhu, H.-Y., Xu, L.-C., Wang, S.-P., Liu, S., Liu, G.-D., et al. (2021). Antimicrobial and Cytotoxic Phenolic Bisabolane Sesquiterpenoids from the Fungus Aspergillus flavipes 297. Fitoterapia 155, 105038. doi:10.1016/j.fitote.2021.105038
Cheng, X., Li, H., Wu, P., Xu, L., Xue, J., and Wei, X. (2019). Two New Bisabolane-type Sesquiterpenoids from the Cooking Liquid of Curcuma Longa Rhizomes. Phytochemistry Lett. 29, 169–172. doi:10.1016/j.phytol.2018.12.004
Chung, Y.-M., Wei, C.-K., Chuang, D.-W., El-Shazly, M., Hsieh, C.-T., Asai, T., et al. (2013). An Epigenetic Modifier Enhances the Production of Anti-diabetic and Anti-inflammatory Sesquiterpenoids from Aspergillus sydowii. Bioorg. Med. Chem. 21, 3866–3872. doi:10.1016/j.bmc.2013.04.004
Costa, M., Coello, L., Urbatzka, R., Pérez, M., and Thorsteinsdottir, M. (2019). New Aromatic Bisabolane Derivatives with Lipid-Reducing Activity from the marine Sponge Myrmekioderma Sp. Mar. Drugs 17, 375. doi:10.3390/md17060375
Ge, Y., Tang, W.-L., Huang, Q.-R., Wei, M.-L., Li, Y.-Z., Jiang, L.-L., et al. (2021). New Enantiomers of a Nor-Bisabolane Derivative and Two New Phthalides Produced by the marine-derived Fungus Penicillium chrysogenum LD-201810. Front. Microbiol. 12, 727670. doi:10.3389/fmicb.2021.727670
Georgantea, P., Ioannou, E., Vagias, C., and Roussis, V. (2013). Perezoperezone and Curcuperezone: Bisabolane Dimers from the Soft Coral Pseudopterogorgia Rigida. Tetrahedron Lett. 54, 6920–6922. doi:10.1016/j.tetlet.2013.10.041
Georgantea, P., Ioannou, E., Vagias, C., and Roussis, V. (2014). Bisabolane and Chamigrane Sesquiterpenes from the Soft Coral Pseudopterogorgia Rigida. Phytochemistry Lett. 8, 86–91. doi:10.1016/j.phytol.2014.02.006
Hu, Z.-B., Yu, X.-Q., Wang, B., Liu, A.-H., Zhao, T.-S., Guo, Y.-W., et al. (2020). Structurally Diverse Halosesquiterpenoids from the Red Alga Laurencia Composita Yamada. Fitoterapia 146, 104716. doi:10.1016/j.fitote.2020.104716
Iwashima, M., Terada, I., Iguchi, K., and Yamori, T. (2002). New Biologically Active marine Sesquiterpenoid and Steroid from the Okinawan Sponge of the Genus Axinyssa. Chem. Pharm. Bull. 50, 1286–1289. doi:10.1248/cpb.50.1286
Jian Li, J., Wang, H.-F., Chen, G., Huang, S.-D., Zhang, W.-Y., Hua, H.-M., et al. (2015). Structure Determination of Two New Bisabolane-type Sesquiterpenes from the Rhizomes ofCurcuma Longaby NMR Spectroscopy. Magn. Reson. Chem. 53, 536–538. doi:10.1002/mrc.4215
Li, D., Xu, Y., Shao, C.-L., Yang, R.-Y., Zheng, C.-J., Chen, Y.-Y., et al. (2012). Antibacterial Bisabolane-type Sesquiterpenoids from the Sponge-Derived Fungus Aspergillus Sp. Mar. Drugs 10, 234–241. doi:10.3390/md10010234
Li, X.-D., Li, X.-M., Yin, X.-L., Li, X., and Wang, B.-G. (2019a). Antimicrobial Sesquiterpenoid Derivatives and Monoterpenoids from the Deep-Sea Sediment-Derived Fungus Aspergillus versicolor SD-330. Mar. Drugs 17, 563. doi:10.3390/md17100563
Li, X.-D., Li, X., Li, X.-M., Yin, X.-L., and Wang, B.-G. (2019b). Antimicrobial Bisabolane-type Sesquiterpenoids from the Deep-Sea Sediment-Derived Fungus Aspergillus versicolor SD-330. Nat. Product. Res. 35, 4265–4271. (Online ahead of print. doi:10.1080/14786419.2019.1696792
Li, K.-L., Dai, Y., She, J.-L., Zeng, Y.-B., Dai, H.-F., Ou, S.-L., et al. (2021). Bisabolanoic Acid A, a New Polychiral Sesquiterpene with AChE Inhibitory Activity from a Mangrove-Derived Fungus Colletotrichum Sp. J. Asian Nat. Prod. Res. 24, 88–95. doi:10.1080/10286020.2021.1873297
Liang, Y., Li, X.-M., Cui, C.-M., Li, C.-S., Sun, H., and Wang, B.-G. (2012). Sesquiterpene and Acetogenin Derivatives from the marine Red Alga Laurencia Okamurai. Mar. Drugs 10, 2817–2825. doi:10.3390/md10122817
Lu, Z., Zhu, H., Fu, P., Wang, Y., Zhang, Z., Lin, H., et al. (2010). Cytotoxic Polyphenols from the marine-derived Fungus Penicillium expansum. J. Nat. Prod. 73, 911–914. doi:10.1021/np100059m
Lv, J.-J., Wang, Y.-F., Zhang, J.-M., Yu, S., Wang, D., Zhu, H.-T., et al. (2014). Anti-hepatitis B Virus Activities and Absolute Configurations of Sesquiterpenoid Glycosides from Phyllanthus Emblica. Org. Biomol. Chem. 12, 8764–8774. doi:10.1039/c4ob01196a
Newman, D. J., and Cragg, G. M. (2020). Natural Products as Sources of New Drugs over the Nearly Four Decades from 01/1981 to 09/2019. J. Nat. Prod. 83, 770–803. doi:10.1021/acs.jnatprod.9b01285
Niu, S., Yang, L., Zhang, G., Chen, T., Hong, B., Pei, S., et al. (2020). Phenolic Bisabolane and Cuparene Sesquiterpenoids with Anti-inflammatory Activities from the Deep-Sea-Derived Aspergillus sydowii MCCC 3A00324 Fungus. Bioorg. Chem. 105, 104420. doi:10.1016/j.bioorg.2020.104420
Shi, Z.-Z., Miao, F.-P., Fang, S.-T., Yin, X.-L., and Ji, N.-Y. (2019). Trichobisabolins A-H, Eight New Bisabolane Derivatives from the marine-alga-epiphytic Fungus Trichoderma Asperellum Y6-2. Fitoterapia 134, 372–377. doi:10.1016/j.fitote.2019.03.010
Shu, H.-Z., Peng, C., Bu, L., Guo, L., Liu, F., and Xiong, L. (2021). Bisabolane-type Sesquiterpenoids: Structural Diversity and Biological Activity. Phytochemistry 192, 112927. doi:10.1016/j.phytochem.2021.112927
Song, Y.-P., Miao, F.-P., Fang, S.-T., Yin, X.-L., and Ji, N.-Y. (2018). Halogenated and Nonhalogenated Metabolites from the marine-alga-endophytic Fungus Trichoderma Asperellum Cf44-2. Mar. Drugs 16, 266. doi:10.3390/md16080266
Song, X.-Q., Yu, J.-H., Sun, J., Liu, K.-L., Zhang, J.-S., and Zhang, H. (2021). Bioactive Sesquiterpenoids from the Flower Buds of Tussilago Farfara. Bioorg. Chem. 107, 104632. doi:10.1016/j.bioorg.2021.104632
Sun, J.-Z., Chen, K.-S., Liu, H.-L., van Soest, R., and Guo, Y.-W. (2010). New Epoxy-Substituted Nitrogenous Bisabolene-type Sesquiterpenes from a Hainan SpongeAxinyssasp. Helvetica Chim. Acta 93, 517–521. doi:10.1002/hlca.200900261
Sun, L.-L., Shao, C.-L., Chen, J.-F., Guo, Z.-Y., Fu, X.-M., Chen, M., et al. (2012). New Bisabolane Sesquiterpenoids from a marine-derived Fungus Aspergillus Sp. Isolated from the Sponge Xestospongia Testudinaria. Bioorg. Med. Chem. Lett. 22, 1326–1329. doi:10.1016/j.bmcl.2011.12.083
Wang, P., Yu, J.-H., Zhu, K., Wang, Y., Cheng, Z.-Q., Jiang, C.-S., et al. (2018). Phenolic Bisabolane Sesquiterpenoids from a Thai Mangrove Endophytic Fungus, Aspergillus Sp. Xy02. Fitoterapia 127, 322–327. doi:10.1016/j.fitote.2018.02.031
Wang, J., Liu, L., Hong, L.-L., Zhan, K.-X., Lin, Z.-J., Jiao, W.-H., et al. (2021). New Bisabolane-type Phenolic Sesquiterpenoids from the marine Sponge Plakortis Simplex. Chin. J. Nat. Medicines 19, 626–631. doi:10.1016/s1875-5364(21)60062-6
Wei, M.-Y., Wang, C.-Y., Liu, Q.-A., Shao, C.-L., She, Z.-G., and Lin, Y.-C. (2010). Five Sesquiterpenoids from a marine-derived Fungus Aspergillus Sp. Isolated from a Gorgonian Dichotella Gemmacea. Mar. Drugs 8, 941–949. doi:10.3390/md8040941
Wongbundit, S., Mahidol, C., Kaweetripob, W., Prachyawarakorn, V., Eurtivong, C., Sahakitpichan, P., et al. (2020). Biscurcudiols, Myrmekioperoxides, and Myrmekiodermaral from the Thai marine Sponge Myrmekioderma Sp. Tetrahedron 76, 131162. doi:10.1016/j.tet.2020.131162
Wu, Y., Chen, Y., Huang, X., Pan, Y., Liu, Z., Yan, T., et al. (2018). α-Glucosidase Inhibitors: Diphenyl Ethers and Phenolic Bisabolane Sesquiterpenoids from the Mangrove Endophytic Fungus Aspergillus flavus QQSG-3. Mar. Drugs 16, 307. doi:10.3390/md16090307
Wu, J.-S., Yao, G.-S., Shi, X.-H., Rehman, S. U., Xu, Y., Fu, X.-M., et al. (2020). Epigenetic Agents Trigger the Production of Bioactive Nucleoside Derivatives and Bisabolane Sesquiterpenes from the marine-derived Fungus Aspergillus versicolor. Front. Microbiol. 11, 85. doi:10.3389/fmicb.2020.00085
Xiao-Dong Li, X.-D., Li, X.-M., Xu, G.-M., Zhang, P., and Wang, B.-G. (2015). Antimicrobial Phenolic Bisabolanes and Related Derivatives from Penicillium aculeatum SD-321, a Deep Sea Sediment-Derived Fungus. J. Nat. Prod. 78, 844–849. doi:10.1021/acs.jnatprod.5b00004
Zhang, P., Wei, Q., Yuan, X., and Xu, K. (2020). Newly Reported Alkaloids Produced by marine-derived Penicillium Species (Covering 2014-2018). Bioorg. Chem. 99, 103840. doi:10.1016/j.bioorg.2020.103840
Keywords: marine-derived bisabolanes, sesquiterpenoids, chemical diversity, biological activities, lead compounds
Citation: Li C-S, Liu L-T, Yang L, Li J and Dong X (2022) Chemistry and Bioactivity of Marine-Derived Bisabolane Sesquiterpenoids: A Review. Front. Chem. 10:881767. doi: 10.3389/fchem.2022.881767
Received: 23 February 2022; Accepted: 09 March 2022;
Published: 07 April 2022.
Edited by:
Xuetao Xu, Wuyi University, ChinaReviewed by:
Peng Zhang, Chinese Academy of Agricultural Sciences (CAAS), ChinaWeiyi Wang, State Oceanic Administration, China
Copyright © 2022 Li, Liu, Yang, Li and Dong. This is an open-access article distributed under the terms of the Creative Commons Attribution License (CC BY). The use, distribution or reproduction in other forums is permitted, provided the original author(s) and the copyright owner(s) are credited and that the original publication in this journal is cited, in accordance with accepted academic practice. No use, distribution or reproduction is permitted which does not comply with these terms.
*Correspondence: Jing Li, lijing20220128@163.com; Xin Dong, dongxin198901@163.com