Recent advances in hydrogels-based osteosarcoma therapy
- 1Department of Orthopedics, China-Japan Union Hospital of Jilin University, Changchun, China
- 2Department of Dermatology, China-Japan Union Hospital of Jilin University, Changchun, China
- 3Department of Laboratory Medicine, Nanfang Hospital, Southern Medical University, Guangzhou, China
- 4State Key Laboratory of Magnetic Resonance and Atomic and Molecular Physics, National Center for Magnetic Resonance in Wuhan, Innovation Academy for Precision Measurement Science and Technology, Chinese Academy of Science, Wuhan, China
Osteosarcoma (OS), as a typical kind of bone tumors, has a high incidence among adolescents. Traditional tumor eradication avenues for OS such as chemotherapy, surgical therapy and radiation therapy usually have their own drawbacks including recurrence and metastasis. In addition, another serious issue in the treatment of OS is bone repair because the bone after tumor invasion usually has difficulty in repairing itself. Hydrogels, as a synthetic or natural platform with a porous three-dimensional structure, can be applied as desirable platforms for OS treatment. They can not only be used as carriers for tumor therapeutic drugs but mimic the extracellular matrix for the growth and differentiation of mesenchymal stem cells (MSCs), thus providing tumor treatment and enhancing bone regeneration at the same time. This review focuses the application of hydrogels in OS suppression and bone regeneration, and give some suggests on future development.
Introduction
Osteosarcoma (OS), as a well-known primary bone tumor, involves the invasion of tumors into bone tissue and often occurs in children and adolescents (Mann et al., 2022). Reports suggested that OS has become the second leading cause of death among young cancer patients, especially to the stage of tumor lung metastasis (Siclari and Qin, 2010; Roessner et al., 2021). OS patients may suffer from disability and even death, eliciting heavy blows and losses to the society. Although developing quickly, there are often no obvious clinical signs or severe pain in the early stage of OS. Therefore, it’s critical but tricky for the diagnosis and treatment of OS. To date, clinical treatment strategies include allogeneic bone transplantation and mechanically processed prosthesis (Gianferante et al., 2017; Simpson and Brown, 2018). However, defects such as insufficient allogeneic bone sources and poor biocompatibility severely limit their applications. Besides, most OS can be clean up by surgical intervention, but usually fails to completely ablate the tumor, thus causing post-surgery recurrence and metastasis (Lin et al., 2015; Chen et al., 2017; Friesenbichler et al., 2017; Haghiralsadat et al., 2017; Liu et al., 2021; Wang et al., 2021; Xu et al., 2022). Thus, to avoid postoperative recurrence and metastasis as much as possible, chemotherapy and radiotherapy are combined after surgery. Unfortunately, radiotherapy is reluctant to exert effect in OS and OS is susceptible to chemotherapy resistance (Bohnke et al., 2007; Campbell, 2009; Gianferante et al., 2017; Shoag et al., 2019; Pattee et al., 2020). What’s more, the patients receiving chemotherapy often suffer from side effects including hair loss and vomiting, which will obviously decrease the quality of life (Bosma et al., 2018). At the same time, patients suffered from OS and surgical resection will have bone defects, eliciting acute pain and disability. Thus, implementing the development of OS therapy is a pretty tough work. Correspondingly, innovative and effective methods are urgently needed to guide the therapy of OS in clinical problems (Liao et al., 2021a).
As discussed above, it’s vital to ensure the complete resection of OS after surgery but remains difficulty. Besides, radical resection is dangerous because of the complex anatomical structure and blood vessels in the bone tissues (Wang et al., 2018a; Liu et al., 2019; Wang et al., 2019; Zhang et al., 2019; Yang et al., 2020a; Pan et al., 2020). Along with the development of biotechnology and nanotechnology, novel alternative strategies with less side effects are developed. Specifically, photothermal therapy (PTT) is becoming a promising method that can covert near-infrared (NIR) light into thermal damage in tumor tissues (Chu and Dupuy, 2014; Chen et al., 2016; Liu et al., 2019; Wang et al., 2020; Xu et al., 2021), rejecting tumor region without damaging other organs or tissues (Xing et al., 2016; Shan et al., 2018; Pan et al., 2019; Hou et al., 2020; Jiang et al., 2020). PTT is based on various nanoparticles such as gold (Li Volsi et al., 2017; Mahmoodzadeh et al., 2018; Liao et al., 2019), carbon (Du et al., 2019; Farzin et al., 2019; Guo et al., 2022; Zhang et al., 2022) and copper nanomaterials (Liu et al., 2018). For example, PTT using gold nanoparticles has desirable therapeutic efficacy for prostate cancer in clinical trials (Rastinehad et al., 2019; Taneja, 2020). However, these nanomaterials usually have unsatisfactory biocompatibility and limited bioavailability. Thus, an appropriate carrier is needed to avoid these defects of nanoparticles and benefit their biological applications. More importantly, bone metabolism is becoming unbalanced because of the invasion of tumors into bone, leading to bone defect that is difficult to repair itself (Velasco et al., 2015; Wang et al., 2018b; Khajuria et al., 2018; Zhang et al., 2018). Therefore, therapeutic drugs, growth factors and/or stem cells are urgently needed. Benefiting from the continuous development in biomaterials science, bone tissue engineering scaffolds become a fascinating material with great hope to bone regeneration (Gong et al., 2009; Ni et al., 2014; Li et al., 2018a; Shi et al., 2019). Among various tissue engineering scaffolds, hydrogels with excellent bioactivity, biocompatibility and biodegradability have attracted much attention of researchers. Hydrogels are three-dimensional porous mesh gel with abundant water absorbance (Xu et al., 2020). It can not only afford a vehicle for tumor therapeutic drug but mimic the extracellular matrix (ECM) for the growth and differentiation of mesenchymal stem cells (MSCs), thus providing tumor treatment and enhancing bone regeneration at the same time (Chen et al., 2020). Moreover, when integrating hydrogels with other drug delivery systems such as liposomes and microspheres, carriers with better performance can be created by synergism.
Herein, we discussed the recent advances in the use of hydrogels to achieve OS therapy, with emphasis on suppressing the tumors and Promoting bone regeneration (Scheme 1). We believe that This review will provide a useful reference for hydrogels-based OS therapy and the field of complex diseases to combine tumor therapy and tissue engineering.
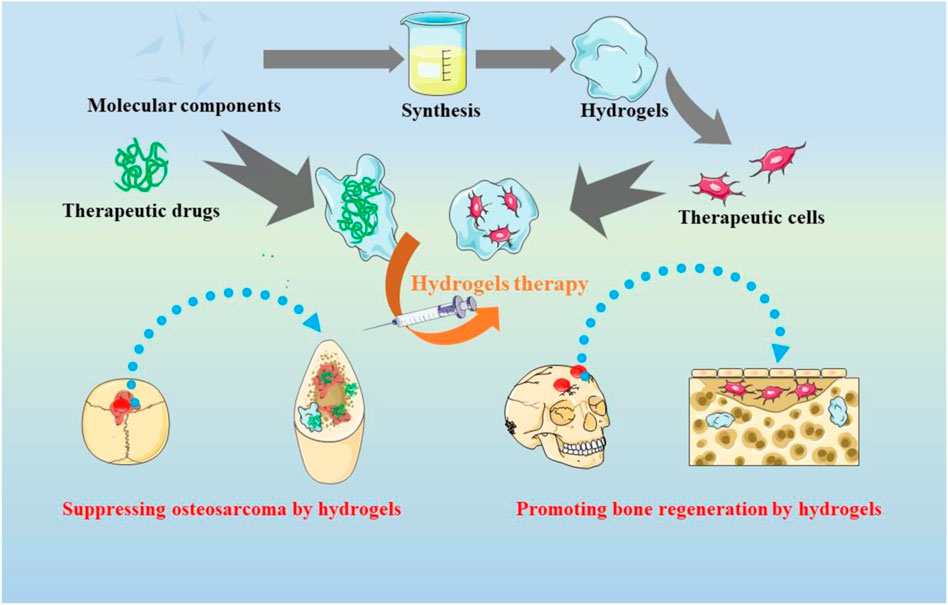
SCHEME 1. The applications of hydrogels-based therapeutics for suppressing the tumors and promoting bone regeneration in osteosarcoma treatment.
Suppressing the tumors by hydrogels
The conventional therapy strategies for OS comprise the combination of chemotherapy with surgical methods (Dou et al., 2013). Chemotherapy for OS began in the 1970s, which includes doxorubicin (DOX), cisplatin et al. Despite great expectations are expected in chemotherapy, the overall efficacy of which are no more than 60% (Lai et al., 2007) attributing to the high toxic effects of chemotherapy and drug resistance in secondary cancer. Therefore, it’s crucial to construct an artificial implant for the local administration and controlled-release of chemical drugs (Wu et al., 2018).
Several researches have demonstrated that hydrogels are capable of treating tumors due to their porous structure and versatile biocompatibility. It’s acceptable to administer therapeutic drugs or functional cells into the resected OS area with the help of hydrogels (Yang et al., 2020b). With the advantages of providing continuous drug release for tumor illumination, hydrogels encapsulated with drugs can afford localized tumor therapy, replacing systemic chemotherapy administered intravenously (Zheng et al., 2017; Yang et al., 2018; Chen et al., 2019; Peng et al., 2022). As an example, chitosan-based hydrogels are designed for therapeutic agents and cell delivery for tumor therapy (Pan et al., 2019). Besides, thermoresponsive hydrogel based on PEG-g-chitosan (PCgel) can benefit T lymphocyte infiltration into the gel and allow a sustainable release of cells (Jiang et al., 2020). Further, reports have suggested the gelatin gel for the release of anti-carcinogenic drugs (Hu et al., 2018). Wu et al. united gelatin methacryloyl (GelMA) hydrogel with gemcitabine hydrochloride loaded liposomes for OS ablation, which exhibited desirable properties in antitumor and sustained release (Wu et al., 2018). Specifically, the hydrogel system showed feasible application in eradicating OS in vivo by MG63-bearing mice.
Because of the complexity and diversity of tumor pathogenesis, the effect of single chemical drug may be compromised. Thus, synergistic chemotherapy is needed to solve the problem. For instance, Combretastatin A-4 (CA4) are able to bind the tubulin of endothelial cells, disturbing the formation of blood vessel and ultimately, eliciting tumor necrosis through inhibiting the supply of oxygen and nutrients (Perez-Perez et al., 2016). Unfortunately, CA4 can only work on the internal tumors with rich vascular, but often fail to treat the edge of the tumor tissues. Nevertheless, the peripheral tumor tissues are sensitive to traditional drugs like DOX and docetaxel (DTX). For this issue, Zheng et al. developed an injectable thermosensitive hydrogel system for the co-encapsulation and sequential release of CA4 and DTX (Figure 1A) (Zheng et al., 2017). CA4 was released preferentially, which could damage the neovascularization system and inhibit the exchange of nutrients. The followed release of DTX could clean up the surface cells of tumor tissues and lead to apoptosis of the tumor (Figures 1B,C). Likewise, Sun et al. co-loaded Oxaliplatin (OXA) and Alendronate (ALN) onto mPEG45-PLV19 thermosensitive hydrogel (Sun et al., 2020). OXA is a widely accepted anticancer drug, which can induce immunogenic death (ICD) for tumor elimination. ALN have bone affinity as well as the effect of inhibiting bone destruction. Studies found that the system could inhibit the progress of OS and prevent tumor lung metastasis.
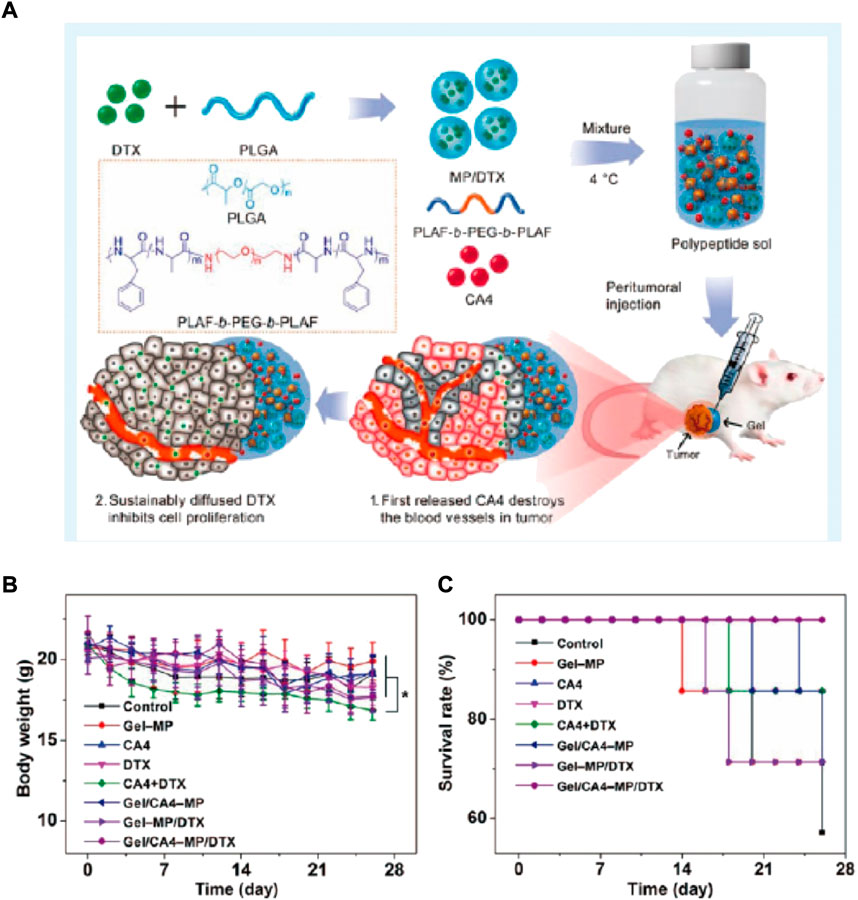
FIGURE 1. (A) Sequential Drug Delivery with Gel-MP Construct, Showing Preparation of Gel/CA4−MP/DTX for Two-Pronged Locally Synergistic Chemotherapy of Osteosarcoma. (B) Variations of body weight and survival rate. (A) Change of body weight and (B) survival rate of K7 osteosarcoma-grafted mice after treatment of PBS as control, Gel−MP, CA4, DTX, CA4+DTX, Gel/CA4−MP, Gel−MP/DTX, or Gel/CA4−MP/DTX. [Data were represented as mean ± SD (n = 10; (*) p < 0.05)].
Currently, the OS treatment regimen is mostly DOX, methotrexate (MTX), and cisplatin (CDDP) based. The clinical efficacy of this regimen was proved by the systemic administration of the aforementioned drugs (Bielack et al., 2009). Ma et al. successfully established a system based on poly (L-lactide-co-glycolide)-poly (ethylene glycol)-poly (lactide-co-glycolide) (PLGA-PEG-PLGA) for delivering CDDP, MTX, and DOX (Ma et al., 2015). In the human osteosarcoma model of nude mice, the triple-delivery system could induce enhanced tumor apoptosis, displaying high tumor suppression efficacy. Furthermore, the evaluation of alteration in mice’s bodies and their organs histological analysis in ex vivo experiment revealed less toxic effects and obvious organ damage after localized treatment. Therefore, local co-delivery of CDDP, MTX, and DOX via thermos-sensitive hydrogels might be a promising option for better osteosarcoma treatment. “Smart” hydrogels are novel biomaterials that are influenced by external stimuli. Thus, multiple investigations have been carried out to determine the scope of bio-medical implementations, for instance, regenerative engineering and therapeutic delivery. Jalili et al. established nano-engineered hydrogel comprising poly (NIPAM-co-AM)/MNPs, for local and on-demand injection for delivering drugs (doxorubicin (DOX)) (Figure 2A). In this investigation, shear-thinning hydrogels capable of self-recovering were engineered by manipulating gelatin methacrylate (GelMA) network’s crosslinking density. Prior to this crosslinking GelMA pre-polymer solution was mixed with DOX-loaded Poly (NIPAM-co-AM)/MNP nanogels (GelMA/(poly (NIPAM-co-AM)/MNPs)). The magnetic field and temperature-dependent DOX release from (GelMA/(poly (NIPAM-co-AM)/MNPs)) were evaluated. Lastly, the efficacy of this new form of DOX-carrying drug on pre-osteoblast and osteosarcoma cells was investigated in vivo (Figure 2B).
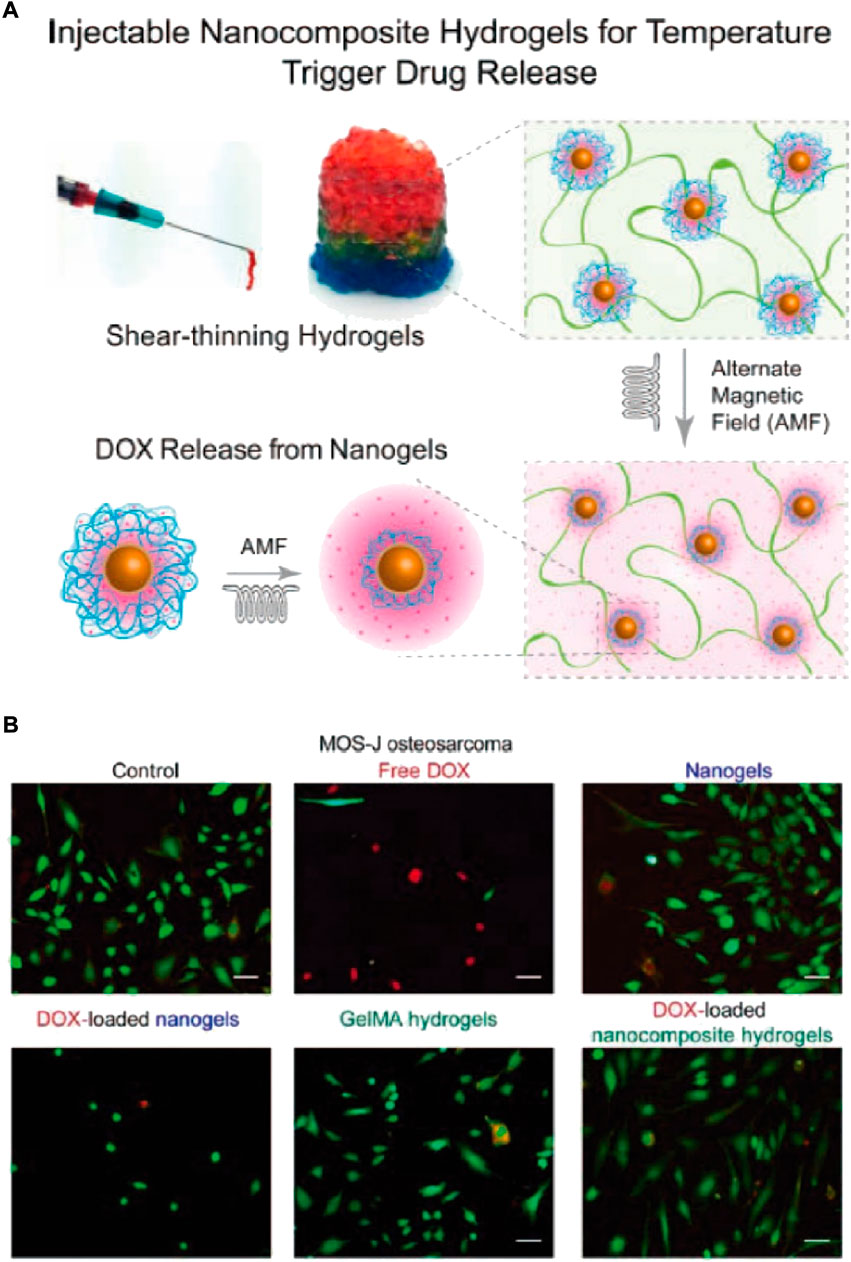
FIGURE 2. (A) Schematic depiction of nanocomposite hydrogels fabrication that can be injected for drug release in response to stimuli. (B) MOS-Js, live or dead staining indicated more dead cells upon exposure with free DOX than nanogels and nanocomposites loaded DOX (Scale bar 100 μm).
Currently, the therapeutic drug’s maximum tolerated dose (MTD) is not only important for determining the formulation’s concentration, but also for predicting its success in the clinical setting (Kim et al., 2010; Ranade et al., 2014). Even though the MTD of some drugs showed improvement to some extent through the polymer-regulated delivery mechanism, the MTD of drugs that are delivered by hydrogel still needs additional research. For localized OS treatment, Yang et al. used thermo-sensitive hydrogel to incorporate DOX into the poly (D,Llactide-co-glycolide)-poly (ethylene glycol)-poly (D,L-lactide-co-glycolide) (PLGA-PEG-PLGA) (Yang et al., 2018). The PLGA-PEG-PLGA triblock copolymer was successfully prepared and proved by 1H NMR. Furthermore, hydrogel characters, including rheological evaluation, sol-gel phase transition, and drug release in the in vitro experiment were studied. The DOX-packed hydrogel’s cytotoxicity was evaluated in vitro, in K-7 (mouse osteosarcoma cancer) and Saos-2 (human osteosarcoma cancer) cells. Lastly, the DOX-loaded hydrogel’s antitumor efficacy was determined in vivo in the K-7 mice tumor model. DOX-loaded hydrogel’s systemic toxicity and the safety of its local delivery were assessed by mice’s organ pathological analysis and their survival rate. Similarly, Yu et al. suggested a procedure for local Sun and chlorin e6 (Ce6) delivery by zwitterionic redox-responsive hydrogels for preventing the relapse of osteosarcoma (Figure 3A) (Yu et al., 2020). Yu synthesized hydrogels using a redox-responsive cross-linker (DSDMA), in which drugs Ce6 and Sun were introduced, a complex called Sun/Ce6@Gel (Figure 3B). This composite was administered in the residual cavity instantly post tumor eradication. Ce6 and Sun are liberated from the zwitterionic hydrogels because of redox sensitivity post-implantation at the surgery site (Figure 3C). This should result in reduced anti-apoptotic and increased pro-apoptotic gene expression. The potency of Ce6-and Sun-packed hydrogel as a postoperative osteosarcoma therapy was determined in both in vitro and in vivo conditions (Figures 3D–F).
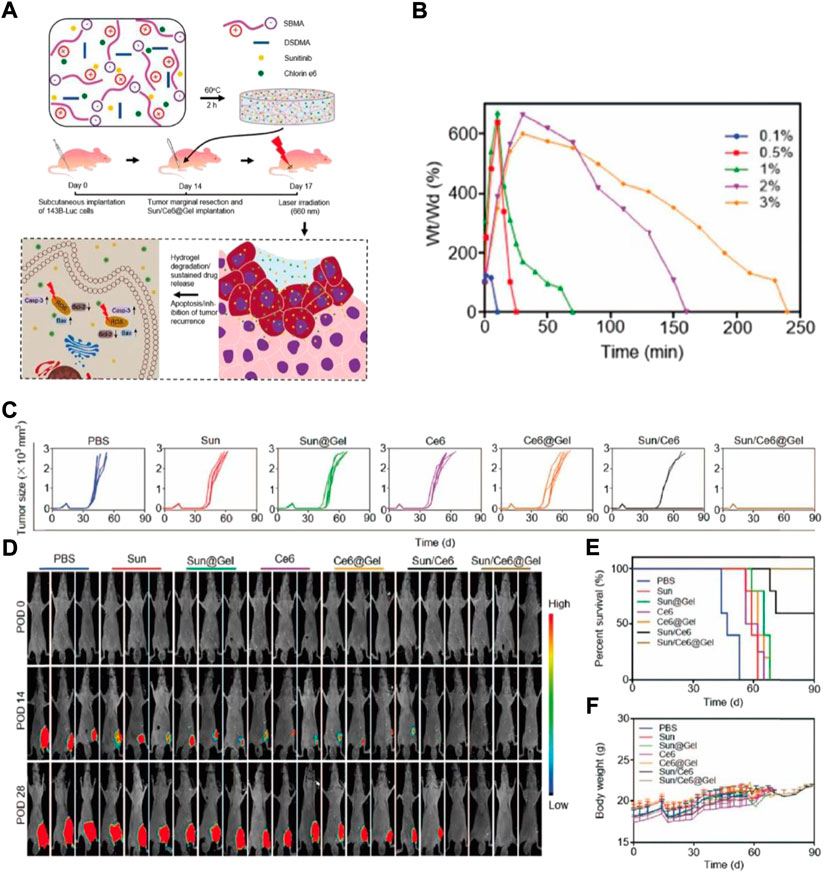
FIGURE 3. (A) Schematic depiction of Sun/Ce6@Gel synthesis and Sun and Ce6 synergistic antitumor impact in inhibiting mice tumor recurrence. Sun/Ce6@gel was generated by fusing Ce6, Sun, DSDMA, and SBMA for 2 h, and was administered in the residual cavity, thereby it inhibited tumor relapse post osteosarcoma marginal eradication. Casp-3: caspase-3. The degeneration and DSDMA–SBMA hydrogels release data. (B) Hydrated DSDMA–SBMA hydrogels weight alternations in the solution of PBS supplemented with DTT (100 mM). (C) Individual tumor growth kinetics during the treatment. (D) Invivo nude mice tumor recurrence bioluminescence images. POD, postoperative days. (E) Survival rates (F) Mice’s body weights were measured, and the effective doses of Sun and Ce65 were selected as 5mg and 1 mg per kg, respectively.
Promoting bone regeneration by hydrogels
Osteosarcoma originates at the epiphyseal end with a rich blood supply. The osteosarcoma effect on bone is huge, and the body takes time to repair or heal itself. Therefore, stem cells, small-molecule, external scaffolds, or growth factor drugs are required (Velasco et al., 2015; Wang et al., 2018b). Recently, computer-assisted digital technology, material mechanics, and bone tissue engineering scaffolds (e.g., 3D printed scaffolds, microspheres, and hydrogels) have progressed a lot with the continuous advancement in the field of biomaterials (Li et al., 2017; Xu et al., 2017). Hydrogels can imitate extracellular matrix (ECM) and improve bone repair by proliferating and differentiating MSCs (Liu et al., 2022). Thus, bone regeneration has widely been studied because of its outstanding osteoinductivity and bio-compatibility, -activity, and—degradability (Feng et al., 2019). Yap et al. established a novel thermoreversible hydrogel scaffold comprising glyoxal (Gx), PLuronic F127, and carboxymethyl hexanoyl chitosan (CA), injected for encapsulating human osteosarcoma MG-63 cells. These hydrogel encapsulated cells proliferated >400% during a 5-day incubation period. The results suggest that F127/CA/Gx hydrogel can envelop cells for tissue engineering (Yap and Yang, 2020). Prosthodontic-inspired photopolymerization stimulated by blue light is a gentle process for initiating the polymerization of monomers (Chen et al., 2020; Downs et al., 2020; Feng et al., 2022), The blue light initiator in the hydrogel system commences hydrogel cross-linking. Human Bone contains 50%–70% inorganic calcium and phosphorus. Nano hydroxyapatite (Ca10(PO4)6(OH)2, nHA) has been proven to provide nutrition for bone defects and also help repair bone (Li et al., 2018b; Tan et al., 2021). However, nHA can also inhibit tumors (Barbanente et al., 2021). The hybrid nHA hydrogel is hypothesized to furnish an ECM mimic post osteosarcoma eradication and stimulate bone defect restoration. Liao et al. used light-induced photopolymerization for developing GNRs/nHA hybrid hydrogel (Figure 4A) (Liao et al., 2021b). To generate a biocompatible hydrogel, methacrylate gelatin (GelMA) and methacrylated chondroitin sulfate (CSMA) were used. The nHA and GNRs dissipated easily in the hydrogel. The developed GelMA/CSMA hydrogel, GNRs/nHA hybrid were used for eradicating the residual tumor after surgery via PTT and for healing defects after bone tumor surgical resection (Figure 4B). The GelMA/CSMA hydrogel photothermally treated tumors residues left after surgery and repaired the bone deformities in a tibia osteosarcoma mice model (Figures 4C–E).
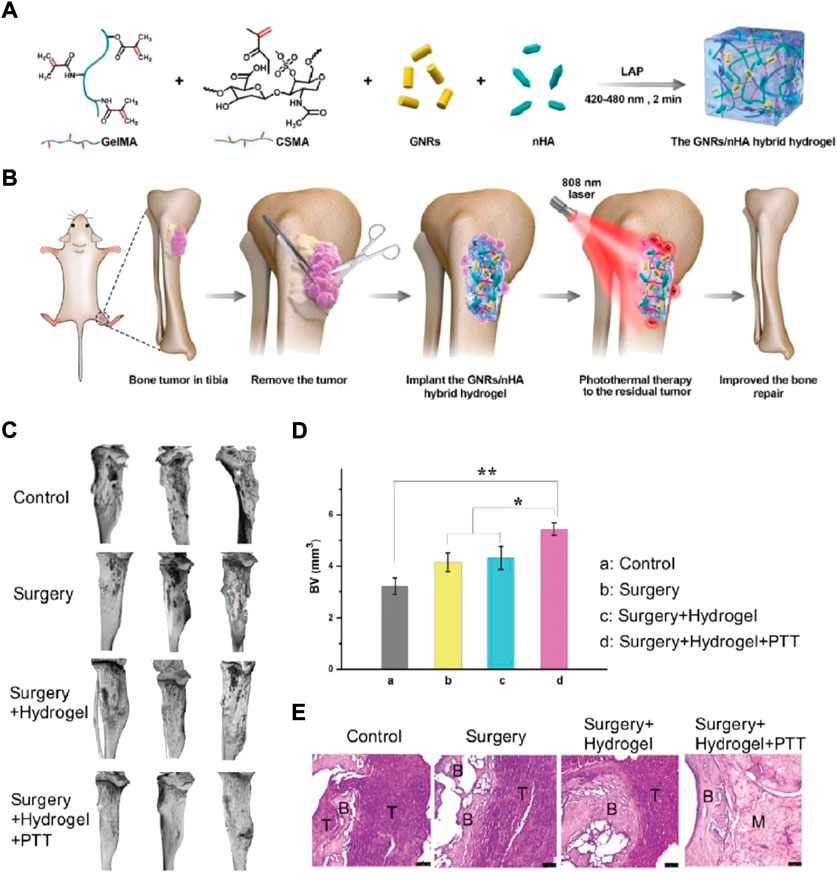
FIGURE 4. (A) The diagrammatic GNRs/nHA hybrid hydrogel preparation. (B) The hybrid GNRs/nHA hydrogel application for photothermal treatment and regeneration of bone tumor. (C) The micro-CT reconstruction in each group of mice after treatment for 2 weeks; (D) The bone volume (BV) parameter in each mice group (*p < 0.05, **p < 0.01); (E) The tibia bone tumor H&E stained images of different groups.
Osteocytes are crucial for the bone remodeling process, during which the trapped osteoblasts phenotypically alter to mature as osteocytes. The underlying osteocyte mechanisms are still debatable, and it has few study models. E. J. Lee et al. studied how alterations in the mechanical features of bone matrix that lack minerals can affect the phenotypic transformation of osteoblast to osteocyte in a 3D setting via bioprinting-based technology called Combing Extrusion printing on Cellulose scaffolds with Lamination (ExCeL) (Lee et al., 2019). Similarly, Vashisth et al. established a biomimetic 3D hybrid scaffold after studying the natural bone architecture with nano-microscale features, favorable porous interconnected structure, and mechanical strength (Vashisth and Bellare, 2018). The key hybrid scaffold constituents are core-sheath nanofibers and hydrogel, which are organized suitably to generate a microenvironment that resembles bone. The core-sheath nanofibers are specifically coiled tightly into a ring to imitate the osteon and reinforced in a hydrogel matrix.
In comparison with traditional biometal scaffolds (like that of titanium and titanium-based alloys), Young’s polyetheretherketone (PEEK) model resembles more human cortical bone, thereby, alleviating osteoporosis and osteonecrosis risk triggered by stress shielding (Wang et al., 2014; Torstrick et al., 2018). Based on this, Yin et al. fabricated a novel and versatile coating made from GelMA hydrogels and TOB-laden MXene nanosheets on an inert orthopedic PEEK material, to eliminate remaining cancerous cells, prevent infection related to bacteria, and guide the regeneration of bone tissues (Yin et al., 2020).
How cancerous cells and their normal counterparts have anchorage-dependency and react to the stiffness and adhesion ligand density of the same ECM is still unclear. Jiang et al. analyzed the impact of ECM adhesion ligand density and stiffness on osteosarcoma cells (bone cancerous cells) and osteoblasts (bone-producing cells) via poly (ethylene glycol) diacrylate (PEGDA) and GelMA hydrogels (Figure 5A) (Jiang et al., 2019). When osteosarcoma cells were cultured in 3D PEGDA/GelMA hydrogel matrix, they showed high dependence on the stiffness of the matrix by modulating the integrin-induced pathway of focal adhesion (FA), whereas osteoblasts showed high sensitivity toward matrix adhesion ligand density by modulating the integrin-induced pathway of adherens junction (AJ) (Figure 5B). But in the 2D hydrogels surface culture, bone cancerous cells presented a different behavior and showed sensitivity to the matrix adhesion ligand density due to their “forced” attachment to the substrate, similar to anchorage-dependent osteoblasts.
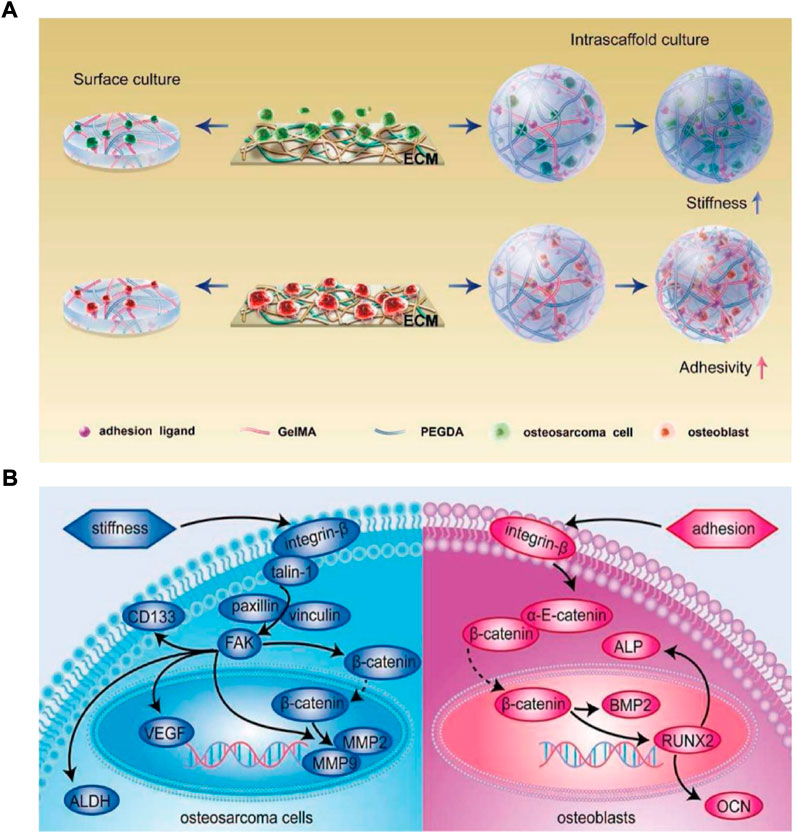
FIGURE 5. (A) Schematic illustration of various anchorage-independent osteosarcoma cells and anchorage-dependent osteoblasts responses to adhesive activity and stiffness of scaffold (B) Respond of osteoblasts and osteosarcoma cell’s mechanisms to stiffness and adhesion ligands of ECM, respectively. ECM’s stiffness and not the matrix adhesion influences the growth of osteosarcoma cells by regulating the integrin-induced FA signaling pathway, while osteoblasts are primarily influenced by ECM adhesion ligands via the integrin-induced AJ signaling pathway modulation.
Conclusion and prospects
Hydrogels are enormous water meshes that characteristically resemble extracellular matrix. These are very porous and have excellent biological compatibility and degradability. They are capable of introducing growth factors that can repair bone defects (Torstrick et al., 2018; Zhang et al., 2018). Therefore, they are good suiters for repairing bone. Literature indicates potential hydrogels application for regenerating bone tissue. For potential bone cancer therapy, these should first be capable of curing tumors. Administering drugs or other molecules directly at the resected tumor site for treatment is highly advised. Hydrogels provide sustainable drug release for tumor eradication (Ali Gumustas et al., 2016; Hu et al., 2020). Some act by delivering the drug directly to the specific system 126. Localized hydrogel therapy for cancer treatment can replace systemic chemotherapy given orally or intravenously (Zheng et al., 2017; Yang et al., 2018; Chen et al., 2019). With the discovery of new hydrogel functions, their implementations are no longer limited to repairing tissues, it has extended to bone repair and tumor eradication (Table 1).
However, hydrogel’s application in osteosarcoma is limited for the following reasons, first, despite extensive literature research on hydrogels, clinical applications have encountered bottlenecks, and only a few hydrogels have been approved and commercialized (Fan et al., 2022; Li et al., 2022). Additionally, cytotoxicity is often stimulated because of the hydrogel’s inorganic nature and the metal ions involved. Hydrogels are mainly developed from raw materials that are non-essential to organisms. With further investigations solving the aforementioned issues associated with osteosarcoma therapy-related hydrogels, it is expected that a promising candidate might be discovered that would contribute to human health and well-being.
Author contributions
HT and JZ: manuscript preparation, figures and tables preparation, and manuscript editing and revision. JZ: literature collection and evaluation, and draft manuscript preparation. RW and NF: figure legend preparation, references insertion, and graphic abstract preparation.
Acknowledgments
We greatly acknowledge the financial support from Science and Technology Development Program of Jilin Provinces (Nos. YDZJ202201ZYTS043, and YDZJ202201ZYTS520), State Key Laboratory of Magnetic Resonance and Atomic and Molecular Physics, National Center for Magnetic Resonance in Wuhan, Innovation Academy for Precision Measurement Science and Technology (T152205).
Conflict of interest
The authors declare that the research was conducted in the absence of any commercial or financial relationships that could be construed as a potential conflict of interest.
Publisher’s note
All claims expressed in this article are solely those of the authors and do not necessarily represent those of their affiliated organizations, or those of the publisher, the editors and the reviewers. Any product that may be evaluated in this article, or claim that may be made by its manufacturer, is not guaranteed or endorsed by the publisher.
References
Ali Gumustas, S., Isyar, M., Topuk, S., Yilmaz, I., Oznam, K., Onay, T., et al. (2016). Systematic evaluation of drug-loaded hydrogels for application in osteosarcoma treatment. Curr. Pharm. Biotechnol. 17 (10), 866–872. doi:10.2174/1389201017666160519113104
Barbanente, A., Palazzo, B., Esposti, L. D., Adamiano, A., Iafisco, M., Ditaranto, N., et al. (2021). Selenium-doped hydroxyapatite nanoparticles for potential application in bone tumor therapy. J. Inorg. Biochem. 215, 111334. doi:10.1016/j.jinorgbio.2020.111334
Bielack, S., Jurgens, H., Jundt, G., Kevric, M., Kuhne, T., Reichardt, P., et al. (2009). Osteosarcoma: The COSS experience. Cancer Treat. Res. 152, 289–308. doi:10.1007/978-1-4419-0284-9_15
Bohnke, A., Jung, J., Taubert, H., Hauptmann, S., and Bartel, F. (2007). Both somatic and germline genetics of the TP53-pathway influence ovarian cancer incidence and survival. Verh. Dtsch. Ges. Pathol. 91, 233–242.
Bosma, S. E., Wong, K. C., Paul, L., Gerbers, J. G., and Jutte, P. C. (2018). A cadaveric comparative study on the surgical accuracy of freehand, computer navigation, and patient-specific instruments in joint-preserving bone tumor resections. Sarcoma 2018, 1–9. doi:10.1155/2018/4065846
Campbell, P. J. (2009). Somatic and germline genetics at the JAK2 locus. Nat. Genet. 41 (4), 385–386. doi:10.1038/ng0409-385
Chen, Q., Xu, L., Liang, C., Wang, C., Peng, R., and Liu, Z. (2016). Photothermal therapy with immune-adjuvant nanoparticles together with checkpoint blockade for effective cancer immunotherapy. Nat. Commun. 7, 13193. doi:10.1038/ncomms13193
Chen, X., Chen, Y., Jiang, J., Wu, L., Yin, S., Miao, X., et al. (2017). Nano-pulse stimulation (NPS) ablate tumors and inhibit lung metastasis on both canine spontaneous osteosarcoma and murine transplanted hepatocellular carcinoma with high metastatic potential. Oncotarget 8 (27), 44032–44039. doi:10.18632/oncotarget.17178
Chen, Y., Hao, Y., Huang, Y., Wu, W., Liu, X., Li, Y., et al. (2019). An injectable, near-infrared light-responsive click cross-linked azobenzene hydrogel for breast cancer chemotherapy. J. Biomed. Nanotechnol. 15 (9), 1923–1936. doi:10.1166/jbn.2019.2821
Chen, Y., Zhang, J., Liu, X., Wang, S., Tao, J., Huang, Y., et al. (2020). Noninvasive in vivo 3D bioprinting. Sci. Adv. 6 (23), eaba7406. doi:10.1126/sciadv.aba7406
Chu, K. F., and Dupuy, D. E. (2014). Thermal ablation of tumours: Biological mechanisms and advances in therapy. Nat. Rev. Cancer 14 (3), 199–208. doi:10.1038/nrc3672
Dou, X. Q., Zhang, D., and Feng, C. L. (2013). Wettability of supramolecular nanofibers for controlled cell adhesion and proliferation. Langmuir 29 (49), 15359–15366. doi:10.1021/la4040276
Downs, F. G., Lunn, D. J., Booth, M. J., Sauer, J. B., Ramsay, W. J., Klemperer, R. G., et al. (2020). Multi-responsive hydrogel structures from patterned droplet networks. Nat. Chem. 12 (4), 363–371. doi:10.1038/s41557-020-0444-1
Du, Y., Liu, X., Liang, Q., Liang, X. J., and Tian, J. (2019). Optimization and design of magnetic ferrite nanoparticles with uniform tumor distribution for highly sensitive MRI/MPI performance and improved magnetic hyperthermia therapy. Nano Lett. 19 (6), 3618–3626. doi:10.1021/acs.nanolett.9b00630
Fan, Z., Jiang, C., Wang, Y., Wang, K., Marsh, J., Zhang, D., et al. (2022). Engineered extracellular vesicles as intelligent nanosystems for next-generation nanomedicine. Nanoscale Horiz. 7 (7), 682–714. doi:10.1039/d2nh00070a
Farzin, A., Hassan, S., Emadi, R., Etesami, S. A., and Ai, J. (2019). Comparative evaluation of magnetic hyperthermia performance and biocompatibility of magnetite and novel Fe-doped hardystonite nanoparticles for potential bone cancer therapy. Mater. Sci. Eng. C 98, 930–938. doi:10.1016/j.msec.2019.01.038
Feng, Q., Xu, J., Zhang, K., Yao, H., Zheng, N., Zheng, L., et al. (2019). Dynamic and cell-infiltratable hydrogels as injectable carrier of therapeutic cells and drugs for treating challenging bone defects. ACS Cent. Sci. 5 (3), 440–450. doi:10.1021/acscentsci.8b00764
Feng, T., Wu, H., Ma, W., Wang, Z., Wang, C., Wang, Y., et al. (2022). An injectable thermosensitive hydrogel with a self-assembled peptide coupled with an antimicrobial peptide for enhanced wound healing. J. Mat. Chem. B 10 (32), 6143–6157. doi:10.1039/d2tb00644h
Friesenbichler, J., Maurer-Ertl, W., Bergovec, M., Holzer, L. A., Ogris, K., Leitner, L., et al. (2017). Clinical experience with the artificial bone graft substitute Calcibon used following curettage of benign and low-grade malignant bone tumors. Sci. Rep. 7 (1), 1736. doi:10.1038/s41598-017-02048-w
Gianferante, D. M., Mirabello, L., and Savage, S. A. (2017). Germline and somatic genetics of osteosarcoma - connecting aetiology, biology and therapy. Nat. Rev. Endocrinol. 13 (8), 480–491. doi:10.1038/nrendo.2017.16
Gong, C. Y., Shi, S., Peng, X. Y., Kan, B., Yang, L., Huang, M. J., et al. (2009). Biodegradable thermosensitive injectable PEG-PCL-PEG hydrogel for bFGF antigen delivery to improve humoral immunity. Growth factors. 27 (6), 377–383. doi:10.3109/08977190903159938
Guo, J., Wei, W., Zhao, Y., and Dai, H. (2022). Iron oxide nanoparticles with photothermal performance and enhanced nanozyme activity for bacteria-infected wound therapy. Regen. Biomater. 9, rbac041. doi:10.1093/rb/rbac041
Haghiralsadat, F., Amoabediny, G., Sheikhha, M. H., Forouzanfar, T., Helder, M. N., and Zandieh-Doulabi, B. (2017). A novel approach on drug delivery: Investigation of A new nano-formulation of liposomal doxorubicin and biological evaluation of entrapped doxorubicin on various osteosarcoma cell lines. Cell J. 19 (1), 55–65. doi:10.22074/cellj.2017.4502
Hou, M., Liu, W., Zhang, L., Zhang, L., Xu, Z., Cao, Y., et al. (2020). Responsive agarose hydrogel incorporated with natural humic acid and MnO2 nanoparticles for effective relief of tumor hypoxia and enhanced photo-induced tumor therapy. Biomater. Sci. 8 (1), 353–369. doi:10.1039/c9bm01472a
Hu, Q., Liu, M., Chen, G., Xu, Z., and Lv, Y. (2018). Demineralized bone scaffolds with tunable matrix stiffness for efficient bone integration. ACS Appl. Mat. Interfaces 10 (33), 27669–27680. doi:10.1021/acsami.8b08668
Hu, Y., Chen, X., Li, Z., Zheng, S., and Cheng, Y. (2020). Thermosensitive in situ gel containing luteolin micelles is a promising efficient agent for colorectal cancer peritoneal metastasis treatment. J. Biomed. Nanotechnol. 16 (1), 54–64. doi:10.1166/jbn.2020.2870
Jiang, T., Zhao, J., Yu, S., Mao, Z., Gao, C., Zhu, Y., et al. (2019). Untangling the response of bone tumor cells and bone forming cells to matrix stiffness and adhesion ligand density by means of hydrogels. Biomaterials 188, 130–143. doi:10.1016/j.biomaterials.2018.10.015
Jiang, Y. W., Gao, G., Hu, P., Liu, J. B., Guo, Y., Zhang, X., et al. (2020). Palladium nanosheet-knotted injectable hydrogels formed via palladium-sulfur bonding for synergistic chemo-photothermal therapy. Nanoscale 12 (1), 210–219. doi:10.1039/c9nr08454a
Khajuria, D. K., Kumar, V. B., Gigi, D., Gedanken, A., and Karasik, D. (2018). Accelerated bone regeneration by nitrogen-doped carbon dots functionalized with hydroxyapatite nanoparticles. ACS Appl. Mat. Interfaces 10 (23), 19373–19385. doi:10.1021/acsami.8b02792
Kim, S., Nishimoto, S. K., Bumgardner, J. D., Haggard, W. O., Gaber, M. W., and Yang, Y. (2010). A chitosan/β-glycerophosphate thermo-sensitive gel for the delivery of ellagic acid for the treatment of brain cancer. Biomaterials 31 (14), 4157–4166. doi:10.1016/j.biomaterials.2010.01.139
Lai, D., Chen, C. M., Chiu, F. Y., Chang, M. C., and Chen, T. H. (2007). Reconstruction of juxta-articular huge defects of distal femur with vascularized fibular bone graft and Ilizarov's distraction osteogenesis. J. Trauma Inj. Infect. Crit. Care 62 (1), 166–173. doi:10.1097/ta.0b013e31802dccdb
Lee, B. E. J., Shahin-Shamsabadi, A., Wong, M. K., Raha, S., Selvaganapathy, P. R., and Grandfield, K. (2019). A bioprinted in vitro model for osteoblast to osteocyte transformation by changing mechanical properties of the ECM. Adv. Biosyst. 3 (10), e1900126. doi:10.1002/adbi.201900126
Li, C., Armstrong, J. P., Pence, I. J., Kit-Anan, W., Puetzer, J. L., Correia Carreira, S., et al. (2018). Glycosylated superparamagnetic nanoparticle gradients for osteochondral tissue engineering. Biomaterials 176, 24–33. doi:10.1016/j.biomaterials.2018.05.029
Li, D., Nie, W., Chen, L., McCoul, D., Liu, D., Zhang, X., et al. (2018). Self-Assembled hydroxyapatite-graphene scaffold for photothermal cancer therapy and bone regeneration. J. Biomed. Nanotechnol. 14 (12), 2003–2017. doi:10.1166/jbn.2018.2646
Li, Qing, Shao, Xinxin, Dai, Xianglin, Guo, Qiong, Yuan, Bolei, Liu, Ying, et al. (2022). Recent trends in the development of hydrogel therapeutics for the treatment of central nervous system disorders. NPG Asia Mat. 14, 14. doi:10.1038/s41427-022-00362-y
Li Volsi, A., Scialabba, C., Vetri, V., Cavallaro, G., Licciardi, M., and Giammona, G. (2017). Near-infrared light responsive folate targeted gold nanorods for combined photothermal-chemotherapy of osteosarcoma. ACS Appl. Mat. Interfaces 9 (16), 14453–14469. doi:10.1021/acsami.7b03711
Li, Y., Xiao, Y., and Liu, C. (2017). The horizon of materiobiology: A perspective on material-guided cell behaviors and tissue engineering. Chem. Rev. 117 (5), 4376–4421. doi:10.1021/acs.chemrev.6b00654
Liao, J., Han, R., Wu, Y., and Qian, Z. (2021). Review of a new bone tumor therapy strategy based on bifunctional biomaterials. Bone Res. 9 (1), 18. doi:10.1038/s41413-021-00139-z
Liao, J., Jia, Y., Chen, L., Zhou, L., Li, Q., Qian, Z., et al. (2019). Magnetic/gold core-shell hybrid particles for targeting and imaging-guided photothermal cancer therapy. J. Biomed. Nanotechnol. 15 (10), 2072–2089. doi:10.1166/jbn.2019.2839
Liao, J., Shi, K., Jia, Y., Wu, Y., and Qian, Z. (2021). Gold nanorods and nanohydroxyapatite hybrid hydrogel for preventing bone tumor recurrence via postoperative photothermal therapy and bone regeneration promotion. Bioact. Mat. 6 (8), 2221–2230. doi:10.1016/j.bioactmat.2021.01.006
Lin, L., Hao, R., Xiong, W., and Zhong, J. (2015). Quantitative analyses of the effect of silk fibroin/nano-hydroxyapatite composites on osteogenic differentiation of MG-63 human osteosarcoma cells. J. Biosci. Bioeng. 119 (5), 591–595. doi:10.1016/j.jbiosc.2014.10.009
Liu, Q., Qian, Y., Li, P., Zhang, S., Wang, Z., Liu, J., et al. (2018). The combined therapeutic effects of (131)iodine-labeled multifunctional copper sulfide-loaded microspheres in treating breast cancer. Acta Pharm. Sin. B 8 (3), 371–380. doi:10.1016/j.apsb.2018.04.001
Liu, Y., Bhattarai, P., Dai, Z., and Chen, X. (2019). Photothermal therapy and photoacoustic imaging via nanotheranostics in fighting cancer. Chem. Soc. Rev. 48 (7), 2053–2108. doi:10.1039/c8cs00618k
Liu, Y., Li, Q., Bai, Q., and Jiang, W. (2021). Advances of smart nano-drug delivery systems in osteosarcoma treatment. J. Mat. Chem. B 9 (27), 5439–5450. doi:10.1039/d1tb00566a
Liu, Y., Wang, Q., Liu, X., Nakielski, P., Pierini, F., Li, X., et al. (2022). Highly adhesive, stretchable and breathable gelatin methacryloyl-based nanofibrous hydrogels for wound dressings. ACS Appl. Bio Mat. 5 (3), 1047–1056. doi:10.1021/acsabm.1c01087
Ma, H., He, C., Cheng, Y., Yang, Z., Zang, J., Liu, J., et al. (2015). Localized Co-delivery of doxorubicin, cisplatin, and methotrexate by thermosensitive hydrogels for enhanced osteosarcoma treatment. ACS Appl. Mat. Interfaces 7 (49), 27040–27048. doi:10.1021/acsami.5b09112
Mahmoodzadeh, F., Abbasian, M., Jaymand, M., Salehi, R., and Bagherzadeh-Khajehmarjan, E. (2018). A novel gold-based stimuli-responsive theranostic nanomedicine for chemo-photothermal therapy of solid tumors. Mater. Sci. Eng. C 93, 880–889. doi:10.1016/j.msec.2018.08.067
Mann, K., Waters, A. R., Park, E. R., Perez, G. K., Vaca Lopez, P. L., Kaddas, H. K., et al. (2022). HIAYA CHAT study protocol: A randomized controlled trial of a health insurance education intervention for newly diagnosed adolescent and young adult cancer patients. Trials 23 (1), 682. doi:10.1186/s13063-022-06590-5
Ni, P., Ding, Q., Fan, M., Liao, J., Qian, Z., Luo, J., et al. (2014). Injectable thermosensitive PEG-PCL-PEG hydrogel/acellular bone matrix composite for bone regeneration in cranial defects. Biomaterials 35 (1), 236–248. doi:10.1016/j.biomaterials.2013.10.016
Pan, H., Zhang, C., Wang, T., Chen, J., and Sun, S. K. (2019). In situ fabrication of intelligent photothermal indocyanine green-alginate hydrogel for localized tumor ablation. ACS Appl. Mat. Interfaces 11 (3), 2782–2789. doi:10.1021/acsami.8b16517
Pan, S., Yin, J., Yu, L., Zhang, C., Zhu, Y., Gao, Y., et al. (2020). 2D MXene-integrated 3D-printing scaffolds for augmented osteosarcoma phototherapy and accelerated tissue reconstruction. Adv. Sci. (Weinh). 7 (2), 1901511. doi:10.1002/advs.201901511
Pattee, J., Zhan, X., Xiao, G., and Pan, W. (2020). Integrating germline and somatic genetics to identify genes associated with lung cancer. Genet. Epidemiol. 44 (3), 233–247. doi:10.1002/gepi.22275
Peng, Z., Li, M., Wang, Y., Yang, H., Wei, W., Liang, M., et al. (2022). Self-assembling imageable silk hydrogels for the focal treatment of osteosarcoma. Front. Cell Dev. Biol. 10, 698282. doi:10.3389/fcell.2022.698282
Perez-Perez, M. J., Priego, E. M., Bueno, O., Martins, M. S., Canela, M. D., and Liekens, S. (2016). Blocking blood flow to solid tumors by destabilizing tubulin: An approach to targeting tumor growth. J. Med. Chem. 59 (19), 8685–8711. doi:10.1021/acs.jmedchem.6b00463
Ranade, A. N., Ranpise, N. S., and Ramesh, C. (2014). Exploring the potential of gastro retentive dosage form in delivery of ellagic acid and aloe vera gel powder for treatment of gastric ulcers. Curr. Drug Deliv. 11 (2), 287–297. doi:10.2174/1567201810666131122153041
Rastinehad, A. R., Anastos, H., Wajswol, E., Winoker, J. S., Sfakianos, J. P., Doppalapudi, S. K., et al. (2019). Gold nanoshell-localized photothermal ablation of prostate tumors in a clinical pilot device study. Proc. Natl. Acad. Sci. U. S. A. 116 (37), 18590–18596. doi:10.1073/pnas.1906929116
Roessner, A., Lohmann, C., and Jechorek, D. (2021). Translational cell biology of highly malignant osteosarcoma. Pathol. Int. 71 (5), 291–303. doi:10.1111/pin.13080
Shan, W., Chen, R., Zhang, Q., Zhao, J., Chen, B., Zhou, X., et al. (2018). Improved stable indocyanine green (ICG)-Mediated cancer optotheranostics with naturalized hepatitis B core particles. Adv. Mat. 30 (28), e1707567. doi:10.1002/adma.201707567
Shi, R., Gong, M., Chi, C., Huang, Y., Li, W., Li, G., et al. (2019). Nano twin-fiber membrane with osteogenic and antibacterial dual functions as artificial periosteum for long bone repairing. J. Biomed. Nanotechnol. 15 (2), 272–287. doi:10.1166/jbn.2019.2687
Shoag, J. E., Wise, D. R., Sharaf, R. N., and Sternberg, C. N. (2019). Somatic and germline sequencing in genitourinary oncology: Genetics for the clinician. Curr. Opin. Urol. 29 (4), 315–318. doi:10.1097/mou.0000000000000643
Siclari, V. A., and Qin, L. (2010). Targeting the osteosarcoma cancer stem cell. J. Orthop. Surg. Res. 5, 78. doi:10.1186/1749-799x-5-78
Simpson, E., and Brown, H. L. (2018). Understanding osteosarcomas. J. Am. Acad. Physician Assist. 31 (8), 15–19. doi:10.1097/01.jaa.0000541477.24116.8d
Sun, Y., Li, K., Li, C., Zhang, Y., and Zhao, D. (2020). Thermogel delivers Oxaliplatin and alendronate in situ for synergistic osteosarcoma therapy. Front. Bioeng. Biotechnol. 8, 573962. doi:10.3389/fbioe.2020.573962
Tan, W., Gao, C., Feng, P., Liu, Q., Liu, C., Wang, Z., et al. (2021). Dual-functional scaffolds of poly(L-lactic acid)/nanohydroxyapatite encapsulated with metformin: Simultaneous enhancement of bone repair and bone tumor inhibition. Mater. Sci. Eng. C 120, 111592. doi:10.1016/j.msec.2020.111592
Taneja, S. S. (2020). Re: Gold nanoshell-localized photothermal ablation of prostate tumors in a clinical pilot device study. J. Urology 203 (1), 31. doi:10.1097/ju.0000000000000614
Torstrick, F. B., Lin, A. S. P., Potter, D., Safranski, D. L., Sulchek, T. A., Gall, K., et al. (2018). Porous PEEK improves the bone-implant interface compared to plasma-sprayed titanium coating on PEEK. Biomaterials 185, 106–116. doi:10.1016/j.biomaterials.2018.09.009
Vashisth, P., and Bellare, J. R. (2018). Development of hybrid scaffold with biomimetic 3D architecture for bone regeneration. Nanomedicine Nanotechnol. Biol. Med. 14 (4), 1325–1336. doi:10.1016/j.nano.2018.03.011
Velasco, M. A., Narvaez-Tovar, C. A., and Garzon-Alvarado, D. A. (2015). Design, materials, and mechanobiology of biodegradable scaffolds for bone tissue engineering. Biomed. Res. Int. 2015, 1–21. doi:10.1155/2015/729076
Wang, L., He, S., Wu, X., Liang, S., Mu, Z., Wei, J., et al. (2014). Polyetheretherketone/nano-fluorohydroxyapatite composite with antimicrobial activity and osseointegration properties. Biomaterials 35 (25), 6758–6775. doi:10.1016/j.biomaterials.2014.04.085
Wang, L., Long, N. J., Li, L., Lu, Y., Li, M., Cao, J., et al. (2019). Correction: Multi-functional bismuth-doped bioglasses: Combining bioactivity and photothermal response for bone tumor treatment and tissue repair. Light. Sci. Appl. 8, 54. doi:10.1038/s41377-019-0165-7
Wang, L., Long, N. J., Li, L., Lu, Y., Li, M., Cao, J., et al. (2018). Multi-functional bismuth-doped bioglasses: Combining bioactivity and photothermal response for bone tumor treatment and tissue repair. Light. Sci. Appl. 7, 1. doi:10.1038/s41377-018-0007-z
Wang, X., Shao, J., Abd El Raouf, M., Xie, H., Huang, H., Wang, H., et al. (2018). Near-infrared light-triggered drug delivery system based on black phosphorus for in vivo bone regeneration. Biomaterials 179, 164–174. doi:10.1016/j.biomaterials.2018.06.039
Wang, Y. C., Dai, H. L., Li, Z. H., Meng, Z. Y., Xiao, Y., and Zhao, Z. (2021). Mesoporous polydopamine-coated hydroxyapatite nano-composites for ROS-triggered nitric oxide-enhanced photothermal therapy of osteosarcoma. J. Mat. Chem. B 9 (36), 7401–7408. doi:10.1039/d1tb01084k
Wang, Z., Jia, T., Sun, Q., Kuang, Y., Liu, B., Xu, M., et al. (2020). Construction of Bi/phthalocyanine manganese nanocomposite for trimodal imaging directed photodynamic and photothermal therapy mediated by 808nm light. Biomaterials 228, 119569. doi:10.1016/j.biomaterials.2019.119569
Wu, W., Dai, Y., Liu, H., Cheng, R., Ni, Q., Ye, T., et al. (2018). Local release of gemcitabine via in situ UV-crosslinked lipid-strengthened hydrogel for inhibiting osteosarcoma. Drug Deliv. (Lond). 25 (1), 1642–1651. doi:10.1080/10717544.2018.1497105
Xing, R., Liu, K., Jiao, T., Zhang, N., Ma, K., Zhang, R., et al. (2016). An injectable self-assembling collagen-gold hybrid hydrogel for combinatorial antitumor photothermal/photodynamic therapy. Adv. Mat. 28 (19), 3669–3676. doi:10.1002/adma.201600284
Xu, B., Ye, J., Yuan, F. Z., Zhang, J. Y., Chen, Y. R., Fan, B. S., et al. (2020). Advances of stem cell-laden hydrogels with biomimetic microenvironment for osteochondral repair. Front. Bioeng. Biotechnol. 8, 247. doi:10.3389/fbioe.2020.00247
Xu, P., Hu, L., Yu, C., Yang, W., Kang, F., Zhang, M., et al. (2021). Unsymmetrical cyanine dye via in vivo hitchhiking endogenous albumin affords high-performance NIR-II/photoacoustic imaging and photothermal therapy. J. Nanobiotechnology 19 (1), 334. doi:10.1186/s12951-021-01075-0
Xu, T., Yang, H., Yang, D., and Yu, Z. Z. (2017). Polylactic acid nanofiber scaffold decorated with chitosan islandlike topography for bone tissue engineering. ACS Appl. Mat. Interfaces 9 (25), 21094–21104. doi:10.1021/acsami.7b01176
Xu, Y., Qi, J., Sun, W., Zhong, W., and Wu, H. (2022). Therapeutic effects of zoledronic acid-loaded hyaluronic acid/polyethylene glycol/nano-hydroxyapatite nanoparticles on osteosarcoma. Front. Bioeng. Biotechnol. 10, 897641. doi:10.3389/fbioe.2022.897641
Yang, Q., Yin, H., Xu, T., Zhu, D., Yin, J., Chen, Y., et al. (2020). Engineering 2D mesoporous Silica@MXene-integrated 3D-printing scaffolds for combinatory osteosarcoma therapy and NO-augmented bone regeneration. Small 16 (14), e1906814. doi:10.1002/smll.201906814
Yang, Z., Liu, J., and Lu, Y. (2020). Doxorubicin and CDCUR inclusion complex coloaded in thermosensitive hydrogel PLGAPEGPLGA localized administration for osteosarcoma. Int. J. Oncol. 57 (2), 433–444. doi:10.3892/ijo.2020.5067
Yang, Z., Yu, S., Li, D., Gong, Y., Zang, J., Liu, J., et al. (2018). The effect of PLGA-based hydrogel scaffold for improving the drug maximum-tolerated dose for in situ osteosarcoma treatment. Colloids Surfaces B Biointerfaces 172, 387–394. doi:10.1016/j.colsurfb.2018.08.048
Yap, L. S., and Yang, M. C. (2020). Thermo-reversible injectable hydrogel composing of pluronic F127 and carboxymethyl hexanoyl chitosan for cell-encapsulation. Colloids Surfaces B Biointerfaces 185, 110606. doi:10.1016/j.colsurfb.2019.110606
Yin, J., Han, Q., Zhang, J., Liu, Y., Gan, X., Xie, K., et al. (2020). MXene-based hydrogels endow polyetheretherketone with effective osteogenicity and combined treatment of osteosarcoma and bacterial infection. ACS Appl. Mat. Interfaces 12 (41), 45891–45903. doi:10.1021/acsami.0c14752
Yu, Z., Xiao, Z., Shuai, X., and Tian, J. (2020). Local delivery of sunitinib and Ce6 via redox-responsive zwitterionic hydrogels effectively prevents osteosarcoma recurrence. J. Mat. Chem. B 8 (30), 6418–6428. doi:10.1039/d0tb00970a
Zhang, K., Jia, Z., Yang, B., Feng, Q., Xu, X., Yuan, W., et al. (2018). Adaptable hydrogels mediate cofactor-assisted activation of biomarker-responsive drug delivery via positive feedback for enhanced tissue regeneration. Adv. Sci. (Weinh). 5 (12), 1800875. doi:10.1002/advs.201800875
Zhang, W., Gu, J., Li, K., Zhao, J., Ma, H., Wu, C., et al. (2019). A hydrogenated black TiO2 coating with excellent effects for photothermal therapy of bone tumor and bone regeneration. Mater. Sci. Eng. C 102, 458–470. doi:10.1016/j.msec.2019.04.025
Zhang, Y., Guo, Z., Zhu, H., Xing, W., Tao, P., Shang, W., et al. (2022). Synthesis of liquid Gallium@Reduced graphene oxide core-shell nanoparticles with enhanced photoacoustic and photothermal performance. J. Am. Chem. Soc. 144 (15), 6779–6790. doi:10.1021/jacs.2c00162
Keywords: osteosarcoma, bone repair, hydrogels, OS suppression, bone regeneration
Citation: Tian H, Wu R, Feng N, Zhang J and Zuo J (2022) Recent advances in hydrogels-based osteosarcoma therapy. Front. Bioeng. Biotechnol. 10:1042625. doi: 10.3389/fbioe.2022.1042625
Received: 12 September 2022; Accepted: 29 September 2022;
Published: 12 October 2022.
Edited by:
Qitong Huang, Gannan Medical University, ChinaReviewed by:
Zhijin Fan, Sun Yat-sen University, ChinaBoya Liu, Boston Children’s Hospital and Harvard Medical School, United States
Copyright © 2022 Tian, Wu, Feng, Zhang and Zuo. This is an open-access article distributed under the terms of the Creative Commons Attribution License (CC BY). The use, distribution or reproduction in other forums is permitted, provided the original author(s) and the copyright owner(s) are credited and that the original publication in this journal is cited, in accordance with accepted academic practice. No use, distribution or reproduction is permitted which does not comply with these terms.
*Correspondence: Jinrui Zhang, jlzhangjr@jlu.edu.cn; Jianlin Zuo, zuojl@jlu.edu.cn