Engineered stem cell exosomes for oral and maxillofacial wound healing
- 1Department of Oral and Maxillofacial Surgery, Hospital of Stomatology, Jilin University, Changchun, China
- 2Jilin Provincial Key Laboratory of Tooth Development and Bone Remodeling, Hospital of Stomatology, Jilin University, Changchun, China
- 3Department of Prosthodontics, Hospital of Stomatology, Jilin University, Changchun, Jilin, China
- 4Laboratory Animal Center, College of Animal Science, Jilin University, Changchun, China
Wound healing of the oral and maxillofacial area affects the quality of life and mental health of the patient; therefore, effective therapies are required to promote wound healing. However, traditional treatment methods have limited efficacy. Exosomes secreted by stem cells used for oral and maxillofacial wound healing have shown outstanding results. Stem cell-derived exosomes possess the regenerative and repair ability of stem cells. Moreover, they are nontumorigenic and have good biosafety. However, the application of natural stem cell exosomes is limited owing to their low yield, impurity, lack of targeting, and low drug delivery rate. Many modification methods have been developed to engineered stem cell exosomes with beneficial properties, such as modifying parent cells and directly processing stem cell exosomes. These methods include coincubation, genetic engineering, electroporation, ultrasound, and artificial synthesis of engineered stem cell exosomes. These engineered stem cell exosomes can cargo nucleic acids, proteins, and small molecules. This gives them anti-inflammatory and cell proliferation regulatory abilities and enables the targeted promotion of efficient soft tissue repair after trauma. Engineered stem cell exosomes can decrease inflammation, promote fibroblast proliferation, and angiogenesis, and decrease scar formation to promote oral and maxillofacial wound healing, including diabetic and burn wounds. Thus, engineered stem cell exosomes are an effective treatment that has the potential for oral and maxillofacial wound healing.
Introduction
Oral and maxillofacial injuries are common oral and maxillofacial conditions (Durham et al., 2017). Severe oral and maxillofacial skin injury caused by trauma or surgery leads to undesirable healing, such as delayed wound closure and scar formation. This results in physical dysfunction and affects maxillofacial appearance (Tanaka et al., 2021). Wound healing is a complex process involving fibroblast and myofibroblast subpopulations, growth factors, cytokines, and extracellular matrix (ECM) components (Chang et al., 2002; Darby and Hewitson, 2007; Driskell et al., 2013; Dalisson and Barralet, 2019; Hinz and Lagares, 2020; Kim et al., 2021). The physiological wound-healing process can be regulated by external factors and internal biological pathways (Gurtner et al., 2008). Oral and maxillofacial skin injuries, including chronic and infectious wounds and large burns, require positive and effective therapy to promote wound healing (Powers et al., 2016; Hall et al., 2017; Wang Y. et al., 2018; Negut et al., 2018). Proteins, drugs, natural compounds, genes, cells, and bioengineered therapies can be used for wound healing (Cho et al., 2019; Veith et al., 2019). Because of the efficient delivery system, exosomes have potential applications in promoting wound healing.
Exosomes are cup-shaped or spherical bilayer phospholipid membrane structures with a diameter of 40–160 nm released from multivesicular bodies (MVBs) by exocytosis after fusion with the cytoplasmic membrane (Sharma et al., 2010; Sokolova et al., 2011; Kahroba et al., 2019; Kalluri and LeBleu, 2020). The exosome structure enables them to carry various bioactive cargos, such as proteins, nucleic acids (DNA and messenger [mRNA], micro [miRNA], and long non-coding [lncRNA] RNAs), lipids, metabolites, and small molecule drugs (Pascucci et al., 2014; Peng H. et al., 2020; Zhou et al., 2020). The cargos depend on donor cell differentiation and environmental stimulation (Pegtel et al., 2014). Exosomes can be secreted by various cells, such as immune, stem, cardiovascular, nerve, and tumor cells and reticulocytes and platelets (Sokolova et al., 2011). Cell-derived exosomes are widely distributed in the peripheral blood, urine, saliva, sweat, milk, ascites, and amniotic fluid (Admyre et al., 2007; Keller et al., 2007; Dai et al., 2008; Gonzales et al., 2009; Lasser et al., 2011; Wang Q. L. et al., 2018; Wu and Liu, 2018). Their biological functions are exerted by releasing their cargo, such as intercellular signal transduction, cell growth, immune response, and tissue repair and regeneration (Isola and Chen, 2017; Gurunathan et al., 2019).
Exosomes derived from stem cells play a vital role in promoting wound healing by regulating biological processes (Vu et al., 2021). Importantly, blood vessels play an integral role in wound healing by providing oxygen and nutrients for tissues and cells (Eble and Niland, 2009). A previous study has shown that human umbilical cord mesenchymal stem cells (HUC-MSCs) exosomes increase angiogenesis to promote burn wound healing (Zhang et al., 2015b). Moreover, exosomes derived from oral tissue stem cells, urine stem cells, HUC-MSCs, bone marrow mesenchymal stem cells (BMSCs), and mesenchymal stromal cells (MSCs) promote diabetic wound healing and skin regeneration by promoting fibroblast proliferation and migration, angiogenesis, and antioxidant stress (Chen et al., 2018; Shi et al., 2020; Yang et al., 2020; An et al., 2021; Pomatto et al., 2021). Scar formation is commonly seen in wound healing, wherein pathological scar formation affects the physiological functions of hair follicles and sweat glands (Takeo et al., 2015; Monavarian et al., 2019). Stem-cell-derived exosomes conduce to decrease scarring. One study showed that HUC-MSC-derived exosomes inhibit myofibroblast formation, which prevents scar formation (Fang et al., 2016). These findings suggested that stem-cell-derived exosomes positively affected wound healing by promoting cell proliferation and angiogenesis and reducing scarring.
While stem-cell-derived exosomes positively affect wound healing, naturally produced exosomes have limitations that can affect their therapeutic effect, which includes low yield, impurity, and lack of targeting (Shao et al., 2018; Thery et al., 2018) (Table 1). However, the engineering of stem-cell-derived exosomes can improve their yield, purity, targeting, drug delivery, and therapeutic efficacy (Kucuk et al., 2021; Liang et al., 2021). In the present review, we focused on engineering stem cell exosomes, their application in wound healing, and their therapeutic mechanisms.
Preparation of engineered stem cell exosomes
Stem cell exosomes can be engineered with specific functions by indirect, direct, and synthetic preparation methods (Figure 1). These methods, including genetic engineering, co-incubation, parent cell surface modification, and artificial synthesis, have different advantages and limitations (Table 2).
Parent cell modification
Exosomes carrying nucleic acids and drugs can be derived by treating exosome-secreting cells (Fitts et al., 2019). In the traditional method, the cells are transfected with recombinant viruses or plasmids to obtain exosomes carrying specific genes. Studies show that parent cells transfected with lentiviral vectors produced exosomes carrying miRNA 31-5p (miR-31-5p), which was used to heal diabetic wounds by RNA interference (RNAi) therapy (Huang et al., 2021b). Moreover, to obtain stem cell exosomes loaded with HOX transcript antisense lncRNA (HOTAIR), MSCs were transfected to overexpress these lncRNAs to promote wound healing (Born et al., 2022). This indicated that the parent cells were modified and produced exosomes loaded with mRNA and proteins, which promoted wound healing. However, this method has various limitations, such as variable transfection efficiency and gene expression. Moreover, hydrophilic or hydrophobic molecules can be loaded on exosomes by co-incubating them with parent cells to improve the therapeutic effect of exosomes. One study showed that exosomes have PD-L1 on their surface after cell stimulation with IFN-γ, which affects the immunosuppressive function of recipient cells (Su et al., 2019). Exosomes carrying nucleic acids can also be derived by processing physical materials. These processes include treating BMSCs with magnetic nanoparticles (NPs) and static magnetic fields to produce exosomes containing overexpressed miRNA 1260a (miR-1260a) (Wu et al., 2021). Exosomes directly produced by parent cells have advantages in targeting and therapeutic effects. However, their disadvantages include low yield and the presence of impurities, and they need to be characterized before use.
Exosome loading
Compared with parent cell modification, exosome modification has more beneficial effects. Exosome-loading methods include co-incubation, electroporation, high- and low-temperature cycling, and ultrasound (Nasiri Kenari et al., 2020). Cargo co-incubation is a common method. By incubating with curcumin, exosomes loaded with small anti-inflammatory molecules improve their therapeutic effect on inflammation (Sun et al., 2010). This method increases drug solubility and utilization but is limited by its low drug loading rate and unsuitability for hydrophobic molecules. Electroporation is another commonly used method for loading exosomes that has a high cargo loading rate. Moreover, electroporation increases the amount of RNA and small hydrophilic molecules loaded in exosomes, which decreases RNA degradation in the wound microenvironment (Fuhrmann et al., 2015). The therapeutic effect of functional RNA is improved by loading miRNA 21-5p (miR-21-5p) into adipose stem cell exosomes via electroporation, resulting in engineered exosomes with good promotive effects on diabetic wound healing (Lv Q. et al., 2020). Electroporation can produce exosomes with gene delivery and load large molecular compounds. However, it is limited to hydrophilic compounds and length-dependent gene delivery (Lamichhane et al., 2015). Considering that electroporation can lead to the aggregation of exosomes, exogenous cargos can be loaded into exosomes via ultrasound (Johnsen et al., 2016). Exosomes derived from HUC-MSCs were treated with ultrasound to carry silver NPs (AgNPs), which improved their antibacterial activity (Qian et al., 2020). Treatment with ultrasound maintains exosome stability and promotes cargo loading better than co-incubation (Haney et al., 2015). It is a beneficial method because of its improved gene and drug delivery. However, its disadvantages include low rates of cargo loading.
Exosome surface modification
Exosome surface modification can improve their targeting abilities, which can be mainly achieved by genetic engineering and chemical modification (Salunkhe et al., 2020). Genetic engineering is effective for displaying genetically engineered proteins on the exosome surface, which requires exosome identification (Wan et al., 2017; Mishra et al., 2021). A previous study showed that targeted peptides can be loaded onto the exosome surface by genetic engineering, which allows tissue specificity and improves efficacy (Curley et al., 2020). Coupling cardiac stem cell exosomes with a targeted heart-homing peptide improves the targetability and uptake of exosomes in myocardial infarcted hearts (Vandergriff et al., 2018). Chemical modifications can load various molecules onto the exosome surfaces via non-covalent or covalent interactions. This has the advantages of fast reaction, high specificity, and water buffer compatibility (Smyth et al., 2014; Armstrong et al., 2017). Exosome surface modification can improve exosome targeting. However, it is limited by the strict separation methods that are required to obtain engineered exosomes with high purity.
Artificial synthesis of exosomes
Artificial synthesis includes both top-down and bottom-up methods. In top-down methods, parent cells are treated to obtain vesicle-forming membrane segments, which are reassembled into exosomes. One study showed that passing ESCs through tiny pores created the derived exosomes promoting fibroblast proliferation, which contributed to tissue recovery and wound healing (Jeong et al., 2014). However, exosomes derived from cell-disrupted phospholipid bilayer membranes have donor cell signaling compounds and lipid-induced toxicity (Lv et al., 2006). The yield of artificial synthesis exosomes is higher than naturally occurring exosomes. However, their immunogenicity is lower, and they lack cargo loading specificity. Bottom-up methods can be used to prepare bilayer structures and simulate exosome components by physical or chemical methods. The lipid bilayer can then be combined with surface proteins to simulate exosome production (Vazquez-Rios et al., 2019). A previous study showed that biologically active anti-inflammatory proteins, namely APO2L and TRAIL, can be combined with liposomes to obtain artificial exosomes (Martinez-Lostao et al., 2010). Artificially synthesized stem cell exosomes have categorical characterization and composition and a controllable production process.
Compared with naturally produced exosomes, engineered exosomes have improved targeting, high drug loading rate, high purity, and large yield. Engineered exosomes with different loading cargoes can be used to promote wound healing via anti-inflammatory, growth-promoting, angiogenesis-promoting, and collagen synthesis-regulating activities.
Engineered stem cell exosomes promote oral and maxillofacial wound healing
Engineered stem cell exosomes have good efficacy in promoting oral and maxillofacial wound healing by regulating inflammation, promoting fibroblast proliferation, improving angiogenesis, and decreasing scar formation.
Engineered stem cell exosomes decrease the levels of inflammatory factor
Long-term inflammation seriously affects the wound-healing process, and sustained local inflammation can lead to abnormal wound healing and pathological scar formation (Wang et al., 2020). Engineered stem cell exosomes can decrease the inflammatory response and promote wound healing by inhibiting pro-inflammatory factor secretion (Figure 2A). One study showed that exosomes produced by treating MSCs with melatonin (MT) or deferoxamine targeted the phosphatase and tensin homolog (PTEN)/AKT signaling pathway to promote diabetic wound healing by shortening inflammatory period (Ding et al., 2019; Liu et al., 2020). Another study has shown that exosomes from MSCs stimulated with inflammatory factors such as tumor necrosis factor (TNF-α) and interferon (IFN)-γ can decrease the release of pro-inflammatory cytokines and have improved anti-inflammatory abilities (Harting et al., 2018). A previous study showed that exosomes from MSCs that are pretreated with lipopolysaccharide can regulate the TLR4/NF-κB/STAT3/AKT signaling pathway via miRNA let-7b to promote diabetic skin wound healing by regulating chronic inflammation regression (Ti et al., 2015). The overexpressed RNA and transcription factors of engineered stem-cell-derived exosomes play a role in promoting wound healing (Hu et al., 2019). Of note, when the transcription factor nuclear factor E2-related factor 2 (Nrf2) was overexpressed in adipose stem-cell-derived exosomes, it inhibited the production of reactive oxygen species and inflammatory cytokines to promote diabetic wound healing (Li et al., 2018). Exosomes loaded with overexpressed miRNA 181c (miR-181c) derived from HUC-MSCs decreased TNF-α and interleukin (IL)-1β and increased IL-10 levels via the TLR4 signaling pathway to promote burn wound healing (Li et al., 2016). Overall, the cargo of engineered stem cell exosomes can decrease the release of inflammatory factors and the duration of the inflammatory response to facilitate wound healing.
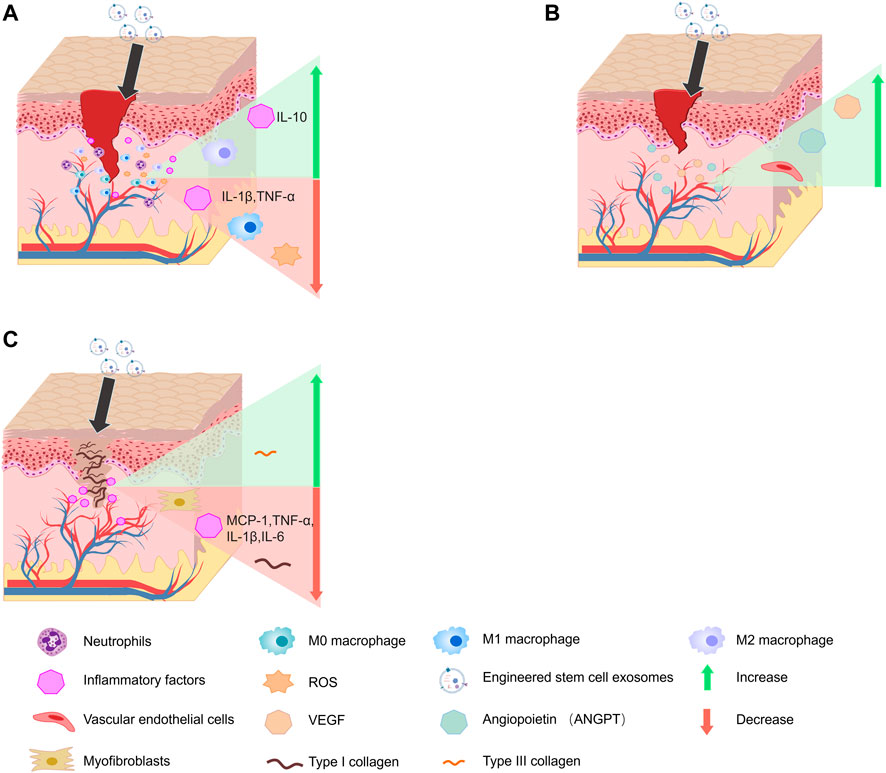
FIGURE 2. Effect of engineered stem cell exosomes on wound healing. (A) Engineered stem cell exosomes decrease the levels of inflammatory factor. (B) Engineered stem cell exosomes promote angiogenesis. (C) Engineered exosomes decrease scar formation.
Engineered stem cell exosomes promote fibroblast proliferation
Fibroblasts are important effector cells in skin wounds, whose function can be increased to promote wound healing by using engineered stem cell exosomes (Bhattacharyya et al., 2013; Lian and Li, 2016). A previous study showed that hypoxic adipose stem cell exosomes promoted fibroblast proliferation and migration and accelerated high-quality diabetic wound healing by activating the PI3K/AKT pathway to regulate the expression of various growth factors (Wang et al., 2021b). Moreover, engineered stem cell exosomes carrying H19 affected the H19/miR-152-3p/PTEN axis, regulating the PI3K/AKT signaling pathway to increase fibroblast proliferation and apoptosis inhibit, accelerating the diabetic wound healing process (Li B. et al., 2020). In addition, Wnt4 delivered by MSC-derived exosomes promoted β-catenin nuclear translocation and activity to increase skin cell proliferation. This played a vital role in wound re-epithelialization (Zhang et al., 2015a). Interestingly, synthetically engineered exosomes, which are effectively taken up by recipient cells, can be used for promoting wound healing. One study showed that nanovesicles obtained from extruded embryonic stem cells (ESCs) activated the mitogen-activated protein kinase (MAPK) signaling pathway to promote fibroblast proliferation and wound healing (Jeong et al., 2014). Thus, engineered stem cell exosomes can help in promoting fibroblast proliferation to accelerate wound healing through their cargos.
Engineered stem cell exosomes promote angiogenesis
Angiogenesis is an intrinsic repair pathway for wound healing and tissue regeneration. Insufficient angiogenesis, which involves genes and proteins, such as vascular endothelial growth factor (VEGF), delays the wound-healing process (Zhao et al., 2017). Therefore, the VEGF receptor (VEGFR) can initiate angiogenesis and promote wound healing (Johnson and Wilgus, 2014). Gene-modified parental cells have been widely used to obtain exosomes that can promote angiogenesis. The proangiogenic ability of vascular endothelial cells can be increased by miRNAs and cytokines uptake by engineered stem cell exosomes (Figure 2B). On study showed that MSC exosomes with high levels of miRNA 126-3p (miR-126-3p) can promote human dermal microvascular endothelial cell proliferation, migration, and angiogenesis (Tao et al., 2017). MSC exosomes carrying miR-21-5p promote angiogenesis by upregulating the VEGF, AKT, and MAPK pathways, which positively affects diabetic wound healing (Huang C. et al., 2021). Moreover, the vascularization ability of exosomes can also be increased by physical processing. One study showed that blue light treatment upregulated miR-135b-5p and miR-499a-3p levels in HUC-MSC-derived exosomes, which promoted human umbilical vein endothelial cell (HUVECs) proliferation, migration, and angiogenesis (Yang et al., 2019). Moreover, exosomes secreted by stem cells that are treated with various biological compounds also have significant angiogenesis-promoting abilities (Liu et al., 2019). A previous study showed that exosomes from BMSCs stimulated with iron oxide (Fe3O4) NPs and static magnetic field promoted angiogenesis by upregulating miR-21-5p targeting SPRY2 and activating PI3K/AKT and ERK1/2 signaling pathways in wound healing (Wu et al., 2020). Exosomes of BMSCs pretreated with deferoxamine activated the PI3K/AKT signaling pathway via miR-126-mediated PTEN downregulation to stimulate angiogenesis in vitro (Ding et al., 2019). Moreover, exosomes treated with superparamagnetic ferric oxide NPs have precise targeting, which accumulates in the damaged area and significantly increases angiogenesis (Li X. et al., 2020). Moreover, chemically-treated stem cell exosomes also promote angiogenesis. Stem cell exosomes pretreated with atorvastatin (ATV) or pioglitazone significantly promoted the angiogenesis of endothelial cells by mediating the PTEN/AKT/eNOS pathway in diabetic wound healing (Yu et al., 2020; Hu et al., 2021). Artificially synthesized exosomes with specific protein composition and RNA load significantly promoted angiogenesis (Tao et al., 2018; Kim et al., 2021). These findings suggested that engineered stem cell exosomes have potential therapeutic effects in promoting wound healing by modulating the proangiogenic ability of endothelial cells.
Engineered exosomes decrease scar formation
Chronic inflammation and myofibroblast aggregation can cause the thickening of pathological scarring in the wound area (Ogawa, 2017; Rippa et al., 2019). The application of engineered stem cell exosomes can decrease scar formation (Figure 2C). A studies has shown that miRNAs 21 (miR-21), 23A (miR-23A), 125b (miR-125b), and 145 (miR-145) in MSC exosomes can inhibit fibroblast and myofibroblast differentiation by targeting TGF-β/Smad2 signaling pathway to decrease scarring (Fang et al., 2016). Moreover, human adipose-derived MSC exosomes overexpressing miRNA 29a (miR-29a) inhibit scar hyperplasia after burn injury by targeting TGF-β2/Smad3 signaling pathway (Yuan et al., 2021). Notably, engineered stem cell exosomes can inhibit scar formation by decreasing inflammatory factor expression. Previous studies have shown that human amniotic fluid stem cell exosomes can decrease scar formation by decreasing the secretion of inflammation-related cytokines via miRNA 146a-5p (miR-146a-5p) (Wgealla et al., 2022). Exosomes derived from MSCs overexpressing tumor necrosis factor (TNF)-stimulated gene-6 (TSG-6) can decrease MCP-1, TNF-α, IL-1β, and IL-6 levels in scar tissue and inhibit the inflammatory response in pathological scars, significantly reducing scar formation (Jiang et al., 2020). Therefore, engineered stem cell exosomes are an effective approach for enhancing their biological activity and improving repair efficacy in reducing scar formation.
The application of engineered exosomes in oral and maxillofacial wound
Oral and maxillofacial injury can easily damage the hard and soft tissues (Lv L. et al., 2020). Because of the special location of oral and maxillofacial, the healing process has an important effect on the physiological function and mental health of patients. The application of engineered exosomes can positively promote the accurate and efficient healing of oral and maxillofacial wounds. Engineered exosomes can increase accumulation at the wound site by precise delivery, thereby effectively promoting wound healing (Li X. et al., 2020). Furthermore, the engineered exosomes can achieve a therapeutic effect on oral and maxillofacial wound healing through their cargos, including diabetes and burn wounds, which are difficult to heal (Aryan et al., 2018; Peng Q. et al., 2020; Liu et al., 2021; Hade et al., 2022; Hsu et al., 2022). Moreover, because oral and maxillofacial nerve repair is directly associated with expression, the application of engineered exosomes can contribute to nerve repair and regeneration and promote the early recovery of expression functions (Yang et al., 2021; Wang Y. et al., 2022). Compared with traditional treatment and cell therapy, engineered stem cell exosomes have better effects on oral and maxillofacial wound healing and have good application prospects (Maqsood et al., 2020; Md Fadilah et al., 2022). Presently, many more efficient and safer cell-free scaffold dressings carrying exosomes have been developed for wound healing (Las Heras et al., 2020). The combined application of exosomes and biomaterials has a positive effect on promoting wound healing, which can maintain the stability of exosomes in vivo with good biocompatibility (Golchin et al., 2022).
The application of exosomes in clinical trials
Stem cell-derived exosomes avoid the risk of cell therapy and have a good application prospect in regenerative medicine (Phinney and Pittenger, 2017). The clinical application of exosomes in wound healing is gradually increasing (Supplementary Table S1) (Li et al., 2022). One study evaluated the effectiveness of exosomes extracted from adipose tissue of patients for wound healing (NCT05475418). In an early Phase 1 clinical trial, exosomes extracted from the plasma of patients were applied to the ulcer site to clear the effectiveness of exosomes in promoting skin wound healing (NCT02565264). In addition, a clinical trial started in March 2022 has completed the safety and tolerability study of the topical application of MSC exosome ointment in Psoriasis (NCT05523011). Moreover, a clinical study on atrophic acne scar treatments using adipose tissue-derived stromal cells (ADSCs) exosomes showed promising therapeutic effects (Kwon et al., 2020).
With the advantages of good biocompatibility and low immunogenicity, exosomes provide new tools for the development of therapeutic drugs for human wound healing (Hade et al., 2021). Moreover, exosomes can protect the cargos they carry by a biomembrane from enzymes and other substances that can damage proteins, and exosome-packaged proteins are more stable and efficient (Lu et al., 2019). Therefore, oral and maxillofacial trauma can be treated by synthesizing engineered exosomes with specific functions. At present, with the in-depth study of engineered exosome preparation technology and treatment mechanism, some exosome-based drugs have been developed, and the clinical application of exosomes still has a broad space to explore.
Discussion
Presently, the application of hydrogel-loaded and ECM-loaded stem cells has positively helped in the process of wound healing (Lee et al., 2007; Rustad et al., 2012; Ariyanti et al., 2019). Stem cells play a crucial role in regenerating damaged organs via their paracrine effects (Ratajczak et al., 2012). Because the knowledge of intercellular functions has increased, exosomes can be developed as a precise and targeted therapeutic strategy (van Niel et al., 2018). Compared with normal cells, stem-cell-derived exosomes can escape phagocytosis and have the advantages of greater biocompatibility, increased retention, and low immunogenicity (Saunderson et al., 2014; Kamerkar et al., 2017; Wortzel et al., 2019; Hassanzadeh et al., 2021). Importantly, stem-cell-derived exosomes have the advantages of easier storage and application, penetration of the blood–brain barrier, and a long circulation half-life (Turturici et al., 2014; Chen et al., 2016; Gowen et al., 2020; Huang et al., 2021c). The function of exosomes is related to the donor cells and environmental stimuli (Stavrou and Ortiz, 2022). Stem cells and exosomes in adipose tissue have a positive impact on promoting angiogenesis, which has great application potential in regenerative medicine (Kamat et al., 2020). Compared with stem cells derived from other tissues, mesenchymal stem cells in adipose tissue are easily accessed and have a high density (Buschmann et al., 2013). Moreover, a study shows that microfragmented adipose tissue (MFAT) has long-lasting anti-inflammatory activity (Nava et al., 2019). Clinical trials proved that MFAT used to treat ulcer caused by prosthesis effectively promote rapid healing of skin ulcers (Copeland and Martin, 2021). Therefore, stem-cell-derived exosomes have beneficial advantages in wound healing and can be potentially used in wound healing therapies.
Exosomes can be used as a delivery system for drugs, proteins, mRNA, miRNA, lncRNA, and small molecules to recipient cells, which can regulate macrophages, fibroblasts, vascular endothelial cells, and myofibroblast functions in wounds to promote healing (Bunggulawa et al., 2018). They promote the uptake by recipient cells and protect RNA from extracellular degradation, thereby enabling them to be an ideal treatment tool for oral and maxillofacial wound healing (Kooijmans et al., 2012; Zhang et al., 2013; Zhang J. et al., 2015).
However, the application of naturally produced exosomes is limited (Villarroya-Beltri et al., 2014; Taylor and Shah, 2015; Bjorge et al., 2017; Bian et al., 2022). Nevertheless, engineered stem cell exosomes loaded with functional cargos can have remarkable therapeutic effects (Marcus and Leonard, 2013). The exosomes are loaded with overexpressed nucleic acids by various methods, such as transfection, co-incubation, ultrasound, and electroporation, which provide them with targeting, anti-inflammatory, cell proliferation, and apoptotic functions (Weaver, 1993; Jo et al., 2014; Smyth et al., 2014; Fang et al., 2016; Garcia-Manrique et al., 2018; Ding et al., 2019; Li B. et al., 2020; Li X. et al., 2020). Importantly, engineered exosomes can be designed for different wound types, such as diabetes and burns, with functions in attenuating anti-inflammatory processes, promoting angiogenesis, and decreasing scar formation (Lv L. et al., 2020) (Figure 3). However, it is necessary to solve the needs of large-scale production and high purity for clinical application. Hollow fiber bioreactors are ideal for large-scale production (Kimiz-Gebologlu and Oncel, 2022). In recent years, with the advancement of technology, microfluidics, electrical, centrifugal and acoustical forces have been introduced into the separation of exosomes, which has led to the rapid development of some new exosome separation technologies. They include polymer-based precipitation, ultrafiltration, bioreactor systems, production of biomimetic vesicles, membrane-based separation methods, and microfluidic methods (Supplementary Table S2) (Wang et al., 2021a; Zhang et al., 2021). Among them, microfluidic devices that can be used for exosome isolation and purification become promising devices for exosome therapy (Chen et al., 2021). The bottom-up production of synthetic exosomes in engineered exosomes can be synthesized by a method similar to liposome microfluidic to realize large-scale production of exosomes (Wang X. et al., 2022), which will promote the application of exosomes in oral and maxillofacial wound healing.
The oral and maxillofacial region contains various important blood vessels and nerves that are vulnerable to trauma and prone to bleeding and infection after any injury (Kageyama et al., 2021). The engineered stem cell exosomes can act as biological carriers delivering various bioactive substances to act on target cells. They can participate in the regulation of wound repair via signal transduction, which can be effective in oral and maxillofacial wound healing (Maqsood et al., 2020).
Conclusion
Engineered stem cell exosomes have high yields, low immunogenicity, and specific functional cargos. Moreover, engineered stem cell exosomes can decrease inflammation and scar formation and promote angiogenesis and fibroblast proliferation. These properties of engineered stem cell exosomes can be leveraged in the future for oral and maxillofacial wound healing.
Author contributions
MH and MD wrote the manuscript. MH, MD, ZY, HZ, SL, JX, HW, HL, LC, DW, and WL searched PubMed and Web of Science for citations and prepared figures. All authors reviewed the manuscript.
Funding
This work was supported by the Fundamental Research Funds for the Central Universities (Grant Nos. 2019JCKT-70 and 2020JCXK-45), the Science and Technology Project of Jilin Provincial Department of Education (No. JJKH20221093KJ), the Jilin Province Department of Finance (Grant No. jcsz2021893-13), the Jilin Scientific and Technological Development Program (Grant Nos. 20210101010JC, 20220505033ZP and 20200801077GH), and the Changchun Scientific and Technological Development Program (Grant No. 21ZY26).
Conflict of interest
The authors declare that the research was conducted in the absence of any commercial or financial relationships that could be construed as a potential conflict of interest.
Publisher’s note
All claims expressed in this article are solely those of the authors and do not necessarily represent those of their affiliated organizations, or those of the publisher, the editors and the reviewers. Any product that may be evaluated in this article, or claim that may be made by its manufacturer, is not guaranteed or endorsed by the publisher.
Supplementary material
The Supplementary Material for this article can be found online at: https://www.frontiersin.org/articles/10.3389/fbioe.2022.1038261/full#supplementary-material
References
Admyre, C., Johansson, S. M., Qazi, K. R., Filen, J. J., Lahesmaa, R., Norman, M., et al. (2007). Exosomes with immune modulatory features are present in human breast milk. J. Immunol. 179 (3), 1969–1978. doi:10.4049/jimmunol.179.3.1969
An, T., Chen, Y., Tu, Y., and Lin, P. (2021). Mesenchymal stromal cell-derived extracellular vesicles in the treatment of diabetic foot ulcers: Application and challenges. Stem Cell. Rev. Rep. 17 (2), 369–378. doi:10.1007/s12015-020-10014-9
Ariyanti, A. D., Zhang, J., Marcelina, O., Nugrahaningrum, D. A., Wang, G., Kasim, V., et al. (2019). Salidroside-pretreated mesenchymal stem cells enhance diabetic wound healing by promoting paracrine function and survival of mesenchymal stem cells under hyperglycemia. Stem Cells Transl. Med. 8 (4), 404–414. doi:10.1002/sctm.18-0143
Armstrong, J. P., Holme, M. N., and Stevens, M. M. (2017). Re-engineering extracellular vesicles as smart nanoscale therapeutics. ACS Nano 11 (1), 69–83. doi:10.1021/acsnano.6b07607
Aryan, A., Bayat, M., Bonakdar, S., Taheri, S., Haghparast, N., Bagheri, M., et al. (2018). Human bone marrow mesenchymal stem cell conditioned medium promotes wound healing in deep second-degree burns in male rats. Cells Tissues Organs 206 (6), 317–329. doi:10.1159/000501651
Bhattacharyya, S., Fang, F., Tourtellotte, W., and Varga, J. (2013). Egr-1: New conductor for the tissue repair orchestra directs harmony (regeneration) or cacophony (fibrosis). J. Pathol. 229 (2), 286–297. doi:10.1002/path.4131
Bian, D., Wu, Y., Song, G., Azizi, R., and Zamani, A. (2022). The application of mesenchymal stromal cells (MSCs) and their derivative exosome in skin wound healing: A comprehensive review. Stem Cell. Res. Ther. 13 (1), 24. doi:10.1186/s13287-021-02697-9
Bjorge, I. M., Kim, S. Y., Mano, J. F., Kalionis, B., and Chrzanowski, W. (2017). Extracellular vesicles, exosomes and shedding vesicles in regenerative medicine - a new paradigm for tissue repair. Biomater. Sci. 6 (1), 60–78. doi:10.1039/c7bm00479f
Boing, A. N., van der Pol, E., Grootemaat, A. E., Coumans, F. A., Sturk, A., and Nieuwland, R. (2014). Single-step isolation of extracellular vesicles by size-exclusion chromatography. J. Extracell. Vesicles 3, 23430. doi:10.3402/jev.v3.23430
Born, L. J., Chang, K. H., Shoureshi, P., Lay, F., Bengali, S., Hsu, A. T. W., et al. (2022). HOTAIR-loaded mesenchymal stem/stromal cell extracellular vesicles enhance angiogenesis and wound healing. Adv. Healthc. Mat. 11 (5), e2002070. doi:10.1002/adhm.202002070
Bunggulawa, E. J., Wang, W., Yin, T., Wang, N., Durkan, C., Wang, Y., et al. (2018). Recent advancements in the use of exosomes as drug delivery systems. J. Nanobiotechnology 16 (1), 81. doi:10.1186/s12951-018-0403-9
Buschmann, J., Gao, S., Harter, L., Hemmi, S., Welti, M., Werner, C. M., et al. (2013). Yield and proliferation rate of adipose-derived stromal cells as a function of age, body mass index and harvest site-increasing the yield by use of adherent and supernatant fractions? Cytotherapy 15 (9), 1098–1105. doi:10.1016/j.jcyt.2013.04.009
Chang, H. Y., Chi, J. T., Dudoit, S., Bondre, C., van de Rijn, M., Botstein, D., et al. (2002). Diversity, topographic differentiation, and positional memory in human fibroblasts. Proc. Natl. Acad. Sci. U. S. A. 99 (20), 12877–12882. doi:10.1073/pnas.162488599
Chen, B. Y., Sung, C. W., Chen, C., Cheng, C. M., Lin, D. P., Huang, C. T., et al. (2019). Advances in exosomes technology. Clin. Chim. Acta 493, 14–19. doi:10.1016/j.cca.2019.02.021
Chen, C. C., Liu, L., Ma, F., Wong, C. W., Guo, X. E., Chacko, J. V., et al. (2016). Elucidation of exosome migration across the blood-brain barrier model in vitro. Cell. Mol. Bioeng. 9 (4), 509–529. doi:10.1007/s12195-016-0458-3
Chen, C. Y., Rao, S. S., Ren, L., Hu, X. K., Tan, Y. J., Hu, Y., et al. (2018). Exosomal DMBT1 from human urine-derived stem cells facilitates diabetic wound repair by promoting angiogenesis. Theranostics 8 (6), 1607–1623. doi:10.7150/thno.22958
Chen, J., Li, P., Zhang, T., Xu, Z., Huang, X., Wang, R., et al. (2021). Review on strategies and technologies for exosome isolation and purification. Front. Bioeng. Biotechnol. 9, 811971. doi:10.3389/fbioe.2021.811971
Cho, H., Blatchley, M. R., Duh, E. J., and Gerecht, S. (2019). Acellular and cellular approaches to improve diabetic wound healing. Adv. Drug Deliv. Rev. 146, 267–288. doi:10.1016/j.addr.2018.07.019
Copeland, R., and Martin, J. (2021). Chronic prosthesis-related residual limb ulcer treated with autologous micro-fragmented adipose tissue. Regen. Ther. 18, 21–23. doi:10.1016/j.reth.2021.02.005
Curley, N., Levy, D., Do, M. A., Brown, A., Stickney, Z., Marriott, G., et al. (2020). Sequential deletion of CD63 identifies topologically distinct scaffolds for surface engineering of exosomes in living human cells. Nanoscale 12 (22), 12014–12026. doi:10.1039/d0nr00362j
Dai, S., Wei, D., Wu, Z., Zhou, X., Wei, X., Huang, H., et al. (2008). Phase I clinical trial of autologous ascites-derived exosomes combined with GM-CSF for colorectal cancer. Mol. Ther. 16 (4), 782–790. doi:10.1038/mt.2008.1
Dalisson, B., and Barralet, J. (2019). Bioinorganics and wound healing. Adv. Healthc. Mat. 8 (18), e1900764. doi:10.1002/adhm.201900764
Darby, I. A., and Hewitson, T. D. (2007). Fibroblast differentiation in wound healing and fibrosis. Int. Rev. Cytol. 257, 143–179. doi:10.1016/S0074-7696(07)57004-X
Ding, J., Wang, X., Chen, B., Zhang, J., and Xu, J. (2019). Exosomes derived from human bone marrow mesenchymal stem cells stimulated by deferoxamine accelerate cutaneous wound healing by promoting angiogenesis. Biomed. Res. Int. 2019, 1–12. doi:10.1155/2019/9742765
Driskell, R. R., Lichtenberger, B. M., Hoste, E., Kretzschmar, K., Simons, B. D., Charalambous, M., et al. (2013). Distinct fibroblast lineages determine dermal architecture in skin development and repair. Nature 504 (7479), 277–281. doi:10.1038/nature12783
Durham, J., Moore, U. J., Hill, C. M., and Renton, T. (2017). Oral surgery II: Part 6. Oral and maxillofacial trauma. Br. Dent. J. 223 (12), 877–883. doi:10.1038/sj.bdj.2017.995
Eble, J. A., and Niland, S. (2009). The extracellular matrix of blood vessels. Curr. Pharm. Des. 15 (12), 1385–1400. doi:10.2174/138161209787846757
Fang, S., Xu, C., Zhang, Y., Xue, C., Yang, C., Bi, H., et al. (2016). Umbilical cord-derived mesenchymal stem cell-derived exosomal MicroRNAs suppress myofibroblast differentiation by inhibiting the transforming growth factor-β/SMAD2 pathway during wound healing. Stem Cells Transl. Med. 5 (10), 1425–1439. doi:10.5966/sctm.2015-0367
Fitts, C. A., Ji, N., Li, Y., and Tan, C. (2019). Exploiting exosomes in cancer liquid biopsies and drug delivery. Adv. Healthc. Mat. 8 (6), e1801268. doi:10.1002/adhm.201801268
Fuhrmann, G., Serio, A., Mazo, M., Nair, R., and Stevens, M. M. (2015). Active loading into extracellular vesicles significantly improves the cellular uptake and photodynamic effect of porphyrins. J. Control. Release 205, 35–44. doi:10.1016/j.jconrel.2014.11.029
Garcia-Manrique, P., Matos, M., Gutierrez, G., Pazos, C., and Blanco-Lopez, M. C. (2018). Therapeutic biomaterials based on extracellular vesicles: Classification of bio-engineering and mimetic preparation routes. J. Extracell. Vesicles 7 (1), 1422676. doi:10.1080/20013078.2017.1422676
Golchin, A., Shams, F., Basiri, A., Ranjbarvan, P., Kiani, S., Sarkhosh-Inanlou, R., et al. (2022). Combination therapy of stem cell-derived exosomes and biomaterials in the wound healing. Stem Cell. Rev. Rep. 18 (6), 1892–1911. doi:10.1007/s12015-021-10309-5
Gonzales, P. A., Pisitkun, T., Hoffert, J. D., Tchapyjnikov, D., Star, R. A., Kleta, R., et al. (2009). Large-scale proteomics and phosphoproteomics of urinary exosomes. J. Am. Soc. Nephrol. 20 (2), 363–379. doi:10.1681/ASN.2008040406
Gowen, A., Shahjin, F., Chand, S., Odegaard, K. E., and Yelamanchili, S. V. (2020). Mesenchymal stem cell-derived extracellular vesicles: Challenges in clinical applications. Front. Cell. Dev. Biol. 8, 149. doi:10.3389/fcell.2020.00149
Gurtner, G. C., Werner, S., Barrandon, Y., and Longaker, M. T. (2008). Wound repair and regeneration. Nature 453 (7193), 314–321. doi:10.1038/nature07039
Gurunathan, S., Kang, M. H., Jeyaraj, M., Qasim, M., and Kim, J. H. (2019). Review of the isolation, characterization, biological function, and multifarious therapeutic approaches of exosomes. Cells 8 (4), 307. doi:10.3390/cells8040307
Hade, M. D., Suire, C. N., Mossell, J., and Suo, Z. (2022). Extracellular vesicles: Emerging frontiers in wound healing. Med. Res. Rev. 42, 2102–2125. doi:10.1002/med.21918
Hade, M. D., Suire, C. N., and Suo, Z. (2021). Mesenchymal stem cell-derived exosomes: Applications in regenerative medicine. Cells 10 (8), 1959. doi:10.3390/cells10081959
Hall, C., Hardin, C., Corkins, C. J., Jiwani, A. Z., Fletcher, J., Carlsson, A., et al. (2017). Pathophysiologic mechanisms and current treatments for cutaneous sequelae of burn wounds. Compr. Physiol. 8 (1), 371–405. doi:10.1002/cphy.c170016
Haney, M. J., Klyachko, N. L., Zhao, Y., Gupta, R., Plotnikova, E. G., He, Z., et al. (2015). Exosomes as drug delivery vehicles for Parkinson's disease therapy. J. Control. Release 207, 18–30. doi:10.1016/j.jconrel.2015.03.033
Harting, M. T., Srivastava, A. K., Zhaorigetu, S., Bair, H., Prabhakara, K. S., Toledano Furman, N. E., et al. (2018). Inflammation-stimulated mesenchymal stromal cell-derived extracellular vesicles attenuate inflammation. Stem Cells 36 (1), 79–90. doi:10.1002/stem.2730
Hassanzadeh, A., Rahman, H. S., Markov, A., Endjun, J. J., Zekiy, A. O., Chartrand, M. S., et al. (2021). Mesenchymal stem/stromal cell-derived exosomes in regenerative medicine and cancer; overview of development, challenges, and opportunities. Stem Cell. Res. Ther. 12 (1), 297. doi:10.1186/s13287-021-02378-7
Hinz, B., and Lagares, D. (2020). Evasion of apoptosis by myofibroblasts: A hallmark of fibrotic diseases. Nat. Rev. Rheumatol. 16 (1), 11–31. doi:10.1038/s41584-019-0324-5
Hsu, H. H., Wang, A. Y. L., Loh, C. Y. Y., Pai, A. A., and Kao, H. K. (2022). Therapeutic potential of exosomes derived from diabetic adipose stem cells in cutaneous wound healing of db/db mice. Pharmaceutics 14 (6), 1206. doi:10.3390/pharmaceutics14061206
Hu, P., Yang, Q., Wang, Q., Shi, C., Wang, D., Armato, U., et al. (2019). Mesenchymal stromal cells-exosomes: A promising cell-free therapeutic tool for wound healing and cutaneous regeneration. Burns Trauma 7, 38. doi:10.1186/s41038-019-0178-8
Hu, Y., Tao, R., Chen, L., Xiong, Y., Xue, H., Hu, L., et al. (2021). Exosomes derived from pioglitazone-pretreated MSCs accelerate diabetic wound healing through enhancing angiogenesis. J. Nanobiotechnology 19 (1), 150. doi:10.1186/s12951-021-00894-5
Huang, C., Luo, W., Wang, Q., Ye, Y., Fan, J., Lin, L., et al. (2021a). Human mesenchymal stem cells promote ischemic repairment and angiogenesis of diabetic foot through exosome miRNA-21-5p. Stem Cell. Res. 52, 102235. doi:10.1016/j.scr.2021.102235
Huang, J., Yu, M., Yin, W., Liang, B., Li, A., Li, J., et al. (2021b). Development of a novel RNAi therapy: Engineered miR-31 exosomes promoted the healing of diabetic wounds. Bioact. Mat. 6 (9), 2841–2853. doi:10.1016/j.bioactmat.2021.02.007
Huang, J., Zhang, J., Xiong, J., Sun, S., Xia, J., Yang, L., et al. (2021c). Stem cell-derived nanovesicles: A novel cell-free therapy for wound healing. Stem Cells Int. 2021, 1–14. doi:10.1155/2021/1285087
Isola, A. L., and Chen, S. (2017). Exosomes: The messengers of health and disease. Curr. Neuropharmacol. 15 (1), 157–165. doi:10.2174/1570159x14666160825160421
Jeong, D., Jo, W., Yoon, J., Kim, J., Gianchandani, S., Gho, Y. S., et al. (2014). Nanovesicles engineered from ES cells for enhanced cell proliferation. Biomaterials 35 (34), 9302–9310. doi:10.1016/j.biomaterials.2014.07.047
Jiang, L., Zhang, Y., Liu, T., Wang, X., Wang, H., Song, H., et al. (2020). Exosomes derived from TSG-6 modified mesenchymal stromal cells attenuate scar formation during wound healing. Biochimie 177, 40–49. doi:10.1016/j.biochi.2020.08.003
Jo, W., Kim, J., Yoon, J., Jeong, D., Cho, S., Jeong, H., et al. (2014). Large-scale generation of cell-derived nanovesicles. Nanoscale 6 (20), 12056–12064. doi:10.1039/c4nr02391a
Johnsen, K. B., Gudbergsson, J. M., Skov, M. N., Christiansen, G., Gurevich, L., Moos, T., et al. (2016). Evaluation of electroporation-induced adverse effects on adipose-derived stem cell exosomes. Cytotechnology 68 (5), 2125–2138. doi:10.1007/s10616-016-9952-7
Johnson, K. E., and Wilgus, T. A. (2014). Vascular endothelial growth factor and angiogenesis in the regulation of cutaneous wound repair. Adv. Wound Care (New. Rochelle. 3 (10), 647–661. doi:10.1089/wound.2013.0517
Kageyama, I., Maeda, S., and Takezawa, K. (2021). Importance of anatomy in dental implant surgery. J. Oral Biosci. 63 (2), 142–152. doi:10.1016/j.job.2021.01.002
Kahroba, H., Hejazi, M. S., and Samadi, N. (2019). Exosomes: From carcinogenesis and metastasis to diagnosis and treatment of gastric cancer. Cell. Mol. Life Sci. 76 (9), 1747–1758. doi:10.1007/s00018-019-03035-2
Kalluri, R., and LeBleu, V. S. (2020). The biology, function, and biomedical applications of exosomes. Science 367 (6478), eaau6977. doi:10.1126/science.aau6977
Kamat, P., Frueh, F. S., McLuckie, M., Sanchez-Macedo, N., Wolint, P., Lindenblatt, N., et al. (2020). Adipose tissue and the vascularization of biomaterials: Stem cells, microvascular fragments and nanofat-a review. Cytotherapy 22 (8), 400–411. doi:10.1016/j.jcyt.2020.03.433
Kamerkar, S., LeBleu, V. S., Sugimoto, H., Yang, S., Ruivo, C. F., Melo, S. A., et al. (2017). Exosomes facilitate therapeutic targeting of oncogenic KRAS in pancreatic cancer. Nature 546 (7659), 498–503. doi:10.1038/nature22341
Keller, S., Rupp, C., Stoeck, A., Runz, S., Fogel, M., Lugert, S., et al. (2007). CD24 is a marker of exosomes secreted into urine and amniotic fluid. Kidney Int. 72 (9), 1095–1102. doi:10.1038/sj.ki.5002486
Kim, S., Kim, Y., Hyun, Y. S., Choi, H., Kim, S. Y., and Kim, T. G. (2021). Exosomes from human cord blood plasma accelerate cutaneous wound healing by promoting fibroblast function, angiogenesis, and M2 macrophage differentiation. Biomater. Sci. 9 (8), 3028–3039. doi:10.1039/d0bm01801e
Kimiz-Gebologlu, I., and Oncel, S. S. (2022). Exosomes: Large-scale production, isolation, drug loading efficiency, and biodistribution and uptake. J. Control. Release 347, 533–543. doi:10.1016/j.jconrel.2022.05.027
Kooijmans, S. A. A., Stremersch, S., Braeckmans, K., de Smedt, S. C., Hendrix, A., Wood, M. J. A., et al. (2013). Electroporation-induced sirna precipitation obscures the efficiency of sirna loading into extracellular vesicles. J. Control Release 172(1), 229–238. doi:10.1016/j.jconrel.2013.08.014
Kooijmans, S. A., Vader, P., van Dommelen, S. M., van Solinge, W. W., and Schiffelers, R. M. (2012). Exosome mimetics: A novel class of drug delivery systems. Int. J. Nanomedicine 7, 1525–1541. doi:10.2147/IJN.S29661
Kowal, J., Arras, G., Colombo, M., Jouve, M., Morath, J. P., Primdal-Bengtson, B., et al. (2016). Proteomic comparison defines novel markers to characterize heterogeneous populations of extracellular vesicle subtypes. Proc. Natl. Acad. Sci. U. S. A. 113 (8), E968–E977. doi:10.1073/pnas.1521230113
Kucuk, N., Primozic, M., Knez, Z., and Leitgeb, M. (2021). Exosomes engineering and their roles as therapy delivery tools, therapeutic targets, and biomarkers. Int. J. Mol. Sci. 22 (17), 9543. doi:10.3390/ijms22179543
Kwon, H. H., Yang, S. H., Lee, J., Park, B. C., Park, K. Y., Jung, J. Y., et al. (2020). Combination treatment with human adipose tissue stem cell-derived exosomes and fractional CO2 laser for acne scars: A 12-week prospective, double-blind, randomized, split-face study. Acta Derm. Venereol. 100 (18), adv00310. doi:10.2340/00015555-3666
Lamichhane, T. N., Raiker, R. S., and Jay, S. M. (2015). Exogenous DNA loading into extracellular vesicles via electroporation is size-dependent and enables limited gene delivery. Mol. Pharm. 12 (10), 3650–3657. doi:10.1021/acs.molpharmaceut.5b00364
Las Heras, K., Igartua, M., Santos-Vizcaino, E., and Hernandez, R. M. (2020). Chronic wounds: Current status, available strategies and emerging therapeutic solutions. J. Control. Release 328, 532–550. doi:10.1016/j.jconrel.2020.09.039
Lasser, C., Alikhani, V. S., Ekstrom, K., Eldh, M., Paredes, P. T., Bossios, A., et al. (2011). Human saliva, plasma and breast milk exosomes contain RNA: Uptake by macrophages. J. Transl. Med. 9, 9. doi:10.1186/1479-5876-9-9
Lee, P. Y., Cobain, E., Huard, J., and Huang, L. (2007). Thermosensitive hydrogel PEG-PLGA-PEG enhances engraftment of muscle-derived stem cells and promotes healing in diabetic wound. Mol. Ther. 15 (6), 1189–1194. doi:10.1038/sj.mt.6300156
Li, B., Luan, S., Chen, J., Zhou, Y., Wang, T., Li, Z., et al. (2020a). The MSC-derived exosomal lncRNA H19 promotes wound healing in diabetic foot ulcers by upregulating PTEN via MicroRNA-152-3p. Mol. Ther. - Nucleic Acids 19, 814–826. doi:10.1016/j.omtn.2019.11.034
Li, M., Fang, F., Sun, M., Zhang, Y., Hu, M., and Zhang, J. (2022). Extracellular vesicles as bioactive nanotherapeutics: An emerging paradigm for regenerative medicine. Theranostics 12 (11), 4879–4903. doi:10.7150/thno.72812
Li, X., Liu, L., Yang, J., Yu, Y., Chai, J., Wang, L., et al. (2016). Exosome derived from human umbilical cord mesenchymal stem cell mediates MiR-181c attenuating burn-induced excessive inflammation. EBioMedicine 8, 72–82. doi:10.1016/j.ebiom.2016.04.030
Li, X., Wang, Y., Shi, L., Li, B., Li, J., Wei, Z., et al. (2020b). Magnetic targeting enhances the cutaneous wound healing effects of human mesenchymal stem cell-derived iron oxide exosomes. J. Nanobiotechnology 18 (1), 113. doi:10.1186/s12951-020-00670-x
Li, X., Xie, X., Lian, W., Shi, R., Han, S., Zhang, H., et al. (2018). Exosomes from adipose-derived stem cells overexpressing Nrf2 accelerate cutaneous wound healing by promoting vascularization in a diabetic foot ulcer rat model. Exp. Mol. Med. 50 (4), 1–14. doi:10.1038/s12276-018-0058-5
Lian, N., and Li, T. (2016). Growth factor pathways in hypertrophic scars: Molecular pathogenesis and therapeutic implications. Biomed. Pharmacother. 84, 42–50. doi:10.1016/j.biopha.2016.09.010
Liang, Y., Duan, L., Lu, J., and Xia, J. (2021). Engineering exosomes for targeted drug delivery. Theranostics 11 (7), 3183–3195. doi:10.7150/thno.52570
Liu, L., Liu, Y., Feng, C., Chang, J., Fu, R., Wu, T., et al. (2019). Lithium-containing biomaterials stimulate bone marrow stromal cell-derived exosomal miR-130a secretion to promote angiogenesis. Biomaterials 192, 523–536. doi:10.1016/j.biomaterials.2018.11.007
Liu, W., Yu, M., Xie, D., Wang, L., Ye, C., Zhu, Q., et al. (2020). Melatonin-stimulated MSC-derived exosomes improve diabetic wound healing through regulating macrophage M1 and M2 polarization by targeting the PTEN/AKT pathway. Stem Cell. Res. Ther. 11 (1), 259. doi:10.1186/s13287-020-01756-x
Liu, Y., Zhuang, X., Yu, S., Yang, N., Zeng, J., Liu, X., et al. (2021). Exosomes derived from stem cells from apical papilla promote craniofacial soft tissue regeneration by enhancing Cdc42-mediated vascularization. Stem Cell. Res. Ther. 12 (1), 76. doi:10.1186/s13287-021-02151-w
Lu, M., Peng, L., Ming, X., Wang, X., Cui, A., Li, Y., et al. (2019). Enhanced wound healing promotion by immune response-free monkey autologous iPSCs and exosomes vs. their allogeneic counterparts. EBioMedicine 42, 443–457. doi:10.1016/j.ebiom.2019.03.011
Lv, H., Zhang, S., Wang, B., Cui, S., and Yan, J. (2006). Toxicity of cationic lipids and cationic polymers in gene delivery. J. Control. Release 114 (1), 100–109. doi:10.1016/j.jconrel.2006.04.014
Lv, L., Sheng, C., and Zhou, Y. (2020a). Extracellular vesicles as a novel therapeutic tool for cell-free regenerative medicine in oral rehabilitation. J. Oral Rehabil. 47 (1), 29–54. doi:10.1111/joor.12885
Lv, Q., Deng, J., Chen, Y., Wang, Y., Liu, B., and Liu, J. (2020b). Engineered human adipose stem-cell-derived exosomes loaded with miR-21-5p to promote diabetic cutaneous wound healing. Mol. Pharm. 17 (5), 1723–1733. doi:10.1021/acs.molpharmaceut.0c00177
Maqsood, M., Kang, M., Wu, X., Chen, J., Teng, L., and Qiu, L. (2020). Adult mesenchymal stem cells and their exosomes: Sources, characteristics, and application in regenerative medicine. Life Sci. 256, 118002. doi:10.1016/j.lfs.2020.118002
Marcus, M. E., and Leonard, J. N. (2013). FedExosomes: Engineering therapeutic biological nanoparticles that truly deliver. Pharm. (Basel) 6 (5), 659–680. doi:10.3390/ph6050659
Martinez-Lostao, L., Garcia-Alvarez, F., Basanez, G., Alegre-Aguaron, E., Desportes, P., Larrad, L., et al. (2010). Liposome-bound APO2L/TRAIL is an effective treatment in a rabbit model of rheumatoid arthritis. Arthritis Rheum. 62 (8), 2272–2282. doi:10.1002/art.27501
Md Fadilah, N. I., Mohd Abdul Kader Jailani, M. S., Badrul Hisham, M. A. I., Sunthar Raj, N., Shamsuddin, S. A., Ng, M. H., et al. (2022). Cell secretomes for wound healing and tissue regeneration: Next generation acellular based tissue engineered products. J. Tissue Eng. 13, 204173142211142. doi:10.1177/20417314221114273
Mishra, A., Singh, P., Qayoom, I., Prasad, A., and Kumar, A. (2021). Current strategies in tailoring methods for engineered exosomes and future avenues in biomedical applications. J. Mat. Chem. B 9 (32), 6281–6309. doi:10.1039/d1tb01088c
Monavarian, M., Kader, S., Moeinzadeh, S., and Jabbari, E. (2019). Regenerative scar-free skin wound healing. Tissue Eng. Part B Rev. 25 (4), 294–311. doi:10.1089/ten.TEB.2018.0350
Muller, L., Hong, C. S., Stolz, D. B., Watkins, S. C., and Whiteside, T. L. (2014). Isolation of biologically-active exosomes from human plasma. J. Immunol. Methods 411, 55–65. doi:10.1016/j.jim.2014.06.007
Nasiri Kenari, A., Cheng, L., and Hill, A. F. (2020). Methods for loading therapeutics into extracellular vesicles and generating extracellular vesicles mimetic-nanovesicles. Methods 177, 103–113. doi:10.1016/j.ymeth.2020.01.001
Nava, S., Sordi, V., Pascucci, L., Tremolada, C., Ciusani, E., Zeira, O., et al. (2019). Long-lasting anti-inflammatory activity of human microfragmented adipose tissue. Stem Cells Int. 2019, 1–13. doi:10.1155/2019/5901479
Negut, I., Grumezescu, V., and Grumezescu, A. M. (2018). Treatment strategies for infected wounds. Molecules 23 (9), 2392. doi:10.3390/molecules23092392
Ogawa, R. (2017). Keloid and hypertrophic scars are the result of chronic inflammation in the reticular dermis. Int. J. Mol. Sci. 18 (3), 606. doi:10.3390/ijms18030606
Pascucci, L., Cocce, V., Bonomi, A., Ami, D., Ceccarelli, P., Ciusani, E., et al. (2014). Paclitaxel is incorporated by mesenchymal stromal cells and released in exosomes that inhibit in vitro tumor growth: A new approach for drug delivery. J. Control. Release 192, 262–270. doi:10.1016/j.jconrel.2014.07.042
Pegtel, D. M., Peferoen, L., and Amor, S. (2014). Extracellular vesicles as modulators of cell-to-cell communication in the healthy and diseased brain. Phil. Trans. R. Soc. B 369 (1652), 20130516. doi:10.1098/rstb.2013.0516
Peng, H., Ji, W., Zhao, R., Yang, J., Lu, Z., Li, Y., et al. (2020a). Exosome: A significant nano-scale drug delivery carrier. J. Mat. Chem. B 8 (34), 7591–7608. doi:10.1039/d0tb01499k
Peng, Q., Yang, J. Y., and Zhou, G. (2020b). Emerging functions and clinical applications of exosomes in human oral diseases. Cell. Biosci. 10, 68. doi:10.1186/s13578-020-00424-0
Phinney, D. G., and Pittenger, M. F. (2017). Concise review: MSC-derived exosomes for cell-free therapy. Stem Cells 35 (4), 851–858. doi:10.1002/stem.2575
Pomatto, M., Gai, C., Negro, F., Cedrino, M., Grange, C., Ceccotti, E., et al. (2021). Differential therapeutic effect of extracellular vesicles derived by bone marrow and adipose mesenchymal stem cells on wound healing of diabetic ulcers and correlation to their cargoes. Int. J. Mol. Sci. 22 (8), 3851. doi:10.3390/ijms22083851
Powers, J. G., Higham, C., Broussard, K., and Phillips, T. J. (2016). Wound healing and treating wounds: Chronic wound care and management. J. Am. Acad. Dermatol. 74 (4), 607–625. doi:10.1016/j.jaad.2015.08.070
Qian, Z., Bai, Y., Zhou, J., Li, L., Na, J., Fan, Y., et al. (2020). A moisturizing chitosan-silk fibroin dressing with silver nanoparticles-adsorbed exosomes for repairing infected wounds. J. Mat. Chem. B 8 (32), 7197–7212. doi:10.1039/d0tb01100b
Ratajczak, M. Z., Kucia, M., Jadczyk, T., Greco, N. J., Wojakowski, W., Tendera, M., et al. (2012). Pivotal role of paracrine effects in stem cell therapies in regenerative medicine: Can we translate stem cell-secreted paracrine factors and microvesicles into better therapeutic strategies? Leukemia 26 (6), 1166–1173. doi:10.1038/leu.2011.389
Rider, M. A., Hurwitz, S. N., and Meckes, D. G. (2016). ExtraPEG: A polyethylene glycol-based method for enrichment of extracellular vesicles. Sci. Rep. 6, 23978. doi:10.1038/srep23978
Rippa, A. L., Kalabusheva, E. P., and Vorotelyak, E. A. (2019). Regeneration of dermis: Scarring and cells involved. Cells 8 (6), 607. doi:10.3390/cells8060607
Rustad, K. C., Wong, V. W., Sorkin, M., Glotzbach, J. P., Major, M. R., Rajadas, J., et al. (2012). Enhancement of mesenchymal stem cell angiogenic capacity and stemness by a biomimetic hydrogel scaffold. Biomaterials 33 (1), 80–90. doi:10.1016/j.biomaterials.2011.09.041
Salunkhe, S., DheerajBasak, M., Chitkara, D., and Mittal, A. (2020). Surface functionalization of exosomes for target-specific delivery and in vivo imaging & tracking: Strategies and significance. J. Control. Release 326, 599–614. doi:10.1016/j.jconrel.2020.07.042
Saunderson, S. C., Dunn, A. C., Crocker, P. R., and McLellan, A. D. (2014). CD169 mediates the capture of exosomes in spleen and lymph node. Blood 123 (2), 208–216. doi:10.1182/blood-2013-03-489732
Shao, H., Im, H., Castro, C. M., Breakefield, X., Weissleder, R., and Lee, H. (2018). New technologies for analysis of extracellular vesicles. Chem. Rev. 118 (4), 1917–1950. doi:10.1021/acs.chemrev.7b00534
Sharma, S., Rasool, H. I., Palanisamy, V., Mathisen, C., Schmidt, M., Wong, D. T., et al. (2010). Structural-mechanical characterization of nanoparticle exosomes in human saliva, using correlative AFM, FESEM, and force spectroscopy. ACS Nano 4 (4), 1921–1926. doi:10.1021/nn901824n
Shi, Q., Huo, N., Wang, X., Yang, S., Wang, J., and Zhang, T. (2020). Exosomes from oral tissue stem cells: Biological effects and applications. Cell. Biosci. 10, 108. doi:10.1186/s13578-020-00471-7
Smyth, T., Petrova, K., Payton, N. M., Persaud, I., Redzic, J. S., Graner, M. W., et al. (2014). Surface functionalization of exosomes using click chemistry. Bioconjug. Chem. 25 (10), 1777–1784. doi:10.1021/bc500291r
Sokolova, V., Ludwig, A. K., Hornung, S., Rotan, O., Horn, P. A., Epple, M., et al. (2011). Characterisation of exosomes derived from human cells by nanoparticle tracking analysis and scanning electron microscopy. Colloids Surfaces B Biointerfaces 87 (1), 146–150. doi:10.1016/j.colsurfb.2011.05.013
Stavrou, A., and Ortiz, A. (2022). Extracellular vesicles: A novel tool in nanomedicine and cancer treatment. Cancers (Basel) 14 (18), 4450. doi:10.3390/cancers14184450
Su, D., Tsai, H. I., Xu, Z., Yan, F., Wu, Y., Xiao, Y., et al. (2019). Exosomal PD-L1 functions as an immunosuppressant to promote wound healing. J. Extracell. Vesicles 9 (1), 1709262. doi:10.1080/20013078.2019.1709262
Sun, D., Zhuang, X., Xiang, X., Liu, Y., Zhang, S., Liu, C., et al. (2010). A novel nanoparticle drug delivery system: The anti-inflammatory activity of curcumin is enhanced when encapsulated in exosomes. Mol. Ther. 18 (9), 1606–1614. doi:10.1038/mt.2010.105
Takeo, M., Lee, W., and Ito, M. (2015). Wound healing and skin regeneration. Cold Spring Harb. Perspect. Med. 5 (1), a023267. doi:10.1101/cshperspect.a023267
Tanaka, S., Hamada, Y., Yokoyama, Y., Yamamoto, H., and Kogo, M. (2021). Osteopontin-derived synthetic peptide SVVYGLR upregulates functional regeneration of oral and maxillofacial soft-tissue injury. Jpn. Dent. Sci. Rev. 57, 174–181. doi:10.1016/j.jdsr.2021.09.002
Tao, S. C., Guo, S. C., Li, M., Ke, Q. F., Guo, Y. P., and Zhang, C. Q. (2017). Chitosan wound dressings incorporating exosomes derived from MicroRNA-126-overexpressing synovium mesenchymal stem cells provide sustained release of exosomes and heal full-thickness skin defects in a diabetic rat model. Stem Cells Transl. Med. 6 (3), 736–747. doi:10.5966/sctm.2016-0275
Tao, S. C., Rui, B. Y., Wang, Q. Y., Zhou, D., Zhang, Y., and Guo, S. C. (2018). Extracellular vesicle-mimetic nanovesicles transport LncRNA-H19 as competing endogenous RNA for the treatment of diabetic wounds. Drug Deliv. (Lond). 25 (1), 241–255. doi:10.1080/10717544.2018.1425774
Taylor, D. D., and Shah, S. (2015). Methods of isolating extracellular vesicles impact down-stream analyses of their cargoes. Methods 87, 3–10. doi:10.1016/j.ymeth.2015.02.019
Thery, C., Amigorena, S., Raposo, G., and Clayton, A. (2006). Isolation and characterization of exosomes from cell culture supernatants and biological fluids. Curr. Protoc. Cell. Biol. 3, Unit 3.22. doi:10.1002/0471143030.cb0322s30
Thery, C., Witwer, K. W., Aikawa, E., Alcaraz, M. J., Anderson, J. D., Andriantsitohaina, R., et al. (2018). Minimal information for studies of extracellular vesicles 2018 (MISEV2018): A position statement of the international society for extracellular vesicles and update of the MISEV2014 guidelines. J. Extracell. Vesicles 7 (1), 1535750. doi:10.1080/20013078.2018.1535750
Ti, D., Hao, H., Tong, C., Liu, J., Dong, L., Zheng, J., et al. (2015). LPS-preconditioned mesenchymal stromal cells modify macrophage polarization for resolution of chronic inflammation via exosome-shuttled let-7b. J. Transl. Med. 13, 308. doi:10.1186/s12967-015-0642-6
Turturici, G., Tinnirello, R., Sconzo, G., and Geraci, F. (2014). Extracellular membrane vesicles as a mechanism of cell-to-cell communication: Advantages and disadvantages. Am. J. Physiology-Cell Physiology 306 (7), C621–C633. doi:10.1152/ajpcell.00228.2013
Van Deun, J., Mestdagh, P., Sormunen, R., Cocquyt, V., Vermaelen, K., Vandesompele, J., et al. (2014). The impact of disparate isolation methods for extracellular vesicles on downstream RNA profiling. J. Extracell. Vesicles 3, 24858. doi:10.3402/jev.v3.24858
van Niel, G., D'Angelo, G., and Raposo, G. (2018). Shedding light on the cell biology of extracellular vesicles. Nat. Rev. Mol. Cell. Biol. 19 (4), 213–228. doi:10.1038/nrm.2017.125
Vandergriff, A., Huang, K., Shen, D., Hu, S., Hensley, M. T., Caranasos, T. G., et al. (2018). Targeting regenerative exosomes to myocardial infarction using cardiac homing peptide. Theranostics 8 (7), 1869–1878. doi:10.7150/thno.20524
Vazquez-Rios, A. J., Molina-Crespo, A., Bouzo, B. L., Lopez-Lopez, R., Moreno-Bueno, G., and de la Fuente, M. (2019). Exosome-mimetic nanoplatforms for targeted cancer drug delivery. J. Nanobiotechnology 17 (1), 85. doi:10.1186/s12951-019-0517-8
Veith, A. P., Henderson, K., Spencer, A., Sligar, A. D., and Baker, A. B. (2019). Therapeutic strategies for enhancing angiogenesis in wound healing. Adv. Drug Deliv. Rev. 146, 97–125. doi:10.1016/j.addr.2018.09.010
Villarroya-Beltri, C., Baixauli, F., Gutierrez-Vazquez, C., Sanchez-Madrid, F., and Mittelbrunn, M. (2014). Sorting it out: Regulation of exosome loading. Semin. Cancer Biol. 28, 3–13. doi:10.1016/j.semcancer.2014.04.009
Vu, N. B., Nguyen, H. T., Palumbo, R., Pellicano, R., Fagoonee, S., and Pham, P. V. (2021). Stem cell-derived exosomes for wound healing: Current status and promising directions. Minerva Med. 112 (3), 384–400. doi:10.23736/S0026-4806.20.07205-5
Wan, S., Zhang, L., Wang, S., Liu, Y., Wu, C., Cui, C., et al. (2017). Molecular recognition-based DNA nanoassemblies on the surfaces of nanosized exosomes. J. Am. Chem. Soc. 139 (15), 5289–5292. doi:10.1021/jacs.7b00319
Wang, J., Ma, P., Kim, D. H., Liu, B. F., and Demirci, U. (2021a). Towards microfluidic-based exosome isolation and detection for tumor therapy. Nano Today 37, 101066. doi:10.1016/j.nantod.2020.101066
Wang, J., Wu, H., Peng, Y., Zhao, Y., Qin, Y., Zhang, Y., et al. (2021b). Hypoxia adipose stem cell-derived exosomes promote high-quality healing of diabetic wound involves activation of PI3K/Akt pathways. J. Nanobiotechnology 19 (1), 202. doi:10.1186/s12951-021-00942-0
Wang, Q. L., Zhuang, X., Sriwastva, M. K., Mu, J., Teng, Y., Deng, Z., et al. (2018a). Blood exosomes regulate the tissue distribution of grapefruit-derived nanovector via CD36 and IGFR1 pathways. Theranostics 8 (18), 4912–4924. doi:10.7150/thno.27608
Wang, X., Zhao, X., Zhong, Y., Shen, J., and An, W. (2022a). Biomimetic exosomes: A new generation of drug delivery system. Front. Bioeng. Biotechnol. 10, 865682. doi:10.3389/fbioe.2022.865682
Wang, Y., Beekman, J., Hew, J., Jackson, S., Issler-Fisher, A. C., Parungao, R., et al. (2018b). Burn injury: Challenges and advances in burn wound healing, infection, pain and scarring. Adv. Drug Deliv. Rev. 123, 3–17. doi:10.1016/j.addr.2017.09.018
Wang, Y., Yu, T., and Hu, F. (2022b). Hypocapnia stimuli-responsive engineered exosomes delivering miR-218 facilitate sciatic nerve regeneration. Front. Bioeng. Biotechnol. 10, 825146. doi:10.3389/fbioe.2022.825146
Wang, Z. C., Zhao, W. Y., Cao, Y., Liu, Y. Q., Sun, Q., Shi, P., et al. (2020). The roles of inflammation in keloid and hypertrophic scars. Front. Immunol. 11, 603187. doi:10.3389/fimmu.2020.603187
Weaver, J. C. (1993). Electroporation: A general phenomenon for manipulating cells and tissues. J. Cell. Biochem. 51 (4), 426–435. doi:10.1002/jcb.2400510407
Weng, Y., Sui, Z., Shan, Y., Hu, Y., Chen, Y., Zhang, L., et al. (2016). Effective isolation of exosomes with polyethylene glycol from cell culture supernatant for in-depth proteome profiling. Analyst 141 (15), 4640–4646. doi:10.1039/c6an00892e
Wgealla, M., Liang, H., Chen, R., Xie, Y., Li, F., Qin, M., et al. (2022). Amniotic fluid derived stem cells promote skin regeneration and alleviate scar formation through exosomal miRNA-146a-5p via targeting CXCR4. J. Cosmet. Dermatol. doi:10.1111/jocd.14956
Wortzel, I., Dror, S., Kenific, C. M., and Lyden, D. (2019). Exosome-mediated metastasis: Communication from a distance. Dev. Cell. 49 (3), 347–360. doi:10.1016/j.devcel.2019.04.011
Wu, C. X., and Liu, Z. F. (2018). Proteomic profiling of sweat exosome suggests its involvement in skin immunity. J. Investig. Dermatol. 138 (1), 89–97. doi:10.1016/j.jid.2017.05.040
Wu, D., Chang, X., Tian, J., Kang, L., Wu, Y., Liu, J., et al. (2021). Bone mesenchymal stem cells stimulation by magnetic nanoparticles and a static magnetic field: Release of exosomal miR-1260a improves osteogenesis and angiogenesis. J. Nanobiotechnology 19 (1), 209. doi:10.1186/s12951-021-00958-6
Wu, D., Kang, L., Tian, J., Wu, Y., Liu, J., Li, Z., et al. (2020). <p>Exosomes Derived from Bone Mesenchymal Stem Cells with the Stimulation of Fe<sub>3</sub>O<sub>4</sub> Nanoparticles and Static Magnetic Field Enhance Wound Healing through Upregulated miR-21-5p</p>. Int. J. Nanomedicine 15, 7979–7993. doi:10.2147/IJN.S275650
Yang, J., Chen, Z., Pan, D., Li, H., and Shen, J. (2020). <p>Umbilical cord-derived mesenchymal stem cell-derived exosomes combined pluronic F127 hydrogel promote chronic diabetic wound healing and complete skin regeneration</p>. Int. J. Nanomedicine 15, 5911–5926. doi:10.2147/IJN.S249129
Yang, K., Li, D., Wang, M., Xu, Z., Chen, X., Liu, Q., et al. (2019). Exposure to blue light stimulates the proangiogenic capability of exosomes derived from human umbilical cord mesenchymal stem cells. Stem Cell. Res. Ther. 10 (1), 358. doi:10.1186/s13287-019-1472-x
Yang, Z., Yang, Y., Xu, Y., Jiang, W., Shao, Y., Xing, J., et al. (2021). Biomimetic nerve guidance conduit containing engineered exosomes of adipose-derived stem cells promotes peripheral nerve regeneration. Stem Cell. Res. Ther. 12 (1), 442. doi:10.1186/s13287-021-02528-x
Yu, M., Liu, W., Li, J., Lu, J., Lu, H., Jia, W., et al. (2020). Exosomes derived from atorvastatin-pretreated MSC accelerate diabetic wound repair by enhancing angiogenesis via AKT/eNOS pathway. Stem Cell. Res. Ther. 11 (1), 350. doi:10.1186/s13287-020-01824-2
Yuan, R., Dai, X., Li, Y., Li, C., and Liu, L. (2021). Exosomes from miR-29a-modified adipose-derived mesenchymal stem cells reduce excessive scar formation by inhibiting TGF-β2/Smad3 signaling. Mol. Med. Rep. 24 (5), 758. doi:10.3892/mmr.2021.12398
Zhang, B., Wang, M., Gong, A., Zhang, X., Wu, X., Zhu, Y., et al. (2015a). HucMSC-exosome mediated-wnt4 signaling is required for cutaneous wound healing. Stem Cells 33 (7), 2158–2168. doi:10.1002/stem.1771
Zhang, B., Wu, X., Zhang, X., Sun, Y., Yan, Y., Shi, H., et al. (2015b). Human umbilical cord mesenchymal stem cell exosomes enhance angiogenesis through the wnt4/β-catenin pathway. Stem Cells Transl. Med. 4 (5), 513–522. doi:10.5966/sctm.2014-0267
Zhang, J., Li, S., Li, L., Li, M., Guo, C., Yao, J., et al. (2015c). Exosome and exosomal microRNA: Trafficking, sorting, and function. Genomics Proteomics Bioinforma. 13 (1), 17–24. doi:10.1016/j.gpb.2015.02.001
Zhang, N., Sun, N., and Deng, C. (2021). Rapid isolation and proteome analysis of urinary exosome based on double interactions of Fe3O4@TiO2-DNA aptamer. Talanta 221, 121571. doi:10.1016/j.talanta.2020.121571
Zhang, Y., Wang, Z., and Gemeinhart, R. A. (2013). Progress in microRNA delivery. J. Control. Release 172 (3), 962–974. doi:10.1016/j.jconrel.2013.09.015
Zhao, P., Sui, B. D., Liu, N., Lv, Y. J., Zheng, C. X., Lu, Y. B., et al. (2017). Anti-aging pharmacology in cutaneous wound healing: Effects of metformin, resveratrol, and rapamycin by local application. Aging Cell. 16 (5), 1083–1093. doi:10.1111/acel.12635
Keywords: engineered, exosomes, stem cells, oral and maxillofacial surgery, wound healing
Citation: Hao M, Duan M, Yang Z, Zhou H, Li S, Xiang J, Wu H, Liu H, Chang L, Wang D and Liu W (2022) Engineered stem cell exosomes for oral and maxillofacial wound healing. Front. Bioeng. Biotechnol. 10:1038261. doi: 10.3389/fbioe.2022.1038261
Received: 06 September 2022; Accepted: 13 October 2022;
Published: 24 October 2022.
Edited by:
Hao Chen, Guangdong Provincial People’s Hospital, ChinaReviewed by:
Carlo Tremolada, Manchester Metropolitan University, United KingdomMohammad Karimipour, Tabriz University of Medical Sciences, Iran
Copyright © 2022 Hao, Duan, Yang, Zhou, Li, Xiang, Wu, Liu, Chang, Wang and Liu. This is an open-access article distributed under the terms of the Creative Commons Attribution License (CC BY). The use, distribution or reproduction in other forums is permitted, provided the original author(s) and the copyright owner(s) are credited and that the original publication in this journal is cited, in accordance with accepted academic practice. No use, distribution or reproduction is permitted which does not comply with these terms.
*Correspondence: Dongxu Wang, wang_dong_xu@jlu.edu.cn; Weiwei Liu, liuweiw@jlu.edu.cn
†These authors have contributed equally to this work and share first authorship