Prebiotics and β-Glucan as gut modifier feed additives in modulation of growth performance, protein utilization status and dry matter and lactose digestibility in weanling pigs
- 1Department of Animal Biosciences, University of Guelph, Guelph, ON, Canada
- 2Department of Animal Biosciences and One Health Institute, University of Guelph, Guelph, ON, Canada
There are growing interests in developing novel gut modifier feed additives and alternative therapeutics to replace antimicrobials to enhance efficiency of nutrient utilization and to address the antimicrobial resistance threat to public health facing the global pork production. Biological mechanisms of supplementing lactose for enhancing weanling pig growth and nitrogen utilization are unclear. Thus, this study was prompted to determine effects of dietary supplementation of 3 prebiotics and oat β-glucan vs. a sub-therapeutic antibiotic on growth performance, whole-body protein utilization status, the apparent total tract dry matter (DM) and lactose digestibility in weanling pigs fed corn and soybean meal (SBM)-based diets. Six experimental diets were formulated with corn (40%), SBM (28%) and supplemented with dried whey powder (20%) and fish meal (9%) with titanium oxide (0.30%) as the digestibility marker. Diet 1 (NC, negative control), as the basal diet, contained no antibiotics and no supplemental prebiotics or β-glucan. Diet 2 (PC, positive control), contained an antibiotic premix (Lincomix-44 at 0.10%) in the basal diet at the expense of cornstarch. Diets 3, 5 and 6 contained 0.75% of the three test prebiotics of retrograded cornstarch (Diet 3), Fibersol-2 (Diet 5, a modified digestion-resistant maltodextrin) and inulin (Diet 6), and the viscous soluble fiber oat β-glucan (Diet 4), respectively, at the expense of cornstarch. A total of 144 Yorkshire pigs, at the age of 21 days (d) and an average body weight (BW) of 5.5 kg, were allocated to 12 floor pens with 3 barrows and 3 gilts per pen, and fed one of the 6 diets for 21 d in 2 study blocks according to a completely randomized block design. Initial and final pig BW, average daily gain (ADG), average daily feed intake (ADFI), representative pig plasma urea concentration as well as the apparent total tract DM and lactose digestibility during d 8-15 were measured. Analyses of variances, Dunnett’s and Tukey’s tests were conducted on the endpoints by using the SAS mixed model. There were no differences (P > 0.05) in ADG, ADFI, feed to gain ratio, plasma urea concentration, the apparent total tract apparent DM and lactose digestibility and the predicted whole-gut lactase digestive capacity among the diets, as examined by the Tukey’s test. There were no differences (P > 0.05) in these endpoints between each of the four treatment diets and the NC or the PC diet as examined by the Dunnett’s test. The total tract lactose digestibility was determined to be at 100%. The predicted whole-gut lactase digestive capacity was about eight times of the daily lactose intake when dietary lactose contents were supplemented at 10 - 12% (as-fed basis). In conclusion, dietary supplementation (at 0.75%) of the prebiotics and the oat β-glucan did not significantly affect the major growth performance endpoints, whole-body protein utilization status as well as the apparent total tract DM and lactose digestibility in the weanling pigs fed the corn and SBM-based diets. The promoting effect for growth and nitrogen utilization associated with dietary supplementation of lactose is due to the fact that lactose is a completely and rapidly digestible sugar rather than acting as an effective prebiotic in weanling pig nutrition.
Introduction
Porcine weaning transition is associated with retarded growth and poor efficiency of nutrient utilization along with gut dysbiosis and changes in gut microbiome and microbiota harboring zoonotic and pathogenic bacteria (Pluske et al., 2002; Lackeyram et al., 2010; Tran et al., 2018; Fan et al., 2022). The main zoonotic bacteria causing weanling porcine gut infectious diseases include the Gram-negative enterotoxigenic E. coli spp. responsible for colibacillosis (Pluske et al., 2002; Nagy and Fekete, 2005; Rhouma et al., 2017; Tran et al., 2018); and Salmonella spp. causing salmonellosis (Pluske et al., 2002; Evangelopoulou et al., 2015; Tran et al., 2018). Being recognized as one of the most common zoonoses, the Gram-negative Campylobacter spp., including the recently identified Campylobacter lanienae, have been well identified in porcine gut and can cause campylobacteriosis in humans (Schweitzer et al., 2011; Silva et al., 2011; Tran et al., 2018; Costa and Iraola, 2019; Fornefett et al., 2021); however, these zoonotic bacteria have limited pathogenic effects on the porcine gut (Rath et al., 2021). The Gram-positive Mycobacterium tuberculosis spp. have also been recognized as zoonotic bacteria in multiple food animal species and their cases in swine production are sporadic and only region-specific (Arega et al., 2013; Tran et al., 2018; Ramos et al., 2020), while pathogenesis of these zoonotic bacteria in pigs has only been experimentally demonstrated (Niroula et al., 2022). Furthermore, the Gram-negative Lawsonia intracellularis (L. intracellularis) is responsible for the prevalent porcine enteric disease, i.e., porcine proliferative enteropathy (PPE), also known as ileitis, particularly in young pigs (Smith and Lawson, 2001; Winkelman et al., 2002; Karuppannan and Opriessnig, 2018; Jansen et al., 2019). In the late 1940s, the development of supplemental vitamins, specifically vitamin B12, led to the discovery that antibiotics could also be used to improve growth and feed intake in food animal production (Summons, 1968; Cromwell, 2001). When comprehensively comparing vaccines and antibiotics for managing L. intracellularis, Jansen et al. (2019) concluded that prophylactic and treatment antibiotics were more cost-effective options while vaccination was a less cost-effective strategy. Therefore, the modern practice of feeding weanling pigs with antimicrobials, including antibiotics and pharmacological levels of copper and zinc oxide as therapeutics and growth promoters, for controlling zoonotic bacteria, managing gut health and enhancing growth, has been established in pork production for more than seven decades.
But the rise of the antimicrobial resistance has motivated the search for novel gut modifier feed additives and alternative therapeutics to replace antimicrobials in the past two decades (Pluske et al., 2002; Heo et al., 2013; Fan and Archbold, 2015; Tran et al., 2018; Wang et al., 2020; Tan et al., 2021; Ma et al., 2021). Numerous non-antimicrobial alternative feed additives have been primarily evaluated for their impact on host gut and immunological responses as well as overall health improvement with prebiotics and other soluble fibers arising as viable options for improving weanling swine health with limited understanding about their effects on efficiency of nutrient utilization (Pluske et al., 2002; Heo et al., 2013; Tran et al., 2018; Jha et al., 2019; Tiwari et al., 2019; Tan et al., 2021). It should be pointed out that newly emerging gut modifier feed additives and therapeutics as alternatives to antimicrobials are highly regulated by government regulatory agencies and are thus implemented differentially by swine and feed industrial end-users in terms of as novel feed additives vs. as medicinal therapeutics in many countries including Canada (Health Canada and Canadian Food Inspection Agency, CFIA, 2019). For example, zinc at 2,000 ppm has been approved as a therapeutic for treatment of diarrhea, while the gut trophic amino acid (AA) L-glutamine has been approved and regulated as a medicinal therapeutic for treatment of gut mucosal trophy in food animals in Canada (Health Canada and Canadian Food Inspection Agency, CFIA, 2019). Interestingly, recent studies suggest that pharmacological levels of antimicrobials improved growth through enhancing feed intake with no impacts on dry matter (DM), energy and crude protein (CP) digestibility in weanling pigs (Chen et al., 2021; Burrough et al., 2022). Therefore, there are both scientific and regulatory needs to further understand efficacy and biological roles of prebiotics and other soluble fibers arising as potentially viable alternative gut modifier feed additives for improving growth and efficiency of nutrient utilization in weanling pigs.
Prebiotics are described as novel ingredients that resist gastrointestinal digestion and absorption by host animal enzymes and can be selectively fermented by intestinal microflora and have a positive impact on the host (Gibson et al., 2004). The classified prebiotics, including retrograded resistant starch (RS), Fibersol-2 (i.e., a commercial supplement that is a resistant maltodextrin) and inulin, have been well documented for their prebiotic effects (Fastinger et al., 2008). Prebiotics are often soluble fiber but not all soluble fiber can be described as a prebiotic. Thus, the aforementioned prebiotics fall into the definition as outlined in the seminal paper by Gibson et al. (2004). Resistant starch can increase fecal bulk, increase the molar ratio of the trophic and signaling volatile short-chain fatty acids such as butyrate and dilute fecal bile acids (Zaman and Sarbini, 2016). Several previous porcine studies supplemented various sources of RS as functional ingredients (e.g., inclusion levels at 7 – 10%) rather than as gut modifier feed additives (e.g., inclusion levels at< 1%) in their diets (Bird et al., 2007; Rideout et al., 2008; Bhandari et al., 2009; Fouhse et al., 2015). Fibersol-2 was shown in shifting fecal bacterial populations of Bifidobacterium to higher proportions and increasing the production of butyrate in health humans (Fastinger et al., 2008). Xing et al. (2018) reported that dietary supplementation (up to 0.40%) of gradient levels of Fibersol-2 as a novel feed additive could improve circulating blood systemic oxidative, immune and lipid parameters without affecting growth and digestibility endpoints in weanling pigs. Inulin was reviewed to have immune modulation effects (Seifert and Watzl, 2007). When included as a functional ingredient (at 5%) in diets, dietary supplementation of crude inulin extract reduced fecal excretion of a key odor compound of skatole and inconsistently modulated efficiency of nutrient utilization in grower pigs (Rideout and Fan, 2004; Rideout et al., 2004). However, weaning pig studies with inulin as feed additives did not reveal significant improvements in growth performances (Pierce et al., 2005; Mair et al., 2010; Uerlings et al., 2021). Overall, there are limited studies regarding prebiotics as gut modifier feed additives to enhance growth and nutrient utilization in weanling pigs.
The viscous soluble fiber, β-glucans, have received some interests because of the recognition that some sources of β-glucans have immune modulating effects (Willment et al., 2001). Because of the heterogenous nature of β-glucans that arise from a variety of sources, their activities are diverse and not completely confirmed through sufficient research, which makes β-glucans ineligible to receive a prebiotic designation (Gibson et al., 2004). Despite this, the research that has shown regulatory effects of specific sources of β-glucans would suggest that dismissing the use of β-glucans to improve growth performance and health parameters would be premature. Oat β-glucan as a functional viscous soluble fibre ingredient was shown effective in modulating bile acid secretion (Lia et al., 1995); regulating the immune system with anti-inflammatory capabilities (Chang et al., 2006) as well as increasing digesta viscosity and decreasing blood glucose peak levels (Hooda et al., 2010; Hooda et al., 2011). In the study by Wu et al. (2021), dietary supplementation (at 0.02%) of a unique bacterial source of β-glucan could improve gut morphology, biochemical and microbiome endpoints with improved growth in weanling pigs. Thus, there is an incentive to further investigate the use of readily available sources of β-glucans as gut modifier feed additives to enhance growth and nutrient utilization in weanling pigs.
It has been well established that the porcine gut lactase activity is at its highest level immediately after birth and during the neonatal suckling period (Manners and Stevens, 1972; Ekstrom et al., 1975; Kelly et al., 1991). Following this period, porcine gut lactase activity decreases dramatically during the weaning transition (Manners and Stevens, 1972; Ekstrom et al., 1975; Kelly et al., 1991; Lackeyram, 2012). Paradoxically, the positive role of dietary supplemental lactose in improving weanling pig growth performance and efficiency of whole-body nitrogen (N) retention has received considerable attention. Crystalline lactose and lactose from dried whey powder are well documented to be the essential dietary carbohydrate for improving growth performance and/or efficiency of the whole-body N retention in weanling pigs (Mahan, 1992; Nessmith et al., 1997; Cromwell et al., 2008; Acosta et al., 2020; Zhao et al., 2021). Mahan and Newton (1993) demonstrated that dextrose was effective but cornstarch was ineffective to replace lactose in improving growth performance and efficiency of the whole-body N utilization in weanling pigs, suggesting that lactose is still likely the highly and rapidly digestible carbohydrate in weanling pigs. However, Fuller (1992) and Zhao et al. (2021) suggested that lactose likely garnered its positive prebiotic effects by modulating the gut microflora such as the commensal bacteria lactobacilli. Conversely, studies by Krause et al. (1995; 1997) did not observe improvements in the adherent lactobacillus counts in the ileum and the cecum in response to lactose supplementation in weanling pigs. Through metagenomic analyses, Wang et al. (2019) catalogued gut bacterial lactose degradation enzyme genes in weanling pigs. Acosta et al. (2020) further shown that bacteria lactobacilli spp. would have played limited roles for improving the whole-body N retention efficiency at the post-absorptive level via improving gut functions. Zhao et al. (2021) proposed that high lactose digestibility could be the biological cause for the supplemental lactose impacts on weanling pigs. Surprisingly, to the best of our knowledge, supplemental lactose digestibility has not been reported in weanling pigs to date. Thus, examination of in vivo responses in lactose digestibility and the whole-gut lactase digestive capacity in the absence and presence of feed antibiotics; as well as the effects of prebiotic and β-glucan supplementation will help further reveal the biological mechanisms of dietary supplemental lactose in the support of growth performance and efficiency of the whole-body N utilization in the weanling pig.
As well documented, L. intracellularis-caused PPE is prevalent and is also partly responsible for weaning-associated growth retardation, morbidity and mortality in weanling pigs under commercial settings; and sub-therapeutic and therapeutic levels of Lincomix-44 were shown to be effective for the control of weanling pig PPE (Winkelman et al., 2002; Jansen et al., 2019). We hypothesized that weanling pigs were not susceptible to PPE under a reasonable sanitary floor pen condition in research settings, thus dietary supplementation of the sub-therapeutic level of Lincomix-44 would be ineffective in improving growth performances and efficiency of nutrient utilization in weanling pigs. Whereas dietary supplementation of the prebiotics of RS, resistant maltodextrin Fibersol-2 and inulin and the oat β-glucan, as the gut modifier feed additives rather than as functional ingredients at much higher levels, would directly and indirectly optimize gut microbiota and modulate gut mucosa, hence improving growth performances and efficiency of nutrient utilization in weanling pigs under a reasonable sanitary floor pen condition in our research settings. Furthermore, weanling pig residual whole-gut lactase digestive capacity would be sufficient to completely digest supplemental lactose, which would not be affected by dietary supplementation of the sub-therapeutic antibiotic and the anticipated gut modifier feed additives. Therefore, the objectives of this study were to investigate the effects of the three prebiotics, i.e., retrograded cornstarch (RCS), Fibersol-2 and inulin, and oat β-glucan on growth performances, the whole-body N utilization status as indicated by changes in blood urea concentration responses as well as the apparent total tract DM and lactose digestibility and the predicted whole-gut lactase digestive capacity in the absence and presence of the sub-therapeutic level of Lincomix-44 in weanling pigs fed corn and soybean meal (SBM)-based diets.
Materials and methods
Animals and management
A total of 144 Yorkshire piglets, with a ratio of one barrow to one gilt, were used for this study. The study was carried out in two blocks with 72 pigs in each block. Each block contained 12 pens and 36 pigs with two replicate pens for each of six diets. Piglets were divided into pens with six animals per pen. Piglets were weaned at 21 days (d) of age and given ad libitum access to their test diets and water. Diets were provided in stainless-steel trough feeders measuring 26.5 cm deep by 76 cm long. Water was provided continuously through one dish per pen measuring 17 cm across and 15 cm deep with a push activated water dispenser.
Pigs were obtained from the University of Guelph Arkell Swine Research Station and transported to the animal research wing in the Department of Animal Biosciences at the University. Each group of six piglets were housed in each floor pen (160 cm in length by 130 m in width and 13 cm off the ground) with rubberized woven wire floors called Tenderfoot® with openings measuring 3.0 cm by 1.5 cm. The room was kept at 24°C and the pens were supplemented with heating lamps elevated about 65 cm above the piglet sleeping area. Each floor pen was equipped with a rubber mat measuring approximately 60 cm by 95 cm that was placed in the corner under the heating lamp to provide a comfortable sleeping area.
The study nursery room and floor pens were thoroughly disinfected prior to the arrival of each block of weaning pigs. The pens were thoroughly cleaned on a biweekly basis with a high-pressure water hose. Due to the design of the pens and the room in which the animals were housed, fewer cleanings would have greatly impacted the sanitary conditions and welfare of the animals due to unreasonable exposure to fecal contamination. Conversely, more frequent cleanings were expected to poorly represent the disease challenge experienced by pigs in a typical commercial facility. Thus, our weanling pig study room and pens were managed under an overall reasonable hygiene condition.
Animals were fed the experimental diets for 21 d with ad libitum access being allowed. Feeders and water dispensers were checked twice per d to ensure proper functioning, adequate fill and to clean and replace diets as needed. Soiled and waste diets were collected in foil trays (120 cm by 90 cm) that were placed beneath the feeders, as well as being removed from the feeders and then dried to an air-dry basis using drying ovens set at 65°C, if necessary, and weighed to determine how much test diet was consumed.
The piglets used in this study were cared for in accordance with the guidelines set out by the Canadian Council on Animal Care (CCAC, 1993). Environment enrichment was provided in the form of bocce balls. Furthermore, pigs were given 5 min per pen per d of positive contact time with a human. Positive contact included scratching and interacting with the piglets using the bocce balls.
Experimental diets and design
Six corn and SBM-based diets were formulated to meet National Research Council (NRC, 1998; NRC, 2012) nutrient requirements for pigs of 5 to 15 kg of body weight (BW) (Table 1). To test our research hypotheses and simplify study diet complexity, we used only one set, rather than weekly changing three different sets, of these six test weanling pig diets throughout the entire 3-wk post-weaning study period. There are also inconsistent literature reports about the levels of lactose supplemented in the weanling pig diets, differing in the phase-1 post-weaning wk-1 diet (15 – 20%) (Mahan, 1992; Cromwell et al., 2008; Acosta et al., 2020; Zhao et al., 2021); the phase-2 post-weaning wk-2 diet (15 – 16%) (Mahan, 1992; Cromwell et al., 2008; Acosta et al., 2020; Zhao et al., 2021) and the phase-3 post-weaning wk-3 diet (0 – 10%) (Cromwell et al., 2008; Zhao et al., 2021). Thus, to focus on testing our hypothesized high lactose digestibility concept, we decided to use only one dietary lactose content at about 10% (on as-fed basis) for the six diets for the entire 3-wk post-weanling study period. Thus, dried whey powder (19.86 g/kg of diets), with an analyzed average lactose content of 54%, was included in the diet formulations to provide 10% lactose in the weanling pig diets in this study (Table 1). A negative control (NC) diet was formulated with no antibiotics and a positive control (PC) diet was formulated with 0.1% a sub-therapeutic antibiotic Lincomix-44 premix according to the standard commercial practice. The three prebiotics of retrograded cornstarch (RCS), Fibersol-2 (F-2) and inulin (IN) as well as the viscous soluble fiber oat β-glucan (β-G) with β-1,3/1,4 branching were added into the diets at a rate of 0.75% at the expense of cornstarch. Titanium oxide was included in the diets (0.30%) as a digestibility marker. The study was conducted according to a complete randomized block design with 6 treatments (diets), 3 blocks and 2 replicate pens per block. Each pen, housing 6 piglets of 3 barrows and 3 gilts, represented an experimental unit. The dietary inclusion at the level of 0.75% for the three prebiotics and the oat β-glucan in the experimental diets were to reflect the ranges of their dietary supplementation levels reported in the current literature (Mair et al., 2010; Park et al., 2018; Xing et al., 2018; Uerlings et al., 2021) in hopefully eliciting positive changes in growth performance, nutrition and and/or physiological endpoints while also being economically feasible for the industry should commercial applications of these potential gut modifiers as feed additives be attempted.
Measurements, sample collection and sample preparation
Experimental pig BW were measured upon their arrival on d 1 of the trial when the piglets were 21 d old and then again at the end of the trial, on d 21, when the piglets were 42 d old. These data were used to calculate growth rates of the weanling pigs over the duration of the trial. Feed intake was measured daily on a per pen basis by weighing back any remaining diets at 0900 and 1700 h. Feeders were checked twice per d to ensure diets were continuously available and to remove spoiled diets from the feeders. The collection trays placed below the feeders in each pen to catch spilled diets were also checked and emptied at 0900 and 1700 h daily. Wet diets removed from the feeders and collection trays were dried to an air-dry basis in drying ovens set at 65°C to allow removal of free water before weighing back to facilitate accurate calculations of diet consumption.
Diet and fecal samples were collected on d 8 and 15 of the trial for digestibility measurements. Fresh fecal samples were collected from at least two pigs per pen and homogenized. Fecal samples were freeze-dried. To create homogenized samples, samples of the pelleted diet and dried fecal samples were ground with a mortar and pestle according to Rideout et al. (2004). Aliquots of freshly collected fecal samples from d 8 and d 15 of the study were stored at -80°C for later processing and the determination of lactose content. Diet samples were stored at 4°C for further analyses.
Venous blood samples were collected by puncture of the orbital sinus on the right or left side of one animal per pen on d 12 of the trial from physically immobilized pigs (Bregendahl et al., 2004). Blood was collected into pre-chilled plastic centrifuge tubes containing heparin for the separation of plasma. After collection, the blood samples were placed on ice and transferred to the lab where they were centrifuged at 2,000 x g for 20 min with a centrifugation temperature of 4°C to separate the plasma fractions. These fractions were then removed, divided into aliquots and stored at -80°C for later analyses of plasma urea concentration.
Chemical and biochemical analyses
Dietary and fecal DM content was analyzed according to the Association of Official Agricultural Chemists (AOAC, 1993). The digestibility marker titanium oxide content in the diet and fecal samples was analyzed by following the procedures of Leone (1973) and Myers et al. (2004). Resulting absorbances in the processed titanium oxide standards, diet and fecal sample were measured at 410 nm by using an Epoch microplate reader (BioTek, Winooski, VT).
Lactose content in diet and fecal samples was analyzed by using a commercial kit (Megazyme, Wicklow, Ireland) that measures β-D-galactose after lactose was hydrolyzed with the enzyme β-galactosidase. The kit works through measuring the absorbance of sample solutions at 340 nm, which reflects NADH content. NADH is produced proportionately in response to the content of lactose in samples. Diet and fecal samples (~0.2 g) were accurately weighed out and transferred into 50 mL plastic centrifuge tubes. After thoroughly mixing with 12.5 mL of distilled and deionized water, the tubes were centrifuged at 1,000 x g for 15 min at 4°C to remove particulate substances. The supernatants were transferred into 50 mL of volumetric flasks and brought to the volume with distilled and deionized water. The prepared aliquot samples (0.400 mL) were deproteinized via incubation of the sample tubes in a boiling water bath, followed by centrifugation at 1,500 x g for 15 min at 4°C. The further prepared supernatant samples were then directly measured for free β-D-galactose content as a background correction prior to further determination of lactose concentrations in the prepared supernatant samples by using the assay kit’s other enzyme and assay solutions.
Plasma urea content was determined using premade kits (Stanbio Laboratory, Boerne, TX) with the kit’s solutions that utilized the enzyme urease to hydrolyze urea to ammonia and carbon dioxide. Ammonium aminates α-ketoglutarate to glutamate while the concurrent oxidation of NADH to NAD+ is catalyzed by glutamate dehydrogenase. The Stanbio diagnostics blood urea (endpoint) reagent is formulated in such a way that the decrease in absorbance at 340 nm, resulting from the oxidation of NADH to NAD+, is directly proportional to urea concentration in the plasma samples. The prepared blood plasma supernatant samples were directly measured for free content as a background correction prior to further determination of urea concentrations in the prepared supernatant samples by using the assay kit’s other enzyme and assay solutions.
Calculations and statistical analysis
The daily whole small intestinal lactase digestive capacity (LDC), referred to as the whole-gut LDC, was predicted according to the concept of Weiss et al. (1998) and Lackeyram et al. (2010) and through using the LDC value directly measured in the Yorkshire weanling pigs by Lackeyram (2012) with the following justifications. Firstly, the mammalian and porcine gut lactase activities are at their peak levels at the birth and during early postnatal suckling and then decline rapidly to their bottom residual activity levels during the weaning transition (Manners and Stevens, 1972; Ekstrom et al., 1975) and these decreases in lactase activity are primarily regulated at the transcriptional level (Kelly et al., 1991; Spodsberg et al., 1999). Secondly, expression of the gut enterocytic lactase activity is cell differentiation-dependent (Fan et al., 2001) and its expression is primarily in the differentiated upper and middle villus enterocytes and is also controlled at the transcriptional level (Troelsen et al., 1997). Thirdly, weanling pigs used in this study and in the study by Lackeyram (2012) were the same Yorkshire pigs from the same swine herd and from the same genetic background. Lastly, previous studies shown that although diets and nutrition such as oral feeding of growth factors and feeding levels affected post-transcriptional intracellular synthesis and processing of lactase, these non-genetic factors had limited effects on lactase activity in young pigs (Kelly et al., 1991; Burrin et al., 2001; Dudley et al., 2001). Nevertheless, weanling pigs used in this study and in the study by Lackeyram (2012) also were also fed with the similar corn and SBM-based diets and were managed under the similar housing conditions. Thus, the whole-gut LDC values associated with the weanling pigs fed the experiential diets in this study was predicted according to Formula 1.
Where LDC is the whole gut lactase digestive capacity (g lactose/pig•d); 342.30 is the molar mass of lactose representing 342.30 g lactose per mole; Vcap is the small intestinal lactase digestive capacity of 151.42 ± 15.79 mmol/(kg•BW•d) previously measured by our group in the weanling Yorkshire pig (Lackeyram, 2012); and BW is the average final live BW of experimental pigs (kg/pig).
Data were analyzed by using the Statistical Analysis Software 9.2 (SAS Institute, Cary, NC) for a mixed model of analysis of variance (ANOVA) according to the completely randomized block design. The animals were sorted completely at random within each block without taking their factors into account. The statistical model for the ANOVA according to a completely randomized block design with 6 diets (treatments), 6 replications (blocks) and 24 experimental units (pens) of a total of 144 animals. Comparisons between each test diet and each of the control diets were conducted by using the Dunnett-Hsu’s test. Comparisons among all the diets were carried out by using the Tukey-Krammer’s test for pairwise comparisons. A linear calibration equation was generated by using respective pure compounds in reaction with reagents for each batch of the sample analyses of titanium oxide, lactose and urea with verified significant slope and intercept estimates at P< 0.05. Differences between and among the treatment diets were considered to be significant at P< 0.05.
Results
The effects of dietary supplementation of the three prebiotics and oat β-glucan on the major growth performance endpoints are summarized and compared in Table 2. There were no differences (P > 0.05) in the initial and the final BW and the 3-week (wk) average daily gain (ADG) of the test weanling pigs among the 6 experimental diets; as well as between each of the tested feed additive supplemental diets and the PC (Diet 2) and the NC (Diet 1) diets. Furthermore, dietary supplementation of these three prebiotics (Diet 3 - RCS; Diet 5 - F-2; and Diet 6 - IN) and oat β-glucan (Diet 4 - β-G) had no effects (P > 0.05) on the 3-wk average daily feed intake (ADFI) and the 3-wk feed to gain ratio (F:G) in the weanling pigs.
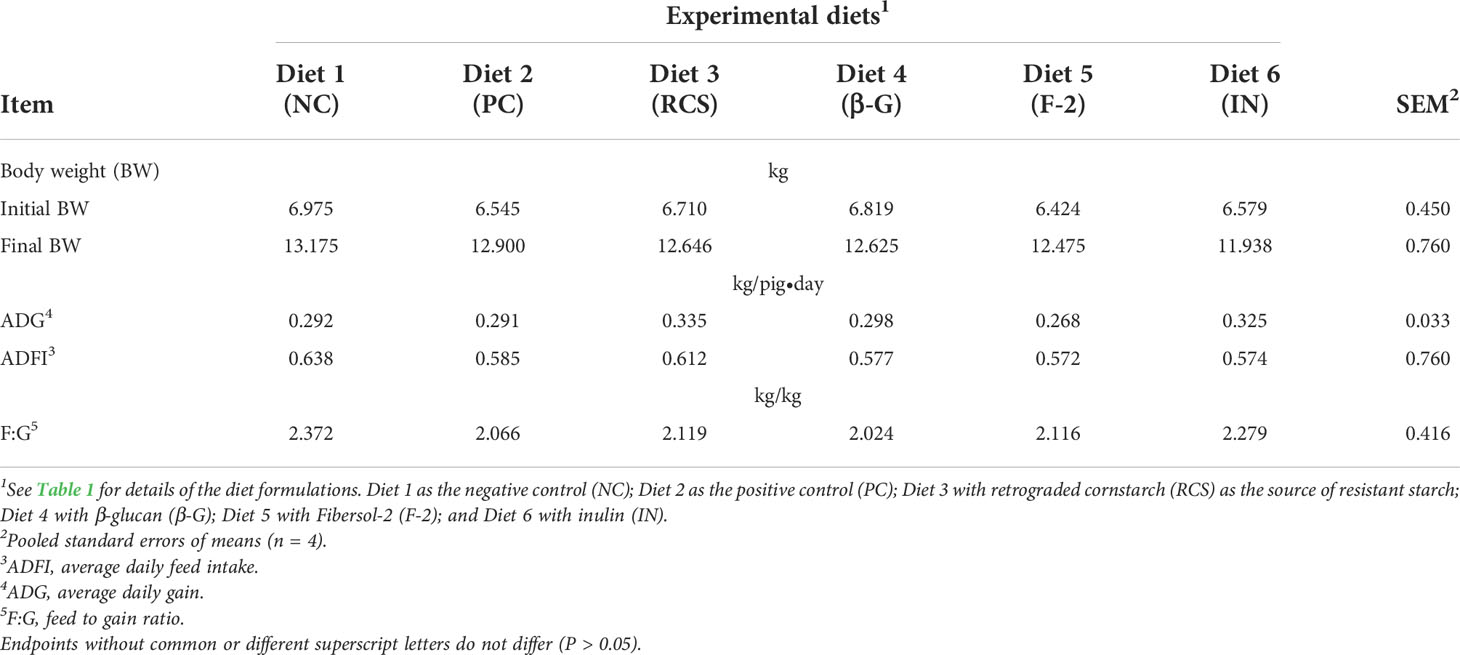
Table 2 The effects of dietary supplementation of three prebiotics and β-glucan on growth performances in the pigs weaned at 3 weeks (wk) of age and fed the corn and soybean meal-based diets for 3 wk.
The effects of dietary supplementation of these three prebiotics and oat β-glucan on the dietary and fecal DM contents, the apparent total tract DM digestibility and plasma urea concentration were summarized and compared in Table 3. Dietary supplementation (at 0.75%) of inulin (Diet 6 - IN) increased (P< 0.05) its dietary DM content; however, the fecal DM content was not affected (P > 0.05) by the dietary treatment in comparison with the NC (Diet-1) when tested by the Dunnett-Hsu’s test. β-Glucan supplementation (at 0.75%, Diet 4 - β-G) and the presence of the sub-therapeutic level of the feed antibiotic in the PC (Diet-2) resulted in numerically lower apparent total tract DM digestibility values; however, these differences were not statistically significant when compared by using the Tukey-Kramer’s test and the Dunnett-Hsu’s test. This discrepancy might have been partly due to the relatively larger SEM valued associated with these measurements. Overall, the dietary treatments did not have effects (P > 0.05) on the apparent total tract DM digestibility and the plasma urea concentration in the weanling pigs.
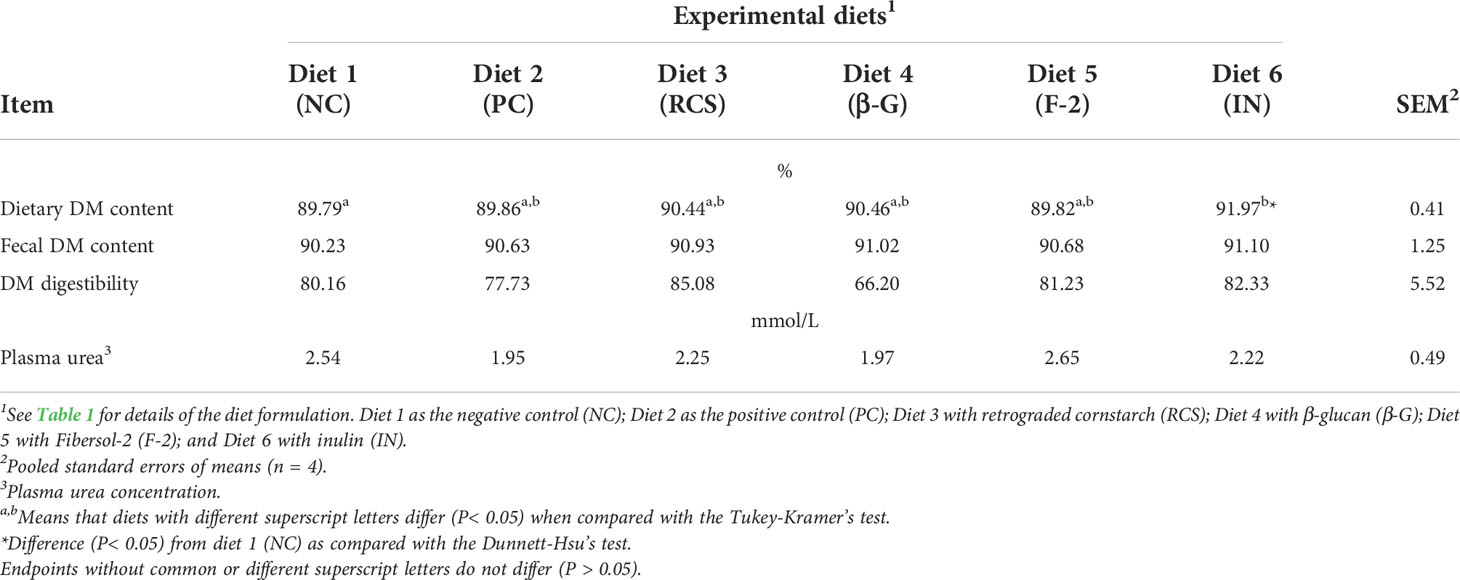
Table 3 The effects of dietary supplementation of three prebiotics and β-glucan on the apparent total tract dry matter (DM) digestibility measured during d-8 and d-15 post-weaning and plasma urea concentration determined in d-12 post-weaning in the weanling pigs fed corn and soybean meal-based diets.
The effects of dietary supplementation of these three prebiotics and oat β-glucan on the dietary and fecal lactose contents, average daily lactose intake (ADLI), the predicted whole-gut LDC and the apparent total tract lactose digestibility were summarized and compared in Table 4. There were no differences (P > 0.05) in dietary lactose contents among the diets (Table 4). Dietary lactose content was aimed to be constant at 10% (on as-fed basis) across the six test diets; however, the analyzed lactose contents varied slightly and ranged from 10.21 (Diet 4 - β-G) to 11.53% (PC - Diet 2) because of diet mixing, sampling and lactose analysis variability. Average daily lactose intake in the weanling pigs was calculated and was found to be not different (P > 0.05) among the diets. Analyses of the fecal lactose contents revealed that all dietary lactose was 100% digested. For all the test diets, fecal lactose content was essentially measured to be at 0%. Furthermore, the whole-gut LDC values in the weanling pigs at the end of the study were also predicted and were found to be not different (P > 0.05) among the diets (Table 4). The predicted whole-gut LDC was averaged at 500.40 g lactose/pig·d and was 7.9 times of the estimated average daily lactose intake (63.48 g lactose/pig·d) across the test diets fed to the weanling pigs in this study, further suggesting that the dietary lactose was completely and rapidly digested via enzymatic hydrolysis by the small intestinal lactase anchored on the brush border membrane of the epithelia within the small intestine in these weanling pigs fed the test diets.
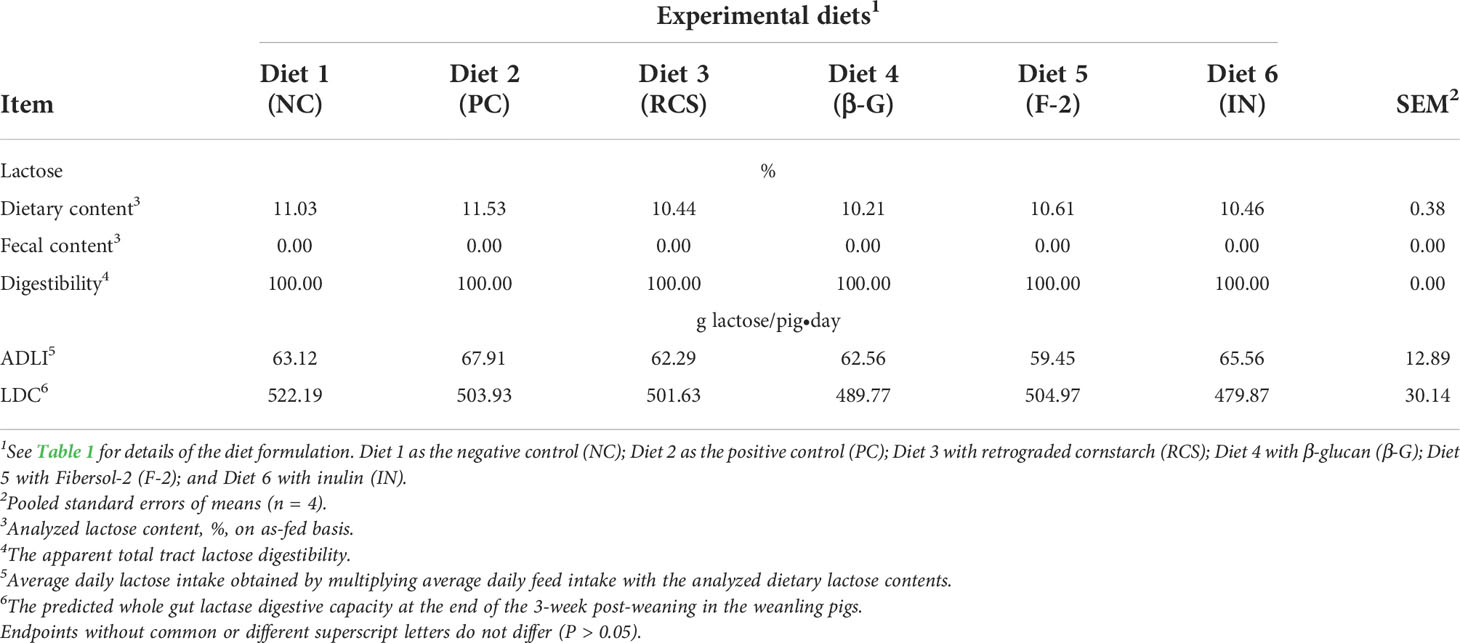
Table 4 The effects of dietary supplementation of three prebiotics and β-glucan on the apparent total tract lactose digestibility measured during d-8 and d-15 post-weaning and the whole-gut lactase digestive capacity (LDC) predicted at the end of the 3-week post-weaning in the weanling pigs fed the corn and soybean meal-based diets.
Discussion
The major objectives of this study were to evaluate the effects of RCS, Fibersol-2, inulin and oat β-glucan on growth performances and blood urea levels and to further investigate the biological mechanisms of dietary supplemental lactose for enhancing growth and whole-body N retention in weanling pig nutrition. No significant differences were detected between any of the diets for the growth performance parameters measured (Table 2). Direct comparison of results from this study with other studies is difficult due to limited research reports available on specific prebiotic and oat β-glucan supplements that look at growth performances and efficiency of nutrient utilization in the weanling pig. There is also variability in inclusion levels (i.e.,< 1% as feed additives vs. at much higher levels as functional dietary ingredients) of the prebiotics and sources of β-glucan used and research conditions such as study pen and room sanitary condition differences, which may further complicate the comparisons between this and previously reported studies.
Retrograded RS is one prebiotic that has been well reported for its effects on porcine growth and gut health endpoints (Regassa and Nyachoti, 2018; Tan et al., 2021). Unfortunately, the available research results have not delivered clear answers regarding its effects on growth performances and nutrient utilization responses in pigs. Studies looking at the growth performance of weanling pigs fed RCS at feed additive levels are scarce. De Schrijver et al. (1999) reported that pigs fed 6% RCS had a decreased ability to digest fat, however, they did not evaluate changes in growth performance. Rideout et al. (2008) reported responses in the cecal butyrate concentration and differential nutrient utilization responses in grower pigs fed diets containing different sources of RS at functional ingredients’ levels but the animals’ growth performance endpoints were not measured. Bhandari et al. (2009) reported that dietary supplementation of 7% raw potato starch enriched in RS could prevent post-weaning diarrhea but had no effects on growth performance in weanling piglets. Fouhse et al. (2015) observed dietary supplementation of gradient levels of RCS, ranging from 2.5 to 21.6% of the directly measured ileal recovered RCS, decreased post-weaning ADG and ADFI but improved the gut prebiotic microbiota at phylum levels in weanling pigs. Thus, results of this study with RCS at the intended feed additive level of 0.75% (Table 2) and the reviewed literature reports of dietary supplementation of different types of RS primarily at functional ingredients’ levels do not support improvements in growth performances in weanling pigs.
Fibersol-2 supplementation (at 0.75%) also did not reveal any improvements in growth performances in the weanling pigs in this study (Table 2). There is a scarcity of literature reports on Fibersol-2 supplementation in the weanling pigs. Rodriguez-Cabezas et al. (2010) reported the effects of Fibersol-2 supplementation on immune responses and found significant improvements in the chemically induced-colitis rat model; and unfortunately changes in growth performances were not presented and discussed in their report. Xing et al. (2018) observed the effects of dietary supplementation of gradient levels of Fibersol-2 (up to 0.40%) on improving anti-oxidative capacity and immunity responses without effects on performances in weanling pigs. Thus, results of this study and the reviewed limited literature reports of different dietary supplementation levels of Fibersol-2 conducted under various experimental conditions do not provide evidence of improved growth performances in weanling pigs fed typical commercial corn and SBM-based diets.
The results from this study showed that dietary supplementation of inulin (at 0.75%) was not sufficient to cause improvements in growth performances in the weanling pigs fed corn and SBM-based diets (Table 2). Results of this study were in agreements with the data reported by Pierce et al. (2006) who added inulin to weaning pig diets at 0.015% and observed no effects on growth performance endpoints. Furthermore, Mair et al. (2010) added inulin to weaning pig diets at 0.40% and could not find any improvements in growth performances. Uerlings et al. (2021) shown citric pulp supplemented at 0.20% was equivalent to 0.20% inulin in improving intestinal parameters in weanling pigs. Again, results of this study and the reviewed literature reports of different dietary supplementation levels of inulin as a potential gut modifier feed additive conducted under various experimental conditions do not support improvements in promoting growth performances in weanling pigs.
Dietary supplementation of oat β-glucan (at 0.75%) did not impact the growth performance parameters of the weanling pigs in this study (Table 2). This is contrary to the findings by Dritz et al. (1995) that dietary supplementation with 0.025% β-glucan did show improvements in ADG and ADFI in the weanling pigs with Streptococcus suis challenge. In agreement with our findings, later work done by Hiss and Sauerwein (2003) who found that supplementing up to 0.03% of yeast-derived β-(1-3/1-6)-glucan had a trend to increase ADFI but did not improve ADG in the weanling pigs. Hahn et al. (2006) in supplementing up to 0.04% β-glucan and Park et al. (2018) in supplementing up to 0.40% β-glucan did not report consistent improvements in performances in weanling pigs. In a recent study by Wu et al. (2021), dietary supplementation (at 0.02%) of β-glucan of bacterial origin could improve gut morphology, biochemical and microbiome endpoints with improvement in ADG but not ADFI and F:G ratio in weanling pigs. These significant growth performance responses might have also resulted from challenging housing and/or unsanitary conditions of the study, because their reported 3-wk ADG values were very low for these ages of commercial cross-bred weanling pigs, ranging from 164 to 177 g/d (Wu et al., 2021). Thus, the reason for differences between this study and those previously reported studies could have been related to the sources of β-glucans used with it ranging from barley and oat β-glucans to bacteria and yeast β-glucans. Furthermore, the differences in dietary levels of supplemented β-glucans would be a natural area for consideration. Nevertheless, the intuitive explanation that higher levels of dietary supplementation of β-glucans would be related to a greater improvement in growth performance seems to be refuted by results of these aforementioned studies.
Consideration of the prebiotic and oat β-glucan supplementations in conjunction with dietary and fecal DM content and the apparent total tract DM digestibility responses is the next area for discussions. It is interesting to observe that DM content in the inulin-supplemented diet (Diet 6) was significantly higher than that in the NC diet (Table 3). This is likely due to the fact that inulin is a good source of soluble fiber and likely holds more bound water. Whereas conventional cornstarch is known to form a semi-crystalline granular structure and likely holds less bound water because of a relatively less available hydrogen-bonding capacity. Furthermore, there is a lack of significant differences with respect to the effects of dietary supplementation of the prebiotics of RCS, Fibersol-2 and inulin and oat β-glucan on the apparent total tract DM digestibility in this study (Table 3). The test Diet-3 containing 0.75% RCS was shown to have no significant differences in the total tract DM digestibility in comparison with the NC or the PC diets. Previous porcine studies involving RCS and other sources of RS were primarily conducted with RCS and RS being included as functional ingredients (e.g., 6 – 10%) rather than as gut modifier feed additives (e.g., with dietary inclusion at< 1%), thus it would be difficult to directly compare nutrient digestibility values between this study with previously reported work (De Schrijver et al., 1999; Rideout et al., 2008; Fouhse et al., 2015). The results of no effects of dietary supplementation (at 0.75%) of Fibersol-2 on the total tract DM digestibility are consistent with the results in the study by Xing et al. (2018) with dietary supplementation (up to 0.40%) of the gradient levels of Fibersol-2 in weanling pigs. Previous studies with inulin supplemented as a gut modifier feed additive primarily focused on gut mucosal morphology and microbiota responses without reporting nutrient digestibility in weanling pigs (Pierce et al., 2006; Mair et al., 2010; Uerlings et al., 2021). Thus, it is inconclusive about the effects of dietary supplementations of RS as a gut modifier feed additive on nutrient digestibility in weanling pigs.
The numerically lower but not statistically different total tract DM digestibility (66.2%) in the Diet-4 supplemented with oat β-glucan (at 0.75%) compared with the other diets was likely due to the fact that oat β-glucan was a highly viscous fibre having negative effects on nutrient digestibility at a significant dietary content (e.g., Li et al., 1996) and the associated pooled SEM value (± 5.2%) was relatively large. Three previous studies with β-glucan supplemented as a gut modifier feed additive primarily focused on gut mucosal morphology, blood biochemical, microbiota and growth performance responses without reporting nutrient digestibility in weanling pigs (Dritz et al., 1995; Hiss and Sauerwein, 2003; Wu et al., 2021). Whereas two other studies with dietary supplementations of gradient levels of β-(1-3/1-6)-glucan (β-G) (0 - 0.40%) and yeast β-glucan (0.01 to 0.04%) shown linear responses of improvements in the total tract DM digestibility in the weanling pig (Hahn et al., 2006; Park et al., 2018). Hence, the effects of dietary supplementations of β-glucans as a gut modifier feed additive on nutrient digestibility are not consistent and are likely affected by sources, doses and test conditions of β-glucans used in the reported weanling pig studies.
The apparent total tract DM digestibility values in the diets determined in this study are within the range of values reported by Omogbenigun et al. (2004); Hahn et al. (2006) and Park et al. (2018) but lower than the values reported by Acosta et al. (2020) in weanling pigs. When fed starch-based and semi-purified diets, the apparent total tract DM digestibility values were typically measured to be at above 90% in weanling pigs (Fan et al., 2001; Fouhse et al., 2015). The total tract starch digestibility values are close to 100% in weanling pigs (Chen et al., 2021; Fan et al., 2022). Thus, dietary fibre content is likely the main limiting factor dictating the apparent total tract DM digestibility in diets of weanling pigs.
Our results also shown that dietary supplementation (at 0.75%) of the prebiotics and oat β-glucan did not have a significant effect on plasma urea concentrations in weanling pigs in this study (Table 4). Because no significant dietary effects were seen on growth performance parameters, it aligns well that no changes in the plasma urea concentrations were noted in the weanling pigs of this study. Houdijk et al. (1998) corroborated these findings in showing no effects on growth performances from the feeding of prebiotic supplements to pigs. Jaworski et al. (2017) shown high dietary fiber level led to lower final BW compared with low dietary fibre in weanling pigs. On other hand, dietary supplementation of the sub-therapeutic level of the antibiotic in the PC diet with 0.1% Lincomix-44 premix did not lead to significantly improved growth performances and the plasma urea concentration (Tables 2, 4). These responses along with the responses of the three prebiotics and the oat β-glucan might have been resulted from the fact that the sanitary conditions for conducting this study in our research facilities with weanling pigs were not challenging enough, for example, likely absence of PPE because of reasonable sanitary pen conditions under our research settings, in comparison with typical commercial swine production conditions. It has been well established that changes in plasma urea concentrations are biomarkers of gut tissue hyperplastic growth, availability of blood circulating AA to peripheral muscle growth, and whole-body status of N utilization in pigs (Coma et al., 1995; Jiang et al., 2000). Plasma urea concentrations have been further correlated with F:G ratios in weanling pigs (Whang and Easter, 2000). Thus, there was no need for us to further determine and compare the whole-body CP retention among the diets in this weanling pig study as reported in our previous study (Rideout and Fan, 2004). Overall, despite the noted positive effects of prebiotic supplements on intestinal microbiota in the literature, there is a continual deficit of data reported in showing positive responses in efficiency of the whole-body N and/or CP etention for support of growth performances in conjunction with prebiotics and/or fermentable soluble fiber feed additives in the weanling pig.
Lactose is known to have growth-promoting and/or the whole-body N and CP retention improvement effects when fed to weanling pigs (Mahan, 1992; Nessmith et al., 1997; Cromwell et al., 2008; Acosta et al., 2020; Zhao et al., 2021) and these effects have been suggested to be likely the result of prebiotic effects (Fuller, 1992; Pierce et al., 2006; Zhao et al., 2021). Contrary to this view, the series of studies by Krause et al. (1995; 1997) and more recently by Acosta et al. (2020) did not show significant evidence of the proposed prebiotic effects resulting from dietary supplementation of lactose in the weanling pigs. Despite the well-established concept that the small intestinal lactase activity decreases dramatically during the weaning transition and the post-weaning growth in the pig (Manners and Stevens, 1972; Ekstrom et al., 1975), our results have shown that dietary lactose supplemented at 10-12% was completely digested in the weanling pig (Table 4), suggesting that lactose is a completely and rapidly digestible sugar in promoting weanling pig growth and whole-body CP retention. The above statements are further substantiated by our prediction that the whole-gut LDC was about eight times of the determined average daily lactose intake (ADLI), supporting the notion that the directly measured total tract dietary lactose digestibility at 100% was primarily via enzymatic hydrolysis by the gut mucosal residual lactase activity in the weanling pigs from this study. This notion considers the very large small intestinal mucosal apical brush border surface area and the predicted exceedingly high whole-gut residual LDC capacity existed in the weanling pig. Furthermore, our results suggest that dietary supplementations of feed antibiotics, representative prebiotics and β-glucan did not affect lactose digestibility in the weanling pigs. These observations of lactose as a highly and rapidly digestible carbohydrate in the weanling pigs is consistent with the conclusion made by Lackeyram (2012) through in vitro small intestinal lactase kinetic analysis in the weanling pigs. To the best of our knowledge, this is the first in vivo study in showing that dietary supplemental lactose is completely digested at the total tract level in the weanling pig.
On the other hand, Acosta et al. (2020) shown that the dietary supplemental lactose mediated its positive effects on the whole-body N retention improvement primarily through enhancing the post-digestive rather than at the digestive level of the N utilization in weanling pigs. Fan (2013) reviewed that rapidly digestible and absorbable sugars could enhance young pig growth via two mechanisms including i) rapidly providing metabolic fuels to meet ATP demands for vital tissues such as red blood cells and brain for weanling pigs with a high metabolic rate; and 2) enhancing speed of appearance of absorbed AA in portal blood circulation. Nutrient and insulin regulate skeletal muscle protein synthesis and sensitivity of these regulations subjects to a postnatal developmental decline in young pigs (Davis et al., 2008). Diets and nutrition also have impacts on endocrine responses and their modulation of growth in weanling pigs (Carroll et al., 1998; Le Dividich and Sève, 2000). Therefore, there is a need to further investigate if lactose can up-regulate blood levels of AA and the major anabolic hormones of insulin and growth hormone and muscle protein fractional synthesis rate in weanling pigs.
It can be concluded that dietary supplementation of the prebiotics of RCS, Fibersol-2 and inulin and oat β-glucan, intended as novel gut modifier feed additives (at 0.75%), in comparison with the sub-therapeutic antibiotic Lincomix-44 was ineffective in improving the major growth performance endpoints, the whole-body N utilization status and the apparent total tract DM digestibility in the weanling pigs fed corn and SBM-based diets. Dietary supplemental lactose at 10-12% was completely digested, which was not affected by the dietary supplementations of the three tested prebiotics and the viscous soluble fiber oat β-glucan and the sub-therapeutic feed antibiotic in the weanling pigs. Therefore, the growth-promoting and the whole-body CP deposition improvement effects associated with dietary lactose supplementation are due to the fact that supplemental lactose is a completely and rapidly digestible sugar rather than acting as an effective prebiotic in the weanling pig.
Data Availability Statement
The original contributions presented in the study are included in the article/supplementary material. Further inquiries can be directed to the corresponding author.
Ethics Statement
The animal study was reviewed and approved by The Animal Care Committee at the University of Guelph.
Author Contributions
M-AH: co-designed the study and written up this manuscript with MF, conducted the animal trial with TA, QW and XY, analyzed the samples, summarized the data and written up the manuscript with MF. MF: conceptualization, resources, funding acquisition, technical and scientific advising and project administration. All contributed to the research reported in the article and approved the submitted version.
Funding
This project was jointly supported by the Natural Science and Engineering Research Council (NSERC) of Canada Discovery Program, Ontario Pork, the Agriculture Adaptation Council of Canada and the Ontario Ministry of Agriculture, Food and Rural Affairs (OMAFRA) – University of Guelph Partnership (now the OMAFRA – University of Guelph Agri-Food Innovation Alliance Program) Program. MANH was also supported by the Highly Qualified Personnel (HQP) and internship scholarship by the above partnership program.
Conflict of Interest
The authors declare that the research was conducted in the absence of any commercial or financial relationships that could be construed as a potential conflict of interest.
Publisher’s Note
All claims expressed in this article are solely those of the authors and do not necessarily represent those of their affiliated organizations, or those of the publisher, the editors and the reviewers. Any product that may be evaluated in this article, or claim that may be made by its manufacturer, is not guaranteed or endorsed by the publisher.
Acknowledgments
We also wish to thank the OMAFRA – University of Guelph Partnership sponsored Arkell Swine Station and Feed mill staff for their support in manufacturing our study diets and providing the test weanling pigs.
Abbreviations
AA, amino acids; ADFI, average daily feed intake; ADG, average daily gain; ADLI, average daily lactose intake; ANOVA, analysis of variance; AOAC, the Association of Official Agricultural Chemists; BW, body weight; CP, crude protein; CCAC, Canadian Council on Animal Care; CFIA, Canadian Food Inspection Agency; d, day; DM, dry matter; F:G, feed to gain ratio; F-2, Fibersol-2; β-G, β-glucan; IN, inulin; LDC, lactase digestive capacity; PC, positive control; PPE, porcine proliferative enteropathy; RCS, retrograded cornstarch; RS, resistant starch; SBM, soybean meal; N, nitrogen; NC, negative control; NRC, National Research Council; and Vcap, the small intestinal lactase digestive capacity; wk, week.
References
Acosta J. A., Gabler N. K., Patience J. F. (2020). The effect of lactose and a prototype lactobacillus acidophilus fermentation product on digestibility, nitrogen balance, and intestinal function of weaned pig. Transl. Anim. Sci. 4, 1–14. doi: 10.1093/tas/txaa045
AOAC (1993). Official methods of analysis. 15th (Washington, DC: Association of Official Analytical Chemists).
Arega S. M., Conraths F. J., Ameni G. (2013). Prevalence of tuberculosis in pigs slaughtered at two abattoirs in Ethiopia and molecular characterization of mycobacterium tuberculosis isolated from tuberculous-like lesions in pigs. BMC Vet. Res. 9, 97. doi: 10.1186/1746-6148-9-97
Bhandari S. K., Nyachoti C. M., Krause D. O. (2009). Raw potato starch in weaned pig diets and its influence on postweaning scours and the molecular microbial ecology of the digestive tract. J. Anim. Sci. 87, 984–993. doi: 10.2527/jas.2007-0747
Bird A. R., Vuaran M., Brown I., Topping D. L. (2007). Two high-amylose maize starches with different amounts of resistant starch vary in their effects on fermentation, tissue and digesta mass accretion, and bacterial populations in the large bowel of pigs. Br. J. Nutr. 97, 134–144. doi: 10.1017/S0007114507250433
Bregendahl K., Liu L., Cant J. P., Bayley H. S., McBride B. W., Milligan L. P., et al. (2004). Fractional protein synthesis rates measured by an intraperitoneal injection of a flooding doses of l-[ring-2H5]phenylalanine in pigs. J. Nutr. 134, 2722–2728. doi: 10.1093/jn/134.10.2722
Burrin D. G., Stoll B., Fan M. Z., Dudley M. A., Donovan S. M., Reeds P. J. (2001). Oral IGF-I alters the posttranslational processing but not the activity of lactase-phlorizin hydrolase in formula-fed neonatal pigs. J. Nutr. 131, 2235–2241. doi: 10.1093/jn/131.9.2235
Burrough E. R., Kerr B., Schweer P., Gabler N. (2022). Dietary pharmacological zinc and copper enhances voluntary feed intake of nursery pigs. Front. Anim. Sci. 3, 874284. doi: 10.3389/fanim.2022.874284
Carroll J. A., Veum T. L., Matteri R. L. (1998). Endocrine responses to weaning and changes in post-weaning diet in the young pig. Domest. Anim. Endocrinol. 15, 183–194. doi: 10.1016/s0739-7240(98)00006-x
CCAC (1993). Guide to the care and use of experimental animals. Eds. Olfert E. D., Cross B. M., McWilliam A. A.. (Ottawa, ON: CCAC).
Chang Y. J., Lee S., Yoo M. A., Lee H. G. (2006). Structural and biological characterization of sulfated-derivatized oat beta-glucan. J. Agric. Food. Chem. 54, 3815–3818. doi: 10.1021/jf060243w
Chen J., Wang M., Archbold T. L., Wang W., Fan W., Yin X., et al. (2021). Starch and dry matter digestibility values are not associated with improved growth and feed efficiency in weanling pigs fed a therapeutic multi-antimicrobial-supplemented diet. J. Anim. Sci. 99 (Suppl. 3), 285. doi: 10.1093/jas/skab235.153 (abstract
Coma J., Zimmerman D. R., Carrion D. (1995). Interactive effects of feed intake and stage of growth on the lysine requirement of pigs. J. Anim. Sci. 73, 3369–3375. doi: 10.2527/1995.73113369x
Costa D., Iraola G. (2019). Pathogenomics of emerging campylobacter species. Clin. Microbiol. Rev. 32, e00072–e00018. doi: 10.1128/CMR.00072-18
Cromwell G. L. (2001). “Antimicrobial and promicrobial agents,” in Swine nutrition, 2nd. Eds. Lewis A. J., Southern L. L. (Boca Raton, FL: CRC Press LLC), 401–426.
Cromwell G. L., Allee G. L., Mahan D. C. (2008). Assessment of lactose level in the mid- to late-nursery phase on performance of weanling pigs. J. Anim. Sci. 86, 127–133. doi: 10.2527/jas.2006-831
Davis T. A., Suryawan A., Orellana R. A., Nguyen H. V., Fiorotto M. L. (2008). Postnatal ontogeny of skeletal muscle protein synthesis in pigs. J. Anim. Sci. 86, E13–E18. doi: 10.2527/jas.2007-0419
De Schrijver R., Vanhoof K., Vande Ginste J. (1999). Nutrient utilization in rats and pigs fed enzyme resistant starch. Nutr. Res. 19, 1349–1361. doi: 10.1016/S0271-5317(99)00092-5
Dritz S. S., Shi J., Kielian T. L., Goodband R. D., Nelssen J. L., Tokach M. D., et al. (1995). Influence of dietary beta-glucan on growth performance, nonspecific immunity, and resistance to Streptococcus suis infection in weanling pigs. J. Anim. Sci. 73, 3341–3350. doi: 10.2527/1995.73113341x
Dudley M. A., Schoknecht P. A., Dudley A. W., Jiang L., Ferraris R. P., Rosenberger J. N., et al. (2001). Lactase synthesis is pretranslationally regulated in protein-deficient pigs fed a protein-sufficient diet. Am. J. Physiol. 280, G621–G628. doi: 10.1152/ajpgi.2001.280.4.G621
Ekstrom K. E., Benevenga N. J., Grummer R. H. (1975). Changes in the intestinal lactase activity in the small intestine of two breeds of swine from birth to 6 weeks of age. J. Nutr. 105, 1032–1038. doi: 10.1093/jn/105.8.1032
Evangelopoulou G., Kritas S., Christodoulopoulos G., Burriel A. R. (2015). The commercial impact of pig salmonella spp. infections in border-free markets during an economic recession. Vet. World 8, 257–272. doi: 10.14202/vetworld.2015.257-272
Fan M. Z. (2013). “Chapter 16. swine nutrition and environment,” in Sustainable swine nutrition. Ed. Chiba L. I. (Hoboken, NJ: John Wiley & Sons, Inc), 365–411.
Fan M. Z., Archbold T. (2015). Novel and disruptive biological strategies for resolving gut health challenges in monogastric food animal production. Anim. Nutr. 1, 138–143. doi: 10.1016/j.aninu.2015.10.002
Fan M. Z., Kerr B., Trabue S., Yin X., Yang Z., Wang W. (2022). “Chapter 20. swine nutrition and environment,” in Sustainable swine nutrition, 2nd. Ed. Chiba L. I. (Hoboken, NJ: John Wiley & Sons, Inc).
Fan M. Z., Stoll B., Burrin D. G., Jiang R. (2001). Enterocyte digestive enzyme activities along the crypt-villus and the longitudinal axis in neonatal pigs. J. Anim. Sci. 79, 371–381. doi: 10.2527/2001.792371x
Fastinger N. D., Karr-Lilienthal L. K., Spears J. K., Swanson K. S., Zinn K. E., Nava G. M., et al. (2008). A novel resistant maltodextrin alters gastrointestinal tolerance factors, fecal characteristics, and fecal microbiota in healthy adult humans. J. Am. Coll. Nutr. 27, 356–366. doi: 10.1080/07315724.2008.10719712
Fornefett J., Busch A., Döpping S., Hotzel H., Rimek D. (2021). Bacterial gastroenteritis caused by the putative zoonotic pathogen campylobacter lanienae: First reported case in Germany. Access Microbiol. 3, 199. doi: 10.1099/acmi.0.000199
Fouhse J. M., Gänzle M. G., Regmi P. R., van Kempen T. A., Zijlstra R. T. (2015). High amylose starch with low in vitro digestibility stimulates hindgut fermentation and has a bifidogenic effect in weaned pigs. J. Nutr. 145, 2464–2470. doi: 10.3945/jn.115.214353
Gibson G. R., Probert H. M., Loo J. V., Rastall R. A., Roberfroid M. B. (2004). Dietary modulation of the human colonic microbiota: updating the concept of prebiotics. Nutr. Res. Rev. 17, 259–275. doi: 10.1079/NRR200479
Hahn T. W., Lohakare J. D., Lee S. L., Moon W. K., Chae B. J. (2006). Effects of supplementation of beta-glucans on growth performance, nutrient digestibility, and immunity in weanling pigs. J. Anim. Sci. 84, 1422–1428. doi: 10.2527/2006.8461422x
Health Canada and CFIA (Canadian Food Inspection Agency) (2019). Guidance document on classification of veterinary drugs and livestock feeds (Ottawa, Canada).
Heo J. M., Opapeju F. O., Pluske J. R., Kim J. C., Hampson D. J., Nyachoti C. M. (2013). Gastrointestinal health and function in weaned pigs: a review of feeding strategies to control post-weaning diarrhea of without using in-feed antimicrobial compounds. J. Anim. Physiol. Anim. Nutr. (Berl) 97, 207–237. doi: 10.1111/j.1439-0396.2012.01284.x
Hiss S., Sauerwein H. (2003). Influence of dietary ss-glucan on growth performance, lymphocyte proliferation, specific immune response and haptoglobin plasma concentrations in pigs. J. Anim. Physiol. Anim. Nutr. (Berl) 87, 2–11. doi: 10.1046/j.1439-0396.2003.00376.x
Hooda S., Matte J. J., Vasanthan T., Zijlstra R. T. (2010). Dietary oat beta-glucan reduces peak net glucose flux and insulin production and modulates plasma incretin in portal-vein catheterized grower pigs. J. Nutr. 140, 1564–1569. doi: 10.3945/jn.110.122721
Hooda S., Metzler-Zebeli B., Vasanthan T., Zijlstra R. (2011). Effects of viscosity and fermentability of dietary fibre on nutrient digestibility and digesta characteristics in ileal-cannulated grower pigs. Br. J. Nutr. 106, 664–674. doi: 10.1017/S0007114511000985
Houdijk J. G. M., Bosch M. W., Verstegen M. W. A., Berenpas H. J. (1998). Effects of dietary oligosaccharides on the growth performance and faecal characteristics of young growing pigs. Anim. Feed Sci. Technol. 71, 35–48. doi: 10.1016/S0377-8401(97)00138-7
Jansen T., Weersink A., von Massow M., Poljak Z. (2019). Assessing the value of antibiotics on farms: modeling the impact of antibiotics and vaccines for managing Lawsonia intracellularis in hog production. Front. Vet. Sci. 6. doi: 10.3389/fvets.2019.00364
Jaworski N. W., Owusu-Asiedu A., Walsh M. C., McCann J. C., Loor J. J., Stein H. H. (2017). Effects of a 3 strain -based direct-fed microbial and dietary fiber concentration on growth performance and expression of genes related to absorption and metabolism of volatile fatty acids in weanling pigs. J. Anim. Sci. 95, 308–319. doi: 10.2527/jas.2016.0557
Jha R., Fouhse J. M., Tiwari U. P., Li L., Willing B. P. (2019). Dietary fiber and intestinal health of monogastric animals. Front. Vet. Sci. 6. doi: 10.3389/fvets.2019.00048
Jiang R., Chang X., Stoll B., Fan M. Z., Arthington J., Weaver E., et al. (2000). Dietary plasma protein reduces small intestinal growth and lamina propria cell density in early weaned pigs. J. Nutr. 130, 21–26. doi: 10.1093/jn/130.1.21
Karuppannan A. K., Opriessnig T. (2018). Lawsonia intracellularis: Revisiting the disease ecology and control of this fastidious pathogen in pigs. Front. Vet. Sci. 5. doi: 10.3389/fvets.2018.00181
Kelly D., King T. P., McFadyen M., Travis A. J. (1991). Effect of lactation on the decline of brush border lactase activity in neonatal pigs. Gut 32, 386–392. doi: 10.1136/gut.32.4.386
Krause D. O., Easter R. A., White B. A., Mackie R. I. (1995). Effect of weaning diet on the ecology of adherent lactobacilli in the gastrointestinal tract of the pig. J. Anim. Sci. 73, 2347–2354. doi: 10.2527/1995.7382347x
Krause D. O., White B. A., Mackie R. I. (1997). Ribotyping of adherent lactobacillus from weaning pigs: a basis for probiotic selection based on diet and gut compartment. Anaerobe 3, 317–325. doi: 10.1006/anae.1997.0118
Lackeyram D. A. (2012). Expression of the small intestinal apical membrane hydrolases in the early-weaned piglet. Ph.D. dissertation (ON, Canada: University of Guelph).
Lackeyram D., Yang C., Archbold T., Swanson K. C., Fan M. Z. (2010). Early weaning reduces small intestinal alkaline phosphatase expression in pigs. J. Nutr. 140, 461–468. doi: 10.3945/jn.109.117267
Le Dividich J., Sève B. (2000). Effects of underfeeding during the weaning period on growth, metabolism, and hormonal adjustments in the piglet. Domest. Anim. Endocrinol. 19, 63–74. doi: 10.1016/s0739-7240(00)00067-9
Leone J. L. (1973). Collaborative study of the quantitative determination of titanium dioxide in cheese. J. AOAC. 56, 535–537. doi: 10.1093/jaoac/56.3.535
Lia A., Hallmans G., Sandberg A. S., Sundberg B., Aman P., Andersson H. (1995). Oat beta-glucan increases bile acid excretion and a fiber-rich barley fraction increases cholesterol excretion in ileostomy subjects. Am. J. Clin. Nutr. 62, 1245–1251. doi: 10.1093/ajcn/62.6.1245
Li S., Sauer W. C., Huang S. X., Gabert V. M. (1996). Effect of beta-glucanase supplementation to hulless barley- or wheat-soybean meal diets on the digestibilities of energy, protein, beta-glucans, and amino acids in young pigs. J. Anim. Sci. 74, 1649–1656. doi: 10.2527/1996.7471649x
Mahan D. C. (1992). Efficacy of dried whey and its lactalbumin and lactose components at two dietary lysine levels on postweaning pig performance and nitrogen balance. J. Anim. Sci. 70, 2182–2187. doi: 10.2527/1992.7072182x
Mahan D. C., Newton E. A. (1993). Evaluation of feed grains with dried skim milk and added carbohydrate sources on weanling pig performance. J. Anim. Sci. 71, 3376–3382. doi: 10.2527/1993.71123376x
Mair C., Plitzner C., Domig K. J., Schedle K., Windisch W. (2010). Impact of inulin and a multispecies probiotic formulation on performance, microbial ecology and concomitant fermentation patterns in newly weaned piglets. J. Anim. Physiol. Anim. Nutr. (Berl) 94, e164–e177. doi: 10.1111/j.1439-0396.2010.01000.x
Ma T., McAllister T. A., Guan L. L. (2021). A review of the resistome within the digestive tract of livestock. J. Anim. Sci. Biotechnol. 12, 121. doi: 10.1186/s40104-021-00643-6
Manners M. J., Stevens J. A. (1972). Changes from birth to maturity in the pattern of distribution of lactase and sucrase activity in the mucosa of the small intestine of pigs. Br. J. Nutr. 28, 113–127. doi: 10.1079/bjn19720014
Myers W. D., Ludden P. A., Nayigihugu V., Hess B. W. (2004). Technical note: A procedure for the preparation and quantitative analysis of samples for titanium dioxide. J. Anim. Sci. 82, 179–183. doi: 10.2527/2004.821179x
Nagy B., Fekete P. Z. (2005). Enterotoxigenic escherichia coli in veterinary medicine. Int. J. Med. Microbiol. 295, 443–454. doi: 10.1016/j.ijmm.2005.07.003
Nessmith W. B., Nelssen J. L., Tokach M. D., Goodband R. D., Bergstrom J. R. (1997). Effects of substituting deproteinized whey and(or) crystalline lactose for dried whey on weanling pig performance. J. Anim. Sci. 75, 3222–3228. doi: 10.2527/1997.75123222x
Niroula N., Lim Z. L., Walker S., Huang Y., Gerdts V., Zriba S., et al. (2022). Domestic pigs experimentally infected with Mycobacterium bovis and Mycobacterium tuberculosis exhibit different disease outcomes. Tuberculosis (Edinb) 133, 102167. doi: 10.1016/j.tube.2022.102167
Omogbenigun F. O., Nyachoti C. M., Slominski B. A. (2004). Dietary supplementation with multienzyme preparations improved nutrient utilization and growth performance in weaned pigs. J. Anim. Sci. 82, 1053–1061. doi: 10.2527/2004.8241053x
Park J. H., Lee S. I., Kim I. H. (2018). Effect of dietary β-glucan supplementation on growth performance, nutrient digestibility, and characteristics of feces in weaned pigs. J. Anim. Sci. Technol. 46, 1193–1197. doi: 10.1080/09712119.2018.1481855
Pierce K. M., Callan J. J., McCarthy P., O’Doherty J. V. (2005). Performance of weanling pigs offered low or high lactose diets supplemented with avilamycin or inulin. Anim. Sci. 80, 313–318. doi: 10.1079/ASC40900313
Pierce K. M., Sweeney T., Brophy P. O., Callan J. J., Fitzpatrick E., McCarthy P., et al. (2006). The effect of lactose and inulin on intestinal morphology, selected microbial populations and volatile fatty acid concentration in the gastrointestinal tract of the weanling pig. Anim. Sci. 82, 311–318. doi: 10.1079/ASC200634
Pluske J. R., Pethick D. W., Hopwood D. E., Hampson D. J. (2002). Nutritional influences on some major enteric bacterial diseases of pig. Nutr. Res. Rev. 15, 333–371. doi: 10.1079/NRR200242
Ramos B., Pereira A. C., Reis A. C., Cunha M. V. (2020). Estimates of the global and continental burden of animal tuberculosis in key livestock species worldwide: A meta-analysis study. One Health 10, 100169. doi: 10.1016/j.onehlt.2020.100169
Rath A., Rautenschlein S., Rzeznitzeck J., Breves G., Hewicker-Trautwein M., Waldmann K. H., et al. (2021). Impact of Campylobacter spp. on the integrity of the porcine gut. Anim. (Basel) 11, 2742. doi: 10.3390/ani11092742
Regassa A., Nyachoti C. M. (2018). Application of resistant starch in swine and poultry diets with particular reference to gut health and function. Anim. Nutr. 4, 305–310. doi: 10.1016/j.aninu.2018.04.001
Rhouma M., Fairbrother J. M., Beaudry F., Letellier A. (2017). Post weaning diarrhea in pigs: risk factors and non-colistin-based control strategies. Acta Vet. Scand. 59 (31). doi: 10.1186/s13028-017-0299-7
Rideout T. C., Fan M. Z. (2004). Nutrient utilization in responses to chicory inulin supplementation in studies with pigs. J. Sci. Food Agric. 84, 1005–1012. doi: 10.1002/jsfa.1751
Rideout T. C., Fan M. Z., Cant J. P., Wagner-Riddle C., Stonehouse P. (2004). Excretion of major odor-causing and acidifying compounds in response to dietary supplementation of chicory inulin in growing pigs. J. Anim. Sci. 82, 1678–1684. doi: 10.2527/2004.8261678x
Rideout T. C., Liu Q., Wood P., Fan M. Z. (2008). Nutrient utilisation and intestinal fermentation are differentially affected by the consumption of resistant starch varieties and conventional fibres in pigs. Br. J. Nutr. 99, 984–992. doi: 10.1017/S0007114507853396
Rodriguez-Cabezas M. E., Camuesco D., Arribas B., Garrido-Mesa N., Comalada M., Bailon E., et al. (2010). The combination of fructooligosaccharides and resistant starch shows prebiotic additive effects in rats. Clin. Nutr. 29, 832–839. doi: 10.1016/j.clnu.2010.05.005
Schweitzer N., Damjanova I., Kaszanyitzky E., Ursu K., Samu P., Tóth A. G., et al. (2011). Molecular characterization of campylobacter lanienae strains isolated from food-producing animals. Foodborne Pathog. Dis. 8, 615–621. doi: 10.1089/fpd.2010.0754
Seifert S., Watzl B. (2007). Inulin and oligofructose: review of experimental data on immune modulation. J. Nutr. 137, 2563S–2567S. doi: 10.1093/jn/137.11.2563S
Silva J., Leite D., Fernandes M., Mena C., Gibbs P. A., Teixeira P. (2011). Campylobacter spp. as a foodborne pathogen: A review. Silva J, leite d, fernandes m, mena c, Gibbs PA, teixeira p. campylobacter spp. as a foodborne pathogen: A review. Front. Microbiol. 2. doi: 10.3389/fmicb.2011.00200
Smith D. G. E., Lawson G. H. K. (2001). Lawsonia intracellularis: getting inside the pathogenesis of proliferative enteropathy. Vet. Microbiol. 82, 331–345. doi: 10.1016/S0378-1135(01)00397-2
Spodsberg N., Troelsen J. T., Carlsson P., Enerbäck S., Sjöström H., Norén O. (1999). Transcriptional regulation of pig lactase-phlorizin hydrolase: involvement of HNF-1 and FREACs. Gastroenterology 116, 842–854. doi: 10.1016/s0016-5085(99)70067-3
Tan F. P. Y., Beltranena E., Zijlstra R. T. (2021). Resistant starch: Implications of dietary inclusion on gut health and growth in pigs: a review. J. Anim. Sci. Biotechnol. 12 (124). doi: 10.1186/s40104-021-00644-5
Tiwari U. P., Singh A. K., Jha R. (2019). Fermentation characteristics of resistant starch, arabinoxylan, and β-glucan and their effects on the gut microbial ecology of pigs: A review. Anim. Nutr. 5, 217–226. doi: 10.1016/j.aninu.2019.04.003
Tran T., Everaert N., Bindelle J. (2018). Review on the effects of potential prebiotics on controlling intestinal enteropathogens salmonella and escherichia coli in pig production. J. Anim. Physiol. Anim. Nutr. (Berl) 102, 17–32. doi: 10.1111/jpn.12666
Troelsen J. T., Mitchelmore C., Spodsberg N., Jensen A. M., Norén O., Sjöström H. (1997). Regulation of lactase-phlorizin hydrolase gene expression by the caudal-related homoeodomain protein cdx-2. Biochem. J. 322, 833–838. doi: 10.1042/bj3220833
Uerlings J., Arévalo Sureda E., Schroyen M., Kroeske K., Tanghe S., De Vos M., et al. (2021). Impact of citrus pulp or inulin on intestinal microbiota and metabolites, barrier, and immune function of weaned piglets. Front. Nutr. 8. doi: 10.3389/fnut.2021.650211
Wang W., Hu H., Zijlstra R. T., Zheng J., Gänzle M. G. (2019). Metagenomic reconstructions of gut microbial metabolism in weanling pigs. Microbiome 7, 48. doi: 10.1186/s40168-019-0662-1
Wang Y., Xu C., Zhang R., Chen Y., Shen Y., Hu F., et al. (2020). Changes in colistin resistance and mcr-1 abundance in escherichia coli of animal and human origins following the ban of colistin-positive additives in China: an epidemiological comparative study. Lancet Infect. Dis. 20, 1161–1171. doi: 10.1016/S1473-3099(20)30149-3
Weiss S. L., Lee E. A., Diamond J. (1998). Evolutionary matches of enzyme and transporter capacities to dietary substrate loads in the intestinal brush border. Proc. Natl. Acad. Sci. U.S.A. 95, 2117–2121. doi: 10.1073/pnas.95.5.2117
Whang K. Y., Easter R. A. (2000). Blood urea nitrogen as an index of feed efficiency and lean growth potential in growing-finishing swine. Asian-Australas J. Anim. Sci. 13, 811–816. doi: 10.5713/ajas.2000.811
Willment J. A., Gordon S., Brown G. D. (2001). Characterization of the human beta-glucan receptor and its alternatively spliced isoforms. J. Biol. Chem. 276, 43818–43823. doi: 10.1074/jbc.M107715200
Winkelman N. L., Crane J. P., Elfring G. D., Kratzer D. D., Meeuwse D. M., Dame K. J., et al. (2002). Lincomycin-medicated feed for the control of porcine proliferative enteropathy (ileitis) in swine. J. Swine Health Prod. 10, 107–111.
Wu Y., Li X., Liu H., Du Y., Zhou J., Zou L., et al. (2021). A water-soluble β-glucan improves growth performance by altering gut microbiome and health in weaned pigs. Anim. Nutr. 7, 1345–1351. doi: 10.1016/j.aninu.2021.04.006
Xing H. T., Yan G. H., Liu Q., Wang Y. Y., Wang B. Z., Zhuang S. (2018). Effects of soluble corn fiber (fibersol-2) on growth performance, biochemical indexes of serum and anti-oxidative capacity in piglets. Acta Vet. Zootech. Sin. 49, 2170–2179. doi: 10.11843/j.issn.0366-6964.2018.10.013
Zaman S. A., Sarbini S. R. (2016). The potential of resistant starch as a prebiotic. Crit. Rev. Biotechnol. 36, 578–584. doi: 10.3109/07388551.2014.993590
Keywords: antibiotics, β-glucan, prebiotics, lactose, digestibility, plasma urea, weanling pigs
Citation: Hayhoe M-AN, Archbold T, Wang Q, Yang X and Fan MZ (2022) Prebiotics and β-Glucan as gut modifier feed additives in modulation of growth performance, protein utilization status and dry matter and lactose digestibility in weanling pigs. Front. Anim. Sci. 3:855846. doi: 10.3389/fanim.2022.855846
Received: 16 January 2022; Accepted: 22 June 2022;
Published: 22 July 2022.
Edited by:
Kendall C. Swanson, North Dakota State University, United StatesReviewed by:
Ashok Kumar Pattanaik, ICAR-Indian Veterinary Research Institute, IndiaHalima Sultana, University of Florida, United States
Copyright © 2022 Hayhoe, Archbold, Wang, Yang and Fan. This is an open-access article distributed under the terms of the Creative Commons Attribution License (CC BY). The use, distribution or reproduction in other forums is permitted, provided the original author(s) and the copyright owner(s) are credited and that the original publication in this journal is cited, in accordance with accepted academic practice. No use, distribution or reproduction is permitted which does not comply with these terms.
*Correspondence: Ming Z. Fan, mfan@uoguelph.ca