Puerarin inhibits inflammation and oxidative stress in female BALB/c mouse models of Graves’ disease
Highlight box
Key findings
• The study examined the potential of puerarin to mitigate Graves’ disease (GD) by inhibiting inflammatory and oxidative stress (OS).
What is known and what is new?
• Environmental factors may contribute to the development of GD, as it has been linked to OS. Puerarin is a well-established flavonoid with recognized anti-inflammatory and antioxidant properties.
• Our previous study has demonstrated a significant decrease in flavone levels among children with GD. In this study, our objective was to investigate the potential impact of puerarin on the pathogenesis of GD, and to determine whether increased consumption of puerarin could potentially prevent the onset of GD and if higher doses confer any advantages over lower doses.
What is the implication, and what should change now?
• The puerarin may have the potential to mitigate GD, but a lower dose exhibited superior protective effects compared to a higher dose.
Introduction
Graves’ disease (GD) is an autoimmune thyroid disease (AITD) and the most frequent cause of hyperthyroidism (1). A significant increase in the prevalence of GD has been observed globally, with a notably higher incidence among the Chinese population compared to Caucasians (2). Previous studies have explored the impacts of various environmental factors, such as iodine, selenium, Vitamin D (VD), smoking, drinking, pressure, infection, ionizing radiation and drugs, on the pathogenesis of GD and identified GD as a complex disorder caused by the complex interplay of genetic and environmental factors (3,4), which leads to sustained autoimmune responses and loss of immune tolerance to thyroid antigens. The thyroid stimulating hormone (TSH) receptor antibody, known as thyrotrophin receptor antibody (TRAB), binds to the TSHR site on the membrane of thyroid cells. The thyroid stimulating antibody (TSAb) continuously stimulates TSHR, leading to activation of cyclic adenosine monophosphate (cAMP) in thyroid cells. The excessive elevation of cAMP leads to an exaggerated increase in oxidative stress (OS), which promotes the excessive proliferation of thyroid cells and subsequent synthesis and secretion of thyroid hormones. Consequently, leads to hyperthyroidism and goiter (5).
Our previous research has demonstrated that levels of flavones, a well-known antioxidant substance, were significantly lower in children with GD compared to controls. Furthermore, the levels of flavones in the GD group exhibited a negative correlation with thyroid peroxidase antibody (TPOAb) levels (2). GD is characterized by increased OS, and TPOAb has been proposed as a good indicator of OS in GD (6). These findings suggest that flavonoids may play a protective role in regulating OS associated with GD. However, the relationship between GD pathogenesis and flavones has not been explored yet.
Animals and human studies have suggested a direct contribution of reactive oxygen species (ROS) to the severity of clinical manifestations of GD, and treatments with antioxidants were found to improve the clinical symptoms of GD patients (7). Therefore, it is suggested that flavone supplementation may have protective effects in GD patients.
Puerarin, the main isoflavone glycoside of Pueraria lobata, is widely cultivated in the southern region of China. It is characterized by its affordable price, easy accessibility, and notable anti-inflammatory and antioxidant properties (8). In traditional Chinese medicine, puerarin has been used to treat many diseases for thousands of years based on its anti-inflammatory and antioxidant effects. However, there are no published reports on the potential effects of puerarin on GD.
Phosphoinositol 3-kinases (PI3Ks) are a family of kinases expressed in almost all mammalian cells and play important roles in regulating OS and inflammatory reactions. Among these effector molecules, AKT is the most studied and universally activated molecule downstream of PI3Ks (9). Studies have reported that TSAb can regulate the proliferation activity of thyroid cells via the PI3K/AKT pathway (10,11). Chinese medicine for hyperthyroidism primarily modulates the proliferation and apoptosis of thyroid cells through regulation of the PI3K/AKT pathway to effectively treat GD (12-14). Furthermore, certain studies suggest that AKT can interact with heat shock protein (HSP) to exert diverse influences on cell signaling mechanisms. The previous studies have suggested that the activation of AKT is analogous to the induction of HSP, and it has been proposed that the formation of the AKT-HSP complex may confer stability to AKT and provide cellular protection against apoptosis. Cellular stress responses are represented at the molecular level by the rapid synthesis of molecular chaperones such as HSP70 and HSP90 (15). HSP has also been reported to regulate the ERK1/2 in the MEK-ERK pathway to play important roles in regulating inflammatory reactions (16).
Numerous investigations have been conducted on the treatment of puerarin in autoimmune diseases, which modulate the ERK1/2 and PI3K/AKT signaling pathways (17-20). Several antioxidants have also been reported to alleviate thyroid diseases via the MAPK/ERK and PI3K/AKT pathways (21). However, whether puerarin as an antioxidant sunstance can alleviate the occurrence and development of GD through the PI3K/AKT/ERK pathways remains unclear.
In this study, we aim to assess the possible protective effects of puerarin on the pathogenesis of GD and explore the underlying mechanisms. We present this article in accordance with the ARRIVE reporting checklist (available at https://tp.amegroups.com/article/view/10.21037/tp-23-370/rc).
Methods
Mouse strains, adenovirus immunization, and puerarin supplementation
A total of 57 female BALB/c mice (~5 weeks of age; Sinosai Biotechnology Co. LTD., Suzhou, China) were randomly divided into 5 groups after 1 week of adaptation (Figure 1): (I) normal mice were intramuscularly injected with phosphate buffer saline (PBS) every 3 weeks and intragastrically administered with warm sterilized water daily [normal control (NC) group, n=6]; (II) Ad-null control mice were intramuscularly injected with control adenovirus (Genechem, Shanghai, China) every 3 weeks and intragastrically administered with warm sterilized water daily [Ad-null control (AN) group, n=6]; (III) recombinant adenovirus expressing TSHR-A subunit (Ad-TSHR289, Genechem) mice were immunized with Ad-TSHR289 every 3 weeks and intragastrically administered with warm sterilized water daily [GD model (AT) group, n=15]; (IV) Ad-TSHR289 mice low-dose with puerarin were injected with Ad-TSHR289 every three weeks and intragastrically administered with low dose puerarin (400 mg/kg) (22), daily [puerarin low dose (ATPL) group, n=15]; and (V) Ad-TSHR289 mice with high-dose puerarin were immunized with Ad-TSHR289 and intragastrically administered with high dose puerarin (1,200 mg/kg) (22), daily [puerarin high dose (ATPH) group, n=15]. All animals were raised in the SPF animal house of the Clinical Research Institute of Pediatrics of Soochow University (temperature: 21–24 ℃; humidity, 45–60%; 12–12 h light/dark cycle). Experiments were performed under a project license (No. SUDA20220620A01) granted by the Ethics Committee of Soochow University, in compliance with Chinese guidelines for the care and use of animals.
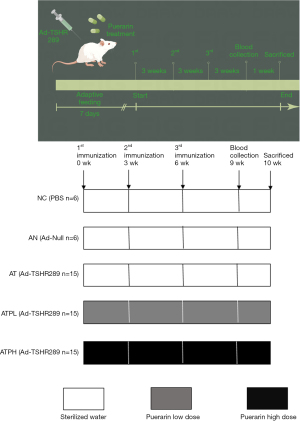
Ad-TSHR289 was used to establish the mouse model of GD (23). For each animal, Ad-TSHR289 (~107 particles) was diluted in 100 µL PBS and injected into the anterior tibialis muscle once every 3 weeks for a total of 3 times. All mice were sacrificed 4 weeks after the third immunization. The success of the test model was confirmed by TT4, FT4 and TRAB values exceeding 3 standard deviations of the AN group, along with thyroid tissue appearance and pathology meeting the standards for GD (23).
Puerarin (Xi’an Ruiying, RY20201109, purity >98%) was dissolved in warm sterilized water, and the daily gavage lasted for 10 weeks from the first immunization till sacrificing the animals.
The tests, treatments, measurements, and animal locations were all processed in a standardized sequence to effectively control for confounding factors. A protocol was prepared before the study without registration.
Measurement of serum-free thyroxine 4 (FT4), total thyroxine 4 (TT4) and TRAB
Blood samples were collected from the orbital venous plexus and placed on ice. The serum was separated within 20 minutes and stored at −80 ℃ until analysis. The levels of TT4, FT4 and TRAB were measured with ELISA kits (TT4, FT4 ELISA kits: Cusabio Biotech, Wuhan, China; TRAB ELISA kits: Assay Genie, Ireland) according to the manufacturer’s instructions.
Histological examination of the thyroid gland
The thyroid gland of each mouse was harvested, and one of the lobes was fixed in 4% paraformaldehyde until embedded in paraffin. Paraffin-embedded tissues were sectioned (5 µm thickness) and stained with hematoxylin-eosin (HE).
Flow cytometric analysis of splenocytes
The antibodies used for flow cytometric analysis include FITC anti-mouse CD3, Pacific Blue anti-mouse CD4, PerCP anti-mouse CD8a, PE/Cyanine 7 anti-mouse CD19, APC anti-mouse CD25, PE anti-mouse FoxP3 and their corresponding isotype controls. All of them were obtained from Biolegend (San Diego, CA, USA).
The mouse spleen cells were suspended in RPMI 1640 (HyClone, Logan, UT, USA) medium with 10% fetal calf serum (Hangzhou Sijiqing Biological Engineering Materials) at a final concentration of 1×107cells/mL. Splenocytes were then suspended in a flow cytometry staining buffer (Biolegend), stained with surface antibodies (anti-mouse CD3, anti-mouse CD4, anti-mouse CD8, anti-mouse CD19 and anti-mouse CD25) for 30 min, incubated with permeabilization buffer (Biolegend) at room temperature for 1 hour and stained with the intracellular antibody (anti-mouse FoxP3) according to the manufacturer’s instructions. Flow cytometric analysis was performed using a flow cytometry machine (Beckmancount, Shanghai, China).
Determination of inflammatory cytokines in mice serum
Plasma samples were collected from each group. Inflammatory factors: interleukin-6 (IL-6), interleukin-10 (IL-10) and tumor necrosis factor-α (TNF-α) were measured using commercial ELISA kits (Multisciences, Hangzhou, China) according to the manufacturer’s instructions.
Determination of OS in mice serum
The levels of superoxide dismutase (SOD), glutathione (GSH), total antioxidant capacity (TaoC), and malondialdehyde (MDA) were quantified using commercial kits (Jiancheng, Nanjing, China). The SOD activity levels were measured at a wavelength of 450 nm using the water-soluble tetrazolium (WST-1) method. The GSH activity levels were determined at a wavelength of 405 nm using the colorimetric method. The absorbance values of MDA, an indicator of lipid peroxidation, were measured at 532 nm using the thiobarbituric acid (TBA) method. The TaoC activity levels were assessed at a wavelength of 405 nm by employing the 2,2’-azino-bis(3-ethylbenzothiazoline-6-sulfonic acid) ABTS method. All OS indexes were calculated using a microplate reader (Thermo scientific, Multiskan FC, Shanghai, China).
Protein extraction from tissue homogenate
The thyroid tissues were homogenized using a radio-immunoprecipitation assay (RIPA) protein lysate (Beyotime, Shanghai, China) and the protein concentration was determined with a bicinchoninic acid (BCA) protein concentration kit (Beyotime) prior to adding SDS loading buffer. The resulting mixture was heated at 100 ℃ for 10 minutes and stored at −20 ℃ until further use.
Western blot (WB)
WB experiments were performed to detect the expressions of signal pathway proteins. The expression of GAPDH (#5174) (CST dilution, 1:2,000; Danvers, MA, USA) was used as the internal control. Briefly, proteins from thyroid tissue homogenate were subjected to gel electrophoresis on SDS-polyacrylamide gel. The separated proteins were transferred onto nitrocellulose membranes and probed with primary rabbit antibodies: anti-AKT (#4691), anti-phospho-AKT (#13038), anti-ERK (#4695), anti-phospho-ERK (#4370), anti-HSP70 (#4872) and anti-HSP90 (#4877), which were all acquired from Cell Signaling Technology (CST dilution, 1:1,000). The membranes were washed three times with TBS-0.05% Tween-20 (TBST), followed by a 2 h incubation at room temperature with HRP-conjugated goat anti-rabbit (1:5,000, CST) and visualized by enhanced chemiluminescence (Pierce, Rockford, IL, USA). The gray value of the protein bands was measured by Image J software, and the target protein gray value normalized to GAPDH.
Statistical analysis
The data were analyzed using SPSS 22.0 statistical software, while the streaming data underwent analysis with Kaluza analysis software. Graphpad Prism 5 was utilized for figure plotting purposes. Statistical significance of differences between the five groups was determined through one-way analysis of variance (ANOVA) followed by Tukey’s test. All values are presented as means ± standard error of the mean (SEM). A value of P<0.05 was considered statistically significant. All experiments were conducted in at least triplicate.
Results
Puerarin alleviated the severity of GD in mice models
We observed no significant differences in FT4, TT4, and TRAB levels between the AN and NC groups during the experiment; however, the AT group exhibited significantly higher levels than controls. Puerarin supplementation reduced FT4, TT4, and TRAB levels in both ATPH and ATPL groups with greater improvement seen in the lower dose group (Figure 2A).
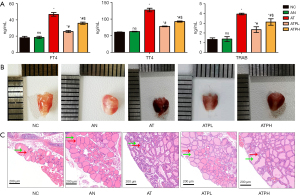
The thyroid glands in the NC and AN groups exhibited a small volume, symmetrical distribution, and a reddish coloration. In contrast, the AT group’s thyroid tissues displayed apparent hyperemia and edema, an increase in volume, and a dark red hue. Puerarin treatment resulted in a shift of thyroid tissue color from dark red to light red, accompanied by a reduction in volume increase and tissue edema, and the ATPL group were great closer to normalcy in the apperace than the ATPH group (Figure 2B).
Furthermore, the histopathological examination of thyroids in each group were observed by HE staining (scale bar =200 µm), the thyroid follicular tissue (green arrows), lymphocyte infiltration in the interstitium, and vascular proliferation (red arrows). Between the NC group and the AN group, there were no abnormal thyroid follicles, abundant glia in follicular cavity, no lymphocyte infiltration and no vascular hyperplasia. However, the AT group revealed heteromorphic thyroid follicles with intrusions into the follicular lumen, less colloid, and more lymphocyte infiltrations and vascular proliferation. Puerarin treatment reduced thyroid follicular atypia, improved gliosis in follicular cavity, and relieved lymphocyte infiltration and vascular hyperplasia, and the histopathological changes were significantly alleviated with greater improvements observed in the ATPL group than the ATPH group (Figure 2C).
Puerarin reversed the changes in lymphocyte subsets proportions in GD mice models
We used flow cytometry to measure the proportion of T cells (CD3+CD4+ and CD3+CD8+), B cells (CD3−CD19+) and Treg cells (CD4+CD25+FoxP3+). The results showed no statistical difference in the NC and AN groups, while the proportions of CD4+ and CD8+ T cells in the AT group were significantly higher than the NC group (CD4+ T cells: 27.49%±0.48% vs. 17.68%±0.70%; CD8+ T cells: 13.68%±0.28% vs. 9.21%±1.11%), while the proportion of B cells in AT group was significantly lower than the NC group (48.05%±2.09% vs. 66.24%±3.11%). The proportion of Treg cells was also significantly lower than the NC group (4.27%±0.49% vs. 7.61%±0.66%). The disorder proportion of lymphocyte subsets were alleviated after treatment with puerarin, and significant improvement was observed in the ATPL group compared with the AT group (CD4+ T cells: 21.16%±0.22% vs. 27.49%±0.48%; CD8+ T cells: 10.76%±0.78% vs. 13.68%±0.28%; B cells: 58.71%±1.19% vs. 48.05%±2.09%; Treg cells: 6.83%±0.49% vs. 4.27%±0.49%). The effects of puerarin in the ATPL group were much better than in the ATPH group (Figure 3A).
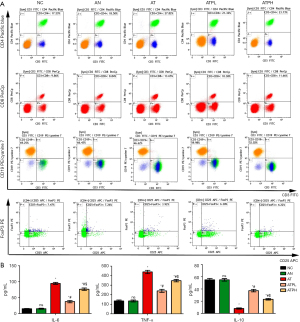
Puerarin restored the anti-inflammatory ability of mice models against GD
The levels of serum inflammatory factors (IL-6, IL-10 and TNF-α) of the NC group and the AN group were similar and have no statistical significance. The levels of pro-inflammatory factors (IL-6, TNF-α) in the AT group were significantly higher than in the NC group but decreased after adding puerarin. At the same time, the levels of anti-inflammatory factor (IL-10) in the AT group were significantly lower than the NC group, but normalized after the puerarin treatment [AT vs. ATPL: IL-6 (pg/mL): 94.73±3.09 vs. 37.67±3.8; TNF-α (pg/mL): 438.96±17.63 vs. 240.55±17.29; IL-10 (pg/mL): 8.04±1.07 vs. 37.31±2.07]. We also observed that the effects of puerarin treatment in the ATPL group were much better compared with the ATPH group (Figure 3B).
Puerarin increased the antioxidant capacity and reduce the lipid peroxidation damage in GD mice models
The OS indexes did not differ significantly between the NC and AN groups. However, levels of MDA, a marker of oxidative injury, were markedly higher in the AT group compared to controls while GSH levels were considerably lower. Puerarin treatment reduced MDA and increased GSH levels relative to the AT group. Furthermore, SOD and TaoC levels in the AT group were significantly higher than those observed in the NC group. Supplementation of puerarin has been demonstrated to increase the levels of SOD and TaoC in a feedback mechanism that balances OS damage. As such, puerarin can enhance the levels of SOD, TaoC and GSH, while reducing lipid peroxidation, thereby restoring balance to OS. Notably, the ATPL group exhibited lower oxidative injury and superior antioxidant capacity compared with ATPH group (Figure 4).
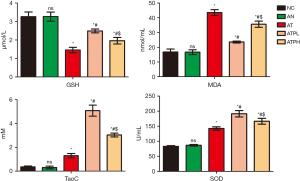
Puerarin alleviated GD progression in mice models via reducing HSP production
We observed no significant disparity in the protein expressions of HSP70 and HSP90 between the NC group and AN group in mouse thyroid tissue. However, compared to controls, the AT group exhibited markedly elevated levels of both proteins, which were subsequently reduced upon administration of puerarin. Notably, the expression levels of HSP70 and HSP90 were more effectively attenuated in the ATPL group than in ATPH group (Figure 5).
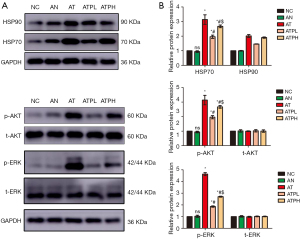
Puerarin alleviated GD progression in mice models via the PI3K/AKT/ERK pathway
We observed no significant disparity in the protein expressions of p-AKT and p-ERK between the NC group and AN group in mouse thyroid tissue. In contrast, a marked elevation was detected in the AT group compared to controls, which was effectively attenuated by puerarin administration. Moreover, the ATPL group exhibited a more significant reduction in the expression levels of proteins involved in the PI3K/AKT/ERK signaling pathway compared to the ATPH group, indicating that puerarin can effectively down-regulate active components of this pathway, inhibit thyroid cell inflammation and proliferation, but promote apoptosis, thereby exerting a protective effect on the thyroid gland (Figure 5). WB analysis was conducted in triplicate (Figure S1).
Discussion
As a bioactive flavone, puerarin is derived from Kudzu roots and has antioxidant and anti-inflammatory effects (24,25). It was shown to be beneficial in treating allergies, colitis and other autoimmune diseases (26,27). In this study, we have discovered that puerarin may alleviate the occurrence and development of GD in female BALB/C mice through its antioxidant and anti-inflammatory effects, also. In addition, the effects were superior with lower puerarin dosage (400 mg/kg/day) than with higher dosage (1,200 mg/kg/day). Additionally, the results also showed that the protective effects of puerarin against GD through its antioxidant and anti-inflammatory might via reducing the HSPs (HSP70 and HSP 90) synthesis and inhibiting the PI3K/AKT/ERK signal pathway.
In a healthy thyroid, the synthesis of thyroid hormones requires the formation of hydrogen peroxide (H2O2). There is an equilibrium between the production and consumption of ROS. However, when this balance is disturbed in favor of OS, the excessive ROS becomes toxic and increases the risk of GD (28). Studies have shown that OS not only damages peripheral tissues and contributes to the clinical manifestations of hyperthyroidism but also affects thyroid cells and worsens autoimmune responses by exposing autoantigens to the immune system. Treating GD with antithyroid drugs to restore thyroid functions can also alleviate OS. Further, antioxidants alone could also ameliorate the clinical severity of GD. However, if antioxidants and antithyroid drugs are taken together, faster and better control of GD could be achieved (29). Among the antioxidant agents, selenium was the most studied and has been proposed as a therapeutic option for Graves hyperthyroidism (GH) and Graves orbitopathy (GO) (30,31). When supplemented with selenium deficiency, it is effective, however, excess selenium intake has been reported to increase the risk of type 2 diabetes or malignancies when the body is not deficient in selenium (32). Furthermore, it would be optimal to conduct serum selenium testing prior to commencing supplementation in order to prevent potential overdose (33). Additionally, extensive research has been conducted on the efficacy of various Chinese medicines in ameliorating symptoms and signs associated with GD, as well as expediting the treatment process (34,35). Such as: Diosgenin (Dio) had control effects on goiter and hyperthyroidism in GD mice through affecting thyroid cell proliferation, but not the expression of TRAB (36). Resveratrol can alleviate the symptoms of GO by reducing the production of ROS and human heme oxygenase 1 (HO-1), as well as inhibit lipogenesis and lipid droplet accumulation (37). Celastrol suppresses the production of inflammatory cytokines, thereby attenuating inflammation and inhibiting the progression of GO (38). Astragalus alleviates symptoms in patients with GD by regulating the immune function and significantly reducing serum levels of inflammatory cytokines (39). However, puerarin, as the representative of flavonoids in this study, was selected by the initial phase to conduct ultrahigh performance liquid chromatography coupled with quadrupole time-of-flight mass spectrometer (UHPLC-QTOF/MS) and ultra-high-performance liquid chromatography coupled with multiple reaction monitoring mass spectrometry (UHPLC-MRM-MS) analyses on blood samples obtained from children with GD and healthy controls. The aim was to identify potential flavonoids that may exhibit protective effects during the progression of GD (2). Considering our geographical location in southern China, which is abundant in pueraria, our objective is to establish a solid foundation for further investigations into possibilities for preventing and treating GD.
In this study, we observed that puerarin can improve the occurrence of GD through its antioxidant and anti-inflammatory effects. While evaluation of the antioxidant defense system in patients with thyroid toxicity has been contradictory (40-43). In this study, we observed that the serum levels of MDA were significantly increased in the AT group of mice, while SOD and TaoC were elevated and GSH was decreased. However, treatment with puerarin reduced MDA levels and increased GSH levels, thereby mitigating OS damage. Additionally, the upregulation of SOD and TaoC further enhanced antioxidant capacity, ultimately restoring balance to OS and protecting against GD occurrence. This comparison supports Bednarek’s findings (41). However, further experiments are required to compare the changes in serum OS indexes of GD mice with those observed in thyroid or liver tissue. In addition, compared to selenium and other antioxidants, puerarin is more easily accessible, cost-effective, and does not require prior testing for selenium deficiency.
Previous studies indicated that pro-inflammatory cytokines are involved in the development of GD, and they might emerge as potential therapeutic targets for GD (44). Pro-inflammatory cytokines are known to cause OS by promoting the production of ROS from immune cells (45). Previous studies have demonstrated that pro-inflammatory cytokines, such as TNF-α and IL-6, may impact the risk of GD by promoting differentiation of CD4 T cells into TH17 cells while inhibiting their differentiation into Treg cells. Additionally, these cytokines can also stimulate the development of cytotoxic T-cells from CD8 T-cells. B-cell production is reduced (46,47). IL-10 was known to be a Th2 cytokine that can suppress inflammation in autoimmune diseases (48-51).
In our study, puerarin has been found to exhibit inhibitory effects on pro-inflammatory factors TNF-α and IL-6, while simultaneously increasing the levels of anti-inflammatory factor IL-10 in order to restore immune balance disrupted by ROS during GD onset. Additionally, it restores the proportion of CD4+ T cells, CD8+ T cells, CD19+ B cells and treg cells which ultimately prevents or delays the possibility of GD (52,53).
Cellular responses to stress are represented at the molecular level by the rapid synthesis of molecular chaperones such as HSP70 and HSP90 (54). During acute inflammation, extracellular HSP70 can activate immune cells and produce inflammatory cytokines to promote the removal of pathogens and subside inflammation (55). GD patients were reported to excessively express HSP (56).
In this study, we found that the levels of HSP70 and HSP90 were increased in the AT group but decreased following puerarin treatment, which suggested that puerarin could protect against the onset of GD and might regulate HSP70 and HSP90 to improve inflammation and OS.
ROS accumulation leads to the activation of the PI3K/Akt signaling pathway, which can regulate Treg cell reduction and induce GD (57). Additionally, HSP acts as a client protein by rapidly synthesizing molecular chaperones such as HSP70 and HSP90 to modulate antioxidant status, thereby regulating the ERK/Akt signaling cascade (15). Our study revealed that the AT group exhibited elevated levels of proteins in the PI3K/AKT/ERK signaling pathway, whereas puerarin treated groups demonstrated reduced levels. This suggests that puerarin may have protective effects against GD by regulating OS to modulate the PI3K/AKT/ERK pathway of the TSHR signaling pathway, thereby restoring immune balance and potentially delaying or preventing GD occurrence.
The addition of puerarin resulted in improved or delayed occurrence of GD both in the ATPL and ATPH groups compared to the AT group, with obvious statistical significance, particularly at lower dosages. These findings suggest that low dosage may better balance the body’s resilience to OS and inflammation-related damage, leading to enhanced antioxidant and anti-inflammatory benefits. While the high dose might consume antioxidant enzymes, resulting in immune dysfunction. In addition, compensatory TSH increase was observed when thyroid hormone synthesis was reduced, which could result in goiters and thyroid lesions, especially if these natural flavonoid compounds are ingested in large amounts (58). Therefore, appropriate intake of puerarin may prevent GD, but the classical toxicological principle “the dose determines toxicity” must be considered when higher doses are ingested (59). However, the optimal timing and dosage need to be explored in further experiments. In addition, due to the limited serum volume and extensive range of detection items, this experiment utilized FT4, TT4 and TRAB as representative indicators for evaluating GD diagnosis. TSH values were not included in the analysis. The conclusion of this study can be further validated by incorporating TSH detection in a subsequent experiment. Additionally, we aim to conduct further investigations on the ROS inhibitors or PI3K/AKT/ERK pathway agonists for rescue experiments, thereby providing additional evidence regarding the involvement of OS and the PI3K/AKT/ERK pathway in puerarin-mediated protection against GD. To establish a theoretical foundation for the potential standalone or combined utilization of this treatment approach with western medicine in addressing GD in future clinical practice.
Conclusions
Puerarin may exert a protective effect on female BALB/C mice with GD by acting as an antioxidant and anti-inflammatory effects via inhibiting the HSP70 and HSP90 and inhibiting the PI3K/AKT/ERK signaling pathway.
Acknowledgments
Funding: This work was supported by
Footnote
Reporting Checklist: The authors have completed the ARRIVE reporting checklist. Available at https://tp.amegroups.com/article/view/10.21037/tp-23-370/rc
Data Sharing Statement: Available at https://tp.amegroups.com/article/view/10.21037/tp-23-370/dss
Peer Review File: Available at https://tp.amegroups.com/article/view/10.21037/tp-23-370/prf
Conflicts of Interest: All authors have completed the ICMJE uniform disclosure form (available at https://tp.amegroups.com/article/view/10.21037/tp-23-370/coif). The authors have no conflicts of interest to declare.
Ethical Statement: The authors are accountable for all aspects of the work in ensuring that questions related to the accuracy or integrity of any part of the work are appropriately investigated and resolved. Experiments were performed under a project license (No. SUDA20220620A01) granted by the Ethics Committee of Soochow University, in compliance with Chinese guidelines for the care and use of animals.
Open Access Statement: This is an Open Access article distributed in accordance with the Creative Commons Attribution-NonCommercial-NoDerivs 4.0 International License (CC BY-NC-ND 4.0), which permits the non-commercial replication and distribution of the article with the strict proviso that no changes or edits are made and the original work is properly cited (including links to both the formal publication through the relevant DOI and the license). See: https://creativecommons.org/licenses/by-nc-nd/4.0/.
References
- Subekti I, Pramono LA. Current Diagnosis and Management of Graves' Disease. Acta Med Indones 2018;50:177-82.
- Xia Q, Liu J, Xu X, et al. Identification of Novel Environmental Substances Relevant to Pediatric Graves' Disease. Front Endocrinol (Lausanne) 2021;12:691326. [Crossref] [PubMed]
- Antonelli A, Ferrari SM, Ragusa F, et al. Graves' disease: Epidemiology, genetic and environmental risk factors and viruses. Best Pract Res Clin Endocrinol Metab 2020;34:101387. [Crossref] [PubMed]
- Wan H, Zhang Y, Chen Y. Characterization of Graves' disease development after partial thyroidectomy for thyroid cancer. Transl Cancer Res 2021;10:3168-76. [Crossref] [PubMed]
- Suh JM, Song JH, Kim DW, et al. Regulation of the phosphatidylinositol 3-kinase, Akt/protein kinase B, FRAP/mammalian target of rapamycin, and ribosomal S6 kinase 1 signaling pathways by thyroid-stimulating hormone (TSH) and stimulating type TSH receptor antibodies in the thyroid gland. J Biol Chem 2003;278:21960-71. [Crossref] [PubMed]
- Zarković M. The role of oxidative stress on the pathogenesis of graves' disease. J Thyroid Res 2012;2012:302537. [Crossref] [PubMed]
- Marcocci C, Leo M, Altea MA. Oxidative stress in graves' disease. Eur Thyroid J 2012;1:80-7. [Crossref] [PubMed]
- Zhou YX, Zhang H, Peng C. Puerarin: a review of pharmacological effects. Phytother Res 2014;28:961-75. [Crossref] [PubMed]
- Zeng J, Zheng S, Chen Y, et al. Puerarin attenuates intracerebral hemorrhage-induced early brain injury possibly by PI3K/Akt signal activation-mediated suppression of NF-κB pathway. J Cell Mol Med 2021;25:7809-24. [Crossref] [PubMed]
- Feng F, Han H, Wu S, et al. Crosstalk Between Abnormal TSHR Signaling Activation and PTEN/PI3K in the Dedifferentiation of Thyroid Cancer Cells. Front Oncol 2021;11:718578. [Crossref] [PubMed]
- Suh JM, Song JH, Kim DW, et al. Regulation of the phosphatidylinositol 3-kinase, Akt/protein kinase B, FRAP/mammalian target of rapamycin, and ribosomal S6 kinase 1 signaling pathways by thyroid-stimulating hormone (TSH) and stimulating type TSH receptor antibodies in the thyroid gland. J Biol Chem 2003;278:21960-71. [Crossref] [PubMed]
- Xin J, Cheng W, Yu Y, et al. Diosgenin From Dioscorea Nipponica Rhizoma Against Graves' Disease-On Network Pharmacology and Experimental Evaluation. Front Pharmacol 2021;12:806829. [Crossref] [PubMed]
- Yang M, Lai Y, Gan D, et al. Possible molecular exploration of herbal pair Haizao-Kunbu in the treatment of Graves' disease by network pharmacology, molecular docking, and molecular dynamic analysis. Front Endocrinol (Lausanne) 2023;14:1236549. [Crossref] [PubMed]
- Zhang Y, Li X, Guo C, et al. Mechanisms of Spica Prunellae against thyroid-associated Ophthalmopathy based on network pharmacology and molecular docking. BMC Complement Med Ther 2020;20:229. [Crossref] [PubMed]
- Moniruzzaman M, Ghosal I, Das D, et al. Melatonin ameliorates H2O2-induced oxidative stress through modulation of Erk/Akt/NFkB pathway. Biol Res 2018;51:17. [Crossref] [PubMed]
- Szelenyi ER, Urso ML. Time-course analysis of injured skeletal muscle suggests a critical involvement of ERK1/2 signaling in the acute inflammatory response. Muscle Nerve 2012;45:552-61. [Crossref] [PubMed]
- Deng HF, Wang XL, Sun H, et al. Puerarin inhibits expression of tissue factor induced by oxidative low-density lipoprotein through activating the PI3K/Akt/eNOS pathway and inhibiting activation of ERK1/2 and NF-κB. Life Sci 2017;191:115-21. [Crossref] [PubMed]
- Ahmad B, Khan S, Liu Y, et al. Molecular Mechanisms of Anticancer Activities of Puerarin. Cancer Manag Res 2020;12:79-90. [Crossref] [PubMed]
- Zhao J, Cheng YY, Fan W, et al. Botanical drug puerarin coordinates with nerve growth factor in the regulation of neuronal survival and neuritogenesis via activating ERK1/2 and PI3K/Akt signaling pathways in the neurite extension process. CNS Neurosci Ther 2015;21:61-70. [Crossref] [PubMed]
- Zhao L, Wang Y, Liu J, et al. Protective Effects of Genistein and Puerarin against Chronic Alcohol-Induced Liver Injury in Mice via Antioxidant, Anti-inflammatory, and Anti-apoptotic Mechanisms. J Agric Food Chem 2016;64:7291-7. [Crossref] [PubMed]
- Su X, Shen Z, Yang Q, et al. Vitamin C kills thyroid cancer cells through ROS-dependent inhibition of MAPK/ERK and PI3K/AKT pathways via distinct mechanisms. Theranostics 2019;9:4461-73. [Crossref] [PubMed]
- Zeng X, Xi Y, Jiang W. Protective roles of flavonoids and flavonoid-rich plant extracts against urolithiasis: A review. Crit Rev Food Sci Nutr 2019;59:2125-35. [Crossref] [PubMed]
- Chen CR, Pichurin P, Chazenbalk GD, et al. Low-dose immunization with adenovirus expressing the thyroid-stimulating hormone receptor A-subunit deviates the antibody response toward that of autoantibodies in human Graves' disease. Endocrinology 2004;145:228-33. [Crossref] [PubMed]
- Wang J, Zhang T, Ma C, et al. Puerarin attenuates airway inflammation by regulation of eotaxin-3. Immunol Lett 2015;163:173-8. [Crossref] [PubMed]
- Jeon YD, Lee JH, Lee YM, et al. Puerarin inhibits inflammation and oxidative stress in dextran sulfate sodium-induced colitis mice model. Biomed Pharmacother 2020;124:109847. [Crossref] [PubMed]
- Peng JH, Cui T, Huang F, et al. Puerarin ameliorates experimental alcoholic liver injury by inhibition of endotoxin gut leakage, Kupffer cell activation, and endotoxin receptors expression. J Pharmacol Exp Ther 2013;344:646-54. [Crossref] [PubMed]
- Zhu G, Wang X, Wu S, et al. Neuroprotective effects of puerarin on 1-methyl-4-phenyl-1,2,3,6-tetrahydropyridine induced Parkinson's disease model in mice. Phytother Res 2014;28:179-86. [Crossref] [PubMed]
- Song Y, Driessens N, Costa M, et al. Roles of hydrogen peroxide in thyroid physiology and disease. J Clin Endocrinol Metab 2007;92:3764-73. [Crossref] [PubMed]
- Lanzolla G, Marcocci C, Marinò M. Oxidative Stress in Graves Disease and Graves Orbitopathy. Eur Thyroid J 2020;9:40-50. [Crossref] [PubMed]
- Vrca VB, Skreb F, Cepelak I, et al. Supplementation with antioxidants in the treatment of Graves' disease; the effect on glutathione peroxidase activity and concentration of selenium. Clin Chim Acta 2004;341:55-63. [Crossref] [PubMed]
- Drutel A, Archambeaud F, Caron P. Selenium and the thyroid gland: more good news for clinicians. Clin Endocrinol (Oxf) 2013;78:155-64. [Crossref] [PubMed]
- Stranges S, Marshall JR, Natarajan R, et al. Effects of long-term selenium supplementation on the incidence of type 2 diabetes: a randomized trial. Ann Intern Med 2007;147:217-23. [Crossref] [PubMed]
- Duntas LH. Selenium and the thyroid: a close-knit connection. J Clin Endocrinol Metab 2010;95:5180-8. [Crossref] [PubMed]
- He Q, Dong H, Gong M, et al. New Therapeutic Horizon of Graves' Hyperthyroidism: Treatment Regimens Based on Immunology and Ingredients From Traditional Chinese Medicine. Front Pharmacol 2022;13:862831. [Crossref] [PubMed]
- Hai YP, Lee ACH, Chen K, et al. Traditional Chinese medicine in thyroid-associated orbitopathy. J Endocrinol Invest 2023;46:1103-13. [Crossref] [PubMed]
- Cai H, Wang Z, Zhang HQ, et al. Diosgenin relieves goiter via the inhibition of thyrocyte proliferation in a mouse model of Graves' disease. Acta Pharmacol Sin 2014;35:65-73. [Crossref] [PubMed]
- Li H, Min J, Chen Y, et al. Polydatin attenuates orbital oxidative stress in Graves' orbitopathy through the NRF2 pathway. Chem Biol Interact 2020;315:108894. [Crossref] [PubMed]
- Gu WZ, Chen R, Brandwein S, et al. Isolation, purification, and characterization of immunosuppressive compounds from tripterygium: triptolide and tripdiolide. Int J Immunopharmacol 1995;17:351-6. [Crossref] [PubMed]
- Wu J, Liu DF, Chen Y. Effects of Radix Astragali on IL-1beta, TNF-alpha and antigen expression of peripheral blood mononuclear cells in patients with Graves disease. Zhongguo Zhong Xi Yi Jie He Za Zhi 2011;31:1487-90.
- Abalovich M, Llesuy S, Gutierrez S, et al. Peripheral parameters of oxidative stress in Graves' disease: the effects of methimazole and 131 iodine treatments. Clin Endocrinol (Oxf) 2003;59:321-7. [Crossref] [PubMed]
- Bednarek J, Wysocki H, Sowiński J. Oxidative stress peripheral parameters in Graves' disease: the effect of methimazole treatment in patients with and without infiltrative ophthalmopathy. Clin Biochem 2005;38:13-8. [Crossref] [PubMed]
- Aslan M, Cosar N, Celik H, et al. Evaluation of oxidative status in patients with hyperthyroidism. Endocrine 2011;40:285-9. [Crossref] [PubMed]
- Komosinska-Vassev K, Olczyk K, Kucharz EJ, et al. Free radical activity and antioxidant defense mechanisms in patients with hyperthyroidism due to Graves' disease during therapy. Clin Chim Acta 2000;300:107-17. [Crossref] [PubMed]
- Zhu P, Wu X, Zhou J, et al. Gene polymorphisms of pro-inflammatory cytokines may affect the risk of Graves' disease: a meta-analysis. J Endocrinol Invest 2021;44:311-9. [Crossref] [PubMed]
- El-Akabawy G, El-Sherif NM. Zeaxanthin exerts protective effects on acetic acid-induced colitis in rats via modulation of pro-inflammatory cytokines and oxidative stress. Biomed Pharmacother 2019;111:841-51. [Crossref] [PubMed]
- Tanaka T, Narazaki M, Kishimoto T. Interleukin (IL-6) Immunotherapy. Cold Spring Harb Perspect Biol 2018;10:a028456. [Crossref] [PubMed]
- Li J, Zhang H, Huang W, et al. TNF-α inhibitors with anti-oxidative stress activity from natural products. Curr Top Med Chem 2012;12:1408-21. [Crossref] [PubMed]
- Inoue N, Watanabe M, Wada M, et al. IL-10 -592A/C polymorphism is associated with severity of Hashimoto's disease. Cytokine 2013;64:370-4. [Crossref] [PubMed]
- Saraiva M, O'Garra A. The regulation of IL-10 production by immune cells. Nat Rev Immunol 2010;10:170-81. [Crossref] [PubMed]
- Ding YG, Chen G, Li Q, et al. Frequency of IL-10-producing regulatory B cells associated with disease activity in thyroid-associated orbitopathy. Int J Ophthalmol 2018;11:1458-62. [Crossref] [PubMed]
- Ji X, Wan J, Chen R, et al. Low frequency of IL-10-producing B cells and high density of ILC2s contribute to the pathological process in Graves' disease, which may be related to elevated-TRAb levels. Autoimmunity 2020;53:78-85. [Crossref] [PubMed]
- Wang Y, Fang S, Zhou H. Pathogenic role of Th17 cells in autoimmune thyroid disease and their underlying mechanisms. Best Pract Res Clin Endocrinol Metab 2023;37:101743. [Crossref] [PubMed]
- Zheng H, Xu J, Chu Y, et al. A Global Regulatory Network for Dysregulated Gene Expression and Abnormal Metabolic Signaling in Immune Cells in the Microenvironment of Graves' Disease and Hashimoto's Thyroiditis. Front Immunol 2022;13:879824. [Crossref] [PubMed]
- Huang L, Mivechi NF, Moskophidis D. Insights into regulation and function of the major stress-induced hsp70 molecular chaperone in vivo: analysis of mice with targeted gene disruption of the hsp70.1 or hsp70.3 gene. Mol Cell Biol 2001;21:8575-91. [Crossref] [PubMed]
- Calderwood SK, Mambula SS, Gray PJ Jr. Extracellular heat shock proteins in cell signaling and immunity. Ann N Y Acad Sci 2007;1113:28-39. [Crossref] [PubMed]
- Heufelder AE, Goellner JR, Wenzel BE, et al. Immunohistochemical detection and localization of a 72-kilodalton heat shock protein in autoimmune thyroid disease. J Clin Endocrinol Metab 1992;74:724-31. [Crossref] [PubMed]
- Woeller CF, Roztocil E, Hammond C, et al. TSHR Signaling Stimulates Proliferation Through PI3K/Akt and Induction of miR-146a and miR-155 in Thyroid Eye Disease Orbital Fibroblasts. Invest Ophthalmol Vis Sci 2019;60:4336-45. [Crossref] [PubMed]
- Chung HJ, Chung MJ, Houng SJ, et al. Toxicological evaluation of the isoflavone puerarin and its glycosides. Eur Food Res Technol 2009;230:145-53.
- Paunkov A, Chartoumpekis DV, Ziros PG, et al. Impact of Antioxidant Natural Compounds on the Thyroid Gland and Implication of the Keap1/Nrf2 Signaling Pathway. Curr Pharm Des 2019;25:1828-46. [Crossref] [PubMed]