Changes in serum lactate dehydrogenase levels as markers of pathological response and prognosis in colorectal liver metastases patients receiving neoadjuvant chemotherapy followed by resection
Introduction
Colorectal cancer (CRC) is the fourth most deadly cancer causing almost 900,000 deaths annually worldwide (1) and at least 50% of CRC patients develop liver metastases (2,3). However, only 15–25% of CRC patients can undergo liver resection as a potentially curative treatment (4). The clinical outcome for patients with colorectal liver metastases (CRLM) has been improved greatly due to surgical resection, leading to 5-year overall survival (OS) rates ranging 31–60%. Nevertheless, the high recurrence rate of 50–75% after liver resection remains a major problem (5,6). For CRLM patients with high-risk recurrence factors, the administration of neoadjuvant chemotherapy (NAC) can improve their survival by down-staging the disease, treating micro-metastases, and increasing the complete resection rate. As an important indicator for chemotherapy efficacy, the favorable pathological response rate to NAC was only approximately 50% (7-9). Thus, to guide the therapy management of CRLM patients, it is crucial to explore and identify predictors related to the efficacy of NAC and prognosis.
Previous studies have shown that various factors are independent prognostic factors in patients with CRLM. Kim et al. (10) found that primary tumor location had a prognostic effect in patients who underwent simultaneous liver resection for CRLM, and right-sided tumors with the KRAS-mutation yielded the worst oncological outcome. Chen et al. (11) showed that preoperative D-dimer and GGT levels were reliable biomarkers in predicting major postoperative complications or survival of CRLM patients after hepatic resection. Further, Frühling et al. (12) found that a high preoperative Glasgow Prognostic Score, measured by preoperative C-reactive protein and albumin, was correlated with poor survival in CRLM patients.
Serum lactate dehydrogenase (LDH) levels are related to tumor prognosis, and changes in LDH can be used to monitor the chemotherapeutic effects of extensive cancer. Notably, LDH assays are relatively inexpensive and easy to perform. Thus, we focused on the prognostic value of serum LDH levels. As a key enzyme of glycolysis with a wide distribution in tissues, LDH catalyzes the reversible transformation of pyruvate to lactate under anaerobic conditions (13). Glycolysis is elevated in most malignant cells and provides the largest proportion of energy needed for cell proliferation. LDH is overexpressed in hypoxic cancer cells, and research has shown a correlation between hypoxia and systemic inflammation, which is the seventh hallmark of cancer (14,15). Serum LDH levels serve as a prognostic marker of various malignancies, including lung cancer (16), CRC (17), gastric cancer (18), breast cancer (19). Changes in LDH levels have been associated with a risk of treatment failure in patients with mature B-cell non-Hodgkine’s lymphoma. However, only limited data are available on the effects of LDH and changes in LDH levels on the pathological response and outcomes of CRLM patients. Therefore, we focused on the prognostic value of changes in serum LDH levels in patients with CRLM.
To our knowledge, this study is the first to investigate the predictive role of serum LDH levels in patients with CRLM treated by liver resection followed by NAC. This study aimed to evaluate the effects of preoperative LDH serum levels, pre-NAC LDH serum levels, and variations in LDH serum levels on pathological response and prognosis of patients. We present the following article in accordance with the REMARK reporting checklist (available at https://dx.doi.org/10.21037/apm-21-584).
Methods
Patients and treatments
We reviewed the data of CRLM patients at the Cancer Hospital Chinese Academy of Medical Sciences from January 2010 to December 2018. To be eligible to participate in this study, patients had to meet the following inclusion criteria: (I) pathologically diagnosed with colorectal adenocarcinoma liver metastases; and (II) received NAC followed by liver resection. Patients were excluded from the study if they were diagnosed with other malignancies or lacked follow-up and clinical data. Ultimately, 152 patients met the above criteria at our hospital and were included in this study. The flow diagram depicting the process used to select the CRLM patients was shown in Figure 1. The study was conducted in accordance with the Declaration of Helsinki (as revised in 2013). The study was approved by the Institutional Review Board of the Ethics Committee of Cancer Hospital Chinese Academy of Medical Sciences (ID NCC2019C-016). All included patients gave their informed consent to participate in the study.
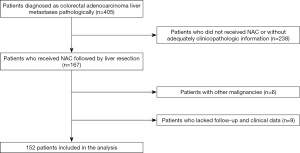
Demographic and clinicopathological data were collected from hospital records, including age, sex, body mass index (BMI), comorbidity, and American Society of Anesthesiology (ASA) score. Liver resection was defined as major or minor liver resection. NAC were administered to patients with high clinical risk-scoring system scores or initially unresectable liver metastases. The NAC regimens consisted of 5-fluorouracil/capecitabine and oxaliplatin or irinotecan with or without targeted agents, such as bevacizumab or cetuximab. Within 4-6 weeks of the completion of NAC, patients underwent liver resection. Computed tomography (CT) or magnetic resonance imaging (MRI) scans were used to evaluate NAC clinical responses of patients according to the Response Evaluation Criteria in Solid Tumors (RECIST1.1) (20). Tumor regression grade (TRG) was used to measure the pathological response (21); a pathological TRG of 1–3 represented a favorable response to NAC. We used peripheral venepuncture to collect blood samples to evaluate the serum LDH levels of patients (normal range: 120–250 U/L) 1 week before NAC and 1 week before surgery. All information was summarized in Table 1.
Table 1
Item | Pre-NAC LDH <145.0 (n=31) (%) | Pre-NAC LDH ≥145.0 (n=121) (%) | P | Preoperative LDH <231.0 (n=126) (%) | Preoperative LDH ≥231.0 (n=26) (%) | P | LDH changes =0 (n=31) (%) | LDH changes =1 (n=95) (%) | LDH changes =2 (n=26) (%) | P | All patients (n=152) (%) |
---|---|---|---|---|---|---|---|---|---|---|---|
Age ≥60 year | 9 (29.0) | 46 (38.0) | 0.353 | 43 (34.1) | 12 (46.2) | 0.245 | 9 (29.0) | 34 (35.8) | 12 (46.2) | 0.404 | 55 (36.2) |
Female | 7 (22.6) | 39 (32.2) | 0.297 | 34 (27.0) | 12 (46.2) | 0.053 | 7 (22.6) | 27 (28.4) | 12 (46.2) | 0.127 | 49 (32.2) |
BMI >24 kg/m2 | 13 (41.9) | 72 (59.5) | 0.079 | 67 (53.2) | 18 (69.2) | 0.133 | 13 (41.9) | 54 (56.8) | 18 (69.2) | 0.113 | 85 (56.0) |
Comorbidity | 14 (45.2) | 57 (47.1) | 0.846 | 60 (47.6) | 11 (42.3) | 0.621 | 14 (45.2) | 46 (48.4) | 11 (42.3) | 0.84 | 71 (46.7) |
ASA score 3–4 | 3 (9.7) | 18 (14.9) | 0.454 | 20 (15.9) | 1 (3.8) | 0.192 | 3 (9.7) | 17 (17.9) | 1 (3.8) | 0.165 | 21 (13.8) |
Preoperative CEA ≥10 ng/mL | 9 (29.0) | 56 (46.3) | 0.083 | 49 (38.9) | 16 (61.5) | 0.034 | 9 (29.0) | 40 (42.1) | 16 (61.5) | 0.046 | 65 (42.8) |
Synchronous metastasis | 30 (98.8) | 104 (86.0) | 0.096 | 114 ((09.5) | 20 (76.9) | 0.107 | 30 (96.8) | 84 (88.4) | 20 (76.9) | 0.081 | 134 (88.2) |
Primary site Colon | 13 (41.9) | 68 (56.2) | 0.156 | 70 (55.6) | 11 (42.3) | 0.218 | 13 (41.9) | 57 (60.0) | 11 (42.3) | 0.101 | 81 (53.3) |
Right hemicolon | 27 (87.1) | 107 (88.4) | 1.000 | 112 (88.9) | 22 (84.6) | 0.779 | 27 (87.1) | 85 (89.5) | 22 (84.6) | 0.721 | 134 (88.2) |
Heterochronous resection | 11 (35.5) | 34 (28.1) | 0.422 | 36 (28.6) | 9 (34.6) | 0.539 | 11 (35.5) | 25 (26.3) | 9 (34.6) | 0.517 | 45 (29.6) |
R0 resection | 17 (54.8) | 81 (66.9) | 0.209 | 79 (62.7) | 19 (73.1) | 0.314 | 17 (54.8) | 62 (65.3) | 19 (73.1) | 0.346 | 98 (64.5) |
Major resection | 15 (48.4) | 55 (54.5) | 0.540 | 64 (50.8) | 17 (65.4) | 0.175 | 15 (48.4) | 49 (51.6) | 17 (65.4) | 0.379 | 70 (46.1) |
RFA | 4 (12.9) | 25 (20.7) | 0.327 | 27 (21.4) | 2 (7.7) | 0.177 | 4 (12.9) | 23 (24.2) | 2 (7.7) | 0.126 | 29 (19.1) |
Bilobar distribution | 16 (51.6) | 58 (47.9) | 0.715 | 61 (48.4) | 13 (50.0) | 0.883 | 16 (51.6) | 45 (47.4) | 13 (50.0) | 0.909 | 74 (48.7) |
Extrahepatic metastases | 2 (6.5) | 15 (12.4) | 0.537 | 13 (10.3) | 4 (15.4) | 0.686 | 2 (6.50) | 11 (11.6) | 4 (15.4) | 0.578 | 17 (11.2) |
Diameter of metastases ≥3.0 cm | 10 (32.3) | 63 (52.1) | 0.049 | 55 (43.7) | 18 (69.2) | 0.017 | 10 (32.3) | 45 (47.4) | 18 (69.2) | 0.020 | 73 (48.0) |
Multiple metastases | 24 (77.4) | 82 (67.9) | 0.297 | 89 (70.6) | 17 (65.4) | 0.596 | 24 (77.4) | 65 (68.4) | 17 (65.4) | 0.555 | 106 (69.7) |
Operation time ≥337.7 min | 12 (38.7) | 64 (52.9) | 0.159 | 61 (48.4) | 15 (57.7) | 0.389 | 12 (38.7) | 49 (51.6) | 15 (57.7) | 0.318 | 76 (50.0) |
Blood loss ≥300 mL | 15 (48.4) | 62 (51.2) | 0.777 | 60 (47.6) | 17 (65.4) | 0.099 | 15 (48.4) | 45 (47.4) | 17 (65.4) | 0.255 | 77 (50.7) |
Blood transfusion | 1 (3.2) | 30 (24.8) | 0.008 | 23 (18.3) | 8 (30.8) | 0.149 | 1 (3.2) | 22 (23.2) | 8 (30.8) | 0.020 | 31 (20.4) |
Poorly differentiated | 7 (22.6) | 36 (29.8) | 0.429 | 37 (29.4) | 6 (23.1) | 0.517 | 7 (22.6) | 30 (31.6) | 20 (23.1) | 0.508 | 43 (28.3) |
T3–T4 | 26 (83.9) | 106 (87.6) | 0.583 | 111 (88.1) | 21 (80.8) | 0.492 | 26 (83.9) | 85 (89.5) | 21 (80.8) | 0.399 | 132 (86.8) |
Lymph node positive | 26 (83.9) | 83 (68.6) | 0.092 | 93 (73.0) | 17 (65.4) | 0.432 | 26 (83.9) | 66 (69.5) | 17 (65.4) | 0.222 | 109 (71.7) |
Oxaliplatin | 20 (64.5) | 81 (66.9) | 0.739 | 83 (65.9) | 18 (69.2) | 0.949 | 20 (64.5) | 63 (66.3) | 18 (69.2) | 0.929 | 101 (66.5) |
Irinotecan | 6 (19.4) | 17 (14.0) | 19 (15.1) | 4 (15.4) | 6 (19.4) | 13 (13.7) | 4 (15.4) | 23 (15.1) | |||
Oxaliplatin + irinotecan | 5 (16.1) | 23 (19.0) | 24 (19.0) | 4 (15.4) | 5 (16.1) | 19 (20.0) | 4 (15.4) | 28 (18.4) | |||
NAC cycles ≥5 | 13 (41.9) | 64 (52.9) | 0.276 | 64 (50.8) | 13 (50.0) | 0.941 | 13 (41.9) | 51 (53.7) | 13 (50.0) | 0.523 | 77 (50.7) |
Targeted therapy | 11 (35.5) | 39 (32.2) | 0.731 | 41 (32.5) | 9 (34.6) | 0.837 | 11 (35.5) | 30 (31.6) | 9 (34.6) | 0.903 | 50 (32.9) |
Second-line chemotherapy | 4 (12.9) | 17 (14.0) | 1.000 | 16 (12.7) | 5 (19.2) | 0.571 | 4 (12.9) | 12 (12.6) | 5 (19.2) | 0.706 | 21 (13.8) |
TRG 1–3 | 16 (53.5) | 48 (39.7) | 0.175 | 54 (43.2) | 10 (38.5) | 0.656 | 16 (53.3) | 38 (40.0) | 10 (38.5) | 0.395 | 64 (42.1) |
Clinical response | 17 (54.8) | 57 (47.1) | 0.442 | 64 (50.8) | 10 (38.5) | 0.252 | 17 (54.8) | 47 (49.5) | 10 (38.5) | 0.454 | 74 (48.7) |
Postoperative complications | 16 (51.6) | 67 (55.4) | 0.708 | 67 (53.2) | 16 (61.5) | 0.435 | 16 (51.6) | 51 (53.7) | 16 (61.5) | 0.723 | 83 (54.6) |
Postoperative major complications | 4 (12.9) | 28 (23.1) | 0.212 | 23 (18.3) | 9 (34.6) | 0.062 | 4 (12.9) | 19 (20.0) | 9 (34.6) | 0.124 | 32 (21.1) |
Postoperative chemotherapy | 21 (67.7) | 66 (54.5) | 0.185 | 71 (56.3) | 16 (61.5) | 0.626 | 21 (67.7) | 50 (52.6) | 16 (61.5) | 0.299 | 87 (57.2) |
BMI, body mass index; ASA, American Society of Anesthesiologists; CEA, carcino-embryonic antigen; RFA, radiofrequency ablation; LDH, lactate dehydrogenase; NAC, neo-adjuvant chemotherapy; TRG, tumor regression grade.
Follow-up and endpoints
Patients in the study were followed-up with regularly. Specifically, patients were followed-up with 1 month after surgery, and every 3 months thereafter. CT/MRI scans and serum carcinoembryonic antigen (CEA) measurements were routinely conducted. Progression-free survival (PFS) and OS were the primary outcomes. PFS was defined as the interval from the date of diagnosis to the date of progression or the last follow-up date. OS was defined as the interval from the date of diagnosis to the date of death or the last follow-up date. The subordinate outcome was postoperative complications, the severity of which was described according to the Clavien-Dindo classification system (22). Clavien-Dindo scores of III-V were classified as major complications.
Statistical analysis
The optimal cutoff values of pre-NAC LDH levels and preoperative LDH levels for histological response and postoperative complications were identified by the highest Youden index (sensitivity + specificity − 1). X-tile analysis was performed to investigate the optimal cutoff values of LDH for OS as the optimal cutoff values of survival. We divided the LDH levels into two groups according to the cutoff values and compared the demographic and clinicopathological data of different groups. Based on the changes in pre-NAC LDH level and preoperative LDH level, we established the LDH change scores as follows: 0 (both pre-NAC and preoperative LDH level < cut-off value), 2 (both pre-NAC and preoperative LDH level ≥ cut-off value), and 1 (other combinations). Categorical variables were analyzed by Chi-square or Fisher’s exact test and continuous variables were analyzed by the Mann-Whitney U test. Survival was calculated using the Kaplan-Meier method and compared by the log-rank test. The relationships between tumor characteristics and pathological response, postoperative major complications were detected by the multivariable logistic regression analysis. Univariate and multivariate Cox regression models were performed for the analyses of survival. Factors with P<0.10 by univariate analysis were retained in multivariate models. A two-tailed P value of less than 0.05 was considered significant. All analyses were performed using the SPSS 23.0 and GraphPad Prism 8.0.
Results
Clinicopathological characteristics
Of the 152 patients, 103 were male (67.8%) and 49 were female (32.2%). Patients had a median age of 56 years [interquartile range (IQR): 50–63 years]. Eighty-five patients (56.0%) had a BMI ≥24 kg/m2. Comorbidities were discovered in 46.7% of patients (71/152). Only 13.8% of patients (21/152) had an ASA score of 3–4. A total of 42.8% of patients (65/152) had a CEA of ≥10 ng/mL. The primary tumor site in approximately half of the patients (53.5%, 81/152) was the colon. Poorly differentiated and T3–T4 primary tumor stages were observed in 28.3% (43/152) and 86.8% (132/152) of patients, respectively. The majority of patients (88.2%, 134/152) had synchronous liver metastases. The bilobar liver metastases distribution was observed in 74 patients (48.7%). One hundred and six patients (69.7%) had multiple liver metastasis. Patients had a median of three liver metastases (IQR: 1.0–4.0) and the median liver metastasis diameter was 2.8 cm (IQR: 1.8–4.0 cm). Positive lymph nodes were detected in 109 patients (71.7%). Heterochronous resection and major liver resection were performed in 29.6% (45/152) and 46.1% (70/152) of patients, respectively. The median operation time was 337.5 (IQR: 256.3–408.0) min and the median blood loss was 300.0 mL (IQR: 150.0–400.0 mL). The percentage of intraoperative blood transfusion was 20.4% during surgery. One hundred and twenty-nine patients (84.9%) received an oxaliplatin-based regimen. Fifty patients (32.9%) and 21 (13.8%) patients received targeted therapy and second-line therapy, respectively. The median number of NAC cycles was 5 and 77 patients (50.7%) received no less than 5 NAC cycles. Eighty-seven patients (57.2%) underwent adjuvant chemotherapy after surgery. A poor clinical and pathological response was observed in 48.7% (74/152) and 42.1% (64/152) of patients, respectively (see Table 1).
Analyses of the optimal cutoff point for LDH
The mean pre-NAC and preoperative LDH levels of patients were 235.1±216.1 and 197.3±78.3 IU/L, respectively (P<0.001). The optimal cutoff values of pre-NAC LDH and pre-NAC LDH levels for major postoperative complications were 142.5 and 186.5 IU/L, respectively. A pre-NAC LDH level ≥142.5 IU/L and preoperative LDH level of ≥186.5 IU/L were observed in 130 patients (85.5%) and 71 patients (46.7%), respectively. The optimal cutoff of pre-NAC LDH and pre-NAC LDH levels for the histological response was 200.0 and 217.0 IU/L, respectively. A pre-NAC LDH level ≥200.0 IU/L was found in 58 patients (38.2%), and a preoperative LDH level of ≥217.0 IU/L was found in 37 patients (24.3%). Additionally, the optimal cutoff values of pre-NAC LDH and pre-NAC LDH levels for survival were 145.0 and 231.0 IU/L, respectively. A pre-NAC LDH level ≥145.0 and a preoperative LDH level of ≥231.0 IU/L were observed in 121 patients (79.6%) and 26 patients (17.1%), respectively.
Effect of serum LDH levels on histological response
The univariate analysis showed that a pre-NAC LDH level ≥142.5 IU/L (P=0.083) and a preoperative LDH level ≥186.5 IU/L (P=0.093) were not significantly related to pathological response. A BMI ≥24 kg/m2 (P=0.045), a primary tumor site in the right hemicolon (P=0.006), poor differentiation (P=0.023), targeted therapy (P<0.001), clinical response (P=0.003), and LDH level change (P=0.026) were significantly associated with poor pathological response. The multivariate analysis indicated that a grade 2 LDH level change (OR 0.249, 95% CI: 0.066–0.942; P=0.041) independently predicted histological response. Additionally, a primary tumor site in the right hemicolon (OR 3.761, 95% CI: 1.060–13.344; P=0.040), multiple metastases (OR 0.365, 95% CI: 0.151–0.884; P=0.026), poor differentiation (OR 0.355, 95% CI: 0.137–0.916; P=0.032), targeted therapy (OR 5.570, 95% CI: 2.347–13.219; P<0.001) and clinical response (OR 2.337, 95% CI: 1.058–5.165; P=0.036) were independent risk factors of the histological response (see Table 2).
Table 2
Factor | Univariate analysis | Multivariate analysis | ||
---|---|---|---|---|
P | P | HR (95% CI) | ||
Age ≥60 year | 0.969 | |||
Female | 0.591 | |||
BMI >24 kg/m2 | 0.045 | 0.067 | 0.467 (0.206–1.056) | |
Comorbidity | 0.308 | |||
ASA score 3–4 | 0.668 | |||
Preoperative CEA ≥10 ng/mL | 0.065 | 0.430 | 0.718 (0.315–1.634) | |
Synchronous metastasis | 0.292 | |||
Primary site Colon | 0.308 | |||
Right hemicolon | 0.006 | 0.040 | 3.761 (1.060–13.344) | |
Bilobar distribution | 0.286 | |||
Extrahepatic metastases | 0.679 | |||
Diameter of metastases ≥3.0 cm | 0.246 | |||
Multiple metastases | 0.076 | 0.026 | 0.365 (0.151–0.884) | |
Poorly differentiated | 0.023 | 0.032 | 0.355 (0.137–0.916) | |
T3–T4 | 0.087 | 0.412 | 0.608 (0.185–1.998) | |
Lymph node positive | 0.168 | |||
Oxaliplatin-based schemes | 0.732 | |||
NAC cycles ≥5 | 0.268 | |||
Targeted therapy | <0.001 | <0.001 | 5.570 (2.347–13.219) | |
Second-line chemotherapy | 0.366 | |||
Clinical response | 0.003 | 0.036 | 2.337 (1.058–5.165) | |
Pre-NAC LDH ≥142.5 | 0.083 | 0.400 | 0.632 (0.217–1.840) | |
Preoperative LDH ≥186.5 | 0.093 | 0.142 | 0.541 (0.238–1.228) | |
LDH change grade =0 | 0.026 | – | Reference | |
LDH change grade =1 | 0.076 | 0.307 (0.083–1.129) | ||
LDH change grade =2 | 0.041 | 0.249 (0.066–0.942) |
BMI, body mass index; ASA, American Society of Anesthesiologists; CEA, carcino-embryonic antigen; NAC, neo-adjuvant chemotherapy; LDH, lactate dehydrogenase.
Effect of serum LDH levels on major postoperative complications
In this study, 83 patients (54.6%) had postoperative complications, of which 32 were major, and 51 were minor. Thirty patients suffered from surgery-related complications, 37 suffered general complications, and 16 suffered from both surgery-related complications and general complications. The univariate analysis revealed that a preoperative LDH level ≥217 IU/L (P=0.004), LDH level change (P=0.016), and second-line chemotherapy (P=0.019) were significantly associated with poor pathological response. The multivariate analysis indicated that a grade 2 LDH level change (OR 3.523, 95% CI: 1.179–10.530; P=0.024) and second-line chemotherapy (OR 3.562, 95% CI: 1.214–10.949; P=0.021) were significantly associated with postoperative major complications (see Table 3).
Table 3
Factor | Univariate analysis | Multivariate analysis | ||
---|---|---|---|---|
P | P | HR (95% CI) | ||
Age ≥60 year | 0.556 | |||
Female | 0.596 | |||
BMI >24 kg/m2 | 0.119 | |||
Comorbidity | 0.983 | |||
ASA score 3–4 | 0.595 | |||
Preoperative CEA ≥10 ng/mL | 0.498 | |||
Synchronous metastasis | 0.292 | |||
Primary site Colon | 0.983 | |||
Right hemicolon | 0.662 | |||
Heterochronous resection | 0.819 | |||
R0 resection | 0.793 | |||
Major resection | 0.115 | |||
RFA | 0.337 | |||
Bilobar distribution | 0.867 | |||
Extrahepatic metastases | 0.561 | |||
Diameter of metastases ≥3.0 cm | 0.065 | |||
Multiple metastases | 0.466 | |||
Operation time ≥337.7 min | 0.426 | |||
Blood loss ≥300 mL | 0.267 | |||
Blood transfusion | 0.086 | |||
Poorly differentiated | 0.981 | |||
T3–T4 | 1.000 | |||
Lymph node positive | 0.676 | |||
Oxaliplatin-based schemes | 0.977 | |||
NAC cycles ≥5 | 0.267 | |||
Targeted therapy | 0.841 | |||
Second-line chemotherapy | 0.019 | 0.021 | 3.562 (1.214–10.949) | |
TRG 1-3 | 0.563 | |||
Clinical response | 0.154 | |||
Pre-NAC LDH ≥200 | 0.050 | |||
Preoperative LDH ≥217 | 0.004 | |||
LDH change grade =0 | 0.016 | – | Reference | |
LDH change grade =1 | 0.091 | 2.367 (0.871–6.429) | ||
LDH change grade =2 | 0.024 | 3.523 (1.179–10.530) |
BMI, body mass index; ASA, American Society of Anesthesiologists; CEA, carcino-embryonic antigen; RFA, radiofrequency ablation; LDH, lactate dehydrogenase; NAC, neo-adjuvant chemotherapy; TRG, tumor regression grade.
Effect of pre-NAC LDH level and preoperative LDH level on survival
By the end of the follow-up period, 58.6% of patients had died, and 82.2% had relapsed. The median OS was 42.5 months (95% CI: 32.0–53.0), and the median PFS was 7.9 months (95% CI: 5.6–10.2). The 1- and 3-year PFS rates were 32.2% and 18.4%, respectively. The 1-, 3- and 5-year OS rates were 91.4%, 51.1% and 30.9%, respectively.
Elevated pre-NAC LDH levels were significantly correlated with better PFS (mPFS: 8.1 months vs. 5.1 months; P=0.016; see Figure 2A); however, elevated preoperative LDH levels were not correlated with PFS (mPFS: 8.1 vs. 7.1 months; P=0.062; see Figure 2B). Elevated pre-NAC LDH levels had no significant effect on OS (mOS:36.8 vs. 35.2 months; P=0.306; see Figure 2C), and elevated preoperative LDH levels had no significant effect on OS (mOS: 57.7 vs. 35.2 months; P=0.079; see Figure 2D). LDH level changes were significantly correlated with better PFS (mPFS: 5.1 vs. 8.0 months vs. 8.1 months; P=0.020; see Figure 3A); however, LDH level changes were not correlated with OS (mOS: 35.2 vs. 34.6 vs. 57.6 months; P=0.177; see Figure 3B).
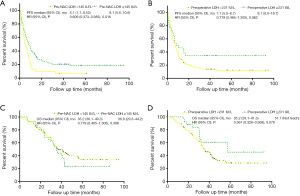
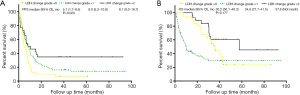
The univariate analysis revealed that a pre-NAC ≥145 IU/L (HR 0.601, 95% CI: 0.395–0.914; P=0.017), a grade 1 LDH level change (HR 0.649, 95% CI: 0.423–0.997; P=0.048), and a grade 2 LDH level change (HR 0.440, 95% CI: 0.240–0.806; P=0.008) were significantly associated with PFS. The univariate analysis also revealed R0 resection (HR 0.476, 95% CI: 0.331–0.687; P<0.001), major resection (HR 1.485, 95% CI: 1.041–2.118; P=0.029), radiofrequency ablation (RFA) (HR 1.760, 95% CI: 1.105–2.695; P=0.009), bilobar distribution (HR 1.739, 95% CI: 1.220–2.479; P=0.002), multiple metastases (HR 2.134, 95% CI: 1.415–3.219, P<0.001), lymph node positive (HR 1.795, 95% CI: 1.177–2.738; P=0.007), NAC cycles ≥5 (HR 1.454, 95% CI: 1.022–2.070; P=0.038), second-line chemotherapy (HR 1.820, 95% CI: 1.113–2.977; P=0.017), TRG (HR 0.611, 95% CI: 0.424–0.881; P=0.008), and postoperative chemotherapy (HR 0.693, 95% CI: 0.487–0.988; P=0.042) had significantly predictive effects for PFS. The multivariate analysis indicated that a pre-NAC ≥145 IU/L (HR 0.584, 95% CI: 0.359–0.950; P=0.030), a grade 1 LDH level change (HR 0.584, 95% CI: 0.359–0.950; P=0.030), and a grade 2 LDH level change (HR 0.447, 95% CI: 0.231–0.864; P=0.017) significantly predicted PFS and R0 resection (HR 0.629, 95% CI: 0.415–0.954; P=0.029), TRG (HR 0.535, 95% CI: 0.352–0.814; P=0.003) and postoperative chemotherapy (HR 0.690, 95% CI: 0.476–1.000; P=0.050) as independent predictors of PFS (see Table 4).
Table 4
Item | Univariate analysis | Multivariate analysis | |||
---|---|---|---|---|---|
P | HR (95% CI) | P | HR (95% CI) | ||
Age ≥60 year | 0.093 | 0.726 (0.499–1.055) | |||
Female | 0.953 | 0.998 (0.673–1.452) | |||
BMI >24 kg/m2 | 0.282 | 1.215 (0.852–1.731) | |||
Comorbidity | 0.921 | 1.018 (0.716–1.448) | |||
ASA score 3–4 | 0.169 | 1.412 (0.864–2.306) | |||
Preoperative CEA ≥10 ng/mL | 0.431 | 1.154 (0.808–1.647) | |||
Synchronous metastasis | 0.516 | 1.202 (0.689–2.098) | |||
Primary site Colon | 0.597 | 0.909 (0.640–1.293) | |||
Right hemicolon | 0.141 | 0.628 (0.338–1.167) | |||
Heterochronous resection | 0.540 | 1.127 (0.769–1.650) | |||
R0 resection | <0.001 | 0.476 (0.331–0.687) | 0.029 | 0.629 (0.415–0.954) | |
Major resection | 0.029 | 1.485 (1.041–2.118) | |||
RFA | 0.009 | 1.760 (1.105–2.695) | |||
Bilobar distribution | 0.002 | 1.739 (1.220–2.479) | |||
Extrahepatic metastases | 0.635 | 0.874 (0.501–1.525) | |||
Diameter of metastases ≥3.0 cm | 0.997 | 1.001 (0.703–1.425) | |||
Multiple metastases | <0.001 | 2.134 (1.415–3.219) | |||
Operation time ≥337.7 min | 0.229 | 1.240 (0.873–1.762) | |||
Blood loss ≥300 mL | 0.782 | 1.051 (0.740–1.493) | |||
Blood transfusion | 0.494 | 1.163 (0.755–1.792) | |||
Poorly differentiated | 0.290 | 1.229 (0.839–1.801) | |||
T3–T4 | 0.412 | 1.236 (0.723–2.204) | |||
Lymph node positive | 0.007 | 1.795 (1.177–2.738) | |||
Oxaliplatin-based schemes | 0.301 | 0.780 (0.488–1.249) | |||
NAC cycles ≥5 | 0.038 | 1.454 (1.022–2.070) | |||
Targeted therapy | 0.061 | 1.422 (0.985–2.052) | |||
Second-line chemotherapy | 0.017 | 1.820 (1.113–2.977) | |||
TRG 1-3 | 0.008 | 0.611 (0.424–0.881) | 0.003 | 0.535 (0.352–0.814) | |
Clinical response | 0.529 | 0.893 (0.629–1.269) | |||
Postoperative complications | 0.101 | 1.344 (0.944–1.915) | |||
Postoperative major complications | 0.861 | 0.961 (0.620–1.491) | |||
Postoperative chemotherapy | 0.042 | 0.693 (0.487–0.988) | 0.050 | 0.690 (0.476–1.000) | |
Pre-NAC LDH ≥145 | 0.017 | 0.601 (0.395–0.914) | 0.030 | 0.584 (0.359–0.950) | |
Preoperative LDH ≥231 | 0.065 | 0.617 (0.369–1.031) | |||
LDH change grade =0 | – | Reference | – | Reference | |
LDH change grade =1 | 0.048 | 0.649 (0.423–0.997) | 0.030 | 0.584 (0.359–0.950) | |
LDH change grade =2 | 0.008 | 0.440 (0.240–0.806) | 0.017 | 0.447 (0.231–0.864) |
BMI, body mass index; ASA, American Society of Anesthesiologists; CEA, carcino-embryonic antigen; RFA, radiofrequency ablation; LDH, lactate dehydrogenase; NAC, neo-adjuvant chemotherapy; TRG, tumor regression grade.
The univariate analysis indicated that the following factors had possible predictive effects for OS: R0 resection (HR 0.039, 95% CI: 0.256–0.596; P<0.001), bilobar distribution (HR 1.172, 95% CI: 1.125–2.605; P=0.012), multiple metastases (HR 1.719, 95% CI: 1.060–2.786; P=0.028), positive lymph nodes (HR 1.776, 95% CI: 1.058-2.981; P=0.030), second-line chemotherapy (HR 2.137, 95% CI: 1.220–3.745; P=0.008), TRG (HR 0.618, 95% CI: 0.400–0.955, P=0.030), postoperative complications (HR 2.369, 95% CI: 1.536–3.653; P<0.001), and postoperative chemotherapy (HR 0.544, 95% CI: 0.358–0.826; P=0.004). A preoperative LDH ≥231 and a grade 2 LDH level change tended to indicate better OS (P<0.1). The multivariate analysis indicated that a preoperative LDH ≥231 IU/L (HR 0.405, 95% CI: 0.192–0.852; P=0.017) and a grade 2 LDH level change (HR 0.362, 95% CI: 0.157–0.834; P=0.017) were independent predictors of OS. The multivariate analysis also found that R0 resection (HR 0.378, 95% CI: 0.231–0.619; P<0.001), second-line chemotherapy (HR 3.091, 95% CI: 1.578–6.054; P=0.001), postoperative complications (HR 2.566, 95% CI: 1.596–4.216, P<0.001), and postoperative chemotherapy (HR 0.453, 95% CI: 0.285–0.721; P=0.001) could predicted OS independently (see Table 5).
Table 5
Item | Univariate analysis | Multivariate analysis | |||
---|---|---|---|---|---|
P | HR (95% CI) | P | HR (95% CI) | ||
Age ≥60 year | 0.214 | 0.753 (0.481–1.178) | |||
Female | 0.789 | 0.941 (0.601–1.472) | |||
BMI >24 kg/m2 | 0.217 | 1.306 (0.855–1.995) | |||
Comorbidity | 0.447 | 0.849 (0.558–1.294) | |||
ASA score 3–4 | 0.662 | 1.155 (0.652–2.044) | |||
Preoperative CEA ≥10 ng/mL | 0.454 | 1.173 (0.773–1.780) | |||
Synchronous metastasis | 0.926 | 1.032 (0.533–1.998) | |||
Primary site Colon | 0.867 | 0965 (0.636–1.464) | |||
Right hemicolon | 0.962 | 1.107 (0.509–2.030) | |||
Heterochronous resection | 0.063 | 1.509 (0.977–2.329) | |||
R0 resection | <0.001 | 0.390 (0.256–0.596) | <0.001 | 0.378 (0.231–0.619) | |
Major resection | 0.053 | 1.515 (0.994–2.310) | |||
RFA | 0.080 | 1.541 (0.950–2.499) | |||
Bilobar distribution | 0.012 | 1.712 (1.125–2.605) | |||
Extrahepatic metastases | 0.939 | 0.974 (0.504–1.882) | |||
Diameter of metastases ≥3.0 cm | 0.163 | 1.345 (0.887–2.041) | |||
Multiple metastases | 0.028 | 1.719 (1.060–2.786) | |||
Operation time ≥337.7 min | 0.763 | 1.066 (0.703–1.617) | |||
Blood loss ≥300 mL | 0.758 | 1.068 (0.704–1.619) | |||
Blood transfusion | 0.109 | 1.506 (0.913–2.484) | |||
Poorly differentiated | 0.295 | 1.271 (0.812–1.998) | |||
T3–T4 | 0.481 | 1.267 (0.656–2.449) | |||
Lymph node positive | 0.030 | 1.776 (1.058–2.981) | |||
Oxaliplatin-based schemes | 0.744 | 1.103 (0.612–1.987) | |||
NAC cycles ≥5 | 0.076 | 1.463 (0.961–2.225) | |||
Targeted therapy | 0.262 | 1.282 (0.831–1.977) | |||
Second-line chemotherapy | 0.008 | 2.137 (1.220–3.745) | 0.001 | 3.091 (1.578–6.054) | |
TRG 1-3 | 0.030 | 0.618 (0.400–0.955) | |||
Clinical response | 0.146 | 0.733 (0.482–1.114) | |||
Postoperative complications | <0.001 | 2.369 (1.536–3.653) | <0.001 | 2.566 (1.596–4.216) | |
Postoperative major complications | 0.615 | 1.150 (0.668–1.981) | |||
Postoperative chemotherapy | 0.004 | 0.544 (0.358–0.826) | 0.001 | 0.453 (0.285–0.721) | |
Pre-NAC LDH ≥145 | 0.308 | 0.778 (0.480–1.261) | |||
Preoperative LDH ≥231 | 0.084 | 0.560 (0.290–1.082) | 0.017 | 0.405 (0.192–0.852) | |
LDH change grade =0 | – | Reference | – | Reference | |
LDH change grade =1 | 0.553 | 0.861 (0.526–1.411) | 0.701 | 0.895 (0.510–1.572) | |
LDH change grade =2 | 0.071 | 0.502 (0.237–1.061) | 0.017 | 0.362 (0.157–0.834) |
BMI, body mass index; ASA, American Society of Anesthesiologists; CEA, carcino-embryonic antigen; RFA, radiofrequency ablation; LDH, lactate dehydrogenase; NAC, neo-adjuvant chemotherapy; TRG, tumor regression grade.
Discussion
This study was the first to investigate the predictive value of changes in serum LDH levels on pathological response, major postoperative complications, and OS in CRLM patients treated by surgery followed NAC. A grade 2 LDH level change was significantly associated with a poor pathological response and the occurrence of major postoperative complications. Further, a preoperative LDH ≥231 IU/L and a pre-NAC LDH ≥145 IU/L were identified as independent predictive factors of OS and PFS, respectively. Additionally, patients with a grade 2 LDH level change had significantly better survival outcomes compared with other grades.
Serum LDH level is an indirect marker of tumor hypoxia, neo-angiogenesis, metastasis, and development in several malignancies (23-28), including CRC (25,28). Given the possible effects of chemotherapy on serum LDH levels, there is significantly more clinical utility in investigating the predictive ability of LDH levels before patients receive NAC. This may provide information about the effectiveness of NAC and the biological characteristics of tumors. In this study, we found that worse TRG was more common among patients with a grade 2 LDH level change, which indicated that elevated LDH levels were a risk factor of poor pathological response. Pre-NAC LDH levels may be related to the nature of the tumor, as pre-NAC LDH levels was not affected by treatment (29).
The mechanism of LDH involvement in poor pathological response remains unclear; however, several possible mechanisms may be involved. First, LDH plays a crucial role in the Warburg effect, which is a common phenomenon in cancer cells. Unlike normal differentiated cells that generate energy by glycolysis only under anaerobic conditions, most cancer cells rely on aerobic glycolysis for energy generation (30). LDH is a key enzyme for glycolysis, which catalyzes the conversion of glucose to lactate. The Warburg effect seems to be a common feature of malignant cells that is important for their tumorigenic potential. Serum LDH levels reflect cellular metabolism and enzyme release by tumors (30,31). Second, previous studies have shown that MYC and PI3K/Akt/mTOR pathways regulate cellular LDH expression levels at translational and transcriptional levels (32,33). Thus, changes in LDH levels may present a reflection of aberrant oncogene activity. The pathological response of NAC in 50% of patients was poor, and chemotherapeutics may have side effects (34,35). Thus, higher LDH levels may indicate that a chemotherapy scheme is not the most eligible scheme for patients or that the most optimal time to receive surgery is not after receiving NAC treatment. Pre-NAC LDH levels may be a potentially effective and easily available marker to select patients who would benefit from NAC. Patients with elevated pre-NAC LDH levels may represent a suitable population for whom a multimodality treatment approach would improve the pathological response of NAC.
Our study found that major postoperative complications were more likely to appear in patients with elevated pre-NAC LDH levels and elevated preoperative LDH levels. There are several possible reasons for this phenomenon. First, elevated LDH serum levels are thought to result from enzyme leakage following the destruction of hepatocytes, especially in patients with hepatitis (36). In addition, it has been proven that hepatocytes increase the production of LDH under hypoxic conditions until they become necrotic (37). Massive hepatocyte necrosis is the most notable character of acute liver failure resulting from liver resection.
Additionally, LDH can be an accurate symbol of hepatic injury (38,39). Further, LDH levels are associated with the dysfunction of various organs (40,41), which can lead to major postoperative complications. Previous studies have shown that the mortality and recurrence rates of patients with postoperative complications were 43% and 38% higher than those without complications, respectively (42). Our findings have led us to reach the same conclusion. Consequently, such an invasive procedure for patients with increased LDH levels may decrease the quality of life, extend recovery time, and increase economic and physical stress.
Recent studies have indicated that pre-treatment LDH levels significantly predict the outcome of metastatic CRC patients treated with chemotherapy (35). However, the predictive value of LDH in CRLM patients is unclear. This study found that increased LDH levels were significantly correlated with favorable PFS and OS in CRLM patients receiving liver resection; however, these results differ from those of other studies (43). It may be that tumor cells obtain energy mainly through glycolysis, and LDH is the key catalytic enzyme for glycolysis. Tumor cells contain a considerable amount of LDH (13). After receiving NAC, tumor cells are destroyed by chemotherapy, leading to the release of LDH from destroyed cells. LDH level may indicate the extent of tumor destruction (44,45). Thus, there appears to be a relationship between preoperative LDH levels and survival.
Considering that NAC may cause changes in LDH levels, as a combined predictor of the pre-NAC LDH levels and preoperative LDH levels, a grade of changes in LDH levels are comprehensive and accurate markers in CRLM patients. The grade is more capable to predict pathological response and postoperative complications and has some predictive effects on PFS and OS. Compared to tests for other potential predictive markers, the test used to measure serum LDH levels is inexpensive, widely used, and easy to perform. Thus, serum LDH levels can be used to guide the treatment of CRLM, and oncologists should consider monitoring dynamic changes in serum LDH levels.
This study had several limitations. First, this was a retrospective and single-center study, so selective bias was inevitable. Further, some toxicity events and complications may have been underreported, especially in less serious cases. In addition, the follow-up period for the survival analysis was relatively short, and the results of this study may require further validation from other cohorts of prospective studies. Despite these limitations, this study provides some guidance for clinical practice.
In conclusion, our study demonstrated that pre-NAC serum LDH levels, preoperative LDH levels, and changes in LDH levels have predictive effects on pathological response and prognosis in CRLM patients who undergo NAC followed by liver resection. Therefore, changes in serum LDH levels warrant more attention from oncologists, especially the dynamic monitoring of changes in LDH levels.
Acknowledgments
We would like to thank L. Huleatt and J. Chapnick for their help in polishing our paper.
Funding: This study was supported by the State Key Project on Infection Diseases of China [Grant number 2017ZX10201021-007-003], the National The capital health research and development of special [Grant number 2018-1-4021], the National Natural Science Foundation of China [Grant numbers 81672461, 81972311], CAMS Innovation Fund for Medical Sciences (CIFMS) [Grant number 2017-12M-4-002], the Non-profit Central Research Institution Fund of Chinese Academy of Medical Sciences [Grant number 2019PT310026] and Sanming Project of Medicine in Shenzhen [Grant number SZSM202011010].
Footnote
Reporting Checklist: The authors have completed the REMARK reporting checklist. Available at https://dx.doi.org/10.21037/apm-21-584
Data Sharing Statement: Available at https://dx.doi.org/10.21037/apm-21-584
Conflicts of Interest: All authors have completed the ICMJE uniform disclosure form (available at https://dx.doi.org/10.21037/apm-21-584). The authors have no conflicts of interest to declare.
Ethics Statement: The authors are accountable for all aspects of the work in ensuring that questions related to the accuracy or integrity of any part of the work are appropriately investigated and resolved. The study was conducted in accordance with the Declaration of Helsinki (as revised in 2013). The study was approved by the Institutional Review Board of the Ethics Committee of Cancer Hospital Chinese Academy of Medical Sciences (ID NCC2019C-016). All included patients gave their informed consent to participate in the study.
Open Access Statement: This is an Open Access article distributed in accordance with the Creative Commons Attribution-NonCommercial-NoDerivs 4.0 International License (CC BY-NC-ND 4.0), which permits the non-commercial replication and distribution of the article with the strict proviso that no changes or edits are made and the original work is properly cited (including links to both the formal publication through the relevant DOI and the license). See: https://creativecommons.org/licenses/by-nc-nd/4.0/.
References
- Dekker E, Tanis PJ, Vleugels JLA, et al. Colorectal cancer. Lancet 2019;394:1467-80. [Crossref] [PubMed]
- Hackl C, Neumann P, Gerken M, et al. Treatment of colorectal liver metastases in Germany: a ten-year population-based analysis of 5772 cases of primary colorectal adenocarcinoma. BMC Cancer 2014;14:810. [Crossref] [PubMed]
- Engstrand J, Nilsson H, Strömberg C, et al. Colorectal cancer liver metastases - a population-based study on incidence, management and survival. BMC Cancer 2018;18:78. [Crossref] [PubMed]
- Yoshino T, Arnold D, Taniguchi H, et al. Pan-Asian adapted ESMO consensus guidelines for the management of patients with metastatic colorectal cancer: a JSMO-ESMO initiative endorsed by CSCO, KACO, MOS, SSO and TOS. Ann Oncol 2018;29:44-70. [Crossref] [PubMed]
- Mitry E, Fields AL, Bleiberg H, et al. Adjuvant chemotherapy after potentially curative resection of metastases from colorectal cancer: a pooled analysis of two randomized trials. J Clin Oncol 2008;26:4906-11. [Crossref] [PubMed]
- Battula N, Tsapralis D, Mayer D, et al. Repeat liver resection for recurrent colorectal metastases: a single-centre, 13-year experience. HPB (Oxford) 2014;16:157-63. [Crossref] [PubMed]
- Kishi Y, Zorzi D, Contreras CM, et al. Extended preoperative chemotherapy does not improve pathologic response and increases postoperative liver insufficiency after hepatic resection for colorectal liver metastases. Ann Surg Oncol 2010;17:2870-6. [Crossref] [PubMed]
- Du Pasquier C, Roulin D, Bize P, et al. Tumor response and outcome after reverse treatment for patients with synchronous colorectal liver metastasis: a cohort study. BMC Surg 2020;20:78. [Crossref] [PubMed]
- Serayssol C, Maulat C, Breibach F, et al. Predictive factors of histological response of colorectal liver metastases after neoadjuvant chemotherapy. World J Gastrointest Oncol 2019;11:295-309. [Crossref] [PubMed]
- Kim HS, Lee JM, Kim HS, et al. Prognosis of Synchronous Colorectal Liver Metastases After Simultaneous Curative-Intent Surgery According to Primary Tumor Location and KRAS Mutational Status. Ann Surg Oncol 2020;27:5150-8. [Crossref] [PubMed]
- Chen Q, Zhao H, Wu J, et al. Preoperative D-dimer and Gamma-Glutamyltranspeptidase Predict Major Complications and Survival in Colorectal Liver Metastases Patients After Resection. Transl Oncol 2019;12:996-1004. [Crossref] [PubMed]
- Frühling P, Hellberg K, Ejder P, et al. The prognostic value of C-reactive protein and albumin in patients undergoing resection of colorectal liver metastases. A retrospective cohort study. HPB (Oxford) 2021;23:970-8. [Crossref] [PubMed]
- Feron O. Pyruvate into lactate and back: from the Warburg effect to symbiotic energy fuel exchange in cancer cells. Radiother Oncol 2009;92:329-33. [Crossref] [PubMed]
- Maftouh M, Avan A, Sciarrillo R, et al. Synergistic interaction of novel lactate dehydrogenase inhibitors with gemcitabine against pancreatic cancer cells in hypoxia. Br J Cancer 2014;110:172-82. [Crossref] [PubMed]
- Greten FR, Grivennikov SI. Inflammation and Cancer: Triggers, Mechanisms, and Consequences. Immunity 2019;51:27-41. [Crossref] [PubMed]
- Liu L, He Y, Ge G, et al. Lactate dehydrogenase and creatine kinase as poor prognostic factors in lung cancer: A retrospective observational study. PLoS One 2017;12:e0182168 [Crossref] [PubMed]
- Li G, Wang Z, Xu J, et al. The prognostic value of lactate dehydrogenase levels in colorectal cancer: a meta-analysis. BMC Cancer 2016;16:249. [Crossref] [PubMed]
- Namikawa T, Ishida N, Tsuda S, et al. Prognostic significance of serum alkaline phosphatase and lactate dehydrogenase levels in patients with unresectable advanced gastric cancer. Gastric Cancer 2019;22:684-91. [Crossref] [PubMed]
- Liu D, Wang D, Wu C, et al. Prognostic significance of serum lactate dehydrogenase in patients with breast cancer: a meta-analysis. Cancer Manag Res 2019;11:3611-9. [Crossref] [PubMed]
- Eisenhauer EA, Therasse P, Bogaerts J, et al. New response evaluation criteria in solid tumours: revised RECIST guideline (version 1.1). Eur J Cancer 2009;45:228-47. [Crossref] [PubMed]
- Rubbia-Brandt L, Giostra E, Brezault C, et al. Importance of histological tumor response assessment in predicting the outcome in patients with colorectal liver metastases treated with neo-adjuvant chemotherapy followed by liver surgery. Ann Oncol 2007;18:299-304. [Crossref] [PubMed]
- Dindo D, Demartines N, Clavien PA. Classification of surgical complications: a new proposal with evaluation in a cohort of 6336 patients and results of a survey. Ann Surg 2004;240:205-13. [Crossref] [PubMed]
- Ostergaard L, Tietze A, Nielsen T, et al. The relationship between tumor blood flow, angiogenesis, tumor hypoxia, and aerobic glycolysis. Cancer Res 2013;73:5618-24. [Crossref] [PubMed]
- Brown JE, Cook RJ, Lipton A, et al. Serum lactate dehydrogenase is prognostic for survival in patients with bone metastases from breast cancer: a retrospective analysis in bisphosphonate-treated patients. Clin Cancer Res 2012;18:6348-55. [Crossref] [PubMed]
- Bar J, Spencer S, Morgan S, et al. Correlation of lactate dehydrogenase isoenzyme profile with outcome in patients with advanced colorectal cancer treated with chemotherapy and bevacizumab or cediranib: Retrospective analysis of the HORIZON I study. Clin Colorectal Cancer 2014;13:46-53. [Crossref] [PubMed]
- Faloppi L, Scartozzi M, Bianconi M, et al. The role of LDH serum levels in predicting global outcome in HCC patients treated with sorafenib: implications for clinical management. BMC Cancer 2014;14:110. [Crossref] [PubMed]
- Armstrong AJ, George DJ, Halabi S. Serum lactate dehydrogenase predicts for overall survival benefit in patients with metastatic renal cell carcinoma treated with inhibition of mammalian target of rapamycin. J Clin Oncol 2012;30:3402-7. [Crossref] [PubMed]
- Passardi A, Scarpi E, Tamberi S, et al. Impact of Pre-Treatment Lactate Dehydrogenase Levels on Prognosis and Bevacizumab Efficacy in Patients with Metastatic Colorectal Cancer. PLoS One 2015;10:e0134732 [Crossref] [PubMed]
- Buder-Bakhaya K, Hassel JC. Biomarkers for Clinical Benefit of Immune Checkpoint Inhibitor Treatment-A Review From the Melanoma Perspective and Beyond. Front Immunol 2018;9:1474. [Crossref] [PubMed]
- Vander Heiden MG, Cantley LC, Thompson CB. Understanding the Warburg effect: the metabolic requirements of cell proliferation. Science 2009;324:1029-33. [Crossref] [PubMed]
- Sun Q, Chen X, Ma J, et al. Mammalian target of rapamycin up-regulation of pyruvate kinase isoenzyme type M2 is critical for aerobic glycolysis and tumor growth. Proc Natl Acad Sci U S A 2011;108:4129-34. [Crossref] [PubMed]
- Kim JW, Dang CV. Cancer's molecular sweet tooth and the Warburg effect. Cancer Res 2006;66:8927-30. [Crossref] [PubMed]
- Kim JW, Dang CV. Multifaceted roles of glycolytic enzymes. Trends Biochem Sci 2005;30:142-50. [Crossref] [PubMed]
- Nordlinger B, Sorbye H, Glimelius B, et al. Perioperative chemotherapy with FOLFOX4 and surgery versus surgery alone for resectable liver metastases from colorectal cancer (EORTC Intergroup trial 40983): a randomised controlled trial. Lancet 2008;371:1007-16. [Crossref] [PubMed]
- Tamandl D, Klinger M, Eipeldauer S, et al. Sinusoidal obstruction syndrome impairs long-term outcome of colorectal liver metastases treated with resection after neoadjuvant chemotherapy. Ann Surg Oncol 2011;18:421-30. [Crossref] [PubMed]
- Kotoh K, Enjoji M, Kato M, et al. A new parameter using serum lactate dehydrogenase and alanine aminotransferase level is useful for predicting the prognosis of patients at an early stage of acute liver injury: a retrospective study. Comp Hepatol 2008;7:6. [Crossref] [PubMed]
- Kotoh K, Kato M, Kohjima M, et al. Lactate dehydrogenase production in hepatocytes is increased at an early stage of acute liver failure. Exp Ther Med 2011;2:195-9. [Crossref] [PubMed]
- Wang Y, Liu H, Liu S, et al. Incidence, predictors and prognosis of genotype 4 hepatitis E related liver failure: A tertiary nested case-control study. Liver Int 2019;39:2291-300. [Crossref] [PubMed]
- Ferriero R, Nusco E, De Cegli R, et al. Pyruvate dehydrogenase complex and lactate dehydrogenase are targets for therapy of acute liver failure. J Hepatol 2018;69:325-35. [Crossref] [PubMed]
- Gong H, Sheng X, Xue J, et al. Expression and role of TNIP2 in multiple organ dysfunction syndrome following severe trauma. Mol Med Rep 2019;19:2906-12. [Crossref] [PubMed]
- Su Y, Ju MJ, Ma JF, et al. Lactate dehydrogenase as a prognostic marker of renal transplant recipients with severe community-acquired pneumonia: a 10-year retrospective study. Ann Transl Med 2019;7:660. [Crossref] [PubMed]
- Dorcaratto D, Mazzinari G, Fernandez M, et al. Impact of Postoperative Complications on Survival and Recurrence After Resection of Colorectal Liver Metastases: Systematic Review and Meta-analysis. Ann Surg 2019;270:1018-27. [Crossref] [PubMed]
- Miao P, Sheng S, Sun X, et al. Lactate dehydrogenase A in cancer: a promising target for diagnosis and therapy. IUBMB Life 2013;65:904-10. [Crossref] [PubMed]
- Urbańska K, Orzechowski A. Unappreciated Role of LDHA and LDHB to Control Apoptosis and Autophagy in Tumor Cells. Int J Mol Sci 2019;20:2085. [Crossref] [PubMed]
- Pathria G, Scott DA, Feng Y, et al. Targeting the Warburg effect via LDHA inhibition engages ATF4 signaling for cancer cell survival. EMBO J 2018;37:e99735 [Crossref] [PubMed]