Bone stress injuries in female athletes
Introduction
Bone stress injuries are a frequently seen type of overuse injury in females and make up to 20% of injuries seen in sports medicine clinics (1). Female athletes and military recruits in particular are at higher risk for bone stress injures as their higher physical activity levels and often lower body mass index may predispose them to periods of low energy availability and insufficient nutrition (2,3). Increased energy expenditure compounded by inadequate nutrition may lead to menstrual and hormonal irregularities that impair bone health and bone density (2-4). For young female athletes in particular, a hypoestrogenic state during adolescence may lead to lower peak bone mass that is irreversible and may put them at risk for bone stress injuries later in life (3,4).
Epidemiology of bone stress injuries in female athletes
Many athletes, including runners, dancers and military recruits, are at a higher risk for developing a stress fracture (5,6) with female runners being at highest risk (7). An epidemiological analysis of collegiate athletes from the NCAA Injury Surveillance Program found that 9.13/100,000 females and 4.44/100,000 males were diagnosed with a stress fracture within the 9-year period of the study (8). The most common injury locations were the metatarsals (37.9% of all stress fractures), tibia (21.9%) and lumbar spine/pelvis (12.1%). Female athletes across all sports were observed (baseball/softball, basketball, cross-country, ice hockey, lacrosse, soccer, swim and dive, tennis, indoor and outdoor track) to sustain higher incidences of stress fractures than male athletes. While females were observed to obtain almost twice as many stress fractures than males, there were some sexual dimorphisms in injury location. Males sustained more pelvic stress fractures than females (12.8% vs. 6.9%). Additionally, females were diagnosed with more stress fractures occurring in the femur than males (12.2% vs. 4.4%) (8). While endurance athletes continue to have some of the highest rates of stress fractures, this study found that other high-risk populations include gymnasts. Wentz et al. in a systematic review found that the incidence of stress fractures was higher in female athletes and military recruits than males (9.7% in female athletes vs. 6.5% in male athletes; 9.2% in female military recruits vs. 3% in male military recruits) (3). However, they found that female athletes and recruits with normal body mass index and markers of bone health were less likely to develop a stress fracture suggesting these factors may be more important than sex alone. Tenforde et al. conducted a prospective survey study of 748 competitive high school runners over a mean period of 2.3±1.2 competitive seasons (9). They found 5.4% of girls and 4.0% of boys sustained a stress fracture with tibial stress fractures more common in girls and metatarsal fractures more common in boys. They found low body mass index, late menarche and prior participation in dance or gymnastics were risk factors for stress fractures in girls, and prior history of stress fracture a risk factor for both boys and girls (9).
Most stress fractures occur in the lower extremities. Though high risk locations vary by population, some of the most common areas susceptible to stress fractures are the tibia, hip/pelvis area and metatarsals (5,6,8,10,11). While stress fractures of the upper extremities are less common, they do occur in athletes that bear weight with their upper bodies (i.e., weightlifters, rowers, volleyball players, gymnasts, overhead athletes), and such fractures are normally in the ribs and humerus (12,13). Stress fractures of the upper extremities and torso account for about 10% of all stress fractures (12).
Pathophysiology of bone stress injuries
Bone stress injuries often occur as a result of an accumulation of microdamage to bone from excessive strain from forces above the patient’s baseline level of activity without adequate recovery (Figure 1) (4-6). Conversely, bone stress injuries can also occur due to abnormal or depressed bone remodeling in response to normal strain as seen in people with disordered eating or hormonal abnormalities disrupting bone metabolism (4). Repetitive loading of bone alters the microstructure of bone and signals bone remodeling by upregulating osteoclastic and osteoblastic activity (15,16). Osteoblastic activity generally lags behind osteoclastic activity and when bone is persistently stressed without adequate time for recovery, the bone is at risk for fatigue fracture or stress injury (15). Bone stress injuries occur as a continuum ranging from stress reactions demonstrating bony microfracture without cortical disruption to stress fracture with a clear fracture line through the cortex visible on imaging (15). With continued load and strain progressive accumulation of microdamage can allow for crack propogation and macroscopic fracture (4). Additionally, poor muscle strength and fatigue can put an athlete at risk for stress injury as more force is transmitted through bone that can lead to increased microdamage (4).
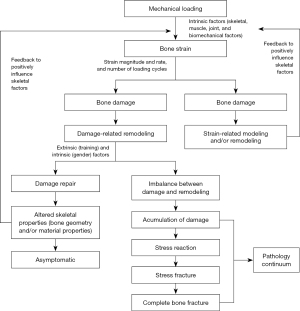
Risk factors for bone stress injuries
The causes of bone stress injuries are multifactorial and include both intrinsic and extrinsic factors (Table 1). Intrinsic risk factors are comprised of characteristics that are inherent to the athlete and affect the body’s ability to respond to applied loads (14). These include demographic factors, hormonal abnormalities, bone quality, cardiovascular health, muscular strength, anatomical alignment, muscle fatigue/imbalance, gait, footstrike, posture and insufficient neuromuscular function. Extrinsic factors are comprised of training schedule, training equipment (footwear, training surface) and diet (5,6). Many of these factors, if insufficiently adjusted to meet the loads demanded of the body, can culminate in low bone mineral content, low bone mass density and decreased bone diameters; all of which increase overall risk of stress fracture (10,16).
Table 1
Intrinsic risk factors | Extrinsic risk factors |
---|---|
Female sex; Race; Poor aerobic fitness or muscle strength; Low bone mineral density; Smaller bone geometry; Prior history of stress fracture; Low calcium and vitamin D | High training volume; Increased training intensity; Rapid changes in training program; Poor or older athletic footwear; Hard or uneven training surface; Tobacco use; Medications (corticosteroids, thyroid hormone, anticonvulsants); Disordered eating/poor caloric intake |
Anatomic factors | |
● Foot structure (pes planus or pes cavus) | |
● Leg length discrepancy | |
● Wider pelvis structure | |
● Increased hip external rotation | |
● Genu valgus or varus | |
Hormonal factors | |
● Delayed menarche (age ≥15) | |
● Menstrual irregularities |
Extrinsic risk factors
Extrinsic risk factors include the training environment or factors external to the athlete. Important to this is type of sport played and training specific factors. Athletes who participate in sports that involve high magnitude loads in a short period such as sprinting and cumulative high volume loads such as long distance running are at risk for bone stress injuries due to high strain rates and cyclic load respectively (14). Rizzone et al. found that the collegiate sports with the highest rate of stress fractures were women’s cross-country (28.59/100,000 AEs), women’s gymnastics (25.58/100,000 AEs), and women’s outdoor track (22.26/100,000 AEs) (8). Factors specific to training programs can influence risk of bone stress injury including volume, frequency and intensity of training regimen. Studies in runners have shown an increased risk of stress fracture with higher mileage programs (4,17). Additionally, ballet dancers who trained for over 5 hours per day were at higher risk of stress fracture than those who trained less than 5 hours (18). Shoe wear and orthotics have been implicated in risk of bone stress injuries with older shoes and not wearing custom orthoses having been associated with higher risk of stress injuries in the military population (19,20). However, other studies have no relationship between footwear and risk of bone stress injury (19,21). Sun et al. found the influence of foot strike pattern, particularly rearfoot strike patterns, while running was more important than that of shoe condition on plantar pressure characteristics (22). Additionally, training surface may theoretically contribute to the development of bone stress injuries with the potential for increased impact of forces through bone with running on hard surfaces and for muscle fatigue and overloading of bone with training on uneven surfaces (4,14).
Intrinsic risk factors
There are a number of risk factors inherent to females that may predispose them to bone stress injuries. While likely multifactorial, female sex alone is a risk factor for bone stress injury as many studies have found a higher incidence of bone stress injuries in females compared to males (3,4,23). A number of factors including anatomic factors (e.g., pes planus, pes cavus, leg length discrepancy, genu varum/valgum, smaller bone geometry), aerobic fitness and muscle strength, and biomechanical factors may place females at a higher risk of stress fractures. This is predominantly due to stress to bone from features of the applied load or affecting the ability of the bone to resist deformation in the setting of the load (Table 1) (3,4,15,23).
Important to the risk of stress injuries in female athletes includes the relationship between energy availability, menstrual function and bone density, also known as the “female athlete triad” (15,24). The female athlete triad is a combination of three symptoms: low energy availability, irregular menstruation, and low bone density (24). Athletes who present with one or more of these symptoms are noted to be at a higher risk of developing a bone stress injury. Nose-Ogura et al. found that when a patient has one component of the female athlete triad, they increase their risk of a stress fracture by 2.5 times (25). A patient with two or more components of the female athlete triad are at an even greater risk and are 4.7 times more likely to incur a stress fracture (25). The risk of stress fractures for athletes with amenorrhea has been seen to increase by 12.9 times. Athletes with low bone mineral density follow a similar trend by having a 4.5 times higher risk. Low energy availability also increases the risk of stress fracture by 1.1 times (25). Low energy availability is a pervasive problem among female athletes, and its effects are magnified in athletes who actively restrain or limit their energy intake. Disordered eating in combination with strenuous training, can lead to hypothalamic-pituitary axis dysfunction, estrogen deficiency and menstrual irregularities that may result in lower bone mineral density that may not be reversible (4,26).
Aside from adequate caloric intake, vitamin D and calcium are recognized as important nutritional factors to achieve and preserve bone health (9,27). While some studies have had conflicting results, a number of studies have shown a reduced incidence of stress fractures with adequate calcium and vitamin D intake (28-30). Lappe et al. showed a 20% risk reduction of stress fractures in female navy recruits taking 2,000 mg calcium and 800 IU vitamin D vs. placebo (28). Nieves et al. found that female distance runners who consumed less than 800 mg of calcium per day had almost 6 times the rate of bone stress injuries than those who consumed more than 1,500 mg (29). Overall, most literature supports the importance of active young females meeting or exceeding the current recommended daily levels of vitamin D (600–800 IU) and calcium (1,000–1,300 mg) (14,31).
Diagnosis and classification of bone stress injuries
Important to the diagnosis and classification of bone stress injuries are the level of symptoms, the anatomic site, and the grade of injury. Patients typically present with pain in the affected area that is insidious in onsent. Point tenderness at the injury site of a stress fracture has been shown to appear in 66–100% of cases (32). Bones stress injuries have varying levels of severity, and these can be broken up into low- and high-risk fractures (Table 2). Low-risk stress fractures tend to be on the compression side of bone and have a favorable history of responding well to activity modification (14,15). High-risk fractures are more often on the tension side of bone and have a higher risk of complications including recurrence, nonunion or completion of fracture (14,15). When diagnosing a stress fracture, the first line of imaging is typically an X-ray. However, due to microdamage to bone, they are often undetectable by X-ray (35). While bone scans have traditionally been a preferred imaging modality for diagnosis of bone stress injuries, their accuracy and specificity for stress fractures has been shown to be low (36). Therefore, magnetic resonance imaging (MRI) has become the modality of choice for grading of severity of stress fractures. While multiple MRI grading systems exist, the Fredericson MRI Classification System originally made for tibial bone stress injuries remains a frequently used grading system (Table 3) (14,37).
Table 2
Low-risk | High-risk |
---|---|
Pelvis; Femoral diaphysis; Posteromedial tibia; Fibula; Calcaneus; First to fourth metatarsals | Olecranon; Scaphoid; Femoral neck (tension side); Patella; Anterior tibia; Medial malleolus; Talus; Tarsal navicular; Proximal fifth metatarsal; Great toe sesamoids |
Table 3
Grade of stress injury | MRI findings |
---|---|
0 | No abnormality |
1 | Periosteal edema with no associated marrow signal changes |
2 | Periosteal edema and bone marrow edema visible on T2-weighted images only |
3 | Periosteal edema and bone marrow edema visible on T1-weighted and T2-weighted images |
4a | Multiple focal areas of intracortical signal changes and bone marrow edema visible on T1-weighted and T2-weighted images |
4b | Linear areas of intracortical signal changes and bone marrow edema visible on T1-weighted and T2-weighted images |
General treatment principles for bone stress injuries
Low risk bone stress injuries
Treatment of bone stress injuries begins with proper classification and grade of bone stress injury, identification of both intrinsic and extrinsic risk factors, and assessment of the athlete’s competitive status (4,15). Proper diagnosis is important as management can vary from relative rest to surgical (4). A treatment plan should be shaped to meet the patient’s athletic and individual goals while allowing for bone healing. Treatment of bone stress injuries can be approached in a two-phase process (Table 4) (2,38). The first phase involves pain control, activity modification, and identifying and addressing underlying risk factors. Pain control tactics include applying ice to the affected area, physical therapy modalities, and oral analgesics. Avoiding non-steroidal anti-inflammatory drugs (NSAIDs) has been recommended by some due to potential adverse effects on bone healing (2,39). Weight bearing as tolerated may be allowed in low-risk stress fractures if no pain with ambulation but participation in sports should be stopped. If unable to walk with a normal gait due to discomfort, partial weight bearing with an assistive device such as crutches or use of a walking boot or post op shoe depending on injury site may be necessary. A period of relative rest that allows for low impact cross training activities (e.g., cycling, swimming, pool running, elliptical) that do not cause pain may be appropriate to help the athlete maintain cardiovascular fitness. Complete rest is indicated if the athlete has pain with cross training activities and in certain recurrent or high-risk bone stress injuries.
Table 4
Treatment phases | Main components | ||
---|---|---|---|
Low-risk/high-risk | Nonoperative |
||
Phase I | Pain control | Ice | |
Physical therapy modalities | |||
Oral analgesics | |||
Activity modification | Weight bearing modifications | ||
Relative rest |
|||
Identify intrinsic/extrinsic risk factors | Nutritional deficiencies | ||
Hormonal imbalances | |||
Low bone mineral density | |||
Training regimen errors | |||
Biomechanical errors | |||
Accelerated healing techniques | Pneumatic compression devices | ||
Low intensity pulsed ultrasound | |||
Extracorporeal shock wave therapy | |||
Bone stimulators | |||
Pharmacologic agents* | Bisphosphates | ||
Recombinant parathyroid hormone | |||
Intranasal calcitonin | |||
Oral contraceptives | |||
Phase II | Return to running/sport | Timing dependent on site/grade | |
Confirm healing on imaging on high-risk injuries | |||
Begins 10–14 days after pain free | |||
Running to begin 1 week after no bony tenderness to palpation | |||
Gradual return to activity over 3–6 weeks period modified by symptoms |
*, caution of use of supplemental pharmacologic agents in skeletally immature and pre-menopausal women.
High-risk bone stress injuries
Management of high-risk bone stress injuries involves a different initial approach as these are at a higher risk of a poorer prognosis if completion of fracture occurs as well as complications including nonunion, malunion, and refracture (15). Depending on site and grade of the stress fracture the recommended treatment ranges from complete rest to surgical management. Complete healing should be verified prior to return to sport. Low grade stress injuries at high-risk sites can generally be managed with a period of non-weight bearing. Surgical management of stress fractures at high-risk sites may be appropriate in certain cases to allow for possible expedited healing and return to play for foot fractures such as navicular or fifth metatarsal (40). Possible prevention of recurrence in subsequent seasons of play, and prevention of catastrophic fracture progression as in displaced femoral neck stress fractures or medial malleolus stress fractures (15).
Identification and treatment of risk factors
During the initial treatment phase both intrinsic and extrinsic risk factors for bone stress injuries should be identified and addressed. Intrinsic risk factors including disordered eating, nutritional deficiencies, hormonal imbalances, medications and low bone density should be recognized (4). Specific to female athletes, a detailed menstrual history should be taken including age of menarche and menstrual status. Laboratory testing should be considered if nutritional issues are present or in recurrent stress fractures and should include serum calcium, 25 hydroxy (OH) vitamin D3, phosphorus, parathyroid hormone, thyroid stimulating hormone, alkaline phosphatase, albumin and prealbumin (33,41). Hormonal levels [follicle stimulating hormone (FSH), luteinizing hormone (LH), estradiol] may be tested in those with menstrual irregularities (33,41). Bone density testing with dual energy X-ray absorptiometry (DEXA) scan may be ordered in those with recurrent or history of multiple bone stress injuries (33). Athletes should undergo a gait and biomechanical analysis to evaluate any deficiencies in gait or form, asymmetrical muscle imbalances, and any structural abnormalities including leg length discrepancies, pes planus or pes cavus (4). This should be conducted by a trained physician, physical therapist, and/or athletic trainer. Additionally, a team approach to female athletes with bone stress injuries and the female athlete triad with co-management between the treating physician and potentially a psychologist, gynecologist, and nutritionist as well as family, trainers and coach should be employed. A detailed physical activity history is important to consider to identify any changes in training including increases in intensity, volume and frequency as to adjust training plans appropriately in the future (14).
Adjunctive therapies for accelerated bone healing
While there are no proven methods of accelerated healing of bone stress injuries, “accelerated” tissue healing techniques have been described. Pneumatic leg braces may be used in fibula and tibia stress fractures to promote healing and to help foster a pain free gait (42). While some studies have shown some healing benefit using low intensity pulsed ultrasound (LIPUS) (14,43), a recent systematic review of 26 randomized controlled trials determined based on moderate to high quality evidence studies that LIPUS does not lead to improved patient outcomes and likely has no effect on radiographic bone healing (44). Extracorporeal shockwave therapy has been shown to be effective in treating recalcitrant bone stress injuries and nonunions (14,45), but little evidence exists on its utility in treating acute stress injuries. Bone stimulators have also been used, however, evidence of their efficacy remains inconclusive (2). Pharmacologic treatments to increase bone mineral strength in the setting of low bone mineral density or nonhealing fractures include bisphosphates and recombinant parathyroid hormone. However, trials evaluating the safety and efficacy of these medications in this young patient population are limited, particularly with bisphosphonates as they have possible long lasting teratogenic effects (46). Intranasal calcitonin has been shown to decrease pain associated with osteoporotic fractures (47) and possibly strengthen bone microstructure (48); however, studies have been only been conducted in postmenopausal women. While use of oral contraceptives to help regulate hormonal abnormalities has been shown to be protective against bone stress injuries (31,49), some recent data has suggested that the use of combined oral contraceptive pills in adolescents may lead to premature physeal closure (50) and less peak bone mineral density accrual at the hip (51) and spine (52) than non-users.
Return to sport
Phase II involves preparing the athlete to return to impact running or sporting activity. The return to sport protocol for stress fractures is highly individualized. It is based on the grade and location of injury, as well as the athletic goals of the patient. Overtreatment of a stress fracture results in unnecessary loss of training; while undertreatment results in a high chance of injury progression and extended recovery time (53). This phase can be initiated after the athlete has been pain free for 10 to 14 days (2). Running can be generally begun 1 week after the resolution of bony tenderness to palpation (2). A gradual return to running program should be followed over a period of 6 weeks and should be dictated by the patient’s pain level. It is advised that there is no more than a 10% increase in activity per week in order to prevent injury recurrence (15). Timing for return to sports participation varies based on location and severity of the stress fracture. Miller et al. looked at return to sport timing in 57 Division I collegiate track and cross-country runners with stress fractures and found that mean time to return to unrestricted sport participation was 12.9±5.2 weeks (range, 6–27 weeks) with no difference in return to sport timing between injury sites and grade II or III stress fractures (53). They also found a trend toward increased time to return in women (13.9±5.7 weeks) compared with men (11.3±3.8 weeks), but this was not statistically significant (P=0.068). Arendt et al. reported the return to sports rates of 74 athletes with lower extremity bone stress injuries at their collegiate institution (54). Using MRI for grading severity of stress injury, they found return to sport was 3.3 weeks for grade 1, 5.5 weeks for grade 2, 11.4 weeks for grade 3 and 14.3 weeks for grade 4 injuries (54). Dobrindt et al. additionally aimed to determine return to sport timing based on injury site and MRI grading of bone stress injuries (55). They found that return to sport timing of only the low-risk/low-grade stress fracture group differed (was significantly less) from all other groups (low-risk/high-grade, high-risk/low-grade, high-risk/high-grade) suggesting the importance of both site and grade in treatment.
Conclusions
Bone stress injuries are common amongst female athletes and can cause significant morbidity and time away from sport. Young female athletes are at a particular risk for bone stress injuries due to a number of intrinsic and extrinsic risk factors, with the female athlete triad and low energy availability as the primary factors leading to impaired bone health. Most low-risk bone stress injuries can be treated with a period of relative rest and addressing underlying risk factors. However, surgical management may be required for certain high risk or recurrent bone stress injuries. Knowledge of the risk factors, classification and treatment of bone stress injuries in females can assist in appropriate management and prevention strategies. Please find a supplemental Q&A between the authors and editors in Supplementary file (Appendix 1).
Acknowledgments
Funding: None.
Footnote
Provenance and Peer Review: This article was commissioned by the Guest Editors (Sommer Hammoud and Robin V. West) for the series “Sports Related Injuries of the Female Athlete” published in Annals of Joint. The article has undergone external peer review.
Conflicts of Interest: All authors have completed the ICMJE uniform disclosure form (available at http://dx.doi.org/10.21037/aoj.2020.04.04). The series “Sports Related Injuries of the Female Athlete” was commissioned by the editorial office without any funding or sponsorship. The authors have no other conflicts of interest to declare.
Ethical Statement: The authors are accountable for all aspects of the work in ensuring that questions related to the accuracy or integrity of any part of the work are appropriately investigated and resolved.
Open Access Statement: This is an Open Access article distributed in accordance with the Creative Commons Attribution-NonCommercial-NoDerivs 4.0 International License (CC BY-NC-ND 4.0), which permits the non-commercial replication and distribution of the article with the strict proviso that no changes or edits are made and the original work is properly cited (including links to both the formal publication through the relevant DOI and the license). See: https://creativecommons.org/licenses/by-nc-nd/4.0/.
References
- Fredericson M, Jennings F, Beaulieu C, et al. Stress fractures in athletes. Top Magn Reson Imaging 2006;17:309-25. [Crossref] [PubMed]
- Chen YT, Tenforde AS, Fredericson M. Update on stress fractures in female athletes: epidemiology, treatment, and prevention. Curr Rev Musculoskelet Med 2013;6:173-81. [Crossref] [PubMed]
- Wentz LM, Liu PY, Ilich JZ, et al. Female Distance Runners Training In Southeastern United States Have Adequate Vitamin D Status. Int J Sport Nutr Exerc Metab 2016;26:397-403. [Crossref] [PubMed]
- Zeni AI, Street CC, Dempsey RL, et al. Stress injury to the bone among women athletes. Phys Med Rehabil Clin N Am 2000;11:929-47. [Crossref] [PubMed]
- Mandell JC, Khurana B, Smith SE. Stress fractures of the foot and ankle, part 2: site-specific etiology, imaging, and treatment, and differential diagnosis. Skeletal Radiol 2017;46:1165-86. [Crossref] [PubMed]
- Mandell JC, Khurana B, Smith SE. Stress fractures of the foot and ankle, part 1: biomechanics of bone and principles of imaging and treatment. Skeletal Radiol 2017;46:1021-9. [Crossref] [PubMed]
- Jones BH, Thacker SB, Gilchrist J, et al. Prevention of lower extremity stress fractures in athletes and soldiers: a systematic review. Epidemiol Rev 2002;24:228-47. [Crossref] [PubMed]
- Rizzone KH, Ackerman KE, Roos KG, et al. The Epidemiology of Stress Fractures in Collegiate Student-Athletes, 2004-2005 Through 2013-2014 Academic Years. J Athl Train 2017;52:966-75. [Crossref] [PubMed]
- Tenforde AS, Carlson JL, Chang A, et al. Association of the Female Athlete Triad Risk Assessment Stratification to the Development of Bone Stress Injuries in Collegiate Athletes. Am J Sports Med 2017;45:302-10. [Crossref] [PubMed]
- Davey T, Lanham-New SA, Shaw AM, et al. Fundamental differences in axial and appendicular bone density in stress fractured and uninjured Royal Marine recruits--a matched case-control study. Bone 2015;73:120-6. [Crossref] [PubMed]
- Loud KJ, Gordon CM, Micheli LJ, et al. Correlates of stress fractures among preadolescent and adolescent girls. Pediatrics 2005;115:e399-406. [Crossref] [PubMed]
- Miller TL, Harris JD, Kaeding CC. Stress fractures of the ribs and upper extremities: causation, evaluation, and management. Sports Med 2013;43:665-74. [Crossref] [PubMed]
- Sinha AK, Kaeding CC, Wadley GM. Upper extremity stress fractures in athletes: clinical features of 44 cases. Clin J Sport Med 1999;9:199-202. [Crossref] [PubMed]
- Warden SJ, Davis IS, Fredericson M. Management and prevention of bone stress injuries in long-distance runners. J Orthop Sports Phys Ther 2014;44:749-65. [Crossref] [PubMed]
- Diehl JJ, Best TM, Kaeding CC. Classification and return-to-play considerations for stress fractures. Clin Sports Med 2006;25:17-28. vii. [Crossref] [PubMed]
- Hughes JM, Popp KL, Yanovich R, et al. The role of adaptive bone formation in the etiology of stress fracture. Exp Biol Med (Maywood) 2017;242:897-906. [Crossref] [PubMed]
- Macera CA, Pate RR, Powell KE, et al. Predicting lower-extremity injuries among habitual runners. Arch Intern Med 1989;149:2565-8. [Crossref] [PubMed]
- Kadel NJ, Teitz CC, Kronmal RA. Stress fractures in ballet dancers. Am J Sports Med 1992;20:445-9. [Crossref] [PubMed]
- Finestone A, Giladi M, Elad H, et al. Prevention of stress fractures using custom biomechanical shoe orthoses. Clin Orthop Relat Res 1999;182-90. [Crossref] [PubMed]
- Gardner LI, Dziados JE, Jones BH, et al. Prevention of lower extremity stress fractures: a controlled trial of a shock absorbent insole. Am J Public Health 1988;78:1563-7. [Crossref] [PubMed]
- Milgrom C, Finestone A, Shlamkovitch N, et al. Prevention of overuse injuries of the foot by improved shoe shock attenuation. A randomized prospective study. Clin Orthop Relat Res 1992;189-92. [PubMed]
- Sun X, Yang Y, Wang L, et al. Do Strike Patterns or Shoe Conditions have a Predominant Influence on Foot Loading? J Hum Kinet 2018;64:13-23. [Crossref] [PubMed]
- Nattiv A, Armsey TD. Stress injury to bone in the female athlete. Clin Sports Med 1997;16:197-224. [Crossref] [PubMed]
- Skolnick AA. “Female athlete triad” risk for women. JAMA 1993;270:921-3. [Crossref] [PubMed]
- Nose-Ogura S, Yoshino O, Dohi M, et al. Risk factors of stress fractures due to the female athlete triad: Differences in teens and twenties. Scand J Med Sci Sports 2019;29:1501-10. [Crossref] [PubMed]
- Moreira CA, Bilezikian JP. Stress Fractures: Concepts and Therapeutics. J Clin Endocrinol Metab 2017;102:525-34. [PubMed]
- Recker RR, Davies KM, Hinders SM, et al. Bone gain in young adult women. JAMA 1992;268:2403-8. [Crossref] [PubMed]
- Lappe J, Cullen D, Haynatzki G, et al. Calcium and vitamin d supplementation decreases incidence of stress fractures in female navy recruits. J Bone Miner Res 2008;23:741-9. [Crossref] [PubMed]
- Nieves JW, Melsop K, Curtis M, et al. Nutritional factors that influence change in bone density and stress fracture risk among young female cross-country runners. PM R 2010;2:740-50; quiz 794. [Crossref] [PubMed]
- Ruohola JP, Laaksi I, Ylikomi T, et al. Association between serum 25(OH)D concentrations and bone stress fractures in Finnish young men. J Bone Miner Res 2006;21:1483-8. [Crossref] [PubMed]
- Kelsey JL, Bachrach LK, Procter-Gray E, et al. Risk factors for stress fracture among young female cross-country runners. Med Sci Sports Exerc 2007;39:1457-63. [Crossref] [PubMed]
- Saunier J, Chapurlat R. Stress fracture in athletes. Joint Bone Spine 2018;85:307-10. [Crossref] [PubMed]
- Miller TL, Best TM. Taking a holistic approach to managing difficult stress fractures. J Orthop Surg Res 2016;11:98. [Crossref] [PubMed]
- McInnis KC, Ramey LN. High-Risk Stress Fractures: Diagnosis and Management. PM R 2016;8:S113-24. [Crossref] [PubMed]
- Matcuk GR, Mahanty SR, Skalski MR, et al. Stress fractures: pathophysiology, clinical presentation, imaging features, and treatment options. Emerg Radiol 2016;23:365-75. [Crossref] [PubMed]
- Nye NS, Covey CJ, Sheldon L, et al. Improving Diagnostic Accuracy and Efficiency of Suspected Bone Stress Injuries. Sports Health 2016;8:278-83. [Crossref] [PubMed]
- Fredericson M, Bergman AG, Hoffman KL, et al. Tibial stress reaction in runners. Correlation of clinical symptoms and scintigraphy with a new magnetic resonance imaging grading system. Am J Sports Med 1995;23:472-81. [Crossref] [PubMed]
- Andrish J. The management of recurrent patellar dislocation. Orthop Clin North Am 2008;39:313-27. vi. [Crossref] [PubMed]
- Ziltener JL, Leal S, Fournier PE. Non-steroidal anti-inflammatory drugs for athletes: an update. Ann Phys Rehabil Med 2010;53:278-82, 282-8.
- Mallee WH, Weel H, van Dijk CN, et al. Surgical versus conservative treatment for high-risk stress fractures of the lower leg (anterior tibial cortex, navicular and fifth metatarsal base): a systematic review. Br J Sports Med 2015;49:370-6. [Crossref] [PubMed]
- Astur DC, Zanatta F, Arliani GG, et al. Stress fractures: definition, diagnosis and treatment. Rev Bras Ortop 2015;51:3-10. [Crossref] [PubMed]
- Swenson EJ, DeHaven KE, Sebastianelli WJ, et al. The effect of a pneumatic leg brace on return to play in athletes with tibial stress fractures. Am J Sports Med 1997;25:322-8. [Crossref] [PubMed]
- Mayr E, Nogler M, Benedetti MG, et al. A prospective randomized assessment of earlier functional recovery in THA patients treated by minimally invasive direct anterior approach: a gait analysis study. Clin Biomech (Bristol, Avon) 2009;24:812-8. [Crossref] [PubMed]
- Schandelmaier S, Kaushal A, Lytvyn L, et al. Low intensity pulsed ultrasound for bone healing: systematic review of randomized controlled trials. BMJ 2017;356:j656. [PubMed]
- Moretti B, Notarnicola A, Garofalo R, et al. Shock waves in the treatment of stress fractures. Ultrasound Med Biol 2009;35:1042-9. [Crossref] [PubMed]
- Goolsby MA, Boniquit N. Bone Health in Athletes. Sports Health 2017;9:108-17. [Crossref] [PubMed]
- Silverman SL. Nasal calcitonin. Endocrine 1997;6:199-202. [Crossref] [PubMed]
- Rizzoli R, Sigaud A, Azria M, et al. Nasal salmon calcitonin blunts bone microstructure alterations in healthy postmenopausal women. Osteoporos Int 2015;26:383-93. [Crossref] [PubMed]
- Cobb KL, Bachrach LK, Sowers M, et al. The effect of oral contraceptives on bone mass and stress fractures in female runners. Med Sci Sports Exerc 2007;39:1464-73. [Crossref] [PubMed]
- Joy E. Is the pill the answer for patients with the female athlete triad? Curr Sports Med Rep 2012;11:54-5. [Crossref] [PubMed]
- Brajic TS, Berger C, Schlammerl K, et al. Combined hormonal contraceptives use and bone mineral density changes in adolescent and young women in a prospective population-based Canada-wide observational study. J Musculoskelet Neuronal Interact 2018;18:227-36. [PubMed]
- Pikkarainen E, Lehtonen-Veromaa M, Möttönen T, et al. Estrogen-progestin contraceptive use during adolescence prevents bone mass acquisition: a 4-year follow-up study. Contraception 2008;78:226-31. [Crossref] [PubMed]
- Miller TL, Jamieson M, Everson S, et al. Expected Time to Return to Athletic Participation After Stress Fracture in Division I Collegiate Athletes. Sports Health 2018;10:340-4. [Crossref] [PubMed]
- Arendt E, Agel J, Heikes C, et al. Stress injuries to bone in college athletes: a retrospective review of experience at a single institution. Am J Sports Med 2003;31:959-68. [Crossref] [PubMed]
- Dobrindt O, Hoffmeyer B, Ruf J, et al. Estimation of return-to-sports-time for athletes with stress fracture - an approach combining risk level of fracture site with severity based on imaging. BMC Musculoskelet Disord 2012;13:139. [Crossref] [PubMed]
Cite this article as: Bishop ME, Ahlmen A, Rosendorf J, Erickson BJ, Cohen S. Bone stress injuries in female athletes. Ann Joint 2021;6:37.