Using Spheroids as Building Blocks Towards 3D Bioprinting of Tumor Microenvironment
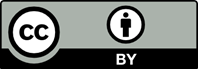
Cancer still ranks as a leading cause of mortality worldwide. Although considerable efforts have been dedicated to anticancer therapeutics, progress is still slow, partially due to the absence of robust prediction models. Multicellular tumor spheroids, as a major three-dimensional (3D) culture model exhibiting features of avascular tumors, gained great popularity in pathophysiological studies and high throughput drug screening. However, limited control over cellular and structural organization is still the key challenge in achieving in vivo like tissue microenvironment. 3D bioprinting has made great strides toward tissue/organ mimicry, due to its outstanding spatial control through combining both cells and materials, scalability, and reproducibility. Prospectively, harnessing the power from both 3D bioprinting and multicellular spheroids would likely generate more faithful tumor models and advance our understanding on the mechanism of tumor progression. In this review, the emerging concept on using spheroids as a building block in 3D bioprinting for tumor modeling is illustrated. We begin by describing the context of the tumor microenvironment, followed by an introduction of various methodologies for tumor spheroid formation, with their specific merits and drawbacks. Thereafter, we present an overview of existing 3D printed tumor models using spheroids as a focus. We provide a compilation of the contemporary literature sources and summarize the overall advancements in technology and possibilities of using spheroids as building blocks in 3D printed tissue modeling, with a particular emphasis on tumor models. Future outlooks about the wonderous advancements of integrated 3D spheroidal printing conclude this review
1. Sung H, Ferlay J, Siegel RL, et al., 2021, Global Cancer Statistics 2020: GLOBOCAN Estimates of Incidence and Mortality Worldwide for 36 Cancers in 185 Countries. rtdCA Cancer J Clin, 71:209–49. https://doi.org/10.3322/caac.21660.
2. Jackson SJ, Thomas GJ, 2017, Human Tissue Models in Cancer Research: Looking Beyond the Mouse. Dis Model Mech, 10:939–42. https://doi.org/10.1242/dmm.031260
3. Yoshida GJ, 2020, Applications of Patient-derived Tumor Xenograft Models and Tumor Organoids. J Hematol Oncol,13: 4. https://doi.org/10.1186/s13045-019-0829-z
4. Goto T, 2020, Patient-Derived Tumor Xenograft Models: Toward the Establishment of Precision Cancer Medicine. J Pers Med, 10:64. https://doi.org/10.3390/jpm10030064
5. Lai Y, Wei X, Lin S, et al., 2017, Current Status and Perspectives of Patient-derived Xenograft Models in Cancer Research. J Hematol Oncol, 10:106. https://doi.org/10.1186/s13045-017-0470-7
6. Ozturk MS, Lee VK, Zou H, et al., 2020, High-resolution Tomographic Analysis of In Vitro 3D Glioblastoma Tumor Model under Long-term Drug Treatment. Sci Adv, 6:eaay7513. https://doi.org/10.1126/sciadv.aay7513
7. Rijal G, Li W, 2017, A Versatile 3D Tissue Matrix Scaffold System for Tumor Modeling and Drug Screening. Sci Adv, 3:e1700764. https://doi.org/10.1126/sciadv.1700764
8. Ferreira LP, Gaspar VM, Mano JF, 2018, Design of Spherically Structured 3D In Vitro Tumor Models Advances and Prospects. Acta Biomater, 75:11–34. https://doi.org/10.1016/j.actbio.2018.05.034
9. Stock K, Estrada MF, Vidic S, et al., 2016, Graeser, Capturing Tumor Complexity In Vitro: Comparative Analysis of 2D and 3D Tumor Models for Drug Discovery. Sci Rep., 6:28951. https://doi.org/10.1038/srep28951
10. Laschke MW, Menger MD, 2017, Life is 3D: Boosting Spheroid Function for Tissue Engineering. Trends Biotechnol, 35:133–44. https://doi.org/10.1016/j.tibtech.2016.08.004
11. Cui X, Hartanto Y, Zhang H, 2017, Advances in Multicellular Spheroids Formation. J R Soc Interface, 14:20160877. https://doi.org/10.1098/rsif.2016.0877
12. Achilli TM, Meyer J, Morgan JR, 2012, Advances in the Formation, Use and Understanding of Multi-cellular Spheroids. Expert Opin Biol Ther, 12:1347–60. https://doi.org/10.1517/14712598.2012.707181
13. Nunes AS, Barros AS, Costa EC, et al., 2019, 3D Tumor Spheroids as In Vitro Models to Mimic In Vivo Human Solid Tumors Resistance to Therapeutic Drugs. Biotechnol Bioeng, 116:206–26. https://doi.org/10.1002/bit.26845
14. Edmondson R, Broglie JJ, Adcock AF, et al., 2014, Three dimensional Cell Culture Systems and their Applications in Drug Discovery and Cell-based Biosensors. Assay Drug Dev Technol., 12:207–18. https://doi.org/10.1089/adt.2014.573
15. Zhuang P, Sun AX, An J, et al., 2018, 3D Neural Tissue Models: From Spheroids to Bioprinting. Biomaterials, 154:113–33. https://doi.org/10.1016/j.biomaterials.2017.10.002
16. Zhuang P, An J, Chua CK, et al., 2020, Bioprinting of 3D In Vitro Skeletal Muscle Models: A Review. Mater Des, 193:108794. https://doi.org/10.1016/j.matdes.2020.108794
17. Shin YJ, Shafranek RT, Tsui JH, et al., 2021, 3D Bioprinting of Mechanically Tuned Bioinks Derived from Cardiac Decellularized Extracellular Matrix. Acta Biomater, 119:75–88. https://doi.org/10.1016/j.actbio.2020.11.006
18. Ma X, Liu J, Zhu W, et al., 2018, 3D Bioprinting of Functional Tissue Models for Personalized Drug Screening and In Vitro Disease Modeling. Adv Drug Deliv Rev, 132:235–51. https://doi.org/10.1016/j.addr.2018.06.011
19. Kang Y, Datta P, Shanmughapriya S, et al., 2020, 3D Bioprinting of Tumor Models for Cancer Research. ACS Appl Bio Mater, 3:5552–73. https://doi.org/10.1021/acsabm.0c00791
20. Zhang YS, Duchamp M, Oklu R, et al., 2016, Bioprinting the Cancer Microenvironment. ACS Biomater Sci Eng, 2:1710–21. https://doi.org/10.1021/acsbiomaterials.6b00246
21. Albritton JL, Miller JS, 2017, 3D Bioprinting: Improving In Vitro Models of Metastasis with Heterogeneous Tumor Microenvironments. Dis Model Mech, 10:3–14. https://doi.org/10.1242/dmm.025049
22. Tang M, Rich JN, Chen S, 2021, Biomaterials and 3D Bioprinting Strategies to Model Glioblastoma and the Blood Brain Barrier. Adv Mater, 33:2004776. https://doi.org/10.1002/adma.202004776
23. Wang M, Zhao J, Zhang L, et al., 2017, Role of Tumor Microenvironment in Tumorigenesis. J Cancer, 8:761–73. https://doi.org/10.7150/jca.17648
24. Baghban R, Roshangar L, Jahanban-Esfahlan R, et al., 2020, Tumor Microenvironment Complexity and Therapeutic Implications at a Glance. Cell Commun Signal, 18:59. https://doi.org/10.1186/s12964-020-0530-4
25. Winkler J, Abisoye-Ogunniyan A, Metcalf KJ, et al., 2020, Concepts of Extracellular Matrix Remodelling in Tumour Progression and Metastasis. Nat Commun, 11:5120. https://doi.org/10.1038/s41467-020-18794-x
26. Anderson NM, Simon MC, 2020, The Tumor Microenvironment. Curr Biol, 30:R921–R925. https://doi.org/10.1016/j.cub.2020.06.081
27. Cuiffo BG, Karnoub AE, 2012, Mesenchymal Stem Cells in Tumor Development: Emerging Roles and Concepts. Cell Adh Migr, 46:220–30. https://doi.org/10.4161/cam.20875
28. Ribeiro AL, Okamoto OK, 2015, Combined Effects of Pericytes in the Tumor Microenvironment. Stem Cells Int, 2015:868475. https://doi.org/10.1155/2015/868475
29. Nagl L, Horvath L, Pircher A, et al., 2020, Tumor Endothelial Cells (TECs) as Potential Immune Directors of the Tumor Microenvironment New Findings and Future Perspectives. Front Cell Dev Biol, 8:766. https://doi.org/10.3389/fcell.2020.00766
30. Wullkopf L, West AK, Leijnse N, et al., 2018, Cancer Cells’ Ability to Mechanically Adjust to Extracellular Matrix Stiffness Correlates with their Invasive Potential. Mol Biol Cell, 29:2378–85. https://doi.org/10.1091/mbc.E18-05-0319
31. Kalli M, Stylianopoulos T, 2018, Defining the Role of Solid Stress and Matrix Stiffness in Cancer Cell Proliferation and Metastasis. Front Oncol, 8:55. https://doi.org/10.3389/fonc.2018.00055
32. Bahcecioglu G, Basara G, Ellis BW, et al., 2020, Breast Cancer Models: Engineering the Tumor Microenvironment. Acta Biomater, 106:1-21. https://doi.org/10.1016/j.actbio.2020.02.006
33. Wang C, Sinha S, Jiang X, et al., 2021, Matrix Stiffness Modulates Patient-Derived Glioblastoma Cell Fates in Three-Dimensional Hydrogels. Tissue Eng Part A, 27:390–401. https://doi.org/10.1089/ten.TEA.2020.0110
34. Muz B, de la Puente P, Azab F, et al., 2015, The Role of Hypoxia in Cancer Progression, Angiogenesis, Metastasis, and Resistance to Therapy. Hypoxia (Auckland, NZ), 3:83–92. https://doi.org/10.2147/HP.S93413
35. Vajda J, Milojević M, Maver U, et al., 2021, Microvascular Tissue Engineering a Review. Biomedicines, 9:589. https://doi.org/10.3390/biomedicines9060589
36. Chen L, Endler A, Shibasaki F, 2009, Hypoxia and Angiogenesis: Regulation of Hypoxia-inducible Factors Via Novel Binding Factors. Exp Mol Med, 41:849–57. https://doi.org/10.3858/emm.2009.41.12.103
37. Peela N, Truong D, Saini H, et al., 2017, Advanced Biomaterials and Microengineering Technologies to Recapitulate the Stepwise Process of Cancer Metastasis. Biomaterials, 133:176–207. https://doi.org/10.1016/j.biomaterials.2017.04.017
38. Oliveira MB, Neto AI, Correia CR, et al., 2014, Superhydrophobic Chips for Cell Spheroids High-Throughput Generation and Drug Screening. ACS Appl Mater Interfaces, 6:9488–95. https://doi.org/10.1021/am5018607
39. Tung YC, Hsiao AY, Allen SG, et al., 2011, High-throughput 3D Spheroid Culture and Drug Testing Using a 384 Hanging Drop Array. Analyst, 136:473–8. https://doi.org/10.1039/c0an00609b
40. Zhao L, Xiu J, Liu Y, et al., 2019, A 3D Printed Hanging Drop Dripper for Tumor Spheroids Analysis Without Recovery. Sci Rep, 9:19717. https://doi.org/10.1038/s41598-019-56241-0
41. Massai D, Isu G, Madeddu D, et al., A Versatile Bioreactor for Dynamic Suspension Cell Culture. Application to the Culture of Cancer Cell Spheroids. PLoS One, 11:e0154610. https://doi.org/10.1371/journal.pone.0154610
42. Franchi-Mendes T, Lopes N, Brito C, 2021, Heterotypic Tumor Spheroids in Agitation-Based Cultures: A Scaffold-Free Cell Model That Sustains Long-Term Survival of Endothelial Cells. Front Bioeng Biotechnol, 9:447. https://doi.org/10.3389/fbioe.2021.649949
43. Costa EC, de Melo-Diogo D, Moreira AF, et al., 2018, Spheroids Formation on Non-Adhesive Surfaces by Liquid Overlay Technique: Considerations and Practical Approaches. Biotechnol J, 13:417. https://doi.org/10.1002/biot.201700417
44. Lee JM, Park DY, Yang L, et al., 2018, Generation of Uniform-sized Multicellular Tumor Spheroids Using Hydrogel Microwells for Advanced Drug Screening. Sci Rep, 8:17145. https://doi.org/10.1038/s41598-018-35216-7
45. Tu TY, Wang Z, Bai J, et al, 2014, Rapid Prototyping of Concave Microwells for the Formation of 3D Multicellular Cancer Aggregates for Drug Screening. Adv Healthc Mater, 3:609–16. https://doi.org/10.1002/adhm.201300151
46. Kim JA, Choi JH, Kim M, et al, 2013, High-throughput Generation of Spheroids Using Magnetic Nanoparticles for Three-dimensional Cell Culture. Biomaterials, 34:8555–63. https://doi.org/10.1016/j.biomaterials.2013.07.056
47. Chen K, Wu M, Guo F, et al., 2016, Rapid Formation of Size-controllable Multicellular Spheroids Via 3D Acoustic Tweezers. Lab Chip, 16:2636–43. https://doi.org/10.1039/C6LC00444J
48. Sebastian A, Buckle AM, Markx GH, 2007, Tissue Engineering with Electric Fields: Immobilization of Mammalian Cells in multilayer Aggregates Using Dielectrophoresis. Biotechnol Bioeng, 98:694–700. https://doi.org/10.1002/bit.21416
49. Wu Z, Gong Z, Ao Z, et al., 2020, Rapid Microfluidic Formation of Uniform Patient-Derived Breast Tumor Spheroids. ACS Appl Bio Mater, 3:6273–83. https://doi.org/10.1021/acsabm.0c00768
50. Ruppen J, Wildhaber FD, Strub C, et al., 2015, Towards Personalized Medicine: Chemosensitivity Assays of Patient Lung Cancer Cell Spheroids in a Perfused Microfluidic Platform. Lab Chip, 15:3076–85. https://doi.org/10.1039/C5LC00454C
51. Kingsley DM, Roberge CL, Rudkouskaya A, et al., 2019, Laser-based 3D Bioprinting for Spatial and Size Control of Tumor Spheroids and Embryoid Bodies. Acta Biomater, 95:357–70. https://doi.org/10.1016/j.actbio.2019.02.014
52. Kuo CT, Wang JY, Lin YF, et al, 2017, Three-dimensional Spheroid Culture Targeting Versatile Tissue Bioassays Using a PDMS-based Hanging Drop Array. Sci Rep, 7:4363. https://doi.org/10.1038/s41598-017-04718-1
53. Gao B, Jing C, Ng K, et al., 2019, Fabrication of Three dimensional Islet Models by the Geometry-controlled Hanging-drop Method. Acta Mech Sin, 35:329–37. https://doi.org/10.1007/s10409-019-00856-z
54. Ware MJ, Colbert K, Keshishian V, et al., 2016, Generation of Homogenous Three-Dimensional Pancreatic Cancer Cell Spheroids Using an Improved Hanging Drop Technique. Tissue Eng Part C Methods, 22:312–21. https://doi.org/10.1089/ten.TEC.2015.0280
55. Vignesh RA, Kumari S, Poddar P, et al., 2020, Poly(Nisopropylacrylamide)-Based Polymers as Additive for Rapid Generation of Spheroid via Hanging Drop Method. Macromol Biosci, 20:2000180. https://doi.org/10.1002/mabi.202000180
56. He H, He Q, Xu F, et al., 2019, Dynamic Formation of Cellular Aggregates of Chondrocytes and Mesenchymal Stem Cells in Spinner Flask. Cell Prolif, 52:e12587. https://doi.org/10.1111/cpr.12587
57. Shi W, Kwon J, Huang Y, et al., 2018, Facile Tumor Spheroids Formation in Large Quantity with Controllable Size and High Uniformity. Sci Rep, 8:6837. https://doi.org/10.1038/s41598-018-25203-3
58. Lancaster MA, Knoblich JA, 2014, Generation of Cerebral Organoids from Human Pluripotent Stem Cells. Nat Protoc, 9:2329–40. https://doi.org/10.1038/nprot.2014.158
59. Lei X, Ning L, Cao Y, et al., 2011, NASA-Approved Rotary Bioreactor Enhances Proliferation of Human Epidermal Stem Cells and Supports Formation of 3D Epidermis-Like Structure. PLoS One, 6:e26603. https://doi.org/10.1371/journal.pone.0026603
60. Salehi-Nik N, Amoabediny G, Pouran B, et al., 2013, Engineering Parameters in Bioreactor’s Design: A Critical Aspect in Tissue Engineering. Biomed Res Int, 2013:762132. https://doi.org/10.1155/2013/762132
61. Lei KF, Lin BY, Tsang NM, 2017, Real-time and Label-free Impedimetric Analysis of the Formation and Drug Testing of Tumor Spheroids Formed via the Liquid Overlay Technique. RSC Adv, 7:13939–46. https://doi.org/10.1039/C7RA00209B
62. Gaskell H, Sharma P, Colley HE, et al., 2016, Characterization of a Functional C3A Liver Spheroid Model. Toxicol Res (Camb), 5:1053–65. https://doi.org/10.1039/c6tx00101g
63. Costa EC, Gaspar VM, Coutinho P, et al., 2014, Optimization of Liquid Overlay Technique to Formulate Heterogenic 3D Co-cultures Models. Biotechnol Bioeng, 111:1672–85. https://doi.org/10.1002/bit.25210
64. Chen YC, Lou X, Zhang Z, et al., 2015, High-Throughput Cancer Cell Sphere Formation for Characterizing the Efficacy of Photo Dynamic Therapy in 3D Cell Cultures. Sci Rep, 5:12175. https://doi.org/10.1038/srep12175
65. Kim JH, Lim IR, Joo HJ, et al., 2015, Sphere Formation of Adipose Stem Cell Engineered by Poly-2-hydroxyethyl Methacrylate Induces In Vitro Angiogenesis through Fibroblast Growth Factor 2. Biochem Biophys Res Commun, 468:372–9. https://doi.org/10.1016/j.bbrc.2015.10.083
66. Lawrenson K, Grun B, Gayther SA, 2012, Heterotypic Three-dimensional In Vitro Modeling of Stromal-epithelial Interactions during Ovarian Cancer Initiation and Progression. J Vis Exp, 66:e4206. https://doi.org/10.3791/4206
67. Phung YT, Barbone D, Broaddus VC, et al., 2011, Rapid Generation of In Vitro Multicellular Spheroids for the Study of Monoclonal Antibody Therapy. J Cancer, 2:507–14. https://doi.org/10.7150/jca.2.507
68. Kuroda Y, Wakao S, Kitada M, et al., 2013, Isolation, Culture and Evaluation of Multilineage-differentiating Stress enduring (Muse) cells. Nat Protoc, 8:1391–415. https://doi.org/10.1038/nprot.2013.076
69. Ivascu A, Kubbies M, 2006, Rapid Generation of Single tumor Spheroids for High-throughput Cell Function and toxicity Analysis. J Biomol Screen, 11:922–32. https://doi.org/10.1177/1087057106292763
70. Bilandzic M, Stenvers KL, 2014, Assessment of Ovarian Cancer Spheroid Attachment and Invasion of Mesothelial Cells in Real Time. J Vis Exp, 87:51655. https://doi.org/10.3791/51655
71. Huang Z, Yu P, Tang J, 2020, Characterization of Triple-Negative Breast Cancer MDA-MB-231 Cell Spheroid Model. Onco Targets Ther, 13:5395–405. https://doi.org/10.2147/OTT.S249756
72. Fan Y, Avci NG, Nguyen DT, et al., 2015, Engineering a High-Throughput 3-D In Vitro Glioblastoma Model. IEEE J Transl Eng Health Med, 3:4300108. https://doi.org/10.1109/JTEHM.2015.2410277
73. Lee D, Pathak S, Jeong JH, 2019, Design and Manufacture of 3D Cell Culture Plate for Mass Production of Cell-Spheroids. Sci Rep, 9:13976. https://doi.org/10.1038/s41598-019-50186-0
74. Gong X, Lin C, Cheng J, et al., 2015, Generation of Multicellular Tumor Spheroids with Microwell-Based Agarose Scaffolds for Drug Testing. PLoS One, 10:e0130348. https://doi.org/10.1371/journal.pone.0130348
75. Chao C, Ngo LP, Engelward BP, 2020, Spheroid Chip: Patterned Agarose Microwell Compartments Harboring HepG2 Spheroids are Compatible with Genotoxicity Testing. ACS Biomater Sci Eng, 6:2427–39. https://doi.org/10.1021/acsbiomaterials.9b01951
76. Mirab F, Kang YJ, Majd S, 2019, Preparation and Characterization of Size-controlled Glioma Spheroids Using Agarose hydrogel Microwells. PLoS One, 14:e0211078. https://doi.org/10.1371/journal.pone.0211078
77. Thomsen AR, Aldrian C, Bronsert P, et al., 2018, A Deep Conical Agarose Microwell Array for Adhesion Independent Three-dimensional Cell Culture and Dynamic Volume Measurement. Lab Chip, 18:179–89. https://doi.org/10.1039/C7LC00832E
78. Desroches BR, Zhang P, Choi BR, et al., 2012, Functional Scaffold-free 3-D Cardiac Microtissues: A Novel Model for the Investigation of Heart Cells. Am J Physiol Heart Circ Physiol, 302:H2031–42. https://doi.org/10.1152/ajpheart.00743.2011
79. Henslee EA, Dunlop CM, de Mel CM, et al., 2020, DEP-Dots for 3D Cell Culture: Low-cost, High-repeatability, Effective 3D Cell Culture in Multiple Gel Systems. Sci Rep, 10:14603. https://doi.org/10.1038/s41598-020-71265-7
80. Albrecht DR, Tsang VL, Sah RL, et al., Photo- and electropatterning of hydrogel-encapsulated living cell arrays. Lab Chip, 5:111–8. https://doi.org/10.1039/b406953f
81. Agarwal S, Sebastian A, Forrester LM, et al., 2012, Formation of Embryoid Bodies Using Dielectrophoresis. Biomicrofluidics, 6:24101–11. https://doi.org/10.1063/1.3699969
82. Jafari J, Han X, Palmer J, et al., 2019, Remote Control in Formation of 3D Multicellular Assemblies Using Magnetic Forces. ACS Biomater Sci Eng, 5:2532–42. https://doi.org/10.1021/acsbiomaterials.9b00297
83. Urbanczyk M, Zbinden A, Layland SL, et al., Controlled Heterotypic Pseudo-Islet Assembly of Human β-Cells and Human Umbilical Vein Endothelial Cells Using Magnetic Levitation. Tissue Eng Part A, 26:387–99. https://doi.org/10.1089/ten.TEA.2019.0158
84. Lewis NS, El Lewis E, Mullin M, et al., 2017, Magnetically levitated mesenchymal stem cell spheroids cultured with a collagen gel maintain phenotype and quiescence. J Tissue Eng, 8:2041731417704428. https://doi.org/10.1177/2041731417704428
85. Tseng H, Gage JA, Shen T, et al., 2015, A Spheroid Toxicity Assay Using Magnetic 3D Bioprinting and Real-time Mobile Device-based Imaging. Sci Rep, 5:13987. https://doi.org/10.1038/srep13987
86. Wu M, Ozcelik A, Rufo J, et al., 2019, Acoustofluidic Separation of Cells and Particles. Microsyst Nanoeng, 5:32. https://doi.org/10.1038/s41378-019-0064-3
87. Chen B, Wu Y, Ao Z, et al., 2019, High-throughput Acoustofluidic Fabrication of Tumor Spheroids. Lab Chip, 19:1755–63. https://doi.org/10.1039/C9LC00135B
88. Ota H, Miki N, 2011, Microfluidic Experimental Platform for Producing Size-controlled Three-dimensional Spheroids. Sens Actuat A Phys, 169:266–73. https://doi.org/10.1016/j.sna.2011.03.051
89. Hong S, Hsu HJ, Kaunas R, et al., 2012, Collagen Microsphere Production on a Chip. Lab Chip, 12:3277–80. https://doi.org/10.1039/C2LC40558J
90. Damiati S, Kompella UB, Damiati SA, et al., 2018, Microfluidic Devices for Drug Delivery Systems and Drug Screening. Genes (Basel), 9:103. https://doi.org/10.3390/genes9020103
91. McMillan KS, McCluskey AG, Sorensen A, et al., 2016, Emulsion Technologies for Multicellular Tumour Spheroid Radiation Assays. Analyst, 141:100–10. https://doi.org/10.1039/c5an01382h
92. Lee JM, Choi JW, Ahrberg CD, et al., 2020, Generation of Tumor Spheroids Using a Droplet-based Microfluidic Device for Photothermal Therapy. Microsyst Nanoeng, 6:52. https://doi.org/10.1038/s41378-020-0167-x
93. Lee D, Cha C, 2018, The Combined Effects of Co-Culture and Substrate Mechanics on 3D Tumor Spheroid Formation within Microgels Prepared via Flow-Focusing Microfluidic Fabrication. Pharmaceutics, 10:229. https://doi.org/10.3390/pharmaceutics10040229
94. Cui X, Liu Y, Hartanto Y, et al., 2016, Multicellular Spheroids Formation and Recovery in Microfluidics-generated Thermo responsive Microgel Droplets. Colloid Interface Sci Commun, 14:4–7. https://doi.org/10.1016/j.colcom.2016.09.001
95. Zeng W, Xiang D, Fu H, 2019, Prediction of Droplet Production Speed by Measuring the Droplet Spacing Fluctuations in a Flow-Focusing Microdroplet Generator. Micromachines, 10:812. https://doi.org/10.3390/mi10120812
96. Kong T, Wu J, Yeung KW, et al., 2013, Microfluidic Fabrication of Polymeric Core-shell Microspheres for Controlled Release Applications. Biomicrofluidics, 7:44128. https://doi.org/10.1063/1.4819274
97. Tran TM, Lan F, Thompson CS, et al., 2013, From Tubes to Drops: Droplet-based Microfluidics for Ultrahigh-throughput Biology. J Phys D Appl Phys, 46:114004. https://doi.org/10.1088/0022-3727/46/11/114004
98. Chan HF, Zhang Y, Ho YP, et al., 2013, Rapid Formation of Multicellular Spheroids in Double-emulsion Droplets with Controllable Microenvironment. Sci Rep, 3:3462. https://doi.org/10.1038/srep03462
99. Kim C, Chung S, Kim YE, et al., 2011, Generation of Core shell Microcapsules with Three-dimensional Focusing Device for Efficient Formation of Cell Spheroid. Lab Chip, 11:246–52. https://doi.org/10.1039/C0LC00036A
100. Grist SM, Nasseri SS, Laplatine L, et al., 2019, Long-term Monitoring in a Microfluidic System to Study Tumour Spheroid Response to Chronic and Cycling Hypoxia. Sci Rep, 9:17782. https://doi.org/10.1038/s41598-019-54001-8
101. Wang Y, Zhao L, Tian C, et al., 2015, Geometrically Controlled Preparation of Various Cell Aggregates by Droplet-based Microfluidics. Anal Methods, 7:10040–51. https://doi.org/10.1039/C5AY02466H
102. Sun Q, Tan SH, Chen Q, et al., 2018, Microfluidic Formation of Coculture Tumor Spheroids with Stromal Cells As a Novel 3D Tumor Model for Drug Testing. ACS Biomater Sci Eng, 4:4425–33. https://doi.org/10.1021/acsbiomaterials.8b00904
103. Yazdi SR, Shadmani A, Bürgel SC, et al., Adding the “Heart” to Hanging Drop Networks for Microphysiological Multitissue Experiments. Lab Chip, 15:4138–47. https://doi.org/10.1039/c5lc01000d
104. Frey O, Misun PM, Fluri DA, et al., 2014, Reconfigurable Microfluidic Hanging Drop Network for Multi-tissue Interaction and Analysis. Nat Commun, 5:4250. https://doi.org/10.1038/ncomms5250
105. Wu HW, Hsiao YH, Chen CC, et al., 2016, A PDMS-Based Microfluidic Hanging Drop Chip for Embryoid Body Formation. Molecules, 21:882. https://doi.org/10.3390/molecules21070882
106. Dadgar N, Gonzalez-Suarez AM, Fattahi P, et al., 2020, A Microfluidic Platform for Cultivating Ovarian Cancer Spheroids and Testing their Responses to Chemotherapies. Microsyst Nanoeng, 6:93. https://doi.org/10.1038/s41378-020-00201-6
107. Chen SY, Hung PJ, Lee PJ, 2011, Microfluidic Array for Three-dimensional Perfusion Culture of Human Mammary Epithelial Cells. Biomed Microdev, 13:753–8. https://doi.org/10.1007/s10544-011-9545-3
108. Lee K, Kim C, Yang JY, et al., 2012, Gravity-oriented Microfluidic Device for Uniform and Massive Cell Spheroid Formation. Biomicrofluidics, 6:14114–47. https://doi.org/10.1063/1.3687409
109. Fiddes LK, Luk VN, Au SH, et al., 2012, Hydrogel Discs for Digital Microfluidics. Biomicrofluidics, 6:14112–211. https://doi.org/10.1063/1.3687381
110. Eydelnant IA, Li BB, Wheeler AR, 2014, Microgels ondemand. Nat Commun, 5:3355. https://doi.org/10.1038/ncomms4355
111. Aijian AP, Garrell RL, 2015, Digital Microfluidics for Automated Hanging Drop Cell Spheroid Culture. J Lab Autom, 20:283–95. https://doi.org/10.1177/2211068214562002
112. Vadivelu RK, Kamble H, Shiddiky MJ, et al., 2017, Microfluidic Technology for the Generation of Cell Spheroids and Their Applications. Micromachines, 8:94. https://doi.org/10.3390/mi8040094
113. Moshksayan K, Kashaninejad N, Warkiani ME, et al., 2018, Spheroids-on-a-chip: Recent Advances and Design Considerations in Microfluidic Platforms for Spheroid Formation and Culture. Sens Actuat B Chem, 263:151–76. https://doi.org/10.1016/j.snb.2018.01.223
114. Utama RH, Atapattu L, O’Mahony AP, et al., 2020, A 3D Bioprinter Specifically Designed for the High-Throughput Production of Matrix-Embedded Multicellular Spheroids. IScience, 23:101621. https://doi.org/10.1016/j.isci.2020.101621
115. Ling K, Huang G, Liu J, et al., 2015, Bioprinting-Based High-Throughput Fabrication of Three-Dimensional MCF-7 Human Breast Cancer Cellular Spheroids. Engineering, 1:269–74. https://doi.org/10.15302/J-ENG-2015062
116. Vinson BT, Sklare SC, Chrisey DB, 2017, Laser-based Cell Printing Techniques for Additive Biomanufacturing. Curr Opin Biomed Eng, 2:14–21. https://doi.org/10.1016/j.cobme.2017.05.005
117. Armon N, Greenberg E, Edri E, et al., 2021, Laser-Based Printing: From Liquids to Microstructures. Adv Funct Mater, 31:2008547. https://doi.org/10.1002/adfm.202008547
118. Hakobyan D, Médina C, Dusserre N, et al., 2020, Laser assisted 3D Bioprinting of Exocrine Pancreas Spheroid Models for Cancer Initiation Study. Biofabrication, 12:35001. https://doi.org/10.1088/1758-5090/ab7cb8
119. Boyer CJ, Ballard DH, Barzegar M, et al., 2018, High throughput Scaffold-free Microtissues through 3D Printing.
120. Swaminathan S, Clyne AM, 2020, Direct Bioprinting of 3D Multicellular Breast Spheroids onto Endothelial Networks. J Vis Exp, 165:61791. https://doi.org/10.3791/61791
121. Swaminathan S, Hamid Q, Sun W, et al., 2019, Bioprinting of 3D Breast Epithelial Spheroids for Human Cancer Models. Biofabrication, 11:25003. https://doi.org/10.1088/1758-5090/aafc49
122. Horder H, Lasheras MG, Grummel N, et al., 2021, Bioprinting and Differentiation of Adipose-Derived Stromal Cell Spheroids for a 3D Breast Cancer-Adipose Tissue Model. Cells, 10:803. https://doi.org/10.3390/cells10040803
123. Jakab K, Norotte C, Damon B, et al., 2008, Tissue Engineering by Self-assembly of Cells Printed into Topologically Defined Structures. Tissue Eng Part A, 14:413–21. https://doi.org/10.1089/tea.2007.0173
124. Campos DF, Lindsay CD, Roth JG, et al., 2020, Bioprinting Cell-and Spheroid-Laden Protein-Engineered Hydrogels as Tissue-on-Chip Platforms. Front Bioeng Biotechnol, 8:374. https://doi.org/10.3389/fbioe.2020.00374
125. Vinson BT, Phamduy TB, Shipman J, et al., 2017, Laser Direct-write Based Fabrication of a Spatially-defined, Biomimetic Construct as a Potential Model for Breast Cancer Cell Invasion into Adipose Tissue. Biofabrication, 9:25013. https://doi.org/10.1088/1758-5090/aa6bad
126. Chen K, Jiang E, Wei X, et al., 2021, The Acoustic Droplet Printing of Functional Tumor Microenvironments. Lab Chip, 21:1604–12. https://doi.org/10.1039/D1LC00003A
127. Moldovan NI, Hibino N, Nakayama K, 2017, Principles of the Kenzan Method for Robotic Cell Spheroid-Based Three-Dimensional Bioprinting. Tissue Eng Part B Rev, 23:237–44. https://doi.org/10.1089/ten.TEB.2016.0322
128. Arai K, Murata D, Verissimo AR, et al., 2018, Fabrication of Scaffold-free Tubular Cardiac Constructs Using a Bio-3D Printer. PLoS One, 13:e0209162. https://doi.org/10.1371/journal.pone.0209162
129. LaBarge W, Morales A, Pretorius D, et al., 2019, Scaffold-Free Bioprinter Utilizing Layer-By-Layer Printing of Cellular Spheroids. Micromachines, 10:570. https://doi.org/10.3390/mi10090570
130. Murata D, Arai K, Nakayama K, 2020, Scaffold-Free Bio-3D Printing Using Spheroids as “Bio-Inks” for Tissue (Re-) Construction and Drug Response Tests. Adv Healthc Mater, 9:e1901831. https://doi.org/10.1002/adhm.201901831
131. van Pel DM, Harada K, Song D, et al., 2018, Modelling Glioma Invasion Using 3D Bioprinting and Scaffold-free 3D Culture. J Cell Commun Signal, 12:723–30. https://doi.org/10.1007/s12079-018-0469-z
132. Kizawa H, Nagao E, Shimamura M, et al., 2017, Scaffold free 3D Bio-printed Human Liver Tissue Stably Maintains Metabolic Functions Useful for Drug Discovery. Biochem Biophys Reports, 10:186–91. https://doi.org/10.1016/j.bbrep.2017.04.004
133. Ip BC, Cui F, Tripathi A, et al., 2016, The Bio-gripper: A Fluid driven Micro-Manipulator of Living Tissue Constructs for Additive Bio-manufacturing. Biofabrication, 8:25015. https://doi.org/10.1088/1758-5090/8/2/025015
134. Blakely AM, Manning KL, Tripathi A, et al., 2015, Bio-Pick, Place, and Perfuse: A New Instrument for Three-Dimensional Tissue Engineering. Tissue Eng Part C Methods, 21:737–46. https://doi.org/10.1089/ten.TEC.2014.0439
135. Ayan B, Heo DN, Zhang Z, et al., 2020., Aspiration-assisted Bioprinting for Precise Positioning of Biologics. Sci Adv, 6:eaaw5111. https://doi.org/10.1126/sciadv.aaw5111
136. Ayan B, Celik N, Zhang Z, et al., 2020, Aspiration-assisted Freeform Bioprinting of Pre-fabricated Tissue Spheroids in a Yield-stress Gel. Commun Phys, 3:183. https://doi.org/10.1038/s42005-020-00449-4
137. Daly AC, Davidson MD, Burdick JA, 2021, 3D Bioprinting of High Cell-density Heterogeneous Tissue Models through Spheroid Fusion within Self-healing Hydrogels. Nat Commun, 12:753. https://doi.org/10.1038/s41467-021-21029-2
138. Norotte C, Marga FS, Niklason LE, et al., 2009, Scaffold-free Vascular Tissue Engineering Using Bioprinting. Biomaterials, 30:5910–7. https://doi.org/10.1016/j.biomaterials.2009.06.034
139. Bulanova EA, Koudan EV, Degosserie J, et al., 2017, Bioprinting of a Functional Vascularized Mouse Thyroid Gland Construct. Biofabrication, 9:34105. https://doi.org/10.1088/1758-5090/aa7fdd
140. Kozaki S, Moritoki Y, Furukawa T, et al., 2020, Additive Manufacturing of Micromanipulator Mounted on a Glass Capillary for Biological Applications. Micromachines, 11:174. https://doi.org/10.3390/mi11020174
141. Zhuang P, An J, Tan LP, et al., 2018, The Current Status of 3D Bioprinting for Neural Tissue Models. 2018:183–8. https://doi.org/10.25341/D4688F
142. Zhuang P, Ng WL, An J, et al., 2019, Layer-by-layer Ultraviolet Assisted Extrusion-based (UAE) Bioprinting of Hydrogel Constructs with High Aspect Ratio for Soft Tissue Engineering Applications. PLoS One, 14:e0216776. https://doi.org/10.1371/journal.pone.0216776
143. Li X, Liu B, Pei B, et al., 2020, Inkjet Bioprinting of Biomaterials. Chem Rev, 120:10793–833. https://doi.org/10.1021/acs.chemrev.0c00008
144. Koch L, Gruene M, Unger C, et al., 2013, Laser Assisted Cell Printing. Curr Pharm Biotechnol, 14:91–7.
145. Grigoryan B, Sazer DW, Avila A, et al., 2021, Development, Characterization, and Applications of Multi-Material Stereolithography Bioprinting. Sci Rep, 11:3171. https://doi.org/10.1038/s41598-021-82102-w
146. Matai I, Kaur G, Seyedsalehi A, et al., 2020, Progress in 3D Bioprinting Technology for Tissue/Organ Regenerative Engineering. Biomaterials, 226:119536. https://doi.org/10.1016/j.biomaterials.2019.119536
147. Murphy SV, De Coppi P, Atala A, 2020, Opportunities and Challenges of Translational 3D Bioprinting. Nat Biomed Eng, 4:370–80. https://doi.org/10.1038/s41551-019-0471-7
148. Cui X, Li J, Hartanto Y, et al., 2020, Advances in Extrusion 3D Bioprinting: A Focus on Multicomponent Hydrogel- Based Bioinks. Adv Healthc Mater, 9:1901648. https://doi.org/10.1002/adhm.201901648
149. Zhuang P, Greenberg Z, He M, 2021, Biologically Enhanced Starch Bio-Ink for Promoting 3D Cell Growth. Adv Mater Technol, 2021:2100551. https://doi.org/10.1002/admt.202100551
150. McCormack A, Highley CB, Leslie NR, et al., 2020, 3D Printing in Suspension Baths: Keeping the Promises of Bioprinting Afloat. Trends Biotechnol, 38:584–93. https://doi.org/10.1016/j.tibtech.2019.12.020
151. Hermida MA, Kumar JD, Schwarz D, et al., 2020, Three Dimensional In Vitro Models of Cancer: Bioprinting Multilineage Glioblastoma Models. Adv Biol Regul, 75:100658. https://doi.org/10.1016/j.jbior.2019.100658
152. Dai X, Liu L, Ouyang J, et al., 2017, Coaxial 3D Bioprinting of Self-assembled Multicellular Heterogeneous Tumor Fibers. Sci Rep, 7:1457. https://doi.org/10.1038/s41598-017-01581-y
153. Wang X, Li X, Dai X, et al., 2018, Coaxial Extrusion Bioprinted Shell-core Hydrogel Microfibers Mimic Glioma Microenvironment and Enhance the Drug Resistance of Cancer Cells. Colloids Surf B Biointerfaces, 171:291–9. https://doi.org/10.1016/j.colsurfb.2018.07.042
154. Yi HG, Jeong YH, Kim Y, et al., 2019, A Bioprinted Human glioblastoma-on-a-Chip for the Identification of Patient specific Responses to Chemoradiotherapy. Nat Biomed Eng, 3:509–19. https://doi.org/10.1038/s41551-019-0363-x
155. Wang X, Li X, Ding J, et al., 2021, 3D Bioprinted Glioma Microenvironment for Glioma Vascularization. J Biomed Mater Res Part A, 109:915–25. https://doi.org/10.1002/jbm.a.37082
156. Wang X, Li X, Dai X, et al., 2018, Bioprinting of Glioma Stem Cells Improves their Endotheliogenic Potential. Colloids Surf B Biointerfaces, 171:629–37. https://doi.org/10.1016/j.colsurfb.2018.08.006
157. Lee VK, Dai G, Zou H, et al., 2015, Generation of 3-D Glioblastoma-vascular Niche Using 3-D Bioprinting. In: 2015 41st Annual Northeast Biomedical Engineering Conference, p1-2. https://doi.org/10.1109/NEBEC.2015.7117111
158. Wang X, Zhang X, Dai X, et al., 2018, Tumor-like Lung Cancer Model Based on 3D Bioprinting. 3 Biotech, 8:501. https://doi.org/10.1007/s13205-018-1519-1
159. Zhou X, Zhu W, Nowicki M, et al., 2016, 3D Bioprinting a Cell-Laden Bone Matrix for Breast Cancer Metastasis Study. ACS Appl Mater Interfaces, 8:30017–26. https://doi.org/10.1021/acsami.6b10673
160. Zhu W, Holmes B, Glazer RI, et al., 2016, 3D Printed Nanocomposite Matrix for the Study of Breast Cancer Bone Metastasis. Nanomedicine, 12:69–79. https://doi.org/10.1016/j.nano.2015.09.010
161. Han S, Kim S, Chen Z, et al., 2020, 3D Bioprinted Vascularized Tumour for Drug Testing. Int J Mol Sci, 21:2993. https://doi.org/10.3390/ijms21082993
162. Smits IP, Blaschuk OW, Willerth SM, 2020, Novel N-cadherin Antagonist Causes Glioblastoma Cell Death in a 3D Bioprinted Co-culture Model. Biochem Biophys Res Commun, 529:162–8. https://doi.org/10.1016/j.bbrc.2020.06.001
163. Mironov V, Visconti RP, Kasyanov V, et al., 2009, Organ Printing: Tissue Spheroids as Building Blocks. Biomaterials, 30:2164–74. https://doi.org/10.1016/j.biomaterials.2008.12.084
164. Ahmad T, Shin HJ, Lee J, et al., 2018, Fabrication of In Vitro 3D Mineralized Tissue by Fusion of Composite Spheroids Incorporating Biomineral-coated Nanofibers and Human Adipose-derived Stem Cells. Acta Biomater, 74:464–77. https://doi.org/10.1016/j.actbio.2018.05.035
165. Kelm JM, Lorber V, Snedeker JG, et al., 2010, A Novel Concept for Scaffold-free Vessel Tissue Engineering: Self assembly of Microtissue Building Blocks. J Biotechnol, 148:46–55. https://doi.org/10.1016/j.jbiotec.2010.03.002
166. Birey F, Andersen J, Makinson CD, et al., 2017, Assembly of Functionally Integrated human Forebrain Spheroids. Nature, 545:54–9. https://doi.org/10.1038/nature22330
167. Kato-Negishi M, Morimoto Y, Onoe H, et al., 2013, Millimeter-Sized Neural Building Blocks for 3D Heterogeneous Neural Network Assembly. Adv Healthc Mater, 2:1564–70. https://doi.org/10.1002/adhm.201300052
168. Fleming PA, Argraves WS, Gentile C, et al., 2010, Fusion of Uniluminal Vascular Spheroids: A Model for Assembly of Blood Vessels. Dev Dyn, 239:398–406. https://doi.org/10.1002/dvdy.22161
169. Dean DM, Napolitano AP, Youssef J, et al., 2007, Rods, Tori, and Honeycombs: The Directed Self-assembly of Microtissues with Prescribed Microscale Geometries. FASEB J, 21:4005–12. https://doi.org/10.1096/fj.07-8710com
170. Luo J, Meng J, Gu Z, et al., 2019, Topography-Induced Cell Self-Organization from Simple to Complex Aggregates. Small, 15:1900030. https://doi.org/10.1002/smll.201900030
171. Olsen TR, Mattix B, Casco M, et al., 2015, Manipulation of Cellular Spheroid Composition and the Effects on Vascular Tissue Fusion. Acta Biomater, 13:188–98. https://doi.org/10.1016/j.actbio.2014.11.024
172. Mattix B, Olsen TR, Gu Y, et al., 2014, Biological Magnetic Cellular Spheroids as Building Blocks for Tissue Engineering. Acta Biomater, 10:623–9. https://doi.org/10.1016/j.actbio.2013.10.021
173. Whatley BR, Li X, Zhang N, et al., 2014, Magnetic directed Patterning of cell Spheroids. J Biomed Mater Res A, 102:1537–47. https://doi.org/10.1002/jbm.a.34797
174. Lin RZ, Chu WC, Chiang CC, et al., 2008, Magnetic Reconstruction of Three-Dimensional Tissues from Multicellular Spheroids. Tissue Eng Part C Methods, 14:197–205. https://doi.org/10.1089/ten.tec.2008.0061
175. Bratt-Leal AM, Kepple KL, Carpenedo RL, et al., 2011, Magnetic Manipulation and Spatial Patterning of Multicellular Stem Cell Aggregates. Integr Biol (Camb), 3:1224–32. https://doi.org/10.1039/c1ib00064k
176. Fayol D, Frasca G, Le Visage C, et al., 2013, Use of Magnetic Forces to Promote Stem Cell Aggregation During Differentiation, and Cartilage Tissue Modeling. Adv Mater, 25:2611–6. https://doi.org/10.1002/adma.201300342
177. Chen P, Güven S, Usta OB, et al., 2015, Biotunable Acoustic Node Assembly of Organoids. Adv Healthc Mater, 4:1937–43. https://doi.org/10.1002/adhm.201500279
178. Parfenov VA, Koudan EV, Krokhmal AA, et al., 2020, Biofabrication of a Functional Tubular Construct from Tissue Spheroids Using Magnetoacoustic Levitational Directed Assembly. Adv Healthc Mater, 9:2000721. https://doi.org/10.1002/adhm.202000721
179. Heinrich MA, Bansal R, Lammers T, et al., 2019, 3D-Bioprinted Mini-Brain: A Glioblastoma Model to Study Cellular Interactions and Therapeutics. Adv Mater, 31:e1806590. https://doi.org/10.1002/adma.201806590
180. Tang M, Xie Q, Gimple RC, et al., 2020, Three-dimensional Bioprinted Glioblastoma Microenvironments Model Cellular Dependencies and Immune Interactions. Cell Res., 30:833–53. https://doi.org/10.1038/s41422-020-0338-1
181. Weis SM, Cheresh DA, 2011, Tumor Angiogenesis: Molecular Pathways and Therapeutic Targets. Nat Med, 17:1359–70. https://doi.org/10.1038/nm.2537
182. Dey M, Ayan B, Yurieva M, et al., 2021, Studying Tumor Angiogenesis and Cancer Invasion in a Three-Dimensional Vascularized Breast Cancer Micro-Environment. Adv Biol, 2021:e2100090. https://doi.org/10.1002/adbi.202100090
183. Meng F, Meyer CM, Joung D, et al., 2019, 3D Bioprinted In Vitro Metastatic Models via Reconstruction of Tumor Microenvironments. Adv Mater, 31:e1806899. https://doi.org/10.1002/adma.201806899
184. Maloney E, Clark C, Sivakumar H, et al., 2020, Immersion Bioprinting of Tumor Organoids in Multi-Well Plates for Increasing Chemotherapy Screening Throughput. Micromachines, 11:208. https://doi.org/10.3390/mi11020208
185. Li Y, Zhang T, Pang Y, et al., 2019, 3D Bioprinting of Hepatoma Cells and application with microfluidics for pharmacodynamic test of Metuzumab. Biofabrication, 11:34102. https://doi.org/10.1088/1758-5090/ab256c
186. Sun L, Yang H, Wang Y, et al., 2020, Application of a 3D Bioprinted Hepatocellular Carcinoma Cell Model in Antitumor Drug Research. Front Oncol, 10:878. https://doi.org/10.3389/fonc.2020.00878
187. Zhang J, Chen F, He Z, et al., 2016, A Novel Approach for Precisely Controlled Multiple Cell Patterning in Microfluidic Chips by Inkjet Printing and the Detection of Drug Metabolism and Diffusion. Analyst, 141:2940–7. https://doi.org/10.1039/C6AN00395H
188. Xie F, Sun L, Pang Y, et al., 2021, Three-dimensional Bioprinting of Primary Human Hepatocellular Carcinoma for Personalized Medicine. Biomaterials, 265:120416. https://doi.org/10.1016/j.biomaterials.2020.120416
189. Skylar-Scott MA, Uzel SG, Nam LL, et al., 2019, Biomanufacturing of Organ-Specific Tissues with High Cellular Density and Embedded Vascular Channels. Sci Adv, 5:eaaw2459. https://doi.org/10.1126/sciadv.aaw2459
190. Lee J, Oh SJ, An SH, et al., 2020, Machine Learning-based Design Strategy for 3D Printable Bioink: Elastic Modulus and Yield Stress Determine Printability. Biofabrication, 12:35018. https://doi.org/10.1088/1758-5090/ab8707
191. Yin R, Luo Z, Zhuang P, et al., 2021, VirPreNet: A Weighted Ensemble Convolutional Neural Network for the Virulence Prediction of Influenza A Virus Using All Eight Segments. Bioinformatics, 37:737–43. https://doi.org/10.1093/bioinformatics/btaa901
192. Yin R, Zhou X, Rashid S, et al., 2020, HopPER: An Adaptive Model for Probability Estimation of Influenza Reassortment through Host Prediction. BMC Med Genomics, 13:9. https://doi.org/10.1186/s12920-019-0656-7
193. Lazzari G, Nicolas V, Matsusaki M, et al., 2018, Multicellular Spheroid Based on a Triple Co-culture: A Novel 3D Model to Mimic Pancreatic Tumor Complexity. Acta Biomater, 78:296–307. https://doi.org/10.1016/j.actbio.2018.08.008
194. Meier-Hubberten JC, Sanderson MP, 2019, Establishment and Analysis of a 3D Co-Culture Spheroid Model of Pancreatic Adenocarcinoma for Application in Drug Discovery. Methods Mol Biol, 1953:163–79. https://doi.org/10.1007/978-1-4939-9145-7_11
195. De Moor L, Merovci I, Baetens S, et al., 2018, Highthroughput Fabrication of Vascularized Spheroids for Bioprinting. Biofabrication, 10:35009. https://doi.org/10.1088/1758-5090/aac7e6
196. Noguchi R, Nakayama K, Itoh M, et al., 2016, Development of a Three-Dimensional Pre-vascularized Scaffold-free Contractile Cardiac Patch for Treating Heart Disease. J Heart Lung Transplant, 35:137–45. https://doi.org/10.1016/j.healun.2015.06.001