Abstract
In this paper the passive layer cracking process that occurs under tensile stresses and anodic polarization conditions on AA1050, AA5052, AA5056, and AA7005 aluminum alloys was examined by means of direct current (dc) technique, dynamic electrochemical impedance spectroscopy (DEIS), and acoustic emission (AE). Research revealed the advantage of DEIS and AE methods over the dc one. It was also possible to determine the exact moment of the passive layer cracking on investigated aluminum alloys.
Export citation and abstract BibTeX RIS
Aluminum-magnesium alloys (5xxx series) are widely used in a variety of applications in various branches of industry due to their excellent mechanical properties and corrosion resistance.1–11 Many of them strongly depend on magnesium content in the alloy. In general, mechanical properties increase proportionally to magnesium content in the range of .5 Corrosion resistance of aluminum alloys decreases with magnesium addition.9–12 Magnesium contents above
precipitate at grain boundaries and deteriorate alloy properties, leading to formation of relatively thick and friable surface oxides. Such passive films effectively cause reduced corrosion resistance, especially to stress corrosion cracking.10, 11, 13
Aluminum alloys as construction materials are subjected to tensile stresses in many cases. Rupture of the passive layer under tensile stresses conditions is considered as one of the most important aspects that causes faster dissolution of metal in aggressive corrosion environments due to exposure of a bare metal substrate to electrolyte, and so initiating the cracks due to stress-corrosion cracking.14, 15
Research involved with the stress-corrosion cracking is well-known and widely performed all around the world. Classical stress-corrosion cracking research is based on electrochemical and mechanical measurements, and metallographical studies.16, 17 The use of potentiostatic techniques developed the knowledge of the stress-corrosion cracking mechanism18–20 and allowed the determination of the stress-corrosion cracking characteristic potentials.16–19 Classical electrochemical impedance spectroscopy (EIS) also makes possible receiving information, which relates to the stress-corrosion cracking process, but only before and after the moment of cracking, when the investigated system has attained a steady state.21–23 Basic background of such measurements was widely discussed by Gabrielli,24 Macdonald,21 Mansfeld and Lorenz,25 and Stoynova et al.26 However, for now, detailed determination of the moment of the passive layer cracking in the time-strain domain was not possible by means of any electrochemical technique or mechanical test. Dynamic electrochemical impedance spectroscopy (DEIS) and acoustic emission (AE) techniques give such opportunity. Studies performed by Darowicki et al. on 304L stainless steel and AA5056 in chlorides solution confirm that fact.27–30
The aim of this paper was to compare the application of ac technique with the dc one to assess passive layer cracking on different aluminum alloys subjected to tensile stresses under anodic polarization. The authors focused on estimation of proper criterion concerning the moment of the passive layer cracking on the basis of dc and ac techniques as the selection methods used for determination of the initiation stage of the stress-corrosion cracking.
Experimental
Experiments performed during the research were composed of cyclic polarization measurements, ac measurements performed using DEIS and AE technique.
Detailed electrochemical measurements during the tensile test were conducted for AA5052 aluminum alloy, because it contains a relatively small addition of magnesium. However, this paper also contains the comparison of mechanical properties received from previous research carried out on AA1050 (Ref. 30) and AA5056 (Ref. 31) alloys with AA7005 alloy.
Examined samples were prepared in the form of bars according to the ASTM standard. Detailed chemical compositions of investigated materials are presented in Table I.
Table I. Chemical composition of examined aluminum alloys.
Chemical composition, ![]() | ||||||||
---|---|---|---|---|---|---|---|---|
Type of alloy | Al | Mg | Zn | Cr | Fe | Mn | Cu | Other |
AA1050 | min. 99.5 | max. 0.05 | – | – | – | max. 0.05 | max. 0.05 | Balance |
AA5052 | 95.7–97.7 | 2.2–2.8 | – | 0.15–0.35 | max. 0.4 | max. 0.05 | max. 0.05 | Balance |
AA5056 | 92.9–95.4 | 4.5–5.6 | – | 0.05–0.2 | max. 0.4 | 0.05–0.2 | max. 0.1 | Balance |
AA7005 | 91.0–94.7 | 1.0–1.8 | 4.0–5.0 | 0.06–0.2 | max. 0.4 | 0.2–0.7 | – | Balance |
Samples were mounted in a measurement cell and then in a tensile testing machine, MTS 81012. Tensile tests and samples preparations were performed according to the EN 10002-1 AC1 standard at room temperature with the MTS extensometer characterized by the measurement base of
. Scheme of the investigated specimens together with the measurement cell was the same as in the case of previous research.30, 31 Electrochemical measurements were carried out in a three-electrode cell, in which silver/silver chloride electrode was used as a reference electrode and the auxiliary electrode was made of platinum net.
Before the experiment's execution, investigated specimens were exposed to buffered solution (;
) for
to receive stable passive layer. Afterward, the measurements were performed in the same solution with additional content of
sodium chloride.
The rate of stress increment was equal to (according to the standard EN 10002-1
AC1) in order to detect such a rapid electrochemical process as the passive layer cracking. Additionally, electrochemical examinations were realized under tensile stresses in such a way that time of DEIS and AE measurements was correlated with the strain process. Total measurement time was equal to
. The measurement had been stopped when the sample was broken. Maximal recorded strain value for AA5052 was of 18% (0.18).
The measurement setup consisted of electrochemical measurement software Gamry International (the scanning rate in cyclic polarization measurements was equal to ), an ELPAN EP20A potentiostat, and a National Instruments PCI 6120 card that generated the perturbation signal, registered voltage perturbation, and current response signals. Impedance measurements were executed for the frequency range
. Average number of points per decade in the DEIS measurements was 5. Sampling frequency of
resulted from the measurement card settings being at our disposal. In the investigations the multisinusoidal signal composed of 20 sinusoids was utilized. Depending on applied frequency the amplitudes of sinusoidal signals ranged from 5 to
. References 32, 33 contain detailed description of this method.
Acoustic emission measurements were executed by means of the two-channel system, Mistras 2001. Analysis of obtained data was performed on the basis of Mistras standard software.
Results and Discussion
Investigations of passive layer present on the nonstressed AA5052 samples have been initiated with cyclic polarization measurements. In that way a proper cyclic polarization curve was recorded and is presented in Fig. 1.
Figure 1. Potentiodynamic cyclic polarization curve obtained for nonstressed AA5052 aluminum alloy in buffer solution ;
) with addition of
.
Executed measurements allowed us to conclude that the passive state occurred in a wide potential range between and
. Corrosion potential value of AA5052 alloy in
solution was established as
. Moreover, AA5052 alloy did not reveal any tendency for repassivation because the value of repassivation potential was lower than the corrosion potential. Based on the dc measurements, the range of anodic potentials used in further mechanical and electrochemical examinations has been selected as
to avoid the potential hazard of localized corrosion during the experiments.
Taking into consideration the defined electrochemical criterion, dc and ac measurements at potentiostatic conditions were conducted while monotonous tensile process took place simultaneously. Accordingly, an exemplary tensile curve for AA5052 alloy is depicted in Fig. 2. Even significant polarization did not have an effect on the recorded tensile curves.
Figure 2. Exemplary stress-strain dependence recorded for examined AA5052 alloy at .
Total breakdown of specimens was recorded at (0.18). Due to constant changes of strain in time the electrochemical examinations were related to the strain values.
Application of the DEIS method during the tensile tests for various anodic polarization made it possible to record sets of instantaneous impedance spectra reflecting the dynamic changes of the system as a function of strain (Fig. 3A, 3B, 3C and 3D). Impedance values drop together with increasing strain, and so reflect transition from passive into the active state. The decrease in impedance can be explained by the decay in barrier properties of the passive layer due to the cracking process. Additionally, the effect of applied anodic polarization can be observed in diagrams, particularly for higher strain values. However, by tracing the instantaneous changes in the system's impedance, it was not possible to determine precisely the threshold strain value related to the passive layer cracking.
Figure 3. DEIS diagrams recorded during the tensile process for examined AA5052 aluminum alloy at various potentials: (A) , (B)
, (C)
, (D)
.
From each impedance spectrum the dc current component was filtered. As a result the dependency between dc current and strain for different anodic potentials was obtained and is illustrated in Fig. 4. The initial values of dc current are in good agreement with the potentiodynamic experiments performed under nonstressed conditions (see Fig. 1). Thus, it can be assumed that a stable passive layer is present on the AA5052 aluminum alloy surface for the low strain values . After exceeding the strain,
, distinct increases in dc current values were observed according to applied anodic polarization levels, with the maximum at
. This indicates that above
the barrier properties of the passive film started to decay due to passive layer cracking. One must not exclude that the increase in dc current values might occur as a result of pitting corrosion onset. However, more detailed electrochemical analysis of the oxide film capacitance will reveal that the origin of current growth was cracking, not pitting.
Figure 4. Dependencies of dc current as a function of strain recorded for AA5052 aluminum alloy at various potentials:
,
,
,
.
In order to explicitly determine the threshold strain value for which the passive layer cracking occurred, analyses of impedance spectra were performed based on the equivalent circuit presented in Fig. 5, which constitutes a simplified form of the circuit used by Barbucci.34
Figure 5. Equivalent circuit used in the analysis of impedance spectra recorded for AA5052 aluminum alloy passive layer: -electrolyte resistance,
-resistance of the oxide film,
-capacitance of the oxide film,
-charge transfer resistance,
-electric double-layer capacitance.
Particular dependencies of equivalent circuit parameters strain are depicted in Fig. 6a, 6b, 6c and 6d.
Figure 6. Variations of equivalent circuit parameters with applied strain under different anodic potentials for AA5052 aluminum alloy
,
,
,
: (a) capacitance of the oxide film, (b) resistance of the oxide film, (c) electric double-layer capacitance, (d) the charge transfer resistance.
Based on presented results, the variation of individual equivalent circuit parameters with strain can be found. Starting from the oxide film capacitance , recorded threshold strain value was determined as 0.04, indicating stable passive film. In the case of
,
, and
parameters this value was lower,
. Thus, it could be concluded that for
stable passive layer on AA5052 aluminum alloy surface existed, as was the case of impedance spectra (see Fig. 3). Changes in each parameter of employed equivalent circuit detected at the threshold strain value of 0.028 for
,
,
indicate the moment of passive layer cracking process. The threshold strain value of 0.04 recorded for
is related to decay of barrier properties of the passive film due to its cracking, as was the case of dc current.
Observed increase in the oxide film capacitance can be explained according to the following, Eq. 1,23, 35
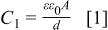
where ε=dielectric constant of vacuum, =dielectric constant of the oxide film,
=area of the electrode effectively covered with the oxide layer,
=thickness of the oxide film.
The oxide film capacitance is directly proportional to the area of the examined samples effectively covered by the oxide layer and inversely proportional to the film thickness. As already presented in Fig. 6a, in the strain range below 0.04 the oxide film capacitance assumes constant values. When the passive layer was ruptured at due to cracking, its thickness in the affected place was approaching zero. In that way the entire passive film lost it tightness. After the cracking one can observe the monotonous growth of the oxide film capacitance related to the loss of protective properties of the passive layer. Figure 7 presents the oxide film capacitance of AA7005 aluminum alloy, which does not show the existence of the passive layer in the active state. So, one has the possibility to compare it with the oxide film capacitance
of AA5052 aluminum alloy covered with the passive layer (see Fig. 6a).
Figure 7. Dependency of the oxide film capacitance strain obtained for AA7005 subjected to tensile stresses in buffered solution (;
) with additional content of
at
.
Substantial fluctuations of both capacitance parameters and
, observed particularly at
for
, resulted from the Le Chatelier–Portevin effect (PLC effect).36
Increasing anodic polarization has led to significant decrease in both resistance parameters and
, especially for
. At this potential level, due to the passive layer cracking process the electrolyte penetrated to ruptured film, causing the increase in dc current and simultaneous decrease in the oxide film resistance
and the charge transfer resistance
.
The above diagram allowed forming an additional basis for the passive layer cracking occurrence. The oxide film capacitance of AA7005 aluminum alloy, passive layer of which is not in the active state, increases monotonously from the beginning of measurement. Such behavior can be explained by the monotonous growth of the active surface during the tensile process.
At this point some aspects involved with the pitting corrosion should also be clarified. Many sources evidently show a decrease in the oxide film capacitance detected as a result of pitting.37–41 Therefore, the authors maintain that detected growth of the oxide film capacitance reflects the passive layer cracking, and not the pitting corrosion onset. It will also be confirmed by the AE measurements.
Simultaneously with mechanical and electrochemical examinations, the acoustic emission amplitude was recorded. Figure 8 presents an exemplary diagram concerning the comparison of AE amplitude, and
parameters, and dc current versus strain at
.
Figure 8. Diagram comparing the AE amplitude, and
parameters, and dc current as a function of strain for AA5052 aluminum alloy at
.
Maximal value of acoustic emission amplitude was estimated at a level of , in a manner that allowed the elimination of registration of signals originated from the metal structure. This was achieved by comparison of AE results received for exposed and not exposed to electrolyte environment specimens. Therefore, AE data recorded during these examinations could be related to electrochemical processes occurring in AA5052 aluminum alloy passive film. The first symptoms of passive layer cracking were visible in the form of single acoustic signals registered for
. A similar situation was detected in the case of the
parameter, which started to increase for
. As a result, good correlation between the acoustic emission amplitude and the capacitance of electric double layer can be stated. Further significant increase in acoustic activity was in good agreement with growing
parameter and dc current for
. Therefore, for this strain value one could state the initiation of corrosion processes on AA5052 aluminum alloy surface. Threshold strain value obtained on the basis of dc measurements was not correlated with the one from DEIS and AE examinations, which implies the application of ac techniques makes possible detection of passive layer cracking earlier than dc measurements. DC measurements allow the registration of corrosion processes on the metal surface; however, they do not allow the determination of changes of electrochemical parameters of examined passive film. As a result, it is clearly visible that passive layer cracking process undergoes far lower strain as compared to the initiation of corrosion processes on the metal surface.
In order to determine in detail the discrepancy between the strain data received from dc and ac measurements, a series of experiments for other aluminum alloys such as AA1050, AA5056, and AA7005 was performed. Each of the examined alloys was tested in 30 measurements to obtain basic statistic analysis. Accordingly, averaged tensile curves were determined and are presented in Fig. 9.
Figure 9. Tensile-strain curves recorded for AA1050, AA5052, AA5056, and AA7005 with designated threshold strain values: obtained with the use of DEIS and dc measurements ○.
Additionally, mechanical properties of examined aluminum and aluminum alloys with their critical parameters are shown in Table II.
Table II. Mechanical properties of AA1050, AA5052, AA5056, and AA7005 alloys with characteristic parameters related to the passive layer cracking process.
Mechanical properties related to the passive layer cracking process | ||||||||
---|---|---|---|---|---|---|---|---|
DEIS measurements | DC measurements | Basic mechanical properties | ||||||
Type of alloy | σ [MPa] | Standard deviation | ![]() | σ [MPa] | Standard deviation | ![]() |
![]() |
![]() |
AA1050 | 94.5 | 5.67 | 0.191 | 97.2 | 9.72 | 0.205 | 109.2 | 82.2 |
AA5052 | 134.5 | 6.71 | 0.028 | 155.0 | 12.71 | 0.040 | 174.1 | 86.0 |
AA5056 | 142.6 | 6.78 | 0.0048 | 165.1 | 12.88 | 0.006 | 265.5 | 160.1 |
AA7005 | 239.6 | 7.19 | 0.0027 | 333.1 | 16.65 | 0.005 | 391.3 | 318.2 |
Taking into consideration data in Table II, one cannot conclude about the moment of the passive layer cracking correctly when it is supported by the dc examinations only. There is a distinct discrepancy between threshold strain values received from dc measurements and the one estimated on the basis of equivalent circuit parameters from the DEIS. Furthermore, when the process proceeded in the region where Hook's law was obeyed one could get the threshold strain value burdened with the maximum error.
As a result of performed analysis, threshold strain values of passive layer cracking recorded using DEIS technique could be related to various regions on the tensile curves of investigated alloys. In the case of AA1050 the threshold strain value was related to plastic deformation region. Addition of 2% Mg (AA5052) caused the displacement of that value to the lower plastic region. Higher magnesium content of 5% (AA5056) resulted in shifting of critical strain value to elastic region very close to the yield strength. The threshold strain value for AA7005 could be attributed to the elastic deformation region distinctly lower than the yield strength. The practical meaning of that analysis is as follows. In the case of AA1050 and AA5052 alloys the threshold strain value exceeded the acceptable strain range in terms of exploitation conditions of these alloys, unlike AA5056 and AA7005 alloys.
The majority of models which describe the stress-corrosion cracking process includes the importance of passive layer presence.14, 15 Loss of barrier properties of passive layer, together with the impact of static tensile stresses and electrochemical environment, are considered the main reasons of stress corrosion cracking. Sources indicate that aluminum alloys of 2xxx series are not subjected to the stress-corrosion cracking processes in the range related to the exploitation deformations, whereas 5xxx series alloys undergo the stress-corrosion cracking at about 75% of elastic limit.42 Threshold values of mechanical properties received for examined passive layer confirm that fact. Accordingly, the explanation of passive layer cracking processes would facilitate the stress-corrosion cracking mechanism settlement.
Conclusion
Detection of the passive layer cracking process can be achieved more precisely based on acoustic emission activity and analysis of electrochemical parameters registered by means of the DEIS. Registered threshold strain value, for which the passive layer cracking process was detected, differs depending on the application of dc and ac measurements. Detailed analysis points out that the threshold strain value obtained from the dc measurements is not correct, because it was determined on the basis of proceeding corrosion processes of aluminum alloy substrate, not from the dynamic changes of examined electrochemical parameters of the passive layer. Acoustic emission technique confirmed the results received from the DEIS measurements. A discrepancy between recorded threshold stress values can lead to significant errors in analysis of aluminum alloy passive layer stability under simultaneous electrochemical and mechanical impacts. The DEIS measurements allow us to conclude that contents of alloying elements have an effect on passive layer cracking conditions, which is very important practical issue in the stress-corrosion cracking prediction. Detailed explanation of the passive layer cracking process on aluminum alloys should be involved with the structure of examined materials. Moreover, it would be crucial to determine the effect of heat-treatment on the passive layer cracking process of aluminum alloys together with the analysis of high-strength alloys of 7xxx series.
Acknowledgment
This work has been supported by grant no. 3T08A 002 29 financed by Polish Ministry of Education and Science.
Gdańsk University of Technology assisted in meeting the publication costs of this article.