Abstract
The electrochemical behavior of zinc in strong alkaline solutions containing potassium hydroxide and poly(ethylene glycol) bis(carboxymethyl) ether (PEG diacid) and polyethylene glycol (PEG) with a molecular weight of 600 was evaluated. Electrochemical studies included anodic, cathodic, and linear polarization along with potentiostatic studies. Zinc corrosion inhibition properties of PEG diacid 600 in strong alkaline solution are much more efficient by far than the inhibition capability of PEG 600. © 2003 The Electrochemical Society. All rights reserved.
Export citation and abstract BibTeX RIS
Metal alkaline cells are widely used as convenient and efficient sources of stored electrochemical energy. In particular, zinc-alkaline cells are safe, lightweight, and inexpensive and have high energy densities. Zinc metal is a favorable anode in primary batteries because of its high capacity (0.82 Ah/g), high discharge efficiency, and high safety features associated with its manufacturing processes and use. Overcoming zinc anode corrosion in alkaline media without the use of mercury, which is considered to be a highly efficient zinc corrosion inhibitor,1 is a crucial issue. The search for corrosion inhibitors that are environmentally benign and can serve as a good or even better alternative to mercury is a highly important task. In recent years, the attention of the scientific community was focused on developing and studying organic polymeric inhibitors that are capable of replacing mercury or mercury salts without degradation in the zinc anode performance or the overall cell characteristics.2 3 4 5 6 7 8 9 10 11 12 13 14 15 16
Zinc corrosion in alkaline media is controlled by cathodic water reduction reaction. One reduction product of this process is hydrogen gas and, therefore, the ability to control the parasitic reaction involving water reduction is the major objective of any cathodic inhibitor employed. The family of poly(ethylene glycol) (PEG) was thoroughly studied and the most efficient inhibitor detected was PEG 600, with a carbon chain length of
9
13
14
15 Previous works conducted by our group13
14
15 compared the electrochemical behavior of PEGs and other inhibitors, such as FORFAC (ethoxylated polyfluoro alchol), Brij30 (alkyl polyethylene oxide,
and
, and GAFAC RA 600. The organic zinc inhibitors studied in these works share one common feature; they all posses the same backbone tail composed of polyoxyethylene moiety
Each inhibitor has a different binding "head": PEG is terminated at both ends with an -OH group while GAFAC RA 600 is terminated with phosphoric acid (POOH).
Our motivation in conducting this work was to study the inhibitor developed at Electric Fuel Ltd., which substituted the PEG hydroxide heads with carboxylic acid groups.16 It is expected that the carboxylic head will exist in alkaline media as carboxylate anion, and hence a possibility of enhanced binding (in the form of two binding sites, or "fingers") to zinc metal takes place, leading to a substantial decrease in corrosion rate.16 This study aims at reporting on the electrochemical behavior of zinc metal in alkaline electrolyte containing modified PEG with two terminating carboxylic acid end groups, PEG diacid [poly(ethylene glycol) bis(carboxymethyl) ether (PEG BCME), Scheme I]. This short communication compares the electrochemical behavior of zinc metal in the presence of PEG 600 and PEG diacid at various concentrations.
Experimental
The results presented here are ascribed only to the inhibition capabilities and adsorption features of the organic polymers on zinc metal since in all of our studies we used a pure zinc metal as the working electrodes and not alloyed zinc powder (also called "battery grade" zinc).
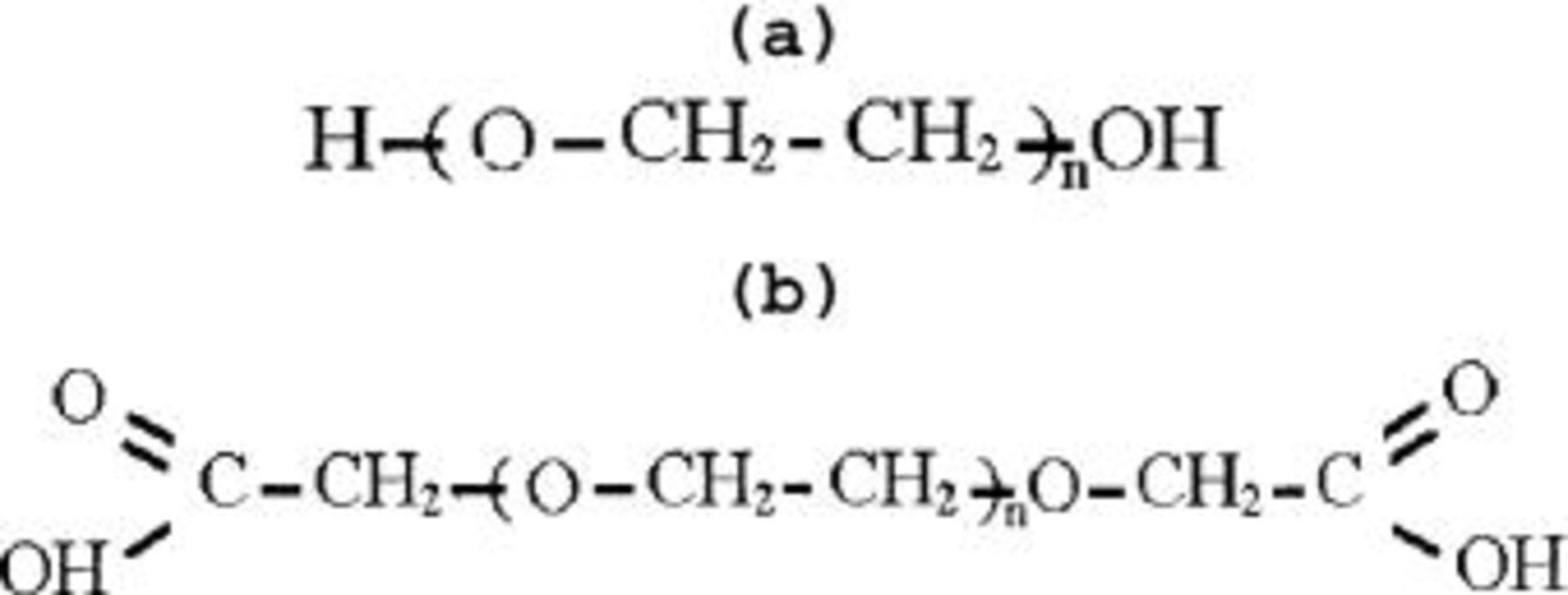
Scheme I. Molecular structure of (a) PEG and (b) PEG diacid.
Pure metal zinc (99.997%, Umicore) served in the electrochemical test as the working electrode and platinum (Pt) served as the counter electrode while standard calomel electrode (SCE) was used as the reference electrode. Thus, all potentials in this report are quoted vs. SCE. The electrochemical studies were conducted with the use of potentiostat/galvanostat PAR 273A. Alkaline electrolyte was prepared by dissolving 8.5 M of KOH (Aldrich, 99.99%) and 25 g/L of ZnO (Aldrich, 99.99%) in deionized (DI) water (with a measured conductivity less than 2 μS/cm). PEG (Aldrich) and poly(ethylene glycol) diacid (PEG bis(carboxymethyl) ether, Aldrich), both with average molecular weights of 600 g/mol, were added in concentrations ranging between 400 and 2000 ppm relative to the alkaline solution. More details on alkaline electrolyte preparation and electrochemical cell configuration are described elsewhere.13 14 15
Results and Discussion
Anodic behavior of zinc in electrolytes containing PEGs.—
Previous work conducted by our group demonstrated that an efficient inhibition of zinc corrosion is obtained with the addition of 800-2000 ppm of PEG. Thus, in this short communication we report mainly on our finding with the application of 800-2000 ppm of inhibitors. Figure 1 presents the anodic curves obtained from zinc polarization in alkaline media containing 800 ppm of PEG and PEG diacid at a scan rate of 1 mV/s. As can be seen, the addition of inhibitors shifts the measured currents at open-circuit potential (OCP) to values, which are lower by an order of magnitude than the values measured from zinc immersed in inhibitor-free alkaline solution. In addition, at a given anodic potential the currents measured from polarized zinc in electrolytes containing inhibitors are also lower than the values measured from zinc polarization in inhibitor-free alkaline solution. However, despite the observed reduction in the anodic currents, the addition of both inhibitors does not prevent active anodic dissolution of zinc metal. Although the measured anodic currents are shifted to lower values, the active dissolution of zinc metal at potentials higher than
vs. SCE) does not stop at any time. Moreover, our studies also indicate that no effect of the inhibitor concentrations on the anodic currents was detected. Thus, neither PEG nor PEG diacid can be regarded as anodic inhibitors.
Figure 1. Anodic profiles obtained from polarized zinc in 8.5 M KOH containing 800 ppm of PEG and PEG diacid at a scan rate of 1 mV/s. The anodic curve obtained from zinc in alkaline electrolyte without the addition of inhibitor is shown for comparison.
Cathodic behavior of zinc in electrolytes containing PEGs.—
The electrochemical behavior of zinc in electrolytes containing 2000 ppm of organic inhibitors was monitored as a function of time. Figure 2 presents the cathodic behavior of zinc in electrolytes containing 2000 ppm of PEG and PEG diacid. The cathodic curve obtained from zinc in alkaline electrolyte without the addition of inhibitor is shown for comparison. As can be seen, the two organic materials can be considered as cathodic inhibitors. Figure 2a shows that upon immersion the best effect of corrosion inhibition is achieved with the use of alkaline solution containing PEG BCME, while the inhibition achieved from PEG solution was not comparable. The potential range in which PEG is highly effective was 120 mV, between
and
After storage time of 1 h in the solution, zinc corrosion rate is further reduced. Figure 2b presents the cathodic behavior of zinc in alkaline solutions containing PEG. The measured currents are further reduced (from
to
, indicating the improved inhibition properties of PEG. One hour after immersion, the inhibition potential window was much broader and was measured to be
below
Figure 2. Cathodic profiles obtained from polarized zinc in 8.5 M KOH containing 2000 ppm of PEG and PEG diacid; (a) immediately upon immersion and (b) after 1 h storage in the electrolyte. The cathodic curve obtained from zinc in alkaline electrolyte without the addition of inhibitor is shown for comparison. All scans are recorded at a scan rate of 1 mV/s.
The immediate potential window in which an effective inhibition of the zinc in PEG diacid solution is achieved was measured to be in the range of
to
The cathodic current drops immediately to
The wide inhibition potential is almost unchanged (and remains wider) as a function of time. This data points out that the inhibition of PEG diacid is strong and efficient to begin with, and that the corrosion inhibition potential is not improved further upon storage. However, the currents measured are further reduced upon 1 h storage.
Potentiostatic measurements.—
To examine the inhibition efficiency of both organic compounds, zinc metal was potentiostatically polarized to This potential was selected because it lies in the potential range of the inhibition window (up to 0.2 V below
, as shown in Fig. 2. Figure 3 presents data obtained from potentiostatic measurements taken at
from zinc electrode immersed in alkaline electrolyte containing 800 ppm of PEG inhibitors. Figure 3 shows clearly that the current measured from the zinc electrode immersed in the alkaline solution containing PEG and PEG diacid is reduced significantly. However, the response time for inhibition is very short once zinc is immersed in PEG diacid electrolyte. The initial current measured is 20 times lower than the current measured from PEG (Fig. 3, inset). The current values obtained from zinc electrodes polarized in PEG and PEG diacid solutions are equal only after 1500 s. The current rise at
is very low once PEG diacid is present in the solution, while with PEG the values are quite high. The results obtained from the potentiostatic steps clearly point out that PEG diacid reduces the drawn currents to much lower values than the currents measured once PEG is present in the electrolytes.
Figure 3. Potentiostatic profiles obtained from zinc electrode polarized to in 8.5 M KOH containing 800 ppm of PEG and PEG diacid.
Figure 4 points out the superiority of PEG diacid over PEG as zinc corrosion inhibitor. In this experiment zinc electrode was allowed to be polarized to in 8.5 M KOH and only after 10 min of polarization was 2000 ppm of inhibitor added. As seen in Fig. 4, PEG diacid reduces the current immediately, without any delay. Current values drop by an order of magnitude within a few seconds while the time needed for zinc to be stabilized in PEG electrolyte at these low current levels is much longer. In a different set of experiments the zinc potential was polarized to
more than 200 mV below
(Fig. 5) in alkaline electrolytes containing a minimal amount of 400 ppm of PEG inhibitors. We observe the unique ability of PEG diacid to inhibit the corrosion at a relatively wide range of potentials even with the use of a fairly small amount of inhibitor, while PEG is incapable of inhibiting zinc corrosion at this extremely low potential.
Figure 4. Data obtained from potentiostatic polarizations of zinc electrode (immersed in 8.5 M KOH) at Inhibitors in a concentration of 2000 ppm were added to the solution after 10 min.
Figure 5. Potentiostatic profiles obtained from zinc electrode polarized to in 8.5 M KOH containing 400 ppm of PEG and PEG diacid.
Conclusions
The results presented here point out that PEG diacid has superior inhibiting characteristics to PEG. At this stage of the research it can be concluded that the inhibition properties of PEG diacid are more effective than PEG. Not only does it significantly reduce the corrosion current but it also expands the inhibition potential to extremely low potential values. Our intentions are to continue to evaluate the electrochemical performance of zinc and zinc electrochemical cells and batteries in the presence of this unique and superior inhibitor and also to identify the surface films and morphological characteristics which may be responsible for the enhanced corrosion inhibition capabilities.
Acknowledgments
This research work was funded by Electric-Fuel Ltd. and The Alexander Goldberg Memorial Research Fund at the Technion, Israel Institute of Technology.
Technion-Israel Institute of Technology assisted in meeting the publication costs of this article.