Abstract
One of the mechanisms explaining relationships between CO2-rich fluids and granitoid magmas at high-temperature crustal metamorphism is the melting of protoliths that originally contained carbonate minerals. In order to study the coupled processes of dehydration/decarbonation and melting, experiments were conducted with carbonate–biotite gneiss from an Archean greenstone belt at pressures of 6, 10, and 15 kbar in the temperature range of 800–950°C, and phase relations in this rock were modeled using the pseudo-section method. The experiments and modeling revealed a subvertical positive dP/dT slope of the solidus of the rock. In comparison to the calculated solidus temperatures, the experiments showed higher melting temperatures (~800°C at 6 kbar and ~850°C at 10 and 15 kbar). The products of the experiments at pressures of 6 and 10 kbar and temperatures >850°C were found out to contain assemblages of clinopyroxene, orthopyroxene, and ilmenite. The products of the experiments at 15 kbar did not contain either orthopyroxene or ilmenite, but calcium garnet and rutile were stable. The first portions of the near-solidus melts at 6 and 10 kbar were poor in SiO2 (44–50 wt %) and were formed because carbonate phases were involved in the melting reactions. With a temperature increase, the melts acquired a granite composition that was close to the composition of melts formed during the melting of the carbonate-free plagioclase + biotite + quartz assemblage. An aqueous–carbonic fluid containing Ca–Mg–Fe carbonate components coexisted with the melts. The phase assemblages and compositions of the granite melts obtained in the experiments are consistent with the modeling results. Comparison of the experimental results with published data on the partial melting of the carbonate-free plagioclase + biotite + quartz assemblages led us to the preliminary conclusion that Ca–Mg–Fe carbonates are able to decrease the melting temperature. The experiments have demonstrated that granite magmas can be derived together with aqueous–carbonic fluids from a carbonate-bearing protolith during high-grade metamorphism in the middle and lower crust. The occurrence of clinopyroxene or two-pyroxene assemblages in granitoids can be considered as a mineralogical indicator of this process.













Similar content being viewed by others
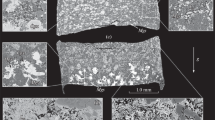
REFERENCES
Acosta-Vigil, A., London, D., and Morgan, G.B., Experiments on the kinetics of partial melting of a leucogranite at 200 MPa H2O and 690–800°C: compositional variability of melts during the onset of H2O-saturated crustal anatexis, Contrib. Mineral. Petrol., 2006, vol. 151, pp. 539–551.
Anovitz, L.M. and Essene, E.J., Phase equilibria in the system CaCO3–MgCO3–FeCO3, J. Petrol., 1987, vol. 28, pp. 389–415.
Aranovich, L.Y. and Newton, R.C., H2O activity in concentrated NaCl solutions at high pressures and temperatures measured by the brucite-periclase equilibrium, Contrib. Mineral. Petrol., 1996, vol. 125, pp. 200–212.
Aranovich, L.Y. and Safonov, O.G., Halogens in high-grade metamorphism, The Role of Halogens in Terrestrial and Extraterrestrial Geochemical Processes, Cham: Springer, 2018.
Bartoli, O. and Cesare, B., Nanorocks: a 10-year-old story, Rendiconti Lincei. Scienze Fisiche e Naturali, 2020, vol. 31, pp. 249–257.
Boettcher, A.L., Robertson, J.K., and Wyllie, P.J., Studies in synthetic carbonatite systems: solidus relationships for CaO–MgO–CO2–H2O to 40 kbar and CaO–MgO–SiO2–CO2–H2O to 10 kbar, J. Geophys. Res. Solid Earth, 1980, vol. 85, pp. 6937–6943.
Bohlen, S.R. and Liotta, J.J., A barometer for garnet amphibolites and garnet granulites, J. Petrol., 1986, vol. 27, pp. 1025–1034.
Bohlen, S.R., Wall, V.J., and Boettcher, A.L., Geobarometry in granulites, Kinetics and Equilibrium in Mineral Reactions, New York: Springer, 1983, pp. 141–171.
Bohlender, F., van Reenen, D.D., and Barton, Jr.J.M., Evidence for metamorphic and igneous charnockites in the southern marginal zone of the Limpopo Belt, Precambrian Res., 1992, vol. 55, pp. 429–449.
Bolder-Schrijver, L.J.A., Kriegsmann, L.M., and Touret, J.L.R., Primary carbonate/CO2 inclusions in sapphirine-bearing granulites from Central Sri-Lanka, J. Metamorph. Geol., 2000, vol. 18, pp. 259–269.
Le Breton, N. and Thompson, A.B., Fluid-absent (dehydration) melting of biotite in metapelites in the early stages of crustal anataxis, Contrib. Mineral. Petrol., 1988, vol. 99, pp. 226–237.
Brown, M., Crustal melting and melt extraction, ascent and emplacement in orogens: mechanisms and consequences, J. Geol. Soc., 2007, vol. 164, pp. 709–730.
Brown, M., Granite: from genesis to emplacement, GSA Bull, 2013, vol. 1079-1113.
Carvalho, B.B., Bartoli, O., Cesare, B., et al., Primary CO2-bearing fluid inclusions in granulitic garnet usually do not survive, Earth Planet. Sci. Lett., 2020, vol. 536, p. 116170.
Cesare, B., Acosta-Vigil, A., Bartoli, O., et al., What can we learn from melt inclusions in migmatites and granulites?, Lithos, 2015, vol. 239, pp. 186–216.
Chappell, B.W. and White, A.J.R., Two contrasting granite types: 25 years later, Austral. J. Earth Sci, 2001, vol. 48, pp. 489–499.
Chappell, B.W., Bryant, C.J., and Wyborn, D., Peraluminous I-type granites, Lithos, 2012, vol. 153, pp. 142–153.
Clemens, J.D., The granulite–granite connexion, Granulites and Crustal Differentiation, Vielzeuf, D. and Vidal, P., Eds., Dordrecht: Kluwer Academic Publishers, 1990.
Clemens, J.D., Partial melting and granulite genesis: a partisan overview, Precambrian Res., 1992, vol. 55, pp. 297–301.
Clemens, J.D., Experimental evidence against CO2-promoted deep crustal melting, Nature, 1993, vol. 363, pp. 336–338.
Clemens, J.D., Droop, G.T., and Stevens, G., High-grade metamorphism, dehydration and crustal melting: a reinvestigation based on new experiments in the silica-saturated portion of the system KAlO2–MgO–SiO2–H2O–CO2 at P ≤ 1.5 GPa, Contrib. Mineral. Petrol., 1997, vol. 129, pp. 308–325.
Connolly, J.A.D., Computation of phase equilibria by linear programming: a tool for geodynamic modeling and its application to subduction zone decarbonation, Earth Planet. Sci. Lett., 2005, vol. 236, pp. 524–541.
Duke, E.F. and Rumble, D., Textural and isotopic variations in graphite from plutonic rocks, south-central New Hampshire, Contrib. Mineral. Petrol., 1986, vol. 93, pp. 409–419.
Duncan, M.S. and Dasgupta, R., Co2 solubility and speciation in rhyolitic sediment partial melts at 1.5–3.0 GPa - implications for carbon flux in subduction zones, Geochim. Cosmochim. Acta, 2014, vol. 124, pp. 328–347.
Ebadi, A. and Johannes, W., Beginning of melting and composition of first melts in the system Qz–Ab–Or–H2O–CO2, Contrib. Mineral. Petrol., 1991, vol. 106, pp. 286–295.
Elkins, L.T. and Grove, T.L., Ternary feldspar experiments and thermodynamic models, Am. Mineral., 1990, vol. 75, pp. 544–559.
Farquhar, J. and Chacko, T., Isotopic evidence for involvement of CO2-bearing magmas in granulite formation, Nature, 1991, vol. 354, pp. 60–63.
Ferrero, S., Wunder, B., Ziemann, M.A., et al., Carbonatitic and granitic melts produced under conditions of primary immiscibility during anatexis in the lower crust, Earth Planet. Sci. Lett., 2016, vol. 454, pp. 121–131.
Le Fort, P., Cuney, M., Deniel, C., et al., Crustal generation of the Himalayan leucogranites, Tectonophysics, 1987, vol. 134, pp. 39–57.
Frezzotti, M.-L., Di Vincenzo, G., Ghezzo, C., et al., Evidence of magmatic CO2-rich fluids in peraluminous graphite-bearing leucogranites from Deep Freeze Range (northern Victoria Land, Antarctica), Contrib. Mineral. Petrol., 1994, vol. 117, pp. 111–123.
Frost, B.R. and Frost, C.D., CO2, melts and granulite metamorphism, Nature, 1987, vol. 327, pp. 503–506.
Frost, B.R., Frost, C.D., and Touret, J.L., Magmas as a source of heat and fluids in granulite metamorphism, Fluid Movements–-Element transport and the composition of the Deep Crust, Netherlands: Springer, 1989.
Frost, B.R., Frost, C.D., Hulsebosch, T.P., et al., Origin of the charnockites of the Louis Lake Batholith, Wind River Range, Wyoming, J. Petrol., 2000, vol. 41, pp. 1759–1776.
Frost, B.R., Barnes, C.G., Collins, W.J., et al., A geochemical classification for granitic rocks, J. Petrol., 2001, vol. 42, pp. 2033–2048.
Gao, P., Zheng, Y.F., and Zhao, Z.F., Experimental melts from crustal rocks: a lithochemical constraint on granite petrogenesis, Lithos, 2016, vol. 266, pp. 133–157.
Gardien, V., Thompson, A.B., Grujic, D., et al., Experimental melting of biotite + plagioclase + quartz ± muscovite assemblages and implications for crustal melting, J. Geophys. Res. Solid Earth, 1995, vol. 100, B8, pp. 15581–15591.
Gardien, V., Thompson, A.B., and Ulmer, P., Melting of biotite + plagioclase + quartz gneisses: the role of H2O in the stability of amphibole, J. Petrol., 2000, vol. 41, pp. 651–666.
Glassley, W.E., Deep crustal carbonates as CO2 fluid sources: evidence from metasomatic reaction zones, Contrib. Mineral. Petrol., 1983, vol. 84, pp. 15–24.
Grant, J.A., 1986. quartz-phlogopite-liquid equilibria and origins of charnockites, Am. Mineral., 1986, vol. 71, pp. 1071–1075.
Grassi, D. and Schmidt, M.W., The melting of carbonated pelites from 70 to 700 km depth, J. Petrol., 2011, vol. 52, pp. 765–789.
Groppo, C., Rapa, G., Frezzotti, M.L., et al., The fate of calcareous pelites in collisional orogens, J. Metamorph. Geol., 2021, vol. 39, pp. 181–207.
Hamilton, D.L., Mackenzie W.S. phase equilibrium studies in the system NaAlSiO4 (nepheline)–KAlSiO4 (kalsilite)–SiO2–H2O, Mineral. Mag., 1965, vol. 34, pp. 214–231.
Hammouda, T. and Keshav, S., Melting in the mantle in the presence of carbon: review of experiments and discussion on the origin of carbonatites, Chem. Geol., 2015, vol. 418, pp. 171–188.
Harley, S.L. and Santosh, M., Wollastonite at nuliyam, kerala, southern india: a reassessment of CO2-infiltration and charnockite formation at a classic locality, Contrib. Mineral. Petrol., 1995, vol. 120, pp. 83–94.
Henry, D.J., Guidotti, C.V., and Thomson, J.A., The Ti-saturation surface for low-to-medium pressure metapelitic biotites: implications for geothermometry and Ti-substitution mechanisms, Am. Mineral., 2005, vol. 90, pp. 316–328.
Herms, P. and Schenk, V., Fluid inclusions in granulite-facies metapelites of the Hercynian ancient lower crust of the Serre, Calabria, Southern Italy, Contrib. Mineral. Petrol., 1992, vol. 112, pp. 393–404.
Herms, P. and Schenk, V., Fluid inclusions in high-pressure granulites of the Pan-African belt in Tanzania (Uluguru Mts): a record of prograde to retrograde fluid evolution, Contrib. Mineral. Petrol., 1998, vol. 130, pp. 199–212.
Holland, T.J., The reaction albite = jadeite + quartz determined experimentally in the range 600-1200oC, Am. Mineral., 1980, vol. 65, pp. 129–134.
Holland, T. and Powell, R., Thermodynamics of order–disorder in minerals; i. symmetric formalism applied to minerals of fixed composition, Am. Mineral., 1996, vol. 81, pp. 1413–1424.
Holloway, J.R., Fluids in evolution of granitic magmas: consequence of finite CO2 solubility, GSA Bull., 1976, vol. 87, pp. 1513–1518.
Huizenga, J.M. and Touret, J.L., Granulites, CO2 and graphite, Gondwana Res., 2012, vol. 22, pp. 799–809.
Johnson, T.E., White, R.W., and Powell, R., Partial melting of metagreywacke: a calculated mineral equilibria study, J. Metamorph. Geol., 2008, vol. 26, pp. 837–853.
Kerrick, D.M. and Caldeira, K., Metamorphic CO2 degassing from orogenic belts, Chem. Geol., 1998, vol. 145, pp. 213–232.
Konnerup-Madsen, J., Composition and microthermometry of fluid inclusions in the Kleivan granite, south Norway, Am. J. Sci., 1977, vol. 277, pp. 673–696.
Konnerup-Madsen, J., Fluid inclusions in quartz from deep-seated granitic intrusions, south Norway, Lithos, 1979, vol. 12, pp. 13–23.
Lamadrid, H.M., Lamb, W.M., Santosh, M., et al., Raman spectroscopic characterization of H2O in CO2-rich fluid inclusions in granulite facies metamorphic rocks, Gondwana Res., 2014, vol. 26, pp. 301–310.
Lamb, W., Carbonates in feldspathic gneisses from the granulite facies: implications for the formation of CO2-rich fluid inclusions, Metamorphic and Crustal Evolution: Papers in Honour of Prof. R.S. Sharma, Ed. Thomas, H., New Delhi: Atlantic, 2005, pp. 163–181.
Lowenstern, J.B., Carbon dioxide in magmas and implications for hydrothermal systems, Miner. Deposita, 2001, vol. 36, pp. 490–502.
Mann, U. and Schmidt, M.W., Melting of pelitic sediments at subarc depths: 1. Flux vs. fluid-absent melting and a parameterization of melt productivity, Chem. Geol., 2015, vol. 404, pp. 150–167.
McCourt, S. and van Reenen, D.D., Structural geology and tectonic setting of the Sutherland greenstone belt, Kaapvaal Craton, South Africa, Precambrian Res., 1992, vol. 55, pp. 93–110.
Mityaev A.S., Safonov O.G., Reutsky, V.N., et al., Isotope Characteristics of carbonates from rocks of greenstone belts as an indicator of a possible source of fluids in Precambrian granulite complexes: an example from the Giyani Greenstone Belt and the Limpopo Granulite Complex, South Africa, Dokl. Earth Sci., 2020, vol. 492, pp. 342–345.
Montel, J.M. and Vielzeuf, D., Partial melting of metagreywackes, Part II. Compositions of minerals and melts, Contrib. Mineral. Petrol., 1997, vol. 128, pp. 176–196.
Moyen, J. and Stevens, G., Experimental constraints on TTG petrogenesis: implications for Archean geodynamics, Geophys. Monogr. Ser., 2006, vol. 164, p. 149.
Moyen, J. and Martin, H., Forty years of TTG research, Lithos, 2012, vol. 148, pp. 312–336.
Nair, R. and Chacko, T., Fluid-absent melting of high-grade semi-pelites: P-T constraints on orthopyroxene formation and implications for granulite genesis, J. Petrol., 2002, vol. 43, pp. 2121–2142.
Newton, R.C., Smith, J.V., and Windley, B.F., Carbonic metamorphism, granulites and crustal growth, Nature, 1980, vol. 288, pp. 45–50.
Ni, H. and Keppler, H., Carbon in silicate melts, Rev. Mineral. Geochem., 2013, vol. 75, pp. 251–287.
Papale, P., Moretti, R., and Barbato, D., The compositional dependence of the saturation surface of H2O + CO2 fluids in silicate melts, Chem. Geol., 2006, vol. 229, pp. 78–95.
Patiño Douce, A.E., Effects of pressure and H2O content on the compositions of primary crustal melts, Trans. R. Soc. Edinb.: Earth Sci., 1996, vol. 87, pp. 11–21.
Patiño Douce, A.E. and Johnston, A.D., Phase equilibria and melt productivity in the pelitic system: implications for the origin of peraluminous granitoids and aluminous granulites, Contrib. Mineral. Petrol., 1991, vol. 107, pp. 202–218.
Patiño Douce, A.E. and Beard, J.S., Dehydration-melting of biotite gneiss and quartz amphibolite from 3 to 15 kbar, J. Petrol., 1995, vol. 36, pp. 707–738.
Patiño Douce, A.E. and Beard, J.S., Effects of P, fO2 and Mg/Fe ratio on dehydration melting of model metagreywackes, J. Petrol., 1996, vol. 37, pp. 999–1024.
Perchuk, L.L. and Gerya, T.V., Formation and evolution of Precambrian granulite terranes: a gravitational redistribution model, Origin and Evolution of Precambrian High-Grade Gneiss Terranes, with Special Emphasis on the Limpopo Complex of Southern Africa, van Reenen, D.D., Kramers, J.D., McCourt, S., Perchuk, L.L., Eds. Geol. Soc. Amer. Mem., 2011, vol. 207, pp. 1–22.
Perchuk, L.L., Gerya, T.V., van Reenen, D.D., et al., The Limpopo metamorphic belt, South Africa: 2. Decompression and cooling regimes of granulites and adjacent rocks of the Kaapvaal Craton, Petrology, 1996, vol. 4, no. 6, pp. 571–599.
Perchuk, L.L., Gerya, T.V., van Reenen, D.D., et al., P-t paths and tectonic evolution of shear zones separating high-grade terrains from cratons: examples from Kola Peninsula (Russia) and Limpopo region (South Africa), Mineral. Petrol., 2000, vol. 69, pp. 109–142.
Peterson, J.W. and Newton, R.C., CO2-enhanced melting of biotite-bearing rocks at deep-crustal pressure–temperature conditions, Nature, 1989, vol. 340, pp. 378–380.
Peterson, J.W. and Newton, R.C., Experimental biotite–quartz melting in the KMASH-CO2 system and the role of CO2 in the petrogenesis of granites and related rocks, Am. Mineral., 1990, vol. 75, pp. 1029–1042.
Pettijohn, F.J., Sedimentary Rocks, New York: Harper & Row, 1975, vol. 3.
Rajesh, H.M., Belyanin, G.A., Safonov, O.G., et al., Pyroxene-bearing low-and high- HREE TTGs from the northeastern margin of the Kaapvaal Craton, southern Africa: Implications for Archean geodynamics, Lithos, 2019, vol. 348, p. 105181.
Rapp, R.P. and Watson, E.B., Dehydration melting of metabasalt at 8–32 kbar: implications for continental growth and crust-mantle recycling, J. Petrol., 1995, vol. 36, pp. 891–931.
Safonov, O.G., Tatarinova, D.S., van Reenen, D.D., et al., Fluid-assisted interaction of peraluminous metapelites with trondhjemitic magma within the Petronella shear-zone, Limpopo Complex, South Africa, Precambrian Res., 2014, vol. 253, pp. 114–145.
Safonov, O.G., Yapaskurt, V.O., Elburg, M., et al., P-T conditions, mechanism and timing of the localized melting of metapelites from the Petronella shear-zone and relationships with granite intrusions in the Southern Marginal Zone of the Limpopo Belt, South Africa, J. Petrol., 2018a, vol. 59, pp. 695–734.
Safonov, O.G., Reutsky, V.N., Varlamov, D.A., et al., Composition and source of fluids in high-temperature graphite-bearing granitoids associated with granulites: examples from the Southern Marginal Zone, Limpopo Complex, South Africa, Gondwana Res., 2018b, vol. 60, pp. 129–152.
Safonov, O.G., van Reenen, D.D., Yapaskurt, V.O., et al., Thermal and Fluid Effects of Granitoid Intrusions on Granulite Complexes: Examples from the Southern Marginal Zone of the Limpopo Complex, South Africa, Petrology, 2018, vol. 26, no. 6, pp. 617–639.
Safonov, O.G., Mityaev, A.S., Yapaskurt, V.O., et al., Carbonate-silicate inclusions in garnet as evidence for a carbonate-bearing source for fluids in leucocratic granitoids associated with granulites of the Southern Marginal Zone, Limpopo Complex, South Africa, Gondwana Res., 2020, vol. 77, pp. 147–167.
Santosh, M. and Omori, S., Co2 flushing: a plate tectonic perspective, Gondwana Res., 2008, vol. 13, pp. 86–102.
Santosh, M., Jayananda, M., and Mahabaleswar, B., Fluid evolution in the Closepet Granite - a magmatic source for CO2 in charnockite formation at Kabbaldurga, J. Geol. Soc. India, 1991, vol. 38, pp. 55–65.
Santosh, M., Tanaka, K., and Yoshimura, Y., Carbonic fluid inclusions in ultrahigh-temperature granitoids from southern India, C.R. Geosci., 2005, vol. 337, pp. 327–335.
Satish-Kumar, M. and Santosh, M., A petrological and fluid inclusion study of calc-silicate-charnockite associations from southern Kerala, India: Implications for CO2 influx, Geol. Mag., 1998, vol. 135, pp. 27–45.
Sawyer, E.W., The influence of source rock type, chemical weathering and sorting on the geochemistry of clastic sediments from the Quetico metasedimentary belt, Superior Province, Canada, Chem. Geol., 1986, vol. 55, pp. 77–95.
Sawyer, E.W., Cesare, B., and Brown, M., When the continental crust melts, Elements, 2011, vol. 7, pp. 229–234.
Shaposhnikov, V.V., and Aranovich, L.Ya., Experimental study of model granite melting in the presence of alkali carbonate solutions at 400 MPa, Geochem. Int., 2015, vol. 53, no. 9, pp. 838–844.
Skjerlie, K.P. and Johnston, A.D., Fluid-absent melting behavior of an F-rich tonalitic gneiss at mid-crustal pressures: implications for the generation of anorogenic granites, J. Petrol., 1993, vol. 34, pp. 785–815.
Skora, S., Blundy, J.D., Brooker, R.A., et al., Hydrous phase relations and trace element partitioning behaviour in calcareous sediments at subduction-zone conditions, J. Petrol., 2015, vol. 56, pp. 953–980.
Srikantappa, C., Raith, M., and Touret, J.L.R., Synmetamorphic high-density carbonic fluids in the lower crust: evidence from the Nilgiri granulites, southern India, J. Petrol., 1992, vol. 33, pp. 733–760.
Stevens, G. and Clemens, J.D., Fluid-absent melting and the roles of fluids in the lithosphere: a slanted summary?, Chem. Geol., 1993, vol. 108, pp. 1–17.
Stevens, G., Clemens, J.D., and Droop, G.T., Melt production during granulite-facies anatexis: experimental data from “primitive” metasedimentary protoliths, Contrib. Mineral. Petrol., 1997, vol. 128, pp. 352–370.
Tacchetto, T., Bartoli, O., Cesare, B., et al., Multiphase inclusions in peritectic garnet from granulites of the Athabasca granulite terrane (Canada): evidence of carbon recycling during Neoarchean crustal melting, Chem. Geol., 2019, vol. 508, pp. 197–209.
Thompson, A.B., Dehydration melting of pelitic rocks and the generation of H2O undersaturated granitic liquids, Am. J. Sci., 1982, vol. 282, pp. 1567–1595.
Thomsen, T.B. and Schmidt, M.W., Melting of carbonated pelites at 2.5–5.0 GPa, silicate-carbonatite liquid immiscibility, and potassium-carbon metasomatism of the mantle, Earth Planet. Sci. Lett., 2008, vol. 267, pp. 17–31.
Tiraboschi, C., Tumiati, S., Sverjensky, D., et al., Experimental determination of magnesia and silica solubilities in graphite-saturated and redox-buffered high-pressure COH fluids in equilibrium with forsterite + enstatite and magnesite + enstatite, Contrib. Mineral. Petrol., 2018, vol. 173, pp. 1–17.
Touret, J.L.R. and Huizenga, J.-M., Fluids in granulites, Geol. Soc. Am. Mem., 2011, vol. 207, pp. 25–37.
Tsuno, K. and Dasgupta, R., Melting phase relation of nominally anhydrous, carbonated pelitic-eclogite at 2.5–3.0 GPa and deep cycling of sedimentary carbon, Contrib. Mineral. Petrol., 2011, vol. 161, pp. 743–763.
Tsuno, K. and Dasgupta, R., The effect of carbonates on near-solidus melting of pelite at 3 GPa: relative efficiency of H2O and CO2 subduction, Earth Planet. Sci. Lett., 2012, vol. 319, pp. 185–196.
Tsunogae, T., Santosh, M., Osanai, Y., et al., Very high-density carbonic fluid inclusions in sapphirine-bearing granulites from Tonagh Island in the Archean Napier Complex, East Antarctica: Implications for CO2 infiltration during ultrahigh-temperature (T > 1100°C) metamorphism, Contrib. Mineral. Petrol., 2002, vol. 143, pp. 279–299.
van Reenen, D.D., Smit, C.A., Perchuk, L.L., et al., Thrust exhumation of the Neoarchean ultrahigh-temperature Southern Marginal Zone, Limpopo Complex: convergence of decompression-cooling paths in the hanging wall and prograde P-T paths in the footwall, Geol. Soc. Amer. Mem., 2011, vol. 207, pp. 189–212.
van Reenen, D.D., Smit, C.A., Perchuk, A.L., et al., The Neoarchaean Limpopo orogeny: exhumation and regional-scale gravitational crustal overturn driven by a granulite diaper, The Archaean Geology of the Kaapvaal Craton, Southern Africa, Cham: Springer, 2019, pp. 185–224.
Vielzeuf, D. and Holloway, J.R., Experimental determination of the fluid-absent melting relations in the pelitic system, Contrib. Mineral. Petrol., 1988, vol. 98, pp. 257–276.
Vielzeuf, D. and Montel, J.M., Partial melting of metagreywackes. Part I. Fluid-absent experiments and phase relationships, Contrib. Mineral. Petrol., 1994, vol. 117, pp. 375–393.
Weinberg, R.F. and Hasalova, P., Water-fluxed melting of the continental crust: a review, Lithos, 2015, vol. 212, pp. 158–188.
Wendlandt, R.F., Influence of CO2 on melting of model granulite facies assemblages—a model for the genesis of charnockites, Am. Mineral., 1981, vol. 66, pp. 1164–1174.
White, R.W., Powell, R., Holland, T.J.B., et al., The effect of TiO2 and Fe2O3 on metapelitic assemblages at greenschist and amphibolite facies conditions: mineral equilibria calculations in the system K2O–FeO–MgO–Al2O3–SiO2–H2O–TiO2–Fe2O3, J. Metamorph. Geol., 2000, vol. 18, pp. 497–511.
White, R.W., Powell, R., Holland, T.J.B., et al., New mineral activity-composition relations for thermodynamic calculations in metapelitic systems, J. Metamorph. Geol., 2014, vol. 32, pp. 261–286.
White, R.W., Palin, R.M., and Green, E.C., High-grade metamorphism and partial melting in Archean composite grey gneiss complexes, J. Metamorph. Geol., 2017, vol. 35, pp. 181–195.
Whitney, D.L. and Evans, B.W., Abbreviations for names of rock-forming minerals, Am. Mineral., 2010, vol. 95, pp. 185–187.
Zen E-an. Aluminum enrichment in silicate melts by fractional cryitallization: some mineralogic and petrographic constraints, J. Petrol., 1986, vol. 27, pp. 1095–1117.
ACKNOWLEDGMENTS
The authors thank A.L Perchuk (Geological Faculty, Moscow State University) for constructive criticism of the initial version of the manuscripts and suggestions for its improvement.
Funding
This study was supported by Russian Foundation for Basic Research, project no. 20-35-90013 for postgraduate students and by the Russian Science Foundation, project 18-17-00206-P (part of this research concerning relations between granite magmatism and the evolution of Precambrian granulite complexes) and was partly carried under the government-financed research projects FMUF-2022-0004; 1021051302305-5-1.5.2;1.5.4 for Korzhinskii Institute of Experimental Mineralogy, Russian Academy of Science.
Author information
Authors and Affiliations
Corresponding author
Ethics declarations
The authors declare that they have no conflicts of interest.
Additional information
Translated by E. Kurdyukov
Rights and permissions
About this article
Cite this article
Mityaev, A.S., Safonov, O.G., Varlamov, D.A. et al. Partial Melting of Carbonate–Biotite Gneiss at the Conditions of the Continental Crust: Experimental and Thermodynamic Modeling. Petrology 30, 278–304 (2022). https://doi.org/10.1134/S0869591122030067
Received:
Revised:
Accepted:
Published:
Issue Date:
DOI: https://doi.org/10.1134/S0869591122030067