Abstract
This paper refers to the transfer of the results of federally funded research towards commercialization in a very short time in order to meet the needs of an emerging and fast growing industry, involving the remediation of produced water from fracking operations on site and at low cost. The focus is on photocatalytic nanogrids: ceramic mats that may be used as covers for produced water stored in ponds and pits. The electrospun mats of the self-supported ceramic photocatalysts are responding to the whole solar spectrum and oxidize benzene and other liquid hydrocarbons in water, turning them into innocuous compounds. There is no hydrocarbon pollution left in the water after catalytic reactions and the nanogrids are reusable. The scalable processing of this technology, as well as the scaled-up proof of concept, is detailed in this work. The pathway from a research funding award in response to the Gulf oil spill remediation, through the inaugural class of the National Science Foundation Innovation Corps (I-Corps) cohorts towards a small business development is also outlined. The impact of the visionary and effective I-Corps program on the fast translation of lab-based technology from proof of concept to prototyping for industry validation is also described.
Export citation and abstract BibTeX RIS
1. Introduction
The Nanogrids-based (Gouma and Lee 2011) technology that is described here was first developed with funding from a National Science Foundation (NSF) grant as a nanotechnology to clean up oil spills in water. This was a RAPID-type solicitation responding to the Gulf oil spill disaster, which required advanced technologies and out-of-the-box thinking. The solar-energy activated nanocatalysts developed in that project were thought to be the perfect solution for cleaning up miles upon miles of petroleum-based oil-contaminated ocean. Thus, our team was selected to try out this 'promising' technology in the first ever group of Innovation Corps (I-Corps), the NSF's elite operation targeting accelerated technology transfer.
2. The I-Corps one-of-a-kind experience
The aim of our I-Corps team was to commercialize our visible light-activated nanocatalysts (Nanogrids) that had just been invented in our university lab as products for the water remediation market. Our initial focus was on our technology's potential for use in the Gulf oil spill clean-up efforts, the total target market value for which was an estimated $8bn back in 2010 (Boehm 2006, NOAA 2011) (see figure 1).
Figure 1. The initial business model canvas upon entering the I-Corps program.
Download figure:
Standard image High-resolution imageIn the course of the I-Corps program, our initial ideas and hypothesis on customer segments, product type, packaging and marketing were all challenged. Our team was sent out of the lab and we met in person or talked on the phone with more then 70 people (potential dealers, distributors, customers) related to the targeted market. We also reached out by email and surveyed another 30 potential customers.
To test the customer segment hypothesis, specific interviews were held with experts belonging to a diverse group spanning venture capital resources (e.g. Dow Chemical Ventures) to government/regulatory agencies (Department of Environmental Conservation; EPA) and from contractors for environmental remediation to water quality testing labs. Next we assessed the market type by interviewing marketers/dealers of environmental remediation products (e.g. ECS Environmental) and by approaching key distributors of such products. We even went on a field study to witness first-hand the challenges associated with remediating underground oil spills.
These exercises helped us identify our target market within weeks rather than the years it would have taken had we followed our initial hypothesis. Most importantly, we identified a key customer segment: 'produced water' discharged in an off-shore oil-producing area. Fracking is such an operation, an creating large amounts of wastewater (Schramm 2011). Current energy exploration and extraction in the US creates 15–20 billion barrels of produced water per year (Sanford 2010). Worldwide, estimates top 50 billion barrels (Veil 2004, Clark and Veil 2009). Energy companies pay somewhere between $3–$12 to dispose of each barrel of produced water (Sanford 2010). Our nanogrids may help eliminate this cost and environmental liability.
Our product is tailored to contain the volatile petroleum hydrocarbons (e.g. benzene) and subsequently decompose them either in-situ or off-site. It can be used as the final remediation step in the 'produced water' clean up and can, in principle, turn 'produced water' from wastewater to drinkable water while treating on-site. We learned from our 'cold calls' and other interviews that new legislation was expected to cancel the energy industry's exception from the Clean Water Act, thus favoring 'new, self-contained, on-site water treatment'. The market for treating produced water is estimated to exceed $4.3 billion by 2015 (Wolfe and Saif 2010). Thus, we had pivoted and experienced an 'epiphany' which helped us in focusing our efforts towards the energy industry (gas and oil extraction) and its need for remediating its wastewater. The final version of the business model canvas as developed throughout the course is shown in figure 2 below.
Figure 2. The final version of our business model as developed at the end of the program.
Download figure:
Standard image High-resolution imageIn summary, what we found was that we truly offer a new product for a niche segment of an existing market. While current remediation treatments of petroleum-oil contaminated water almost leave residual hydrocarbon contamination levels that prevent the disposal of the remediated water to the environment (Arthur 2005, Clark and Veil 2009, Guerra et al 2011), our nanocatalysts can be used to fully remediate hydrocarbons and to provide clean water. We found that industry likes our proposed product and pricing. Figure 3 below breaks out the pricing and packaging options for our product. Furthermore the program guided us on how to collect industry expert/customer testimonials that were critical to our marketing and fundraising efforts. Moreover, statements like 'For small spills your product is in competition with the cost of conventional absorbents plus landfill disposal. In this case, your $10 per sq. foot compares with $10–16 per ton landfill disposal' made by Dan Gray of Hepaco Inc., Tucker, GA, USA, helped us re-evaluate our pricing and packaging options.
Figure 3. Table estimating the cost of our product and comparing it with existing industrial absorbents (different technologies).
Download figure:
Standard image High-resolution imageIt was during this exercise at Stanford, led by legendary business figure and I-Corps founder Steve Blank, that our initial idea of using nanogrids for Gulf oil clean up failed and a new and brighter idea of self-supported photocatalytic mats for produced water remediation was born. Our 'magic clothes', as our demonstrated products, were characterized by Oren Jacob, former CTO of Pixar and I-Corps curriculum instructor, found a new market segment to target, and our team made the 'go' decision at the end of the program. Press releases and news reports refer to our emerging venture and a path to commercialization on which we still make strides have been abundant (https://web.stonybrook.edu/cnsd/formservertemplates/page.html).
3. Nanogrids for the remediation of petroleum-based hydrocarbons
Following the successful completion of the I-Corps program, the commercialization efforts of this technology are being continued by a start-up corporation run by our team members (Lead and PI), and ongoing negotiations for exclusive licensing of the IP owned by our team are in progress. Through the help of an NSF SBIR Phase I grant and other support from NYSERDA and the iClean incubator in Albany, NY, we are getting closer to optimizing the product for large-scale field testing. This is a quantum leap that has been facilitated by the I-Corps program and it speaks volumes to the impact of the latter in transforming university research and in bringing advanced technology to solve urgent problems and expanding industrial horizons.
As outlined below, our start-up operation has further developed the idea of designing the nanogrids for use as covers of fracking pits (i.e. pool covers) to completely decompose BTEX (benzene, toluene, ethylbenzene and xylenes) photocatalytically (and other toxic components in produced water) on-site, preventing them from vaporizing into the air. The processing, composition and proof of concept studies carried out on this water remediation nanotechnology are discussed below.
4. Fracking operations and produced water
Hydraulic fracturing, or fracking, is a technique used to release oil or gas trapped in rock/shale formations (Guerra et al 2011, Schramm 2011). Drillers inject millions of gallons of water, along with sand and other chemicals, at high pressure into a well. In the US alone, 1 million fracking wells produce 20.7 billion barrels of produced water each year. The US's shale oil production could reach up to 5 million barrels per day by 2017, which would make it the top oil producing country in the world (Guerra et al 2011, Schramm 2011). Properly cleaned fracking water could be highly valuable, and it will reduce concerns about the use of large amounts of water in the fracking process. The photocatalytic mats nanotechnology described here has the potential to provide a viable solution to the problem by attacking the removal challenge of BTEX and other toxic compounds.
BTEX, the most common organic contamination in produced water, and other volatile chemicals that are dissolved in liquids, can easily evaporate into the air when the liquid is exposed to the atmosphere (Arthur et al 2005). The rate of vaporization depends on many environmental factors (wind speed, temperature) and of course the concentration of the VOC (Arthur et al 2005). BTEX compounds are among the particular chemicals of concern in terms of their persistence, toxicity and carcinogenicity. The Environmental Protection Agency's new rule, the National Emissions Standards for Hazardous Air Pollutants (NESHAP) issued under section 112 of the Clean Air Act, is focused on BTEX emissions. The current permissive levels for chemical contaminants in drinking water are: maximum contaminant levels (MCL) (ug/liter=ppb): benzene 5; toluene 1000; ethylbenzene 700; xylenes (total) 10 000. Removing BTEX contamination is a challenging task as traditional methods of water treatment, such as flocculation, sedimentation, or filtration, do not remove low level contamination of organic compounds. The industry practice is the use of the best available technology, which in most cases is activated carbon.
Fracking pits, lagoons, or ponds are emerging as popular and widely used methods for storing produced water (Martel and Sahay 2013). A frac-water pond resembles an above-ground swimming pool. Economics favor the selection of ponds over tanks for storing fracking water as it is easy to set the former up with the help of a small crew in as little as 14 h (Igunnu and Chen 2012). Open-air pits on fracking sites, however, present a significant hazard, from the threats of leakage and flooding, and contaminating groundwater supplies to a pathway for human and animal exposure to chemicals through volatilization of toxic compounds in the pit pools. The cost of treatment depends on many factors, but ultimately is a function of the cost of the media used and the cost of their disposal. It is not practical to install industrial-scale carbon adsorption process systems in every well or pit, and the latest development involves the implementation of mobile units (e.g. Siemens units) that are rented out to well operators when there is the need for a short-term or primarily emergency use.
5. Current design and specifications of the nanogrids
The proprietary formula used to make the nanomats that is described in patent application PCT/US2012/044327 yielded a two-phase system consisting of CuO and CuWO4 (see figure 4). Scanning electron microscopy and transmission electron microscopy with electron diffraction analysis in figure 5 confirmed the interconnected nature of the particles of the two phases of the catalyst (nanogrid configuration) and the similarity in the particle sizes of the respective phases.
Figure 4. XRD pattern showing the two main phases of the photocatalyst: CuWO4 and CuO (Nanogrid).
Download figure:
Standard image High-resolution imageFigure 5. (left) Scanning electron microscopy micrograph showing the morphology of the CuO-CuWO4 Nanogrid; and (right) transmission electron microscopy of the same material showing 25 nm average grain size and the ring pattern selected area diffraction in the inset, confirming the two phases present as those shown in the XRD pattern.
Download figure:
Standard image High-resolution image6. Scaling up the size and performance of the photocatalyst
Figure 6 below shows the lab-based testing results of the efficiency of various catalysts in breaking down benzene in water. The high performance of the nanogrids is apparent.
Figure 6. Natural light testing of nine catalysts using the same solution of water + dyed benzene (top: day one and bottom: day nine). Note that the water in the fifth vial from the left that was treated with the Nanogrid is totally clear after exposure to sunlight.
Download figure:
Standard image High-resolution imageYet, the traditional needle electrospinning technique used to produce the nanogrids was of a very low production rate (~0.1 g h−1), and it did not lend itself to industrial scale yields. To process the catalysts in a fast and efficient manner, it was important to speed up the electrospinning process. The high-throughput facility depicted in figure 7 below was used for this purpose. This system used a 24+ multi-jet system to produce electrospun fibrous mats (Sood et al 2014). The 'bowl edge'-type design of the unique apparatus produced Nanogrids at a rate of 30 times faster than the traditional single needle jet process.
Figure 7. Schematic of the high-throughput electrospinning system used from Sood et al 2014; and the specifications of the conditions used to produce the nanomats (right).
Download figure:
Standard image High-resolution imageThe basic concept of the high throughput electrospinning by multiple jets is spinning from the base of a source that is the disc, while a pump regulates the flow of the polymer solution that is fed into the disc (Sood et al 2014). The precursor solution is pumped by the flowmeter. It builds pressure to the solution already filled in the disc, thereby forcing and pushing the solution out of the holes where at first it is a tiny drop at the edge of every hole, and then it stretches and gets pulled to the collector due to the electrostatic field applied between the disc and the drum (collector), finally forming fibers on the cylindrical collector drum. This is a unique facility as it allows the lab determined process and solution parameters based on a single jet to be applied intact to achieve scalable synthesis of the tailored mats.
The process parameters used were:
- working distance: 15 cm;
- applied voltage: 22 kV;
- flow rate: 0.45 ml min−1;
- collecting surface: 4662 cm2;
- production rate: 18 g h−1.
7. Scaled-up pilot study
Large scale testing in natural light to assess the photocatalytic activity of the scaled-up mats was performed in two glass aquariums (length 20" × width 10" × height 12") filled with 5 gallons of benzene contaminated water (50 ppm contaminant level)—one used as the control (see figure 8).
Figure 8. Images of the two sides of a scaled-up self-supported Nanogrid, and demonstration of its use in a pilot study carried out outdoors in open air.
Download figure:
Standard image High-resolution imageThe initial benzene concentration of this pilot study was 50 ppm. After 7 days of sun light exposure, the benzene level went down to 2.3 ppb level (see figure 9). According to EPA regulations, the maximum contaminant level of benzene in water is 5(ug l−1 = ppb). Thus the Nanogrids helped make the water potable and kept the benzene out of the environment.
Figure 9. GC–MS (gas chromatography–mass spectrometry) results of pilot study kept under natural sunlight for: top 5 days and bottom 7 days. After a week the water was clean enough to be drinkable. The photocatalyst also protected the environment from benzene vaporization.
Download figure:
Standard image High-resolution imageThe results above highlight that oxide-based solar energy-assisted oxidation is a feasible and probably the most promising route to effective, inexpensive, and eco-friendly BTEX remediation in produced water. It is as important to completely decompose the targeted contaminant as it is for the resulting products to be innocuous.
The advantage of using semiconductor-based photocatalysts to remediate contaminated water (photocatalytic decomposition of BTEX) is that complete mineralization of the pollutant is feasible. The conversion of a petroleum hydrocarbon to carbon dioxide, water, and water-soluble organics that are rapidly biodegradable by marine bacteria, is feasible through photocatalytic oxidation (29):

A directed self-assembly process involving metal diffusion inside polymer nanofiber mats produced by means of electrospinning and the resulting formation of 3D macroscale mats, Nanogrids, was described herein. CuWO4 utilizes the •OH radicals formed through oxidation of water and hydroxyl ions by the electron-hole separation. These oxidizing species along with other reactive species, such as H2O2, are capable of degrading the polluting substance.
Furthermore, the use of CuWO4 along with CuO was found to give the best response for the decomposition of benzene in water under visible light. As inferred by other workers (Yourey and Bartlett 2011), tungstates of Cu change the valence band with 3d orbitals, thus helping with absorption in the visible spectrum. The depth distribution of BTEX in frac pits is expected to be similar to those in lakes and other water reservoirs (Schmidt et al 2004), thus the photocatalytic cover solution is a viable option to clean up petroleum-based hydrocarbons in water using these photocatalytic mats.
8. Overview
Nanogrids is an innovative nanotechnology platform with unique functionality: it breaks down hydrocarbons using solar energy while floating on the water, and no direct counterpart of this technology exists. Some of its features are common to floating membranes, adsorption filters and photocatalytic dispersions, but it is none of these technologies. It is rather a unique ceramic nanotechnology processed by a nanomanufacturing method targeting a low-tech application (pit storage of produced water). It does not require the backwash clean up of ceramic membranes, makes no use of fluids or energy as photoelectrocatalysts do, and has no high carbon footprint like adsorption filters of activated carbon. It is a unique and disruptive technology that may be applied quickly by unskilled workers (the same people who lay down geomembranes on the pit) and which is reusable and does not absorb the pollutant but rather breaks it down to innocuous components. The transition of this technology from the lab to an industrial-scale prototype has taken less than three years, and such effective translation of research towards commercialization is a direct result of the pioneering and visionary I-Corps program. Our team continues the effort to bring a product to market in record time.
Acknowledgments
This work has been possible through the following grants from the National Science Foundation: DMR-1046599 (P.I.: P. Gouma); IIP-1156513 (P.I.: P. Gouma); IIP-1248807 (P.I.: J. Lee for Gouma Inc).
Biographies
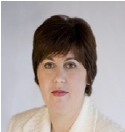
Pelagia-Irene (Perena) Gouma is a tenured full professor in the Department of Materials Science and Engineering of the State University of New York in Stony Brook, US, and the founder and director of the Center for Nanomaterials and Sensor Development (CNSD). She received her PhD in Materials Science and Engineering from the University of Birmingham in the UK, and carried out post-doctoral work at Ohio State University, prior to joining Stony Brook in 2000. Dr Gouma has published more than 120 peer-reviewed articles, several book chapters and a monograph. She also holds six US patents. She was a Fulbright scholar to UNICAMP in Brazil, and received the prestigious Richard M. Fulrath Award of the American Ceramic Society in 2013.
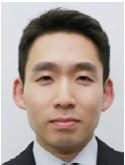
Jusang Lee received his PhD in Materials Science and Engineering at the State University of New York–Stony Brook in 2013. During this time he has participated in the NSF Innovation Corps (I-Corps) project as an entrepreneur lead, and has received the NSF Small Business Innovation Research (SBIR) phase I award. His research interests are the synthesis and characterization of nanostructured metal oxides as novel catalytic/electrocatalytic materials for environmental and energy applications.