Abstract
The effect of the Coriolis force due to the impurity toroidal and poloidal rotation on the in–out impurity density asymmetry in a rotating tokamak plasma is investigated. The role of the Coriolis force is figured out explicitly in the toroidally rotating plasma frame. The in–out impurity density asymmetry can be induced by the Coriolis force with , in this case, when moving along the magnetic field line from the outboard side to the inboard side in a magnetic flux surface, one sees a positive Coriolis force. The combined effects of the centrifugal force, the Coriolis force, the poloidal electric field and the ion-impurity friction force on the in–out impurity density asymmetry are discussed. The proposed theoretical model can be applied to improve the understanding of the in–out impurity density asymmetry observed in the tokamak experiments.
Export citation and abstract BibTeX RIS

Original content from this work may be used under the terms of the Creative Commons Attribution 3.0 licence. Any further distribution of this work must maintain attribution to the author(s) and the title of the work, journal citation and DOI.
1. Introduction
In the magnetic confinement fusion device, such as a tokamak, the heavy impurity ions exist due to the inward radial transport of the impurities released from the divertor plate and the first wall. Impurity transport, especially impurity pinch [1–3], is one of the hot topics in the magnetic confinement fusion area. Impurity accumulation in the core of a tokamak plasma is well known to be a big challenge because of fuel dilution and power loss from radiation. This has been an issue of great concern for several decades. Reduction or suppression of the core impurity accumulation is essential to realize a magnetic confinement fusion reactor, such as the International Thermonuclear Experimental Reactor (ITER) [4]. The experimental observations in the tokamaks [5–9] strongly support that the core impurity accumulation is mainly driven by the neoclassical inward convection [10]. Both the theoretical predictions [11–14] and experimental observations [15] show that the poloidally asymmetric impurity density distribution on a magnetic flux surface can significantly affect the impurity neoclassical transport; the impurity accumulation on the inboard side of a flux surface (in–out asymmetry) can reverse the neoclassical convection from inward to favorable outward direction [13, 16], which is beneficial for removing the impurities from the plasma core. Therefore it is worthwhile to understand the physical mechanisms that drive the in–out impurity asymmetry to avoid the impurity core accumulation through the external controlling.
The well-known centrifugal force effect due to the impurity toroidal rotation in a tokamak plasma can push the impurities to accumulate on the outboard side of the flux surface (out–in asymmetry), which has been pointed out theoretically by Hinton and Wong [17] and Wesson [18] earlier and observed in the tokamak experiments [19, 20]. But the centrifugal force effect can not explain the in–out impurity density asymmetry observed in the JET [21, 22], Alcator C-Mod [23–28] and ASDEX Upgrade [29, 30] tokamaks. To understand the in–out impurity density asymmetry observed in the experiments, the ion-impurity friction force model [31–33] and the ion cyclotron resonance heating (ICRH)-induced poloidal electric field model [25, 34] were proposed. The ion-impurity friction model was applied to explain the in–out asymmetry of the heavy impurities in the edge of the Alcator C-Mod tokamak [24, 26]; the in–out asymmetry of the heavy impurities observed in the core plasma in the JET [21, 22] and Alcator C-Mod [24, 25] tokamak can be due to the ICRH-induced poloidal electric field; the in–out asymmetry of the light impurities observed in the edge of the ASDEX Upgrade [29, 30] was thought to be mainly due to the combined effect of the ion-impurity friction force and the poloidal centrifugal force. The experimental observations in the Alcator C-Mod [23] and ASDEX Upgrade [29, 30] tokamak show that the in–out impurity density asymmetry is strongly related to the impurity poloidal rotation. In this paper we will consider a theoretical model to investigate the Coriolis force effect on the in–out impurity density asymmetry.
It will be shown that in a rotating tokamak plasma the impurity toroidal rotation combining with the impurity poloidal rotation generates the Coriolis force [35, 36], which can affect the in/out impurity density asymmetries largely. The Coriolis force effect has been included in the model used in [30, 37], which is derived in the laboratory frame. The role of the Coriolis force on the in/out impurity density asymmetries will be singled out explicitly in the toroidally rotating plasma frame in this paper. The physical mechanisms to induce the in–out impurity density asymmetry will be discussed in details.
The remaining part of this paper is organized as follows. In section 2, the theoretical model will be presented. In section 3, the effects of the Coriolis force and the poloidal electric field on the in/out impurity density asymmetries are discussed and a numerical example will be shown. The combined effects of the centrifugal force, the Coriolis force and ion-impurity friction force will be discussed in section 4. In section 5, the summary and conclusion will be presented.
2. Theoretical model
The physical picture of the effect of the Coriolis force on the in/out impurity density asymmetries will be explained firstly. In the toroidally rotating frame in a tokamak plasma, the impurity poloidal rotation generates the Coriolis force, , with
,
the impurity density and particle mass, respectively; here
and
are the toroidal rotation angular velocity and the poloidal rotation velocity of the impurities, respectively. This Coriolis force is in the toroidal direction, which can push the impurities along the magnetic field line. When moving along the magnetic field line from the outboard side to the inboard side in a flux surface, if the impurity sees a positive Coriolis force, the impurity is pushed by the Coriolis force to the inboard side and the in–out impurity asymmetry can be induced, otherwise the impurity is pushed to the outboard side and the out–in impurity asymmetry due to the centrifugal force is enhanced further. Therefore the effect of the Coriolis force on the in/out impurity asymmetries depends on both the direction of the Coriolis force determined by
and the helicity of the magnetic field line from the outboard side to the inboard side determined by the sign of
, whose absolute value is the usual safety factor in the tokamaks of large aspect-ratio with circular cross-section. Here
and
are the minor radius of the flux surface and the major radius at the magnetic axis, respectively;
and
are the toroidal and poloidal components of the magnetic field. This new mechanism, which has not been pointed out in the previous studies [25, 31–34], is schematically illustrated in figure 1.
Figure 1. Illustration of the Coriolis force in the toroidally rotating frame in a tokamak plasma with for (a)
; (b)
.
Download figure:
Standard image High-resolution imageTo proceed, we begin with the steady-state equations of continuity and momentum,


with the impurity fluid velocity,
the fluid pressure,
the charge of the impurity ion;
is the magnetic field and
is the friction force between the impurity and the bulk ions, which can cause the in–out impurity density asymmetry as pointed out in [24, 26, 30–33]. The ion temperature anisotropy effect, which can cause the in–out impurity density asymmetry as discussed in [24–26, 30, 34, 37], will not be considered in this paper. The poloidal electric field can be due to the centrifugal force effect on the bulk ions [17, 18] or the minority ion heating [25, 34]. Here we will consider the poloidal electric filed due to the redistribution of the bulk ions on the flux surface due to the Coriolis force effect on the bulk ions besides the centrifugal force effect.
The magnetic field is given by
. We choose a right-hand coordinate system
.
The lowest order divergence-free impurity mass flow, which satisfies equation (1), can be written as [38–41]

where is a function describing the impurity poloidal rotation
and the impurity flow normal to the flux surface
and
.
Substituting equation (3) into (2), one can write the - and
-components of the inertia term in equation (2) as


From the -component of equation (2), one finds
.
Here, is the impurity ion gyro-frequency evaluated with the poloidal magnetic field. There is non-zero radial flow due to the impurity toroidal and poloidal rotation [38–41] besides the impurity radial flow due to the toroidal component of the friction force. With the tokamak plasma of circular cross-section and the impurity toroidal rotation assumed to be rigid on the flux surface, the impurity flow normal to the flux surface is estimated to be
if
, which is satisfied with
and
in the typical tokamak plasmas. Therefore the effect of radial flow,
, shall be ignored in the following. Then equations (4) and (5) are reduced to


The right-hand-side of equation (7) corresponds to the Coriolis force in the toroidally rotating frame generated by the impurity poloidal rotation.
The impurity density poloidal variation can be obtained through the B-parallel component of equation (2)


To obtain equation (8b), the impurity temperature is taken as a flux surface function; and
are used. The parallel ion-impurity friction force can be written as
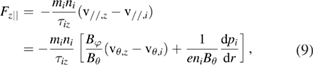
where is the ion-impurity collision time. To obtain equation (9), the radial force balance equations of the impurity and the bulk ions are used and the impurity diamagnetic term is neglected due to its high charge number. The thermal force due to the bulk ion temperature gradient [29, 30] is neglected here. With the help of equations (6) and (7), it is not hard to derive

With , equation (10) can also be written as

The impurity density poloidal variation in a rotating tokamak plasma is determined by equation (11), which is valid for the arbitrary aspect-ratio and shaped tokamak plasma. It can be found that the impurity density poloidal variation is affected by (1) the centrifugal force due to the impurity toroidal rotation; (2) the Coriolis force due to the impurity toroidal and poloidal rotation; (3) the poloidal centrifugal force due to the poloidal variation of the impurity poloidal rotation; (4) the poloidal electric field; and (5) the parallel ion-impurity friction force. The effects of (1) and (4) on the out–in impurity density asymmetry have been discussed in [17, 18]. The in–out impurity density asymmetry due to the poloidal centrifugal force effect has been discussed in [29, 30]. The important role of the parallel ion-impurity friction on the in–out impurity density asymmetry has been pointed out in [24, 26, 30–33]. The impurity density poloidal variation in a rotating tokamak plasma can be obtained by solving equation (11).
To demonstrate the main underling physics for the in–out impurity density asymmetry, we consider a tokamak plasma of large aspect-ratio with circular cross-section. The impurity toroidal rotation will be assumed to be rigid on every flux surface. Then equation (11) will reduce to
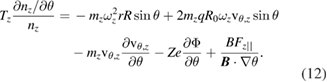
To obtain equation (12) from (11), and
are used.
To discuss the respective contributions to the impurity density poloidal variation, the poloidal-dependent impurity poloidal rotation is expanded as with the up-down symmetric
considered, where
and
are the poloidal-independent and the lowest order cosine-component of
respectively. The ratio between the first three terms on the right-hand-side in equation (12) will be
, where
. In the core tokamak plasma with
, the effect of the centrifugal force due to the impurity toroidal rotation will be dominant; the effect of the Coriolis force will be comparable to the centrifugal force effect and the poloidal centrifugal force effect can be neglected with
; while in the edge regime with
, both the effects of the Coriolis force and the poloidal centrifugal force can not be neglected. Even though the important role of the poloidal centrifugal force on the in–out impurity density asymmetry that has been pointed out in [29, 30], the effect of the Coriolis force is much larger (factor
) than that of the poloidal centrifugal force with
. Hence in the following, we will neglect the poloidal centrifugal force and the impurity density poloidal variation will be determined by the following equation,
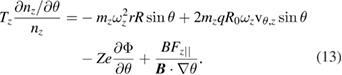
3. Coriolis force effect
To focus on the Coriolis force effect on the in–out impurity density asymmetry, we will neglect the ion-impurity friction force artificially in this section. Integrating equation (13), one finds
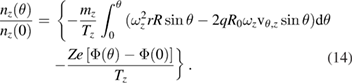
To find , we consider the deuterium as the bulk ion. The bulk ion density poloidal variation due to the bulk ion toroidal and poloidal rotation can be derived in a way similar to the above,
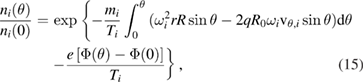
where ωi and vθ,i are the toroidal rotation angular frequency and the poloidal rotation velocity of the bulk ions. The inertia effect can be neglected for the electrons due to the small mass. Then we can have the Boltzmann relation . For the trace impurities,
, the quasi-neutrality condition is simplified to
. Therefore we find

This indicates that a poloidal electric field can be generated through the bulk ion poloidal redistribution due to the centrifugal force and the Coriolis force in a rotating tokamak plasma.
Substituting equation (16) into (14), we can obtain

with ,
. To obtain equation (17), the terms higher than
have been neglected. For the DIII-D experiments [42], in which both the toroidal rotation of the impurity and the bulk ions are measured,
is estimated. We will assume
in the following. The poloidal rotation of the impurity and the bulk ion could be in the same or opposite direction, while
can be found from the experimental measurements [43].
The in–out asymmetry factor of the impurity density can be derived analytically with ,
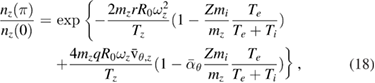
where . In the bracket on the right-hand-side of equation (18), the first term and the second term are the effects of the centrifugal force and the Coriolis force, respectively, which are modified by the poloidal electric field through the terms proportional to Z. The effect of the centrifugal force pushes the impurities to the outboard side, this effect can be weakened by the poloidal electric field, as is similar to [17, 18].
The Coriolis force term can be negative or positive, depending on the sign of when the effect of the poloidal electric field is ignored; note that the sign of q represents the direction of screwing of the magnetic field line from the outboard side to the inboard side, as is illustrated in figure 1; the sign of
represents the direction of the Coriolis force, as is also illustrated in figure 1. When the Coriolis force term has the same sign as that of the centrifugal force term, i.e.
, the out–in impurity asymmetry induced by the centrifugal force is further enhanced by the Coriolis force. When the Coriolis force term has the sign opposite to that of the centrifugal force term, i.e.
, the in–out impurity density asymmetry can be induced by the Coriolis force, if it is strong enough to overcome the centrifugal force effect.
It can be straightforwardly proved that the sign of can be simply determined in the following way: for
in the co-current direction,
will be negative with
in the ion-diamagnetic drift direction and positive with
in the electron-diamagnetic drift direction; for
in the counter-current direction,
will be negative with
in the electron-diamagnetic drift direction and positive with
in the ion-diamagnetic drift direction. This statement is also true for determining the sign of
.
It should be pointed out that the effect of Coriolis force can be modified by the poloidal electric field; when , the effect of the Coriolis force is enhanced by the poloidal electric field; when
, the effect of the Coriolis force is weakened; when
, the effect of the Coriolis force is reversed. Note that the effect of poloidal electric field pointed out here is different from [25, 34].
As an application of the proposed theoretical model, we calculate the in–out density asymmetry of the impurity Boron observed in a rotating ASDEX Upgrade tokamak plasma [29, 30]. The main parameters are
,
,
,
,
,
,
,
.
we have chosen is in the direction of the plasma current; the plasma current is in the counter-clockwise direction and the toroidal magnetic field is in the clockwise direction viewed from above; the toroidal rotation of
and
are both in the co-current direction; the impurity poloidal rotation is in the electron-diamagnetic drift direction. The sign convention is consistent with that in [29, 30]. Since there is no
measurements, we assume
according to [43]. The in–out asymmetry factor of
is evaluated, according to equation (18), to be
for
, respectively, which is qualitatively consistent with the experimental observations [29, 30]. The accurate quantitatively validations of the proposed model should be carried out with the measurements of both the poloidal rotation of the impurity and bulk ions in the tokamak experiments in the future.
The effects of the Coriolis force and the poloidal electric field on the in/out density asymmetries are evaluated by scanning
and
. The contour plot of the in–out asymmetry factor of
in
plane is shown in figure 2. The bottom-left corner indicates an in–out asymmetry due to the Coriolis force (enhanced or weakened by the poloidal electric field); the bottom right corner indicates an out–in asymmetry due to the centrifugal force (enhanced or weakened by the Coriolis force and the poloidal electric field); the upper-right corner indicates an in–out asymmetry due to the effect of the Coriolis force reversed by the poloidal electric field; the upper-left corner indicates an out–in asymmetry due to the effect of the Coriolis force reversed by the poloidal electric field in addition to the centrifugal force. Therefore the in–out impurity asymmetry observed in the ASDEX Upgrade tokamak corresponds to the bottom-left corner, as is shown in figure 2; one concludes that the Coriolis force plays an important role in the in–out impurity asymmetry observed in this experiment.
Figure 2. Contour plot of the in–out density asymmetry factor of the impurity Boron in
plane with
,
,
.
: the impurity poloidally rotates in the ion(electron)-diamagnetic drift direction. The color symbols represents
and
, respectively.
Download figure:
Standard image High-resolution image4. Combined effects of the Coriolis force and the ion-impurity friction force
With the ion-impurity friction force included, the impurity density poloidal variation (equation (13)) can be solved with the impurity density expanded as .
With the trace impurity assumption, the poloidal electric field can be obtained from the quasi-neutrality condition with the help of the momentum equations of the bulk ions and electrons,

The solution of equation (13) is then found to be


where ,
,
,
,
.
To obtain equation (20), and
are used.
Without the Coriolis force effect, equation (20) is similar as the results in [32, 33]. The ion-impurity friction force due to the large pressure gradient of the bulk ions can cause the in–out or out–in impurity density asymmetry, which depends on the direction and the magnitude of the bulk ions poloidal rotation. The combined effects of the centrifugal force, the Coriolis force and the poloidal electric field discussed in section 3 will be reduced by the factor . With
, i.e.
, the effect of the ion-impurity friction on the in/out impurity density asymmetries can be negligible and the in/out impurity density asymmetries will mainly be determined by the combined effects of the centrifugal force, the Coriolis force and the poloidal electric field as discussed in section 3. With
the ion-impurity friction force will induce the in–out impurity density asymmetry; with
the ion-impurity friction force will enhance the out–in impurity density asymmetry due to the centrifugal force effect. The effect of ion-impurity friction force will be dominant with
. In addition, the ion-impurity friction force can induce up-down or down-up impurity density asymmetry, which depends on the directions of the toroidal magnetic field, the direction and the magnitude of the Coriolis force and the bulk ions poloidal rotation.
The combined effects of the Coriolis force and the ion-impurity friction force on the in–out impurity density asymmetry are numerically demonstrated in figure 3. The bulk ion poloidal rotation is scanned artificially with the other parameters fixed. The toroidal rotation of the impurity and bulk ions are in the co-current direction and the impurity poloidal rotation is in the electron-diamagnetic drift direction. With , the combined effects of the centrifugal force, the Coriolis force and the poloidal electric field induce the in–out impurity density asymmetry as discussed in section 3. With the ion-impurity friction force effects included, i.e.
, the in–out impurity density asymmetry can be enhanced or weakened. With
, the in–out impurity density asymmetry decreases with the ion-impurity friction force effect increased. This is due to that the ion-impurity friction force induces the out–in impurity density asymmetry and weakens the in–out impurity density asymmetry. With
, the ion-impurity friction force induces the in–out impurity density asymmetry while the in–out impurity density asymmetry due to the combined effects of the centrifugal force, the Coriolis force and the poloidal electric field is weakened. These two effects compete with each other. The total in–out impurity density asymmetry decreases with the weakening effect stronger than the enhancing effect.
Figure 3. Numerical demonstration of the combined effects of the centrifugal force, the Coriolis force, the poloidal electric field and the ion-impurity friction force on the in–out impurity density asymmetry with
,
,
. The other parameters are same as that in figure 2. The dashed line denotes
.
Download figure:
Standard image High-resolution imageThe poloidal rotation of the bulk ions and the impurities are strongly related with the pressure and temperature gradients of the bulk ions according to the neoclassical theory [43] and the experimental observations [44–47]. Therefore both the the Coriolis force and the ion-impurity friction force will play an important role in the formation of in–out impurity density asymmetry in the large gradients region, such as in the ITB and pedestal regions. The ultimate impurity density poloidal asymmetry should be determined by the combined effects of the centrifugal force, the Coriolis force, the poloidal electric field and the ion-impurity friction force.
5. Summary and conclusion
In summary, the effect of the Coriolis force due to the impurity toroidal and poloidal rotation on the in–out impurity density asymmetry in a rotating tokamak plasma is investigated. In the toroidally rotating plasma frame, the effect of the Coriolis force is figured out explicitly. The in–out impurity density asymmetry can be induced by the Coriolis force, if one sees a positive Coriolis force when moving along the magnetic field line from the outboard side to the inboard side in a magnetic flux surface. It is essential to consider the combined effects of the centrifugal force, Coriolis force, the poloidal electric field and the ion-impurity friction force to explain the in–out impurity density asymmetry observed in the experiments. The proposed theoretical model can be applied to improve the understanding of the in–out impurity density asymmetry observed in the tokamak experiments and provide an opportunity for active controlling of the direction of the impurity neoclassical convection in the tokamak plasmas by controlling the plasma rotation.
Acknowledgments
This work was supported by the National Natural Science Foundation of China under Grant Nos. 11575246, 11375196, 11405174, 11505240 and the National Magnetic Confinement Fusion Program of China under Contract Nos. 2013GB107004, 2014GB113000.