Abstract
This paper presents the case for how students can be helped to increase their scientific vocation by experimental work and the introduction of particle physics into pre-university studies. These two ideas are the two main lines of work of the ADMIRA initiative, which has been created by individuals belonging to different and complementary educational and research institutions. The initiative consists of a network of schools that share Minipix devices, a readout system for the Timepix detector designed at CERN in the framework of the Medipix2 Collaboration. The schools receive logistical and technical support from the local University (the University of Barcelona) and from CERN. The centres involved and the objectives, as well as the characteristics of the project are presented. The technical and didactic materials available are also shown. Finally, some initiative data has been analysed to show its rapid growth, and some students' research is presented showing the diversity of tasks that can be done with such detectors.
Export citation and abstract BibTeX RIS

Original content from this work may be used under the terms of the Creative Commons Attribution 4.0 license. Any further distribution of this work must maintain attribution to the author(s) and the title of the work, journal citation and DOI.
1. Introduction
The ADMIRA 15 Project is an initiative that aims to bring particle physics to schools through experimentation, in order to promote scientific vocations amongst students, by using Timepix detectors, designed at the European Organization for Nuclear Research (CERN) by the Medipix2 Collaboration. This activity began with the collaboration of several professionals in the field of education, research, and innovation in 2017 and is gradually being consolidated as an educational program with unique characteristics in terms of methodology and results.
This paper explains the importance of the initiative from the point of view of physics teaching, the characteristics and particularities of the approach, the tools it uses and the activities it proposes. Finally, some data and the results of the project's impact on participating schools are presented in order to encourage the implementation of similar initiatives in other regions in Europe or beyond.
1.1. Particle physics in pre-university teaching in Catalonia
One of the characteristics of the pre-university physics curriculum in Spain, as well as in other countries, is the lack of concepts and models corresponding to physics developed during the second half of the 20th century (Tuzón and Solbes 2016). In particular, in the region of Catalonia, the students of compulsory secondary education (12–16 years) do not have any formal training on the Standard Model (SM), one of the most successful theories in modern physics. Only the pre-university students studying the scientific-technological option of the baccalaureate (17–18) (45% of the total studying pre-university studies (Departament d'Educació 2019)) and choosing physics as an optional subject, have an introductory course on quantum and nuclear physics. These topics introduce, very superficially, the basic concepts of the SM: interactions, fundamental particle families, intermediate bosons, etc. We, therefore, believe that approximately two-thirds of the high school students do not receive any formal training about the SM or any other physical model of the present day. This fact affects students in several respects:
- The student's interest in the subject of physics is diminished, as these concepts and models are not explained. These concepts of modern physics are those that appear in the news, outreach articles and social media, resulting in the perception that there is a gap between physics done at school and what the learner sees as physics in everyday life (Solbes 2013).
- The lack of identification between students and scientists due to the remoteness of the scientists presented within the curriculum (Galilei, Newton, Maxwell, etc). It is easier to identify with scientists from the second half of the 20th century (Feynman, Penrose, Hawking, etc) with values and attitudes more similar to those of the current day students, than with scientists from the first half of the 20th century and earlier (Planck, Einstein, Bohr, etc). This is especially significant for girls, who see their female references in physics, limited to a few exceptional cases. Although the recognition of female scientists in the SM is not yet complete and glass ceiling situations continue to occur (Ivie and Stowe 2021), many more cases of female success, both in science in general and in physics in particular, may be identified in the second half of the 20th century (Madsen et al 2013).
- Their decision-making capacity as future citizens is conditioned by their lack of knowledge of modern physics. When making decisions of a political nature or participation as a citizen in society, the failure to study the latest models of physics makes it difficult for citizens to understand the current state of fundamental research, the importance of basic science and investment in research, thereby de facto affecting their ability to make informed decisions (Henriksen and Jorde 2001).
- It could be noted that many people nowadays have a false perspective about radioactivity, students included (Prather 2018). That can lead to negative behaviour against nuclear physics and its application. Educating students about the benefits of using nuclear physics in medicine, archaeometry, material reconstruction in industry, among others, is essential to avoid these prejudices.
Other models of current physics (General Relativity and Gravitational Waves, Cosmology and Dark Matter) may provide the same benefits as the SM, but are more difficult to be carried out experimentally in a school lab, making understanding of the abstract concepts involved more complicated, as argued in the following subparagraph.
1.2. The importance of experimentation in higher education
There is an abundance of literature and studies on the importance of experimentation in secondary education in science, both defending it (Hodson 1992, Roth 1994) and showing its limitations (Abrahams and Millar 2008).
When introducing the basic concepts of the physics of the standard model, or even the atomic-molecular theory of matter, the difficulty that students have in accepting the description of the model, is high (Novick and Nussbaum 1981, Morales and Tuzón 2020). The fact that the mathematics involved in theoretical development is well above the level that the students have previously acquired, also does not help to make a formal mathematical description of the subject (Chiu 2015).
Therefore, in the case of physics education of the SM for secondary school students, we could say that experimentation is central to being able to understand what teachers are talking about when introducing these concepts. Similarly, the introduction of modelling (Tuzón and Solbes 2017) and rendering activities—such as Feymann diagrams or other representations (Wiener et al 2017, Nikolopoulos and Pardalaki 2020)—are very valuable tools for model understanding. In addition, many students have false or so called 'alternative' ideas (Prather 2018) about irradiation which could be proven wrong with the proper use of Timepix detectors.
Furthermore, the use of Timepix detectors and their effect on the motivation of the students has been widely documented (Parker et al 2018, Parker et al 2019) by the Institute for Research in Schools (IRIS), as well as its effects by increasing the STEM vocations, especially among girls (IOP 2017, Archer and DeWitt 2021).
1.3. Catalonia as an educational region and its particularities
In the educational system in Catalonia 16 , the students have two specific subjects in the curriculum that are aimed at introducing students to research.
- The first of the subjects, called the Research Project (Diari Oficial de la Generalitat de Catalunya 2019a), is assigned to all Catalan students in the fourth compulsory secondary school course (15–16 years). In this assignment, groups of students must carry out tutor-oriented research work. This project consists of a set of research and discovery activities, carried out by the students on a topic chosen and supported, in part, by themselves, but also under the guidance of a teacher. Throughout the project, the students must show their ability at self-discipline and initiative in the organisation of their individual work, as well as their capacity for cooperation and collaboration within a team.
- The second, called Research Task (RT) (Diari Oficial de la Generalitat de Catalunya 2019b), is carried out by all Catalan baccalaureate students (16–18 years). This assignment runs between first and second baccalaureate courses and makes up 10% of the baccalaureate grade. This subject consists of a set of structured and research-oriented activities, carried out by the student in a field they have chosen and delimited, with the orientation of the teacher. This involves both laboratory and/or field activities as well as bibliographic documentation activities.
The ADMIRA initiative has taken advantage of these subjects by allowing the students to use Timepix as a tool to do their RT. This has led to a diversity of student investigations in many fields and also has created links between the students and their teachers and scientists in local and international institutions. On the other hand, the detector has been widely used at high school physics subject by 16–18 years old students.
2. Timepix detector
The Timepix chip is a hybrid pixel detector consisting of a matrix of 256 × 256 pixels with 55 µm pitch. The chip was designed at CERN in the framework of the Medipix2 collaborations. A review of the detailed technical specifications of the chip can be found in (Llopart et al 2007, Llopart 2007).
A hybrid pixel detector is a 2-dimensional matrix of microscopic radiation-sensitive elements each of which is connected to its own pulse processing electronics (Campbell 2001). The sensor element and the readout electronics are implemented in different substrates and connected together through fine pitch flip chip technology. When a particle interacts with the sensor, it can deposit its energy, or a fraction of it in the material creating charge carriers. The charge carriers move to the collecting electrodes, under the influence of an electric field, inducing an electric current which is processed in the readout electronics.
The physical separation between the sensor and the readout electronics allows to optimize the choice of the sensor material depending on the application. For example, in High Energy Physics, hybrid pixel detector readout chips are used with silicon as sensor material because of its low mass, its homogeneity and low cost. Other applications, for example x-ray radiography, use high Z sensor materials like GaAs or CdTe. A cross section of a hybrid pixel detector is shown in the picture below (figure 1).
Figure 1. (Left) Picture, not to scale illustrating the structure of a hybrid pixel detector. The sensor material is on the top and it is connected through flip chip to the readout electronics in the bottom. (Right) cluster of 55 µm pixels in which signal was induced by an alpha particle event deposited on the sensor measured with the Timepix chip.
Download figure:
Standard image High-resolution imageThe readout electronics consists of a charge sensitive amplifier (Krummenacher 1991) that increases the signal from the sensor. The amplifier is followed by a discriminator that compares the signal deposited by the particle with a threshold level. This means that a pixel only records hits, when the energy deposited exceeds the threshold. This leads to a noise hit free system and allows to measure long shutter times without noise hits. This is a feature that makes these detectors suitable to measure very low radiation levels like those coming from weak natural radiation sources in the school environment or the signals from cosmic rays. In Timepix, the energy deposited by every particle is measured by recording the number of clock cycles that the discriminator output is above the threshold level (this is usually called Time-Over-Threshold). That measurement is proportional to the energy deposited.
The high segmentation achieved by the Timepix devices, and the effects related to the induction of signal in the pixels, allow to identify different particle species in the radiation environment, based on the signature, for every particle, of its characteristic interaction with the detector material. That is, indeed, one of the most valuable characteristics for the educational use of the detector.
For example, low energy X- or γ photons deposit their energy in the sensor through photoelectric effect or Compton scattering in a localized volume that, in the case of Timepix, results in a single pixel hit, or in a small cluster of pixels when there is charge sharing (Ballabriga et al 2016). Alpha particles are identified as large 'round' shapes due to their high energy deposited shallowly, which leads to great transient currents in pixels neighbouring the initial impact point (see figure 1 for a 5.4 MeV alpha particle). Beta particles show substantial deviations in the path because the particle mass is equal to that of orbital electrons in the material with which it is interacting. Muons, which have high kinetic energy, are observed as straight paths (see figure 2).
Figure 2. (Left) Illustration of the interaction of a beta particle (top) and muon (bottom) with the sensor. (Right) measurement of the particle track with Timepix.
Download figure:
Standard image High-resolution imageThe readout system can be made very compact (Granja et al 2018a, 2018b) and the measurement results can be obtained in real time (Turecek and Jakubek 2015). An example is shown in figure 3 where the detector can be shown acquiring data in an aircraft. The image shows a 1 min frame taken at an altitude of ∼5000 m.
Figure 3. (Left) Image of the setup for taking measurements in an airplane. The setup consists of the MiniPIX device read out by a personal computer. (Right) results of a 60 s acquisition at 5000 m height.
Download figure:
Standard image High-resolution image3. The ADMIRA project structure and characteristics
The ADMIRA initiative originated in late 2017 arising from the collaboration of several individuals from different institutions with the aim of creating a local network of schools using Timepix detectors designed at CERN to introduce particle physics into the classroom. In order to do so, it has established, since September 2019, a loan system for the detector for a period of a few weeks for the different schools participating in the initiative. It also provides training to the teachers to optimally use the detector and on topics related to particle detection. The initiative was inspired by the work by B Parker with the Institute for Research in Schools initiative (IRIS 2021).
This diversity and complementarity of the institutions and proximity at the local level are perhaps the two most characteristic features of the project. They are analysed in the following paragraphs.
3.1. Institutions involved in the initiative
The ADMIRA Project works with the collaboration of institutions in diverse fields, such as schools and educational programmes, universities and research centres. In addition, collaboration with Institute of Professional Development and of Science Education (IDP-ICE) of the University of Barcelona (UB) has led to awarding certifications of attendance to the lectures to the teachers participating in the program.
3.1.1. Microelectronics section at CERN.
The technology of hybrid pixel detectors was created at CERN to address the challenging requirements of vertex detectors (Heijne et al 1988). Hybrid pixel detectors with on pixel pulse processing were first tested in a three pixel chip telescope in 1991 (Anghinolfi et al 1992) and successfully used in the lead beam experiment WA97 (Alexeev et al 1995). The results proved that noise hit free images can be taken at high frame rates (Antinori et al 1995).
The Medipix collaborations were later created (Campbell 2011) to develop hybrid pixel detectors and their applications in fields beyond High Energy Physics. The technology has been transferred to many fields of science including radiography, computed tomography, non destructive testing, synchrotron applications, electron microscopy, material analysis using x-ray diffraction, space dosimetry, and other applications (Ballabriga et al 2020).
As for the ADMIRA initiative, the Medipix ASIC design team in the microelectronics section at CERN, have provided two Timepix detectors, they have coordinated the teacher training sessions, enabled contacts between the school network and local scientists and provided technical support.
3.1.2. Institute of Cosmos Sciences of the University of Barcelona (ICCUB).
The ICCUB is an interdisciplinary research institute dedicated to fundamental research in the fields of cosmology, astrophysics, and particle physics by focusing its research lines on the study of the origin and fate of the universe, its current structure, and its constituents.
This institution is responsible for providing the initiative with various logistical resources: it hosts and maintains the project's website, it is responsible for managing the detector loan system and provides meeting rooms for the training sessions. In addition, it provided access to its laboratories and facilities (e.g. x-ray tubes) to students of the schools participating in the programme.
Moreover, some of their scientists have participated in lectures to students and teachers.
3.1.3. Schools and learning programmes.
The first experience with the Timepix detectors started in the Sagrada Família school in Gavà, in 2017. At the school, the first didactic activities with the detector were designed and introduced in the classroom. After the experience in the Sagrada Família school, other schools in the local area had access in 2019 and the web and the materials for the loans were created (ADMIRA 2021a).
Some of the schools in the network have actively contributed to develop and sharing teaching materials. For example, La Salle de Manlleu School and Learn It With Us educational programme (Liwu 2021) have created new activities based on the Timepix detector and have analysed and compared their measurements with those from detectors developed in the framework of the CERN S'Cool LAB's. In their work, they have captured atmospheric radon with the help of an electrically charged balloon and measured its half-life.
3.2. ADMIRA project goals
As said before, the initiative aims to bring research closer to the students in order to promote new scientific and technical vocations. To do so, the project uses MiniPIX detectors, the Timepix readout system developed by ADVACAM (ADVACAM 2021), available to students.
In order to reach as many students as possible, the initiative does so through its teachers. This allows the creation of a network of schools that have access to these instruments and share the results of experiments and the data generated. This data is shared on the project website, designed for the exchange of information among schools.
The contacts that are created between secondary school students, PhD students at the university and researchers are an important part of the project because this helps inspiring and motivating the younger students. In the framework of this initiative, secondary school students have done measurements with x-ray sources at the university laboratories with the guidance of university professors and PhD students. This is a particular example on how the initiative creates links between the different centers.
At the end of the academic year, a conference was going to be organised, in which students from participating schools would present the results of their experiments, as is already done in other similar initiatives. Unfortunatelly, the pandemic situation forced it to be cancelled. Despite of that, some students participated at the Young Photonic Congress (ICFO Outreach 2018) to explain their investigations with Timepix detectors.
The work with the detector enables students to learn concepts about many different fields. Some of the fields that have been studied in the classrooms are natural radiation sources, interaction of radiation with matter, electronics and data/image analysis with algorithms programmed by the students. Examples of this work are provided in the section 4 of this paper and can also been retrieved from the project's webpage.
3.3. Resources
This section details some of the initiative's resources and activities. However, the main asset of the ADMIRA Project is the two MiniPIX detectors that are provided to schools for (a) laboratory training sessions, (b) the investigation at the students' RT or (c) demonstrations in the classrooms. The importance of this resource requires a more extensive explanation, which can be found in previous sections.
How teachers use the detector in the classroom is left up to them, although some guidance and help is provided as is explanined below.
3.3.1. The web page.
The project website (ADMIRA 2021a) is hosted on the servers of ICCUB. Its main functions are (a) to present the initiative and its objectives, (b) to provide educational guidelines for the teachers on how its activities are related to the curriculum in different subjects and courses and (c) to be a space where pedagogical resources and measured data can be shared between the different schools in the network. In addition, it provides the detector booking service through the Agenda section.
3.3.2. Loan kit.
When a school involved in the ADMIRA Project books one of the detectors, it is assigned a time slot of a few weeks to use it. The pickup point is located at the physics faculty of UB, and the teacher receives a kit (see figure 4 below) that contains the ADVACAM's MiniPix detector, a USB cable and a pen-drive with the software and configuration files needed to run the detector. Moreover, a printed copy of the 'Introduction to Medipix' workshop guide is provided in order to introduce teachers and students to the use and capabilities of the MiniPIX detector and, at the same time, show them some experimental particle physics' concepts.
Figure 4. ADMIRA Loan Kit content. From top to bottom and from left to right, 'Initiation to Medipix' workshop guide, Quick start sheets, folder with MiniPIX detector and pendrive with PixetPro Software and the 'Experiment book'.
Download figure:
Standard image High-resolution imageThe kit also contains instructions on how to operate and calibrate the detector and a book with a collection of laboratory experiments based on Timepix devices (Vícha 2017). No radioactive materials are provided, but a list with examples of natural radioactive sources is shared.
In addition, a set of three Quick Start sheets is included: a welcome card with the explanation of the initiative and the content of the kit, another one with PixetPro program installation and configuration instructions and a Timepix operation booklet, with basic instructions on how to make the detector work.
3.4. An activity example. The Laboratory Workshop: Introduction to Medipix. Study of radiation and its properties. Comparison of classical and relativistic models in alpha and beta radiation
In order to share some of the examples for the use of Timepix detectors in teaching particle physics in pre-university studies, the laboratory exercise that is proposed for the students and teachers to familiarize themselves with the detector is explained below. Although this activity is given as a printed document within the detector's kit, it can also be consulted on the project's webpage (ADMIRA 2021b). The workshop guide is presented to teachers in two parts. In the first part, the most significant didactical indications are given, as well as the curricular aspects covered in the exercises and some guidelines to carry out the activity, both with and without detectors, in addition to a small introduction to Inquiry-Based Learning 17 . The second part presents the handout forms to be filled in by the students.
The objectives of the workshop involve advanced concepts on particle physics and scientific models, and allows students to start using a Timepix particle detector and PixetPro software.
After a short introduction to radioactivity and a brief description of the properties of alpha, beta and gamma radiation, some quantum and relativistic concepts and equations are reviewed. All these concepts are covered on the Physics curriculum. They include the dual nature of particles and waves (photon's momentum and De Brogglie wavelength), the energy-mass equivalence principle and the relativistic expression of kinetic energy. The final goal is to present the relationship between the speed and the ratio of the the kinetic and rest energy of the particle as shown below,
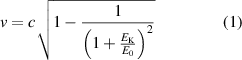
where ν is the speed of the particle, c is the speed of light in vacuum, EK is the kinetic energy of the particle and E0 is the rest energy.
Following this theoretical introduction, some information about the Timepix detector and the PixetPro software is provided, in order to allow students to undestand how the the particles interact with the sensor material and how the path trajectory is obtained. This also shows them how to operate the detector to acquire data.
Once this part is concluded, some indications to perform the experiment are provided to help students to take measurements. They learn to configure the detector in different modes (integral and count mode aswell as frame and energy modes).
In the first part of the activity, they are asked to draw (or to take a screenshot) and analize the diferent trajectories they can observe, as are shown in figures 1 and 2.
Through the identification of the different types of radiation by the trace left in the detector, students can relate these tracks to the characteristics of size, mass, and electric charge of the different particles that produced them.
In the second part, students measure the kinetic energy of the particles deposited in the detector, by selecting one whole path and reading the total energy on the PixetPro's Image Info panel as is shown in figure 5.
Figure 5. Image Info panel of PixetPRO software, showing the measured data of a single beta track. The Total value is the deposited energy (in keV) at the detector, which corresponds to the kinetic energy, assuming all energy is absorbed by the detector. This assumption is not strictly speaking true for high energy particles, like atmospheric muons.
Download figure:
Standard image High-resolution imagePerforming some calculations with equation (1), as is shown in figure 6, the students can obtain the speed of the particle according to the relativisitc and classical models and compare it to the speed of light. With this value, they are asked to compute the De Broglie wavelength of the particle at that speed.
Figure 6. Student's beta calculated data from the measured energy Ek for several beta particles, using classical and relativistic models. Wavelenght is also computed to analyze the quantum nature of beta radiation. The same calculations are performed for alpha radiation.
Download figure:
Standard image High-resolution imageTo help them to understand the meaning of their results, a simple graphical representation of the obtained values is required, so they can check the equivalence of the relativistic model and the classical model on computing the velocity for alpha radiation. On the other hand, the students can prove that only the relativistic model is suitable for computing the velocity of electrons at beta radiation, discarding the classical model for its description.
Below are some of the results obtained by different high school pupils (17–18 years old) in their laboratory experiments which show the velocity computed using the classical (red) and the relativistic (blue) model for five different alpha (figure 7) and beta (figure 8) particles.
Figure 7. Comparison between alpha particles' velocity computed with the classical (red) and relativistic (blue) models. The speed of light (green dashed-line) is also shown as a reference.
Download figure:
Standard image High-resolution imageFigure 8. Comparison between beta particles' velocity computed with the classical (red) and relativistic (blue) models. The speed of light (green dashed-line) is also shown as a reference.
Download figure:
Standard image High-resolution imageThose results show clearly to the students the difference in speed between alpha and beta particles. Furthermore, they also emphasize the equivalence of the classical and relativistic model at low speed, and the need for the relativistic model to describe beta particles, otherwise the computed velocity would be higher than the speed of light.
In order to help students to reach these goals, the indications of the work of Abraham and Millar (2008) have recently been considered. Therefore, in each question, action and requirement of the students user guide, an icon indicates if the action required belongs to the domain of observables or to the domain of the ideas. This classification helps students and teachers to understand what is expected in each question and how both types of actions work together in science to create knowledge.
Shown in figure 9, are two examples of the questions which are presented in the workshop guide. In the first one, students are asked to create a histogram showing the classical and relativistic velocity of each measured apha particle. Acording to Abraham's and Millar's work, that belongs to the domain of the observables, so it is represented with the toolbox icon. In the second one, the students need to evaluate the capability of both models to describe the kinetic energy of this kind of particle. This being an action belonging to the domain of ideas, it is represented with the 'brain-idea' icon.
Figure 9. Activities extracted from the laboratory exercise guide, showing the icons used to indicate if the required action belongs to the domain of observables (top) or the domain of the ideas (bottom).
Download figure:
Standard image High-resolution imageOne of the interesting aspects of using MiniPIX detectors is the astonishment felt by the students, when they approach some material emitting radiation to the detector and visualize the distribution in space of the different particles emitted by the source. Often, an audible 'Wow!' can be heard when the tracks apear on the screen.
As one can see, these didactic objectives and the results obtained by students using the Timepix, represent a very large qualitative leap compared to those that can be observed in experiments using other detection systems, such as Geiger meters (Millar et al 1990). This is mainly due to the features of the Timepix detector and its ability to (a) visualize and distinguish different families of particle based on their interaction with the sensor material and (b) measure energy deposited by particles, which allows the quantitative study of concepts and models that would otherwise only be explained on a theoretical scale.
4. Results
This section is intended to present some of the most significant data relating to the ADMIRA Project, in terms of the participating schools and pupils, as well as its evolution. Similarly, some research carried out by the project's baccalaureate students during their RTs, is shared so that the great variety of topics that such a versatile tool permits, may be appreciated.
It should be borne in mind, however, that the initiative has been impacted by the COVID-19 pandemic. One of the problems arising from the situation of lockdowns and restrictions in 2020 and 2021, has been the difficulty of communicating and as a consequence, monitoring the activities that were carried out by the different schools.
The initiative started in 2017–2018 with one participating school, 4 RTs related to MiniPIX and 27 students using the detector in the Introduction Practice.
Currently, there are 13 schools participating in the program in the academic year 2020–2021, with more than 200 students that used the detectors to take measurements in the labs and experimentally study particle physics. Most of them did the Introduction Practice to Timepix available with the kit. Other schools developed their own experiments. Thirteen students of the Joan Brossa High School in Barcelona, have carried out a study of the radiation of the school environment. Four students from the educational programme Learn It With Us, have done a search for commonly used materials and have completed a study of their radioactivity levels. Forty students of the F.X. Lluch and Rafecas High School, have studied background radiation in the school environment. Lastly, 11 students from La Roca del Vallès High School have mesured the dose deposited on the detector from several radioactive sources at different distances.
We can identify five main different topics investigated by baccalaureate students in their RT with the detector. The following is a brief description of the topics studied. Some of these works have been shared through the project's web page (ADMIRA 2021c).
The first field is that of the study of background radiation and dosimetry. Many RTs are related to the study of different radiation sources (minerals, common objects and substances) their differences in intensity, type of radiation, collimation of the beam, attenuation by different materials, and mass percent of a radioactive element on a pure substance. Dosimetry is also a field that benefits from the characteristics of the detector device because, by identifying different types of particle, it allows to calculate the damage of different radiation in biological tissue.
The second field is the study and detection of muons from cosmic rays, their angle dependence (figure 10) or the interactions with the atmosphere or the detector itself. It is important to mention that, in these topics, students have also used and compared data provided by external scientific institutions, like TimPix Project measurements at ISS (IRIS 2020) or SATRAM experiment at ESA's Proba-V satellite (Waage 2021).
Figure 10. A device designed, built and programed by one student of the ADMIRA Project, allowing her to tilt the MiniPIX automatically in order to analyse the dependence between the number of muons detected and the inclination angle of the detector.
Download figure:
Standard image High-resolution imageIn certain cases, students have learnt concepts to explain their observations, far beyond those usually reached at secondary level, like Bethe-Bloch equation.
There are also applications related to health care which might require some specialized facilities (like x-ray tubes). The acces to these devices is granted by ICCUB through Modern Physics Lab at UB. In addition, one student researched the ability of face masks to filter and retain dust particles containing radon.
The fourth topic is related to particle detectors. In some different RTs, students have built cloud chambers and compared their measurements with Timepix measurements. In addition, one student built a radiation detector device (Keller et al 2019) and compared the measurements with Timepix data. The student presented his work in an online presentation with people participating from different schools and research centers in Europe.
The last topic some students in the network have studied is computing, Artificial Intelligence, and Information Technologies. Two students have trained a neural network to identify the radiation type from the trace it deposits in the segmented detector. Another student has designed, built, and programmed an ARDUINO device (figure 10) allowing her to automate the orientation of the detector (ADMIRA 2021d) and relating the number of muons detected with the tilt angle. Another student has investigated the importance of computing resources in the analysis of scientific data.
Although some of these works do not have the quality level of professional research 18 , some have attained a certain level of excellence. For instance, two of the students participating in the programme have received awards recognizing their research work in 2021 by University of Girona and by the Catalan Chemical Society (Societat Catalana de Química 2021, Universitat de Girona 2021).
5. Conclusions and further developments
Experimental work and the introduction of particle physics into pre-university studies can help increase the scientific vocation amongst students.
The ADMIRA initiative was started by members of different and complementary institutions—Sagrada Familia School in Gavà, ICCUB and the Microelectronics section at CERN—in order to bring detectors to the classrooms allowing students to visualize radiation and to help them understand complex topics related to particle physics. The local aspect has been fundamental to create links between secondary school students with PhD students from nearby universities and with researchers.
The educational aspects of the initiative, together with the logistics have been presented in the paper. The Timepix-based detector is a tool that can impact particle physics education because it allows to visualize different types of particles. The detector and readout allows the students not only to research topics related to particle physics but also in other fields like dosimetry, medicine, chemistry or information technologies.
Finally, some of the project's data has been analysed to show its growth, despite the difficult situation over the past two academic years. The loan system has maximized the number of schools that have had access to the detectors. Also, research generated by ADMIRA students has been presented to show some other didactic possibilities of the detectors and to encourage others to implement similar initiatives.
As can be seen from the research lines presented by the students, the diversity of topics in the initiative is very wide and, in many cases, the access to such devices leads the young students to put very relevant questions to themselves that lead them to very high quality works and to reach levels that go well beyond what is expected in pre-university teaching.
Looking ahead, the CERN participants hope to disseminate the lessons of ADMIRA more widely throughout the CERN member states and beyond. Several 10's of MiniPIX devices are being acquired by CERN to be distributed initially through institutes and individuals already involved in the Medipix Collaboration activites. The idea is to duplicate many aspects of ADMIRA and adapt those to the diffierent local environments. In the meantime, a project will be submitted to the CERN Technology Impact Fund which will permit the development of a common resource repository at CERN and fund the manpower and logistics needed to support such activities. Funding from donors will be sought to support the establishment of activites in the various countries. Annual meetings involving participants from different regions may be organised where students and teachers can meet to compare notes and share best practices. The authors are convinced that, with time, the use of the Timepix (or similar) device more broadly in schools will lead to a better general understanding of radioactivity and the scientific method as well as greater interest for STEM subjects in high school students.
Acknowledgments
The authors would like to acknowledge the Medipix Collaborations for their support in this initiative. The authors would also like to thank Benedikt Bergmann and Robert Filgas (Institute of Experimental and Applied Physics from the Czech Technical University in Prague) for the data from the SATRAM experiment at ESA's Proba-V satellite.
Data availability statement
The data that support the findings of this study are available upon reasonable request from the authors.
Footnotes
- 15
In Catalan, admira means admire, and it is the acronym of Activitats amb Detectors Medipix per Investigar la Radiació a l'Aula, which in English means Activities with Medipix Detectors to Investigate the Radiation in the classrooms.
- 16
A characteristic of the Spanish educational system is its decentralization. Although the basic content of each subject, at each level, and the form of the general organization of schools is determined by the central government of the state, the concrete implementation of the curriculum depends on the government of each region. For this reason, this manuscript focuses on the particular case of Catalonia, which has particularities from which ADMIRA takes advantage.
- 17
Inquiry-Based Learning is a constructivist methodology in which learning is driven by a process of inquiry owned by the student, covering a wide spectrum of approaches, including fieldwork or case studies adapted to disciplinary contexts (CEEBL Learning Technologist 2021).
- 18
Some of the most common problems include the small sample size of measured events, wrong or non-existent experimental error treatment and advanced physics misconceptions.