On a scorching summer day in Darwin, Australia, I stood 10 feet abreast of a 17-foot-long, 1,200-pound adult male saltwater crocodile—the world's largest reptile. It stared me down with eerie, catlike eyes, its chest heaving periodically to exhale a loud blast of spent air through its nostrils like a locomotive purging steam. Perhaps, I thought, my colleagues and I were taking our research beyond the realm of sensibility. I had worked with crocs many times before but never with such a massive one. Sweat pouring off me, I shuffled forward, armed with only a handful of electronics and a four-foot-long PVC pole capped by a device that would measure the force of the animal's bite.
I approached the crocodile from the side to within two feet of its head. Agitated, it opened its maw, displaying 64 enormous spiked teeth, and hissed—a Dantean warning not to come any closer. This was my cue. Gripping the pole, I thrust the bite-force meter into the back of the crocodile's mouth. Its jaws instantly slammed down on the device with a resounding thud, like the report of a cannon. The impact nearly wrested the meter from my hands. Then there was only silence.
I gathered my senses and took in what had just happened. The reptile was completely still, I was okay, and the equipment seemed to be intact. To my delight, the bite-force meter was perfectly sandwiched between the crocodile's back teeth. “Good bite,” I called to my University of Florida colleague Kent A. Vliet, who was standing behind me holding the charge amplifier that recorded the result. “What was the force?”
On supporting science journalism
If you're enjoying this article, consider supporting our award-winning journalism by subscribing. By purchasing a subscription you are helping to ensure the future of impactful stories about the discoveries and ideas shaping our world today.
“Three thousand six hundred and ninety pounds!” he replied. The research crew and a group of interested bystanders buzzed about the number while I maintained my grip on the PVC pole, waiting for the crocodile to release the meter. As my adrenaline receded, I realized that we had just recorded the highest bite force ever registered for a living animal.
That sweltering day in Australia marked the high point of a series of studies my colleagues and I have been carrying out over the past 17 years to understand the biomechanics of feeding in crocodiles, gharials, alligators and caimans—a group known as the crocodilians. Crocodilians have reigned as the undisputed apex predators in warm, near-shore freshwater and estuarine environments for more than 85 million years. Scientists have long wondered what factors have made this group so successful. Our findings not only help explain why modern crocodilians dominate these habitats today, they also elucidate how their prehistoric ancestors came to rule the water's edge in the first place.
Built to kill
The rich fossil record of crocodilians shows that the shape of the body behind the head has remained largely unchanged for millions of years. Body size, however, shifted again and again, with dwarfism (lengths of less than four feet) and gigantism (lengths of more than 30 feet) evolving repeatedly. Modifications to snout and tooth shape accompanied these changes in body size. Clearly, the key to understanding a large part of the group's success lies in gleaning the form, function, performance and dietary relevance of these attributes.
As luck would have it, the 24 living crocodilian species, like their ancient predecessors, have a wide range of body sizes, from four to 23 feet. Modern crocs also show the same array of snout forms seen in fossil crocs. Scientists have already determined the diets associated with the various snout shapes of present-day crocs. Specifically, they have identified medium- and broad-snouted generalists; slender-snouted, needle-toothed forms specialized for consuming small prey, including fish; broad-snouted, bulbous-toothed forms that focus on hard prey such as mollusks; and dog-snouted, semiterrestrial feeders. Study of the feeding biomechanics of these animals in conjunction with what they eat in the wild could tell us how these remarkable predators make a living today and also open the door to understanding their past.
Back when we began our research, however, the state of the science was such that the biomechanical significance of the crocodilian snout and dentition types and body size variability was based purely on speculation and modeling, with virtually no empirical foundation. There were several reasons for this dearth of empirical data. Many of the world's crocodilians are highly endangered from overhunting, making them difficult for scientists to access. They are also dangerous to work with. Furthermore, the technology to determine their bite forces and tooth pressures—essential measurements for figuring out biomechanics—did not exist. This was all about to change.
In 2001 a film producer at National Geographic called me to ask whether I would be willing to determine the bite force for the extinct, 40-foot-long “supercroc” (Sarcosuchus), a croc relative discovered in Niger by paleontologist Paul Sereno of the University of Chicago. I replied that I could do it through bite-force experimentation on living crocodilians but that I would need to see if I could get access to specimens. I immediately contacted Vliet, who is the scientific consultant to the St. Augustine Alligator Farm Zoological Park (SAAF) in Florida—the only facility in the world that housed the 23 species of crocodilian then known—and proposed a series of studies. First, I wanted to determine the adult bite forces and tooth pressures of every extant crocodilian species using the SAAF specimens. Then I wanted to contrast captive alligator data with those from the wild using specimens acquired through local nuisance trappers and Florida Fish and Wildlife Conservation Commission biologists to see if captive values could be used to infer performance in the wild. I also intended to conduct muscle dissections and muscle-stimulation experiments on alligators to construct a model for predicting bite forces in fossil crocodilians and to use those data to understand the feeding performance of extinct crocodilians and their relatives, particularly dinosaurs. SAAF owner David Drysdale graciously gave us permission to do the testing, National Geographic provided funding for the research, and the race was on to figure out how best to collect our data before filming began.
How does one measure the bite force of a crocodile? Good question. I did not know. No one had ever directly measured the bite force for any very large animal, let alone enormous, predatory reptiles. My only experience doing comparable work was testing the bite forces of tiny lizards as a graduate student using tweezerlike metal plates fitted with gauges to measure the force required to squeeze them together. To tackle this much bigger problem, I enlisted the help of an engineer I had worked with previously at Stanford University and a design engineer at Kistler, a company that makes force transducers—devices that record forces when compressed—that are used in the testing of industrial materials. Together we designed portable, water-resistant bite-force meters to use on the crocs. They look like mini bathroom scales but are much more accurate and cost up to $11,000. (I often tell my students that if they really want to know their weight, they can come by my office.) We attached a PVC pipe handle to each device and wired it to a portable charge amplifier with a readout that instantly records the output. We also padded the transducer's steel bite plates with thick layers of bull hide to mimic the feel of an actual prey animal and to protect the crocs' teeth. This turned out to be a more important measure than we had anticipated because one of my colleagues later showed that, like a person eating a jawbreaker, reptiles will not bite with full force if the material their teeth come into contact with is exceptionally hard.
After we had our technological solution in hand, the next step was to develop a protocol for testing the crocodilians. I worked with fellow crocodilian biologist Vliet and SAAF director John Brueggen to formulate a plan that would meet our research objectives while minimizing stress to our study subjects, in accordance with animal welfare guidelines. What we came up with, which miraculously worked from the get-go, entailed lassoing the crocodilians, which are unaccustomed to being handled, in their pens; dragging them out using a small army of people; securing them in place; releasing the jaws, which invariably prompts them to open; and finally, placing the meter on the rear molarlike teeth, where the bite forces are the greatest, to elicit and record a maximal-force bite. During the test, a handler on the animal's back would keep the head straight to prevent rolling. If the animal were to roll during the experiment, the results would not be a pure reflection of jaw muscle contributions to bite force, because the reptile's mass and inertia would figure into the reading. After the test, we would measure and weigh the animals. Weight is rarely recorded for large, nondomesticated animals. Nevertheless, we felt that it was important for our work because it would allow us to compare pound-for-pound bite-force performance among croc species spanning a broad range of sizes and to compare the croc data with those from other animals, regardless of body shape.
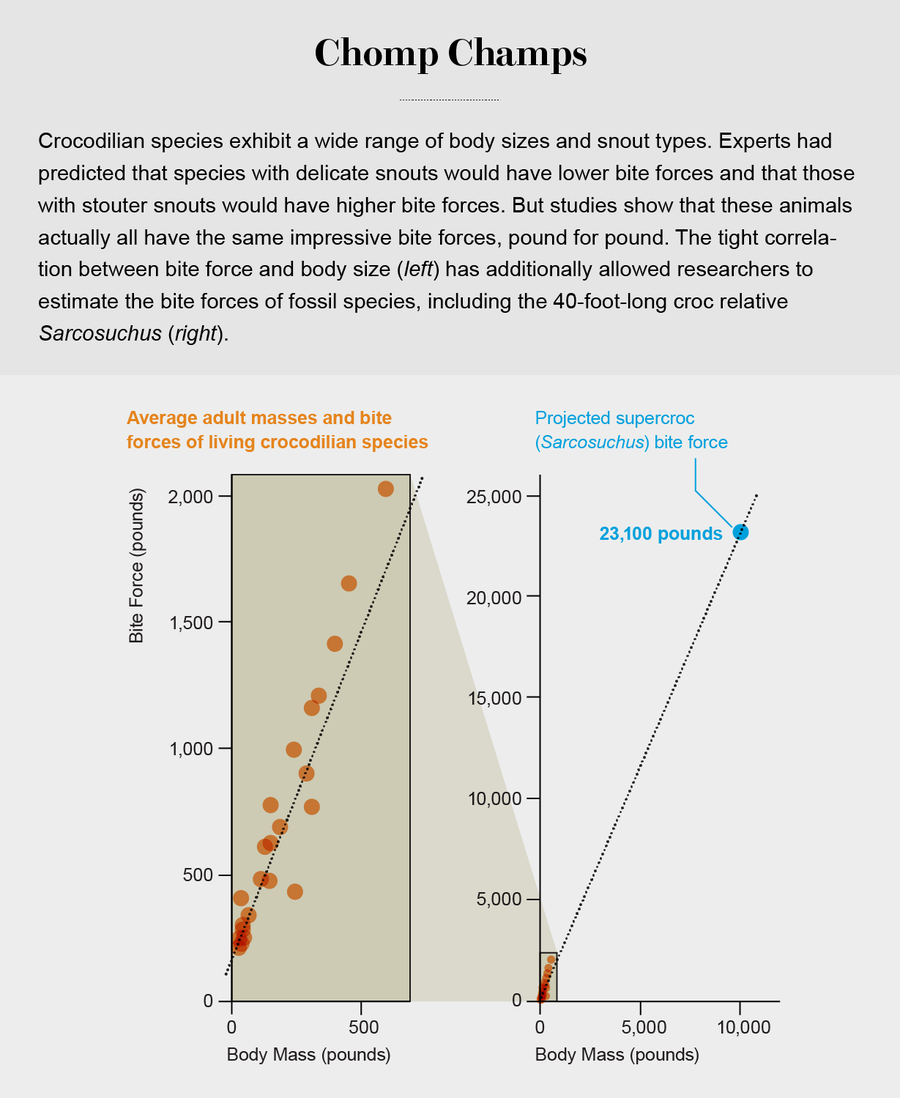
Credit: Jen Christiansen; Source: “Insights into the Ecology and Evolutionary Success of Crocodilians Revealed Through Bite-Force and Tooth-Pressure Experimentation,” by Gregory M. Erickson et al., in PLOS ONE, Vol. 7, No. 3, Article No. E31781; March 14, 2012
The capture and weighing process is like bull riding for scientists—although I once had a discussion with a professional bull rider who said he wanted no part of such an undertaking, noting that “bulls at least don't come back around and eat you.” Similarly, I once caught and tested a 13-foot alligator with Georges St-Pierre, arguably the greatest all-time mixed martial artist, who said it was the scariest thing he had ever done and that my colleagues and I were insane. To be honest, those of us who work closely with these animals do not consider it particularly perilous. Still, the largest crocs do give us pause, and even the smaller ones can take your arm off if you do not pay attention. Like veterinarians interacting with dogs, each of us has learned how to read the crocs' behavior and handle them without causing harm to the animals or ourselves. The cardinal rules are to stay away from the pointy ends and avoid the water's edge. Crocodilians are incredibly stealthy—even 17 footers can be imperceptible in the shallows—and they can explode out of the water to ruin one's day.
We had one last piece of research protocol to figure out. Bite force is commonly seen as a reliable metric to describe a toothed animal's feeding performance. But it is only an indirect proxy. An analogy is horsepower: a 700-horsepower engine produces speed in a Ferrari but not in a dump truck. For our purposes—gauging a crocodilian's ability to successfully hunt the prey animals it encounters at the land-water interface—the most biologically relevant parameter is tooth pressure. Tooth pressure determines whether the teeth can actually penetrate food items composed of skin, cuticle, shell, bone, and so on. Pressures produced by the tooth crowns promote shear stress failure to these tissues, thereby introducing cracks or punctures that open with greater application of bite force, enabling either an immediate kill or a grip with which to drown the quarry.
To estimate tooth pressure after each bite-force test, we would place a board in the animal's jaws and tape the mouth shut. I would then reach in and make molds of the teeth with dental putty, a real-life version of the popular children's game Crocodile Dentist. The board blocks any chomps the croc might make reflexively in response to being touched on the teeth or anywhere in the mouth. But even now, after taking hundreds of molds, I still flinch at the task—putting one's hands in the mouth of a crocodile goes against all basic human instincts. Ultimately we would use the molds to make casts of the teeth in the lab and to measure the contact areas at the tooth tips. We then divided these values into the bite forces to deduce the tooth pressures.
What we learned over the course of our studies of more than 500 individuals representing all the living crocodilian species upends some of the conventional wisdom about these animals. Prior to our research, experts predicted that there would be major differences in relative bite forces among crocodilians. Those with delicate snouts and needlelike teeth that feed on softer prey such as fish would show low bite forces, whereas others that possess robust skulls and stout teeth and are capable of crushing bones and mollusks would show high values. Instead we found that all crocodilians can generate prodigious bite forces. In fact, our statistical analyses revealed that, with the exception of one species (the fish-eating gharial), they all show the same bite forces, pound for pound, regardless of whether they have hard or soft diets or are weak or strong of snout. The higher values in the range—up to 3,700 pounds—eclipse those measured or estimated for carnivorous mammals such as spotted hyenas, lions and tigers (around 1,000 pounds). We humans, for our part, can generate forces of only 200 pounds. I am often asked if one can escape from the jaws of a crocodile or alligator. Large crocodilian bite forces are equivalent to the weight of a car pressing down on the jaws. So if you can bench press a car, then you are good to go.
Notably every crocodilian biologist I spoke to before we conducted this work said that wild alligators—“red in tooth and claw” and athletic from having to struggle for existence in their natural habitats—would show superior bite-force performance, compared with their often obese and lethargic captive counterparts. Instead we found that, pound for pound, they chomp with the same might. This finding was important because it showed that our data on captive animals could be used to speculate on the performance of wild individuals and that we could use those data to explore performance in fossil crocs.
From these findings, we predicted the bite force for Sarcosuchus and some extinct giant crocodilians. The values are around 23,100 pounds, comparable to the weight of a semi. At the other end of the spectrum, we also estimated the bite force for the smallest known crocodilian, the two-and-a-half-foot-long Procaimanoidea, which lived around 40 million years ago. It would have clamped down with just 141 pounds of force. My former graduate student Paul M. Gignac, now an assistant professor at Oklahoma State University Center for Health Sciences, has recently been using these data to gauge bite forces throughout crocodilian evolution.
Reptilian weed trimmers
Our findings have a number of evolutionary implications. Most important, they show that throughout their 85-million-year reign as guardians of the water-land interface, crocodiles have retained the architecture of the muscles that close the jaw. The results also paint a new picture of how crocodilians repeatedly evolved the same five snout and tooth shapes and associated ecological habits. In a way, they are like weed trimmers. If one wants more force during yard work, switch to a model with a bigger engine. Crocodilians regularly achieved the same effect via the evolution of greater body sizes. To switch from cutting grass and brush to specialized applications such as edging sidewalks or sawing tree limbs, change the attachment. Crocodilians became specialized for exploiting different prey through the evolution of different jaw and tooth “attachments.”
The tooth pressure data tell a complimentary story. Like the bite forces, the tooth pressures of crocodilians are unmatched by any other living animal and show increases with animal size. The values range from 20,160 to 358,678 pounds per square inch (psi), dwarfing the previous record estimate of 21,321 psi for the giant fossil fish Dunkleosteus. Our results suggest that a secret to the catholic diets that have helped make crocodilians so successful (no croc is strictly a specialist feeder) is that any species can puncture the common food types in its realm. Different tooth forms simply allow for higher or lower pressures in more specialized feeders, which promotes greater efficiency at puncturing softer prey such as fish or sustaining impacts from hard bones or shells.
Knowing this, my students and I began to take a closer look at the factors that contribute to bite force. In 2010 Gignac examined the muscles of American alligators to develop a means of accurately predicting each muscle's contribution to the bite forces. All crocodilians have what appear at first glance to be bulging neck muscles. These are actually the so-called medial pterygoids, which are among the muscles involved in closing, or adducting, the jaw. In most animals, the medial pterygoids are small and do not add much to bite force. In crocodilians, they generate 60 percent of it.
Animals with powerful bites typically have enlarged temporalis muscles, which sit above the jaws. (These are the muscles that bulge on your temples when you clench you jaw.) Crocs, however, have beefed up their medial pterygoids, which sit under the jaws and protrude down the sides of the neck. Why? The answer has to do with how they hunt. Crocs are remarkably good at sneaking up on prey and seizing it in very shallow water at the shore. They generally approach their quarry with very little of their head protruding from the water aside from their nostrils for breathing, eyes for seeing and ears for hearing. The rest of the giant body remains submerged until the predator lunges at the unsuspecting prey. Crocs are stealthier with their jaw adductors hidden below the water line than they would be if they had instead built up the much more prominently located temporalis. Like submarines at periscope depth, crocs have only the requisite sensing equipment above the water—everything else is down below.
Gignac's analyses of the muscles involved in croc bite force shed light on another puzzle as well. In our initial tests, the slender-snouted, needle-toothed, fish-eating gharial showed aberrant bite-force generation. Its forces fall some 50 percent below the croc norm. When Gignac dissected one of the animals, he found that its medial pterygoids are relatively small, but its upper jaw-closing muscles are large for a crocodilian. That arrangement favors speed of jaw closure over bite force. We suspect that the gharial, being the most piscivorous croc species, sacrificed bite force for more rapid seizure of fish.
This line of research resolves another croc conundrum. During our testing of the giant Australian saltwater crocodile, the animal held onto the bite meter for 10 minutes before releasing it. We discovered that movement of the meter in this situation elicits clenching bites of nearly the same magnitude as the initial full-force bite. I have personally recorded up to 22 of these clenching events and sometimes have waited 25 minutes to recover the equipment—you get the meter back when the crocodile wants to give it back, never earlier. That fact left us wondering about the significance of this behavior and how it was generated.

Open-and-Shut Case: Author Erickson measures the bite force of an alligator. Credit: Gregory M. Erickson
To find out, we hooked a computer up to our bite meter and recorded the forces throughout bite-force experimentation on wild American alligators. This sequence showed that the holding forces were roughly 10 percent of the maximal bite forces. We posit that this hardwired behavior is related to how gators drown large prey. In the wild, alligators typically use an aggressive bite to puncture game and gain a purchase with their teeth. They then take the food item to deeper water and drown it. If the prey struggles, the alligators reengage the teeth with full-force biting. Gignac's dissections revealed that these holding and clinching abilities come from a remarkable physiological specialization of the muscles. He noticed that the massive jaw-closing muscles that generate most of the maximal bite forces are white in color, analogous to the turkey breast muscles that can generate short bursts of force for flight but tire easily from a lack of a blood supply. He then discovered red and pink oxidative muscles for sustaining the low force-holding behavior. They are akin to the dark meat found in turkey legs, which are highly vascularized and rich in myoglobin for sustained walking. Gignac's model shows that together, these previously unrecognized dark muscles generate 10 percent of the alligator's bite force, just enough to keep the teeth engaged.
T. REX bites again
Our work has applications for understanding feeding in animals beyond crocodilians. Gignac and I have since used the data to develop the first viable bite-force model for Tyrannosaurus rex. Previous estimates of T. rex's bite force used models based variously on alligators, lizards and even mammals. Needless to say, the results were disparate, ranging from 18,000 to 245,000 pounds. In contrast, our model, which is specific to archosaurs—the group that comprises crocodilians, birds and their extinct relatives—yielded a value of around 8,000 pounds. That is twice what the largest living crocodilians are capable of. Moreover, T. rex's tooth pressure—431,342 psi—is the highest pressure estimated for any animal. Based on these new estimates, which we published in 2017, we solved the mystery surrounding how, as fossil evidence indicates, the king of dinosaurs could pulverize the bones of its prey. Today only carnivorous mammals with upper and lower teeth that come into full contact, or occlusion, during chewing can manage this feat.
Crocs are magnificent predators, and we have made great progress in figuring how they got that way, but many questions remain unanswered. It is likely, for example, that snout shapes could affect bite force underwater in ways that they do not on land. That means we need to repeat our tests underwater. It is easier said than done: doing so requires that we develop new bite-force meters that work when fully submerged and partner with engineers to understand how water flow might affect jaw-closing velocity and bite force. Moreover, we need to develop new safety protocols: crocs have the upper hand in the aquatic realm. We'll get there. We may be more gray-haired and banged up than we were when we started this research, but we are up to the task.