Abstract
In this review, we discuss the available experimental evidences supporting the chemopreventive efficacy of nonsteroidal anti-inflammatory drugs (NSAIDs) on colorectal cancer and the biological basis for their possible role as anticancer agents. Although the comprehension of the mechanisms underlying the effects of these drugs on colon cancer cells is incomplete, research efforts in identifying the biochemical pathway by which NSAIDs exert their chemopreventive effect have provided a rationale for the potential use of NSAIDs alone or in combination with conventional and experimental anticancer agents in the treatment of colorectal cancer. In this paper, we review three main issues: (i) the role of COX-2 in colon cancer; (ii) the common death pathways between NSAIDs and anticancer drugs; and (iii) the biological basis for the combination therapy with COX-2 selective inhibitors and new selective inhibitors of growth factor signal transduction pathways.
Similar content being viewed by others
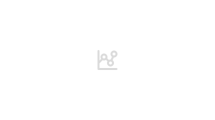
Role of COX-2 in colon cancer
Colorectal cancer is the third most common cancer in the world, and the second most common cause of cancer-related death. Despite the development of new strategies of aggressive surgical and adjuvant therapy, little progress has been made in the successful management of advanced disease; therefore, much hope is currently placed on chemoprevention.
A large body of evidence from epidemiological studies and clinical trials in patients with the hereditary colon cancer syndrome, familial adenomatous polyposis coli (FAP) indicates that aspirin and related drugs, known as nonsteroidal anti-inflammatory drugs (NSAIDs), which share the property of inhibiting the cyclooxygenase (COX) enzyme, hinder the development of colon cancer and perhaps other cancers as well.
COX is the rate-limiting enzyme for the synthesis of eicosanoids, such as prostaglandins, from arachidonic acid. Two COX isoforms have been identified: the constitutively expressed COX-1 and the inducible COX-2. COX-1 is a housekeeping gene and has an important role in protecting the gastroduodenal mucosa. The COX-2 gene, an immediate-early response gene, is rapidly induced in response to tumour promoters, cytokines, and growth factors (DuBois et al, 1994; Di Popolo et al, 2000). NSAIDs may achieve different degrees of inhibition of COX-1 and COX-2 and can be grouped into selective and nonselective inhibitors of COX-2.
The development of COX-2-specific inhibitors, like celecoxib and rofecoxib, drugs that maintain their anti-inflammatory properties while preserving the biosynthesis of protective prostaglandins, further raised interest in this field. A large body of research was therefore performed to clarify the relative involvement of the two COX isoforms in colorectal carcinogenesis and the role of COX-2-selective inhibitors as chemopreventive agents.
COX-2, but not COX-1, expression was found to be increased in colorectal cancer (Eberhart et al, 1994): approximately 50% of adenomas and 80–85% of adenocarcinomas showed increased expression of COX-2. This observation was later confirmed by other investigators. More recently, studies underlying the role of COX-2 in colorectal carcinogenesis were performed in animal models: a series of reports documented that COX-2 mRNA was elevated both in chemically induced colon tumours and intestinal tumours in experimental animal models, and that traditional NSAIDs and new COX-2-selective inhibitors reduced the number of polyps and tumour incidence (Gupta and Dubois, 2001). Although these data indicate that COX-2 plays a wide role in colorectal carcinogenesis and that its pharmacological inhibition by NSAIDs is the central event in the chemoprevention of colon cancer, the mechanisms involved are yet to be clarified. It is also unclear how COX-2 elevation exerts its oncogenic effect at the molecular level, but an expanding body of evidence indicates a pivotal role of COX-2 elevation in the prevention of programmed cell death, that is, apoptosis, a process by which damaged or mutated cells in colonic mucosa are removed (Cao and Prescott, 2002).
Common death pathways between NSAIDS and anticancer drugs
Today, the management of advanced colorectal cancer involves mainly the use of chemotherapeutic drugs. For this purpose, 5-FU or new compounds direct thymidylate synthase (TS) inhibitors like raltitrexed and nolatrexed, or irinotecan (CPT-11) and oxaliplatin, have established activity both as single agents and in combination. Most of these current available cytotoxic drugs have well-recognised intracellular macromolecular targets (i.e. TS for 5-FU, toposisomerase 1 for CPT-11). However, the pattern and the extent of cell damage induced by chemotherapy in human cancer cells have been suggested to depend also on the pathway downstream from drug–target interaction that, once triggered, will initiate apoptosis (Canman et al, 1992). Apoptosis is a complicated process and its proper execution programme requires the coordinated activation and execution of several pathways. There is emerging evidence both that the major target for anticancer therapy is the induction of apoptotic cell death, and that resistance to antitumour treatments relies on reduced sensitivity to apoptosis induction. Hence, novel treatment approaches have tried to overcome this kind of drug resistance by manipulating key regulator proteins in apoptosis machinery (Nicholson, 2000). Figure 1 summarises the major apoptotic pathway in mammalian cells and the current recognised sites of interference of COX inhibitors.
Molecular mechanisms that mediate the effects of NSAIDs and anticancer drugs on survival and apoptosis in colon cancer cells. Schematic representation of cytokine, EGF-related growth factors and TRAIL ligand-dependent signal transduction pathways for survival and apoptosis. Stimulatory and inhibitory effects are indicated by arrows and bars, respectively. Abbreviations: MAPK=mitogen-activated protein kinase, MAPKK=mitogen-activated protein kinase kinase, JNK=jun kinase, IkB=inhibitor kinase B, NF-kB=nuclear factor kappa B, COX=cyclooxygenase, PI3K=phosphatidylinositol 3 kinase.
In order to understand the molecular mechanisms underlying the chemopreventive effect of NSAIDs, a number of studies have assessed the effect of NSAIDs on cell growth and apoptosis in cultured colon cancer cells. We and others have shown that nonselective and COX-2-selective inhibitors are able to inhibit cell growth and induce apoptosis in colon cancer cells (Elder et al, 1997; Ricchi et al, 1997; Di Popolo et al, 2000).
This effect in vitro has been initially attributed to COX inhibition and to the consequent reduction in prostaglandin levels. On the other hand, an expanding literature shows a reduced role of the prostaglandin pathway in the mechanism of action of NSAIDs and new coxib (Song et al, 2002). In fact, several NSAIDs maintain their properties also in COX-2-negative tumour cell lines and independently from COX-2 expression levels (Elder et al, 1997) and, between coxib, Vioxx, although exhibiting similar COX-2 selectivity and clinical efficacy for inflammatory indication with respect to celecoxib, has little or no anticancer activity (Johnson et al, 2001; Waskewich et al, 2002). Although the concentration of NSAIDs required to show these effects in vitro is sometimes higher than the amount required to inhibit prostaglandin production and higher than that achievable in vivo, numerous studies have clearly focused on the induction of apoptosis as the mechanism for growth inhibition of colon cancer cells. Interestingly, most of the death pathways affected by NSAIDs are those involved in anticancer drug-induced apoptosis and resistance (Figure 1).
In fact, high doses of aspirin were shown to antagonise the survival signalling pathway controlled by the transcriptional factor NF-kB (Kopp and Ghosh, 1994) through the inhibition and the destruction of the activity of IKB kinase β (Yin et al, 1998), an enzyme that activates the NF-kB pathway by phosphorylating the inhibitory subunit of NF-kB (IkB). A similar effect on inhibition of IKB kinase β activity was reported for sulindac (Yamamoto et al, 1999). On the other hand, one study reported that inhibition of NF-kB through the adenoviral delivery of a modified form of IkBa sensitised chemoresistant tumours to the apoptotic effect of the antiblastic compound irinotecan (Wang et al, 1999).
Recently, it has been shown that celecoxib-induced apoptosis was mediated by the inhibition of the activation (phosphorylation) of the antiapoptotic protein kinase AKT (Hsu et al, 2000). A related study demonstrated that transfection of the constitutively active akt cDNA in a human lung cancer cell (A549) resulted in the reduction of the cytotoxic effect of topotecan, and concluded that topotecan exhibited its toxic effects by downregulating the Akt survival signalling pathway (Nakashio et al, 2000).
In eucaryotic cells, survival and apoptosis are often regulated by relative level of members of the Bcl-2 family proteins. One study reported that members of the Bcl-2 family of proteins in human colon cancer cell lines were modulated by 5-FU and that the ratio of the antiapoptotic protein bcl-xl to the proapoptotic protein bax may be related to chemosensitivity (Nita et al, 1998). On the other hand, the COX-2-selective inhibitors SC-58125 and NS-398 were reported to sensitise colon and prostate cancer cells, respectively, to apoptosis by downregulating the antiapoptotic protein bcl-2 (Liu et al, 1998). In addition, in a study performed in HCT 116 colorectal cancer cells, the proapoptotic protein bax had a pivotal role both in 5-FU and sulindac-induced apoptosis, and several NSAIDs could decrease the level of the antiapoptotic gene BCL-xl (Zhang et al, 2000). Similarly, aspirin and indomethacin were shown to induce apoptosis through upregulation of the proapoptotic proteins bax and bak and activation of caspase 3 (Zhou et al, 2001).
Several evidences suggest that both TRAIL (tumour necrosis factor-related apoptosis-inducing ligand) and FAS signalling pathways are involved in chemotherapy-induced apoptosis, either activating the initiator caspase 8 or at the level of downstream effector caspase (caspases 3, 6, 7) activation (Petak and Houghton, 2001). In the NSAID field, it was reported that indomethacin and sulindac sulphide induced apoptosis of human leukemic Jurkat cells by a mechanism that required the Fas-associated death domain (Han et al, 2001). Similarly, a recent paper demonstrated that sulindac sulphide and the COX-2-selective inhibitor SC-′236 could induce apoptosis coupled with upregulation of DR5, caspase 8 activation, and Bid cleavage in HCT116 human colon cancer cells (He et al, 2002). Thus, the common interference of anticancer agents and NSAIDs with the apoptosis machinery and signalling, independently from their COX inhibitory effect supports their use either as a single agent or as modulators of the cell death signalling pathway to potentiate or restore cytotoxic anticancer drug action.
The evidence of NSAID proapoptotic and growth inhibitory effects prompted the evaluation of the in vitro effects of a combination treatment with conventional anticancer drugs. Synergistic effects were reported as early as in the 1980s. The combination of indomethacin with methotrexate increased the killing of cultured NC cells without providing an exhaustive mechanism for this effect (Bennett et al, 1987). More recently, a report performed the first extensive screen of commercially available NSAIDs with anticancer drugs and discussed the potential clinical benefits of such a combination (Duffy et al, 1998); the authors observed dose-dependent synergistic effects among the two classes of drugs and concluded that this effect occurred independently from COX inhibition. Further combination therapies were evaluated: either sulindac sulphide, a COX-1 and COX-2 inhibitor, or other compounds devoid of COX-inhibitor property, in combination with paclitaxel and cisplatin, produced synergistic activity against three NSCLC and SCLS cell lines (Soriano et al, 1999). More recently, it was found that nimesulide, a COX-2-selective inhibitor, at clinically achievable concentration reduced the IC50 values of various anticancer agents in a lung cancer line (Hida et al, 2000).
Further studies are needed to better evaluate the combination therapy of cytotoxic drugs and new COX-2 inhibitors other than celecoxib and rofecoxib in colorectal cancer cells to induce the optimal apoptotic effect and to avoid reduction of anticancer drugs activity in the case of concurrent administration. In fact, several conventional NSAIDs and currently used COX-2 inhibitors are mainly antiproliferative and may upregulate the cyclin-dependent kinase inhibitors p27Kip1 and p21WAF1 (Hung et al, 2000; King and Khalili, 2001), thus theoretically limiting the effectiveness of cell cycle-dependent chemotherapeutic agents. In partial support of this hypothesis, we have described that high doses of aspirin and NS-398 were able to reduce CPT-11- and Vp-16-induced toxicity in cultured colon cancer cells independently of their COXs isoform profile expression (Ricchi et al, 2002).
A potential role for NSAIDs in an adjuvant setting can also be hypothesised independently from their effects on cell growth and survival. Several promising classes of noncytotoxic agent are under intense investigation (Sobrero et al, 2000). Most of them target the well-recognised series of steps involved in the process of metastasis. Neoangiogenesis is a crucial event of tumorigenesis and metastatic development, because neovascularisation is required for tumours to grow beyond 2–3 mm in size. There is mounting evidence that prostaglandins participate in angiogenesis, regulating the production of proangiogenic factors such as vascular endothelial growth factor (VEGF). Both COX-1 and COX-2 are involved in tumour vascularisation, and COX-2 inhibitors can directly affect angiogenesis (Gately, 2000). A recent observation suggested that angiogenesis was directly involved in the biology of tumour dormancy and was associated with the risk of recurrence after adjuvant therapy in breast cancer (Gasparini et al, 2001). It was also found that angiogenic inhibitor TNP inhibited tumour growth when administered following cyclophosphamide (Shusterman et al, 2001); the authors also documented that TNP directly inhibited angiogenesis and increased apoptotic index in treated tumours, particularly in the setting of minimal or subclinical disease. Finally, a recent paper demonstrated that NSAIDs were able to suppress PGE2- and PGI-mediated endothelial-cell spreading and migration in vitro and fibroblast growth factor-2-induced angiogenesis in vivo (Dormond et al, 2001).
Despite all the discussed evidences of NSAID interference at different levels with tumour cell proliferation, survival, and metastatic formation, only few reports are available in the literature concerning the treatment of human clinical cancer by NSAIDs. In 1994, Lundholm et al, in a randomised trial, treated 135 undernourished patients with metastatic solid tumours to receive placebo, prednisolone, or indomethacin, until death. Indomethacin-treated patients suffered less pain and had a significant increase in survival (Lundholm et al, 1994). Survival analysis from all patients treated with either indomethacin or prednisolone demonstrated a significantly prolonged survival by anti-inflammatory treatment compared with placebo treatment. They did not observe any serious relevant side effect for indomethacin treatment and propounded for indomethacin with respect to prednisolone, based on the theoretically and experimentally less catabolic and immunoattenuating effect of indomethacin on the host compared with glucocorticoids.
In 1996, Sinicrope et al conducted a phase I trial in 15 patients who had failed prior 5-FU-based therapy (Sinicrope et al, 1996). They administered sulindac (300 mg day−1) associated with the same schedules of systemic 5-FU and per os levamisole used in the intergroup adjuvant trial (450 mg m−2 5-FU+150 mg daily levamisole). Leukopenia was no more frequent than in patients receiving 5-FU and levamisole in the intergroup adjuvant trial. All toxic effects were reversible, and there were no chemotherapy-related deaths. A partial response was seen in one patient, three patients had disease stabilisation, and 10 patients progressed while in the study. Uptill today, celecoxib has been successfully used in randomised clinical trials only as a chemopreventive agent to treat colorectal and duodenal polyps in FAP patients (Steinbach et al, 2000; Phillips et al, 2002); the two doses (100 and 400 mg twice daily) of celecoxib employed were well tolerated compared with placebo, but in the groups of patients receiving the most effective dose (400 mg twice daily), one allergic reaction and a greater incidence of adverse effects (dyspepsia and abdominal pain) were observed.
New trials are currently evaluating coxibs as single agents to treat only other precancerous lesions and cancer that express COX-2 in adjuvant settings (Thun et al, 2002). However, all data discussed above may support the use of NSAIDs and new coxibs for the treatment of cancer in combination with standard therapy also independently from cancer cell COX-2 levels.
Phase I studies are needed to evaluate in vivo the optimal dose and timing of conventional and new COX-2-selective NSAIDs in combination with the currently used chemotherapy regimen for subsequent use in phase II trials.
Further attractive combination therapy
The recent development of inhibitors of biochemical pathways that are altered in cancer cells has given rise to the possibility of evaluating new combination treatment regimens. In colon cancer cells, different growth factors such as EGF, IGFs have been identified as positive regulators of cell growth. In particular, the erb/HER pathway seems to play an important role in the maintenance of neoplastic disease. As discussed above, the COX-2 pathway is implicated in colorectal cancer biology and it appears under the control of growth factor signal transduction in several experimental systems (DuBois et al, 1994; Di Popolo et al, 2000). Thus, it is a conceptually reasonable combination therapy that targets simultaneously COX-2 and growth factor receptor pathways (Figure 1). Recently, both a specific monoclonal antibody against Her-2/neu activity (Herceptin) and a selective EGFR-tyrosine kinase inhibitor (Iressa) came into clinical practice in cancer patients. The above molecules have been shown to synergise in vitro and in animal models the effects of conventional anticancer agents (Ciardiello et al, 2000). First, treatments based on combination therapy with NSAIDs and compounds that target other oncogenic pathways have been successfully tested in chemopreventive settings: Torrance et al (2000) showed that combination treatment of APCmin mice with sulindac and the ErbB tyrosine kinase inhibitor EKI-569 resulted in synergistic antitumour activity that leads to complete polyp prevention in half of all treated mice. Then, DuBois and collaborators, in the field of cancer therapy, clearly showed that the combination of celecoxib and herceptin had additive effects against the HCA rectal adenocarcinoma cell line in vitro and in xenograft, which leads to the almost complete inhibition of tumour growth (Mann et al, 2001).
Thus, coadministration of a selective COX-2 inhibitor with a tyrosine kinase inhibitor could enhance their single-agent anticancer activity and should be evaluated as a therapy in vitro and in colon cancer patients. At the moment, there are no trials designed to evaluate any of the above-mentioned combinations in colon cancer patients.
Conclusions
Since the cure rate for colorectal cancer is low, it is mandatory to develop therapeutic strategies with less toxicity also for adjuvant therapy. Several lines of evidence suggest that COX-2 inhibitors not only counteract the development of malignant tumour at an early stage and cause premalignant tumours to regress, but also stimulate the death of established cancer cells. Therefore, COX-2 is becoming an attractive target for therapeutic strategies in colorectal cancer. The ability of NSAIDs and COX-2 inhibitors to synergise with conventional anticancer drugs and to induce apoptosis also independently from COX-2 expression in vitro further encourages their use in clinical practice. In addition, COX inhibitors are effective in reducing angiogenesis, supporting their use also in adjuvant settings. Recent evidences indicate that COX-2-selective inhibitors may also synergise with new inhibitors of the growth factor signal transduction pathways. Taken together, all this information provides a rationale for NSAIDs used alone or in combination with chemotherapeutic agents within clinical trials in colorectal cancer patients.
Change history
16 November 2011
This paper was modified 12 months after initial publication to switch to Creative Commons licence terms, as noted at publication
References
Bennett A, Gaffen JD, Melhuish PB, Stamford IF (1987) Studies on the mechanism by which indomethacin increases the anticancer effect of methotrexate. Br J Pharmacol 91: 229–235
Canman CE, Tang HY, Normolle DP, Lawrence TS, Maybaum J (1992) Variations in patterns of DNA damage induced in human colorectal tumor cells by 5-fluorodeoxyuridine: implications for mechanisms of resistance and cytotoxicity. Proc Natl Acad Sci 89: 10474–10478
Cao Y, Prescott SM (2002) Many actions of cyclooxygenase-2 in cellular dynamics and in cancer. J Cell Physiol 190: 279–286
Ciardiello F, Caputo R, Bianco R, Damiano V, Fontanini G, Cuccato S, De Placido S, Bianco AR, Tortora G (2000) Antitumor effect and potentiation of cytotoxic drugs activity in human cancer cells by ZD-1839 (Iressa), an epidermal growth factor receptor-selective tyrosine kinase inhibitor. Clin Cancer Res 6: 2053–2063
Di Popolo A, Memoli A, Apicella A, Tuccillo C, di Palma A, Ricchi P, Acquaviva AM, Zarrilli R (2000) IGF-II/IGF receptor pathway up-regulates COX-2 mRNA expression and PGE2 synthesis in Caco-2 human colon carcinoma cells. Oncogene 19: 5517–5524
Dormond O, Foletti A, Paroz C, Ruegg C (2001) NSAIDs inhibit alpha V beta 3 integrin-mediated and Cdc42/Rac-dependent endothelial-cell spreading, migration and angiogenesis. Nat Med 7: 1041–1047
DuBois RN, Awad J, Morrow J, Roberts II LJ, Bishop PR (1994) Regulation of eicosanoid production and mitogenesis in rat intestinal epithelial cells by transforming growth factor-alpha and phorbol ester. J Clin Invest 93: 493–498
Duffy CP, Elliott CJ, O'Connor RA, Heenan MM, Coyle S, Cleary IM, Kavanagh K, Verhaegen S, O'Loughlin CM, NicAmhlaoibh R, Clynes M (1998) Enhancement of chemotherapeutic drug toxicity to human tumour cells in vitro by a subset of non-steroidal anti-inflammatory drugs (NSAIDs). Eur J Cancer 34: 1250–1259
Eberhart CE, Coffey RJ, Radhika A, Giardiello FM, Ferrenbach S, DuBois RN (1994) Up-regulation of cyclooxygenase 2 gene expression in human colorectal adenomas and adenocarcinomas. Gastroenterology 107: 1183–1188
Elder DJ, Halton DE, Hague A, Paraskeva C (1997) Induction of apoptotic cell death in human colorectal carcinoma cell lines by a cyclooxygenase-2 (COX-2)-selective nonsteroidal anti-inflammatory drug: independence from COX-2 protein expression. Clin Cancer Res 3: 1679–1683
Gasparini G, Biganzoli E, Bonoldi E, Morabito A, Fanelli M, Boracchi P (2001) Angiogenesis sustains tumor dormancy in patients with breast cancer treated with adjuvant chemotherapy. Breast Cancer Res Treat 65: 71–75
Gately S (2000) The contributions of cyclooxygenase-2 to tumor angiogenesis. Cancer Metastasis Rev 19: 19–27
Gupta RA, DuBois RN (2001) Colorectal cancer prevention and treatment by inhibition of cyclooxygenase-2. Nat Review 1: 11–21
Han Z, Pantazis P, Wyche JH, Kouttab N, Kidd VJ, Hendrickson EA (2001) Fas-associated death domain protein-dependent mechanism mediates the apoptotic action of non-steroidal anti-inflammatory drugs in the human leukemic Jurkat cell line. J Biol Chem 276: 38748–38754
He Q, Luo X, Huang Y, Sheikh MS (2002) Apo2L/TRAIL differentially modulates the apoptotic effects of sulindac and a COX-2 selective non-steroidal anti-inflammatory agent in Bax-deficient cells. Oncogene 21: 6032–6040
Hida T, Kozaki K, Muramatsu H, Masuda A, Shimizu S, Mitsudomi T, Sugiura T, Ogawa M, Takahashi T (2000) Cyclooxygenase-2 inhibitor induces apoptosis and enhances cytotoxicity of various anticancer agents in non-small cell lung cancer cell lines. Clin Cancer Res 6: 2006–2011
Hsu AL, Ching TT, Wang DS, Song X, Rangnekar VM, Chen CS (2000) The cyclooxygenase-2 inhibitor celecoxib induces apoptosis by blocking Akt activation in human prostate cancer cells independently of Bcl-2. J Biol Chem 275: 11397–11403
Hung WC, Chang HC, Pan MR, Lee TH, Chuang LY (2000) Induction of p27 (K1P1) as a mechanism underlying NS398-induced growth inhibition in human lung cancer cells. Mol Pharmacol 58: 1398–1403
Johnson AJ, song X, Hsu A, Chen C (2001) Apoptosis signaling pathways mediated by cyclooxygenase-2 inhibitors in prostate cancer cells. Adv Enzyme Regul 41: 221–235.
King Jr JG, Khalili K (2001) Inhibition of human brain tumor cell growth by the anti-inflammatory drug, flurbiprofen. Oncogene 20: 6864–6870
Kopp E, Ghosh S (1994) Inhibition of NF-kappa B by sodium salicylate and aspirin. Science 265: 956–959
Liu XH, Yao S, Kirschenbaum A, Levine AC (1998) NS398, a selective cyclooxygenase-2 inhibitor, induces apoptosis and down-regulates bcl-2 expression in LNCaP cells. Cancer Res 58: 4245–4249
Lundholm K, Gelin J, Hyltander A, Lonnroth C, Sandstrom R, Svaninger G, Korner U, Gulich M, Karrefors I, Norli B (1994) Anti-inflammatory treatment may prolong survival in undernourished patients with metastatic solid tumors. Cancer Res 54: 5602–5606
Mann M, Sheng H, Shao J, Williams CS, Pisacane PI, Sliwkowski MX, DuBois RN (2001) Targeting cyclooxygenase 2 and HER-2/neu pathways inhibits colorectal carcinoma growth. Gastroenterology 120: 1713–1719
Nakashio A, Fujita N, Rokudai S, Sato S, Tsuruo T (2000) Prevention of phosphatidylinositol 3′-kinase-Akt survival signaling pathway during topotecan-induced apoptosis. Cancer Res 60: 5303–5309
Nicholson DW (2000) From bench to clinic with apoptosis-based therapeutic agents. Nature 407: 810–816
Nita ME, Tominaga O, Nagawa H, Tsuruo T, Muto T (1998) Dihydropyrimidine dehydrogenase but not thymidylate synthase expression is associated with resistance to 5-fluorouracil in colorectal cancer. Hepatogastroenterology 45: 2117–2122
Petak I, Houghton JA (2001) Shared pathways: death receptors and cytotoxic drugs in cancer therapy. Pathol Oncol Res 7: 95–106
Phillips RK, Wallace MH, Lynch PM, Hawk E, Gordon GB, Saunders BP, Wakabayashi N, Shen Y, Zimmerman S, Godio L, Rodrigues-Bigas M, Su LK, Sherman J, Kelloff G, Levin B, Steinbach G (2002) A randomised, double blind, placebo controlled study of celecoxib, a selective cyclooxygenase 2 inhibitor, on duodenal polyposis in familial adenomatous polyposis. Gut 50: 857–860.
Ricchi P, Pignata S, Di Popolo A, Memoli A, Apicella A, Zarrilli R, Acquaviva AM (1997) Effect of aspirin treatment on cell proliferation and differentiation of colon adenocarcinoma Caco-2 cells. Int J Cancer 73: 880–884
Ricchi P, Di Matola T, Ruggiero G, Zanzi D, Apicella A, di Palma A, Pensabene M, Pignata S, Zarrilli R, Acquaviva AM (2002) Effect of anti-inflammatory drugs on colon carcinoma Caco-2 cell responsiveness to topoisomerase inhibitor drugs. Br J Cancer 86: 1501–1509
Shusterman S, Grupp SA, Barr R, Carpentieri D, Zhao H, Maris JM (2001) The angiogenesis inhibitor tnp-470 effectively inhibits human neuroblastoma xenograft growth, especially in the setting of subclinical disease. Clin Cancer Res 7: 977–984
Sinicrope FA, Pazdur R, Levin B (1996) Phase I trial of sulindac plus 5-fluorouracil and levamisole: potential adjuvant therapy for colon carcinoma. Clin Cancer Res 2: 37–41
Sobrero A, Kerr D, Glimelius B, Van Cutsem E, Milano G, Pritchard DM, Rougier P, Aapro M (2000) New directions in the treatment of colorectal cancer: a look to the future. Eur J Cancer 36: 559–566
Song X, Lin HP, Johnson AJ, Tseng PH, Yang YT, Kulp SK, Chen CS (2002) Cyclooxygenase-2, player or spectator in cyclooxygenase-2 inhibitor-induced apoptosis in prostate cancer cells. J Natl Cancer Inst 94: 585–591
Soriano AF, Helfrich B, Chan DC, Heasley LE, Bunn Jr PA, Chou TC (1999) Synergistic effects of new chemopreventive agents and conventional cytotoxic agents against human lung cancer cell lines. Cancer Res 59: 6178–6184
Steinbach G, Lynch PM, Phillips RK, Wallace MH, Hawk E, Gordon GB, Wakabayashi N, Saunders B, Shen Y, Fujimura T, Su LK, Levin B (2000) The effect of celecoxib, a cyclooxygenase-2 inhibitor, in familial adenomatous polyposis. N Engl J Med 342: 1946–1952.
Thun MJ, Henley SJ, Patrono C (2002) Nonsteroidal anti-inflammatory drugs as anticancer agents: mechanistic, pharmacologic, and clinical issues. J Natl Cancer Inst 94: 252–266
Torrance CJ, Jackson PE, Montgomery E, Kinzler KW, Vogelstein B, Wissner A, Nunes M, Frost P, Discafani CM (2000) Combinatorial chemoprevention of intestinal neoplasia. Nat Med 6: 1024–1028
Yamamoto Y, Yin MJ, Lin KM, Gaynor RB (1999) Sulindac inhibits activation of the NF-kappaB pathway. J Biol Chem 274: 27307–27314
Yin MJ, Yamamoto Y, Gaynor RB (1998) The anti-inflammatory agents aspirin and salicylate inhibit the activity of I(kappa)B kinase-beta. Nature 396: 77–80
Wang CY, Cusack Jr JC, Liu R, Baldwin Jr AS (1999) Control of inducible chemoresistance: enhanced anti-tumor therapy through increased apoptosis by inhibition of NF-kappaB. Nat Med 5: 412–417
Waskewich C, Blumenthal RD, Li H, Stein R, Goldenberg DM, Burton J (2002) Celecoxib exhibits the greatest potency amongst cyclooxygenase (COX) inhibitors for growth inhibition of COX-2-negative hematopoietic and epithelial cell lines. Cancer Res 62: 2029–2033.
Zhang L, Yu J, Park BH, Kinzler KW, Vogelstein B (2000) Role of BAX in the apoptotic response to anticancer agents. Science 290: 989–992
Zhou XM, Wong BC, Fan XM, Zhang HB, Lin MC, Kung HF, Fan DM, Lam SK (2001) Non-steroidal anti-inflammatory drugs induce apoptosis in gastric cancer cells through up-regulation of bax and bak. Carcinogenesis 22: 1393–1397
Acknowledgements
The work performed in the authors' laboratory was supported in part by grants from Associazione Italiana per la Ricerca sul Cancro (AIRC) and Ministero dell'Istruzione dell' Università e della Ricerca Scientifica e Tecnologica (MIUR), Italy. We thank Mr M Berardone for excellent artwork.
Author information
Authors and Affiliations
Corresponding author
Rights and permissions
From twelve months after its original publication, this work is licensed under the Creative Commons Attribution-NonCommercial-Share Alike 3.0 Unported License. To view a copy of this license, visit http://creativecommons.org/licenses/by-nc-sa/3.0/
About this article
Cite this article
Ricchi, P., Zarrilli, R., di Palma, A. et al. Nonsteroidal anti-inflammatory drugs in colorectal cancer: from prevention to therapy. Br J Cancer 88, 803–807 (2003). https://doi.org/10.1038/sj.bjc.6600829
Received:
Revised:
Accepted:
Published:
Issue Date:
DOI: https://doi.org/10.1038/sj.bjc.6600829
Keywords
This article is cited by
-
Anticancer potential of Carica papaya Linn black seed extract against human colon cancer cell line: in vitro study
BMC Complementary Medicine and Therapies (2023)
-
Protective potential of piroxicam on human peripheral blood mononuclear cells against the suppressive capacity of glioblastoma cell lines
Scientific Reports (2022)
-
CMTM6 expression in M2 macrophages is a potential predictor of PD-1/PD-L1 inhibitor response in colorectal cancer
Cancer Immunology, Immunotherapy (2021)
-
Indomethacin Disrupts Autophagic Flux by Inducing Lysosomal Dysfunction in Gastric Cancer Cells and Increases Their Sensitivity to Cytotoxic Drugs
Scientific Reports (2018)
-
Thermodynamics in cancers: opposing interactions between PPAR gamma and the canonical WNT/beta‐catenin pathway
Clinical and Translational Medicine (2017)