Abstract
Feeding habits of fish larvae were analysed in a comparative study of five species (Oreochromis niloticus, O. aureus, Tilapia zilli, Mugil cephalus and Liza ramada) from the Burullus Lake. We investigated the potential influence of larvae size on their feeding and looked for common patterns in larval prey preference. Gut contents of a total of 1068 larvae were examined. The feeding habits of the examined larvae shared some characteristics. As larvae of the five species grew, the preferred prey size and the number and diversity of prey in the gut increased. On the other hand, larval feeding also differed in several aspects, especially differences in the composition of preferred prey items. For Cichlidae larvae, the dominant prey was the rotifer Brachionus plicatilis followed by copepodite stages. For M. cephalus larvae, the copepods Paracalanus sp and Acartia sp were the most important diets, while for L. ramada, decapod larvae were the most important. The Mugilidae larvae consumed significantly larger prey than the cichlid larvae, which could be related to their morphology, especially the mouth size. Ivlev’s selectivity index calculated for larvae showed positive selection for particular prey. In all the species, copepods were the most selected, particularly for Mugillidae larvae, but for Cichlidae larvae, the rotifer Brachionus competed with copepods as a selected prey. This dietary study of fish larvae of these important species is essential for the development of fisheries and can be implemented in further related studies of other areas.
Similar content being viewed by others
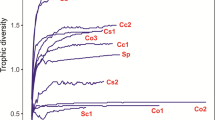
Avoid common mistakes on your manuscript.
Introduction
Lake Burullus is the second largest lake of the northern lakes of the Egyptian coast. The lake lies at the eastern side of Rosetta Nile Branch, occupying a central position along Egypt’s Mediterranean Nile Delta coast. This lake has a distinctive nature, connected to the Mediterranean Sea on the northern side with a small opening called El-Boughaz. Eight drains discharge a mix of industrial, agricultural, sewage, wastes, and fish farm drainage water into the lake. Also, the lake receives fresh Nile water from the western side through the Brenbal canal (El-Adawy et al. 2013). The lake water characteristics, ranging from brackish to saline waters, have resulted in a large variety of fish species inhabiting the lake. Approximately 32 species were recorded in the lake (Hassan and Mohamed 2023). Three species belonging to the family Cichlidae (Tilapia zillii (Gervais 1848), Oreochromis niloticus (Linnaeus 1758), and O. aureus (Steindachner 1864), in addition to two Muglidae species (Mugil cephalus (Linnaeus 1758) and Liza ramada (Risso 1827), are among the most economic species in fish production (Maltby et al. 2011).
Fish fries are considered the potential stock of future fish production in the aquatic ecosystem. Knowing the abundance and distribution of fish larvae in conjunction with ecological conditions is vital for fishery management (El-Tohamy 2012). The quantity and quality of food are the most critical factors affecting the growth of fish larvae (Das et al. 2012). One of the most important influences on the survival of fish larvae is the availability of suitable food, and knowledge of their feeding behaviour is necessary for an understanding of the factors that affect the mortality of the larvae in the wild and the subsequent year-class strengths of the adult fish (Last 1980).
Zooplankton is an essential food for newly hatched fish fries, which depend mainly on plankton as food and energy sources. The fish spawning cycle is linked primarily to zooplankton production cycles, especially Rotifera, Copepoda, and Cladocera (Milstein et al. 2006). According to Penaz (2001), most fish larvae are visually hunting predators exhibiting size-selective feeding. Fish larvae < 20 mm predate mostly on small zooplankton (e.g., rotifers and small cladocerans), while larvae > 20 mm select larger prey species (e.g., Daphnia and copepods) (Mehner and Thiel 1999). The diet of an assemblage of fish larvae in an area will probably reflect the plankton composition within a certain size range in that area or may be related to changes in zooplankton communities through time (Gaughan 1991).
The assemblage of Cichlidae and Muglidae larvae often dominates the 0 + age class in the fish community of the northern Egyptian water bodies in the period from late autumn to late spring (Khalil 1990; El-Tohamy 2012; El Kafrawy et al. 2019). The shifts in habitats and diets observed in the fish larvae between the different systems may be linked to resource limitation (e.g., food item availability) and the risk of predation (Kobler et al. 2009). Cichlids are among the most important freshwater fishes in tropical and subtropical regions; they tolerate poor water conditions (Turner 2007). Their biomass and age state affect zooplankton richness and biomass (Ufodike and Wada 1991). Diet studies indicated that the adults of the three cichlids, O. niloticus, O. aureus, and T. zilli are omnivorous species, feeding on plants, plankton, and small insects, while larvae and juveniles have more varied diets, including copepods, rotifers and cladocerans (McKaye et al. 1995; Attayde et al. 2007; Mohammed et al. 2021). Mugilidae is a widely distributed family; most species are euryhaline, inhabiting coastal marine waters, brackish water lagoons, and estuaries, and may enter freshwaters (Koutrakis 2016). They lay their eggs in open seas, and the young ones drift ashore into saltmarshes and estuaries to grow into juveniles (Nordlie 2000). The larvae of M. cephalus and L. ramada are zooplanktivorous, and the interspecies trophic overlap is high among fry (Torricelli et al. 1988; Tosi and Torricelli 1988; Salvarina et al. 2010). Therefore, when there is resource limitation, e.g., when preferred zooplankton prey as copepods is scarce in a stressed habitat, this could be a limiting factor that could influence the distribution, habitat preference and structuring of larvae assemblages (Gisbert et al. 1995).
Although numerous studies are available on the feeding habits and ecology of several adult fish in Burullus Lake (Sal’nikov et al. 1980; Zaghloul et al. 2007; El-Sayed 2017; Khalil 2019; Elzohry et al. 2020; Hassanien et al. 2020; Azzam et al. 2022), little is known about the ecology and feeding habits of commercial fish larvae in the area as well as in the Egyptian Mediterranean coast, except El-Ghobashy (2009a, b), Abdel-Aziz and Heneash (2011) and El-Tohamy (2012). On the other hand, several studies were conducted on the zooplankton communities in Burullus Lake (e.g. Ramdani et al. 2001; Saad et al. 2006; El-Shabrawy and Bek 2019; Alprol et al. 2021; Alzeny et al. 2021; Alprol et al. 2022), but nothing is known to be dealt with ecology and biology of fish larvae and their relation to zooplankton.
This work aims to describe and compare the food and feeding habits of O. niloticus, O. aureus, T. Zilli, M. cephalus, and L. ramada, larvae of important fish species in the Burullus Lake. Selective feeding, diet composition, and changes in diet at different sizes are discussed.
Materials and Methods
Field Methods
Samples of fish larvae from Lake Burullus were collected concurrently with the zooplankton samples at two stations in the period from April 2015 to March 2016 to study the feeding habits of the fish larvae. Station I in the El-Boughaz area, affected mainly by seawater intrusion more than drainage water, acts as a transition area between the seawater and the brackish water. Vast amounts of marine fries, particularly of the Mugillidae, rush into this area (Maltby et al. 2011). Station II is located at the front of the El-Burollus drain (Fig. 1). Each species larvae collection depends upon the time of occurrence and the place where they occur, using an Ichthioplankton net with a mesh size of about 0.5 mm. O. niloticus (163 individuals), O. aureus (127 individuals), and T. zilli (142 individuals) were collected during April and May from the mouth of station II, while M. cephalus (307 individuals) larvae were collected from September to December and L. ramada (329 individuals) were collected from January to April at station I. Fish larvae samples were kept in ice until arriving to the laboratory.
Laboratory Analysis
In the laboratory, the fish larvae were identified. We measured the total length and total weight of the larvae. For gut analysis, the entire gut was removed, preserved in 5% neutral formalin, and cut open through in a petri dish; the dietary items in the guts were identified to the nearest taxonomic level as possible under a research microscope and counted (expressed as organisms/stomach).
Data Analysis
The index of relative importance (IRI) of each food category was calculated according to the equation of Laroche (1982):
where F% is the frequency of occurrence, and N% is the numerical percentage.
The selectivity for different prey species was quantified by Ivlev’s indicator of selectivity (Ivlev 1961):
where (pi) is the relative abundance of prey items in the environment and (ri) is the relative abundance of prey items in the diet. Ivlev’s Index (E) has values between + 1 and − 1; if (0 > E ≥ + 1), then the prey is preferred, while (0 < E ≤ -1) means that the food item avoided as food by fish larvae, finally if (E = 0) implies that the preys are accepted depending on their density.
Shannon’s (H’) diversity index (Shannon 1948) of the food items was calculated as follows:
where ni is the abundance of species i, N is the total abundance in the sample, and ln is the natural logarithm.
Species Evenness (J΄) was calculated by using the following Heip (1974) equation:
where H΄ is the diversity index, S is the species number, and ln is the natural logarithm.
Spearman’s correlation was used to describe the relationship between some selected parameters. The Student’s t-test for paired samples was used to find the significance between length and weight groups of fish larvae and food item variables. Ward’s clustering method based on the Euclidean distances was used to determine the dietary overlap between species (Krebs 1998). All statistical analyses were carried out using SPSS version 20 software (SPSS Inc., Chicago, IL, USA).
Results
The Stomach Content of Fish Larvae
The present study revealed that zooplankton organisms were the main food of the studied fish larvae. However, the numbers of food items and their numerical densities demonstrated pronounced variations between the different larvae.
Change in Food Composition with Size and Weight of Fish Larvae
The collected larvae of Mugil cephalus were divided into three length groups (Table 1). The food contents varied between 4 and 11 items, with the highest value for the length group of 2.7–2.9 cm. The average numerical density of individuals related positively with length, giving nearly 2, 4, and 5 organisms/stomach for the three-length group, respectively. The Shannon index of the food items varied within the range of 1.13–1.98, with the highest value in the second length group. The evenness values coincided in their variations with those of the Shannon index. M. cephalus larvae were also divided into three groups according to weight. Food’s number and numerical density showed a direct relationship with weight, as the lowest values were found in the smallest weight group, and the highest values occurred in the largest weight group (Table 1). The maximum values of both diversity index and evenness were found at weights of 0.151-0.20 g.
Larvae of Liza ramada were divided into three length and three weight groups (Table 1). For the smallest group (2–2.1 cm), the food was composed of 7 items, increased to 15 items in the second length group, then decreased to 10 in the longest group. The average numerical density of food items was about 1.41 organisms/stomach for the smallest larvae, which increased to about five organisms/stomach for the longest groups. The maximum diversity index of the food items (1.7) was found at lengths of 2–2.1 cm. According to the weight of L. ramada larvae, the number and abundance of food items showed a direct relationship with larvae weight, as the lowest number (6 items) was found in the smallest weight group which showed the lowest average weight (1.32), the highest number (14 items) occurred in the largest weight group. The diversity index of the food items showed more or less similar values with relatively higher ones for the first weight group (0.059–0.0765 g). The evenness values coincided in their variations with those of the Shannon index (Table 1).
The collected larvae of O.niloticus were divided into three length groups (Table 2). The smallest group (0.85–1 cm) has 8 food items increased to 14 items at the length group of 1.1–1.5 cm then decreased to only 5 items at the longest group. The average numerical abundance decreased with increasing lengths. The diversity index of the food items showed comparatively higher value in the second length group, while evenness attained its maxima in the longest group. Relative to the weight, O. niloticus larvae demonstrated 2 groups only, both of them showed a similar number of food items (Table 2). The average numerical density of food items was about 10 organisms/stomach for the smallest weight group, which decreased to about 6 organisms/stomach for the other group.
Oreochromis aureus larvae were grouped into three length groups (Table 2). The number of food items increased with increasing length, while the average abundance of items decreased with increasing length. Also, both Shannon’s diversity index and evenness values increased with increasing length. According to weight, O. aureus larvae were divided into three groups. The food items showed similar numbers with the two first groups, except for the large weight group, which sustained a pronouncedly low number of food items. The abundance of food was the highest (10 organisms/stomach) in the smallest weight group, but it was pronouncedly low (˂ 1organisms/stomach) in the largest weight group (Table 2). The Shannon diversity index reported close values in the two biggest groups (1–1.3) and less so (0.62) in the smallest group which showed the lowest evenness value. The collected larvae of T. zilli, as the previous cichlid (O. aureus ), were divided into three groups according to length and weight (Table 2). The number and abundance of food items were the highest in the second length group of 1–1.3 cm, which showed the maximum value of diversity. According to weight, the food items showed negligible differences between the groups, but their numerical abundance decreased with increasing weight.
As shown in Table 3, statistical analysis based on Student paired sample t-test showed a few cases of significance between length and weight groups of the five collected larvae and variables of number, abundance, Shannon, and evenness of food items. The abundance was significant only between the length and weight groups of M. cephalus. The number of food items was significant between weight groups of L.ramada, O. niloticus, T. zill, and length groups of O. aureus, which showed the only significant variation with the Shannon index.
Species Composition of Food Content in the Fish Larvae
The index of relative importance (IRI) of the food items (Fig. 2) showed that the copepod Paracalanus sp and Acartia sp were the most important food items for M. cephalus (IRI: 857.02 and 395.56, respectively), besides which the decapod larvae, copepodite stages, and the copepod Acartia latisetosa were found among the important food components for the same species, but with lower IRI (Fig. 2). For L. ramada, decapod larvae ranked as the first important food item (IRI: 2320.47), followed by Paracalanus sp (IRI: 235.16), and the copepod Acanthocyclops americanus (IRI: 82.48). For the three species of Cichlidae, the rotifer Brachionus plicatilis was the absolute dominant one with IRI of 2353.82, 3719.51, 3388.55 for O. niloticus, O. aureus, and T. zilli, respectively. In addition, the copepodite stages and the cladoceran Moina micrura showed considerable IRI values but with markedly lower importance in the food of Cichlidae larvae.
A dendrogram of the species distribution using Ward’s method showed two main groups (clusters) hierarchically agglomerated based on Euclidean distances of the dietary overlap among the fish larvae species (Fig. 3). Copepods rotifers feeders (group A in Fig. 3) comprised the three cichlidis species. Although copepods were more diversified as food items, rotifer species like Brachionus plicatilis was the most important for all members. Copepod feeders (group B) included the two species of Mugillidae, which consumed mainly the saltwater copepod. The feeding diversity values were higher for M. cephalus and L. ramada than for other species (Fig. 3).
Dendrogram constructed based on mean percentage of food items for each fish species. TL Total length, TW Total weight, n number of fish examined, H Shannon–Wiener diversity index. Collection time abbreviations, Sp Spring, A Autumn, W Winter. Food items abbreviations, Pro Protozoa, Rot Rotifera, Cop Copepoda, Cla Cladocera, Ost Ostracoda, Mys Mysidacea, Mer Meroplankton
Prey Selection
Clear prey selection was observed in all studied fish species, although in Oreochromis aureus and Tilapia zilli, it was less pronounced (Fig. 4). Mugil cephalus showed positive selectivity for the majority of food items (13 items). Acartia latisetosa, Acartia sp, Paracalanus sp, and decapod larvae were the most preferable for M. cephalus. Acartia clausi, Oithona nana, Eucyclops agilis, Halicyclops magniceps, Moina micrura, and nauplius larvae were not preferable for this fish. Liza ramada showed positive selectivity for ten items, especially Acartia clausi, Acartia sp, Paracalanus sp, and decapod larvae. On the other hand, Diacyclops bicuspidatus odessanus, Halicyclops magniceps, Eucyclops sp, Cypridina mediterranea, Diaphanosoma excisum, Daphnia magna, Monia micrura, nauplius larvae, and copepodite stages showed negative selectivity.
In O. niloticus, eight items were positively selected: Brachionus plicatilis, Eucyclops agilis, copepodite stage, and insect larvae were the most preferable. As the negative food selectivity indicated, nine items were considered escaping zooplankton items with nearly complete avoidance for nauplius larvae and Brachionus angularis. O. aureus and T. zilli were fed by nearly the same items (5 & 4 items, respectively), Brachionus plicatilis, Nitocera lacustris, and copepodite stages showed the highest positive selectivity. On the other side, twelve items escaped. Complete avoidance for many zooplankton items was observed, such as the rotifers Brachionus urceolaris, the copepod Diacyclops bicuspidatus odessanus, the cladoceran Diaphanosoma excisum, in addition to the larvae of nauplii and decapods (Fig. 4).
Discussion
The existence and survival of fish larvae are affected mainly by some factors such as the abundance of their food and the surrounding environmental conditions (El-Tohamy 2012), also larval abundances illustrated clear links between on/off shore current direction and life history of different fish assemblages (Pattrick et al. 2021). Water temperature, photoperiod, and food availability are the main factors controlling fish reproduction (Carrillo et al. 1995). The correlation analysis in the present investigation indicated that temperature (r = -0.65, P˂ 0.001) is the leading environmental factor controlling the distribution of fish larvae in the study area.
During the present study, Mugil cephalus and Liza ramada were collected from station I in the El-Boughaz area, where the water salinity fluctuated between marine and oligohaline, agreed with Hotos and Vlahos (1998), who found a broad range of salinity for Mugilidae. On the other hand, the larvae of Cichlidae were collected from station II at the front of the El-Burollus drain, which sustained much lower salinity all year round. The salinity fluctuation most of the year may result in the low abundance of Cichlidae larvae in the El-Boughaz region. On the other hand, the high input of nutrients at station II most of the year (El-Tohamy et al. 2014) stimulates primary and secondary production and positively affects the nursery function (Kimmerer 2002), particularly for cichlids. Also, it was shown in this study that L. ramada and M. cephalus prefer mesohaline waters and nutrient-rich areas, which are considered a source of food for Mugilidae (Gordo and Cabral 2001). M. cephalus was collected from September to December, and L. ramada was collected from January to April. This agreed with the period of collection of El-Tohamy (2012) from Damietta Coast and that of Almeida (1996) for the L. ramada, ensuring the importance of time for fish larvae. The spatial and temporal existence of fish larvae likely resulted from a combination of biotic factors such as food availability, predation, competition (Hagan and Able 2003), and abiotic factors such as salinity (Martino and Able 2003), temperature (Marshall and Elliott 1998), dissolved oxygen (Maes et al. 1998), and freshwater inflow (Fraser 1997).
The Shannon–Wiener Diversity indices (Hʹ values) indicated that the fishes studied here were characterised by large, and generalist tendencies, consuming a wide variety of food items. Mugilidae higher diversity values than Cichlidae larvae could be related to their morphology, especially the mouth size, which functions as a “filter” to collect more food items (Cardona 2016). Also, as larvae grow, the diversity and proportion of larger items in the diet increase. This shift may be associated with the rise in their energetic requirements (Sanchez-Velasco 1998). The smallest size Cichlidae larvae were mainly dependent on rotifers, while with increasing length and mouth diameter, the feeding habits and food selectivity changed toward the larger zooplankton assemblages like copepods, cladocerans, and insect larvae; findings agreed with that of Thiel et al. (1996). Other species comparisons have also shown such prey size differences related to mouth sizes (e.g. Østergaard et al. 2005; Swalethorp et al. 2015).
A comparison between the stomach contents of the studied larvae and zooplankton in Burullus Lake showed that most larval food items were found in the lake. The most common food items were copepodites, the copepods paracalanus sp, and the rotifer Brachionus plicatilis. Moreover, most freshwater zooplankton species (rotifers, cladocerans, and freshwater copepods like Acanthocyclops americanus and Halicyclops magniceps) were more frequent in the stomach contents of the larvae of Cichlidae, which are best adapted to low salinities. Differing from M. cephalus larvae, the L. ramada larvae tended to consume more cyclopoid and harpacticoid copepods (e.g. Oithona nana and Euterpina acutifrons) than calanoid copepods (Paracalanus sp. and Acartia spp.), this behaviour was also observed in larvae of L. ramada from the Damietta coast in the southeastern Mediterranean (El-Ghobashy 2009a, b and El-Tohamy 2012). Domination of copepods in larval diets was possible thanks to their well-developed ability to capture and handle this evasive prey (Jachner 1991). The common prey organisms for the five species were Acanthocyclops americanus, Paracalanus sp, and Moina micrura. These species were among the least abundant ones in zooplankton but were occasionally abundant in larval diets. It suggested that these organisms were active prey, probably due to their optimal foraging and good visibility. On the other hand, food selectivity by fish larvae towards different meroplanktonic groups was different. Although the larvae of cirripedes, decapods, and insects were more preferred, other important meroplankton components such as polychaetes and bivalve larvae were completely missed from the larval diet. Copepod nauplii were not an important component in the diet of the fish larvae analysed in this study, even though they were significantly dominant in the lake planktonic environment (e.g., Saad et al. 2006; El-Shabrawy and Bek 2019). This is in agreement with the study of El-Tohamy (2012) but contrasts with the composition of the diet of many marine fish larvae, which frequently consume copepod nauplii, because of their great abundance in the environment (e.g. Watson and Davis 1989; Jachner 1991; Welker et al. 1994; Lehtiniemi et al. 2007).
When we look at the feeding selectivity of various zooplankton species, their relative abundances in the natural environment should be considered. According to Confer et al. (1990), food selectivity depends on food abundance; high abundance may be correlated with easier prey capture (Govoni et al. 1986). However, these observations contradict the present study; the selectivity did not depend upon food abundance, as many dominant zooplankton in the lake were missed from the larvae’s diet. We observed prey selection in all studied fish larvae at different sizes. Further, it is interesting that prey selection is also evident at low prey concentrations for certain types. According to Lehtiniemi et al. (2007), prey selection can be divided into two main categories: (1) apparent selection, which means that prey capture is affected by motion behaviour and escape capabilities, and (2) true selection, which is based on predator’s active choice. In Mugilidae, the prey selection is clearly ‘true selection’ because the fastest prey, adults copepod (Viitasalo et al. 2001) were selected over other prey types. M. cephalus and L. ramada selected the largest prey positively, which offers a good energy source for growing larvae, and negatively selected small prey (mostly rotifers and copepod nauplii). In Cichlidae larvae, the prey selection is quite random. Both small (e.g. rotifers) and large (e.g. adult copepods) prey types are positively selected. We noticed that small rotifers are the preferred prey for the three cichlids when they start exogenous feeding, but when larvae grow, their preference switches to copepods and insect larvae. In general, most studied fish larvae preferred to feed on larger prey such as Cypridina Mediterranean, Mysis relicta, decapod, and insect larvae, which give fish more energy and save more effort in searching their diet (Brooks and Dodson 1965). The selectivity index of these previous prey was mostly close to + 1, indicating that some fish larvae preferred the large-sized prey rather than the small ones.
Of note, the fish larvae that overlapped in distribution often ingested different prey types. Species-specific differences in the diversity of prey size and type, may reduce competition for food (Swalethorp et al. 2015). Especially in the El-Boughaz area, we observed that larvae residing here ingested different sizes and types of prey. Such difference in diet was less evident for the El-Burollus drain area where rotifers were the most dominant (Saad et al. 2006), which typically are less preferred when by most fish larvae.
Conclusions
The present study provides a baseline for evaluating larval fish assemblages of five important species in Burullus Lake. The pattern of fish larvae distribution appeared to be linked to specific water characteristics and the availability of their preferred zooplankton prey. Variations and flexibility in individual prey selection may be associated with increased feeding success, which has important implications for the study of larval fish feeding. The fish larvae in the present study preyed on different zooplankton assemblages, including rotifers, cladocerans, copepod larvae (nauplii and copepodites), and adult stages of copepods. The mysid Mysis relicta, decapod, and insect larvae were also reported in the diets of the most studied larvae. The occurrence of different zooplankton sizes in the diet of these larvae may indicate that these larvae can feed upon a wide array of zooplankton assemblages, even those of macrozooplankton. Also, as larvae grow, the proportion of larger items in the diet increases. The food items selected by Mugilidae changed from small-size copepods to decapod larvae. For Cichlidae, the prey sizes changed from the small-size rotifers to the larger copepods and insect larvae.
Data Availability
The data is available upon request.
References
Abdel-Aziz NE, Heneash AM (2011) Zooplankton community at fish fry collection sites along the Mediterranean coast of Egypt. Egypt J Aquat Res 37:243–259
Almeida PR (1996) Estuarine movement patterns of adult thin-lipped grey mullet, Liza ramada (Risso)(Pisces, Mugilidae), observed by ultrasonic tracking. J Exp Mar Biol Ecol 202:137–150
Alprol AE, Heneash AMM, Ashour M, El-Kafrawy S, Soliman AM (2022) Chemical assessment of water quality, heavy metals, and the distribution of zooplankton communities, based on field and GIS data in the drains of Burullus Lake, Egypt. Arab J Geosci 15:1511
Alprol AE, Heneash AMM, Soliman AM, Ashour M, Alsanie WF, Gaber A, Mansour AT (2021) Assessment of water quality, eutrophication, and zooplankton community in Lake Burullus. Egypt Divers 13:268
Alzeny A, Abdel-Aziz NE, El-Ghobashy AE, El-Tohamy WS (2021) Distribution, composition, and abundance of protozoa in Burullus Lake, Egypt, and relationships with environmental variables. Reg Stud Mar Sci 48:102076
Attayde JL, Okun N, Brasil J, Menezes RF, Mesquita P (2007) Impactos Da introdução Da tilápia do Nilo, Oreochromis niloticus, sobre a estrutura trófica dos ecossistemas aquáticos do Bioma Caatinga. Oecologia Brasiliensis 11:450–461
Azzam TA, Hussien MS, El-Ganainy AA, Al-Zayat AM (2022) Species composition, distribution and morphometric parameters of four Penaeid Shrimp Species from Lake Burullus. Egypt J Aquat Biol Fish 26:77–94
Brooks JL, Dodson SI (1965) Predation, Body Size, and composition of Plankton: the effect of a marine planktivore on lake plankton illustrates theory of size, competition, and predation. Science 150:28–35
Cardona L (2016). In: Blaber S (ed) Biology, Ecology and Culture of Grey mullets (Mugilidae). Crosetti D and. Taylor and Francis, Australia
Carrillo M, Zanuy S, Prat F, Cerdá J, Ramos J, Mañanós E, Bromage N (1995) Sea bass (Dicentrarchus labrax). In: Bromage NR, Roberts RJ (eds) Broodstock management and egg and larval quality. Blackwell, Oxford, pp 138–168
Confer JL, Mills EL, O’Bryan L (1990) Influence of prey abundance on species and size selection by young yellow perch (Perca flavescens). Can J Fish Aquat Sci 47:882–887
Das P, Mandal SC, Bhagabati S, Akhtar M, Singh S (2012) Important live food organisms and their role in aquaculture. Front Aquac 5:69–86
El-Adawy A, Negm AM, Elzeir MA, Saavedra OC, El-Shinnawy IA, Nadaoka K (2013) Modeling the hydrodynamics and salinity of El-Burullus Lake (Nile Delta, northern Egypt). J Clean Energy Technol 1:157–163
El-Ghobashy AE (2009a) Ecological and biological assessment of a wild mullet fish fry Collection Station at the Egyptian Mediterranean water. World J Fish Mar Sci 1:268–277
El-Ghobashy AE (2009b) Natural fish fry food of seven commercial species in the Egyptian Mediterranean water. World Appl Sci J 7:320–331
El-Kafrawy SB, Bek MA, Negm AM (2019) An overview of the Egyptian northern coastal lakes. In: Negm AM, Bek MA, Abdel-Fattah S (eds) Egyptian Coastal Lakes and wetlands: part I - characteristics and hydrodynamics. Springer International Publishing, Berlin, pp 3–17
El-Sayed AFM (2017) Fish and fisheries in the Nile Delta. In: Negm A (ed) The Nile Delta. Springer, pp 495–516
El-Shabrawy GM, Bek MA (2019) Responses of zooplankton to long-term environmental changes in the Egyptian coastal lakes. In: Negm A, Bek M, Abdel-Fattah S (eds) Egyptian Coastal Lakes and wetlands: the Handbook of Environmental Chemistry, vol 72. Springer, pp 147–177.
El-Tohamy WS (2012) Food relations between larvae of economic fishes and zooplankton in some Egyptian coastal areas of the Mediterranean Sea. PhD Thesis, Damietta University, Damietta, pp 228
El-Tohamy WS, Abdel Aziz NEM, El Ghobashy AE, Alzeny A (2014) Water quality assessment of Burullus Lake using multivariate analysis. World J fish mar sci 6:564–572
Elzohry E, Eladawy RS, Elsawy SB (2020) An economic study on the determinants of fish production in Burullus Lake. J Sustain Agric Sci 46:135–146
Fraser TH (1997) Abundance, seasonality, community indices, trends and relationships with physicochemical factors of trawled fish in upper Charlotte Harbor, Florida. Bull Mar Sci 60:739–763
Gaughan DJ (1991) Feeding by estuarine and marine fish larvae. Mar Ecol Prog Ser 51:19–33
Gisbert E, Cardona L, Castelló F (1995) Competition between mullet fry. J Fish Biol 47:414–420
Gordo LS, Cabral HN (2001) The fish assemblage structure of a hydrologically altered coastal lagoon: the Óbidos lagoon (Portugal). Hydrobiologia 459:125–133
Govoni JJ, Ortner PB, Al-Yamani F, Hill LC (1986) Selective feeding of spot, Leiostomus xanthurus, and Atlantic croaker, Micropogonias undulatus, larvae in the northern Gulf of Mexico. Mar Ecol Prog Ser 28:175–183
Hagan SM, Able KW (2003) Seasonal changes of the pelagic fish assemblage in a temperate estuary. Estuar Coast Shelf Sci 56:15–29
Hassan NHS, Mohamed EEE (2023) The views and knowledge of the fishermen on the impact of Lake Burullus Development on their community. Egypt J Aquat Biol Fish 27:69–84
Hassanien HA, AlSaqufi AS, El-Beltagi HS, Abou Zied RM, Eldeeb KA (2020) Estimation of flathead grey mullet Mugil cephalus differentiation using multivariate analyses for morphometric data. Fresenius Environ Bull 29:10941–10953
Heip C (1974) A new index measuring evenness. J Mar Biol Assoc UK 54:555–557
Hotos GN, Vlahos N (1998) Salinity tolerance of Mugil cephalus and Chelon labrosus (Pisces: Mugilidae) fry in experimental conditions. Aquac 167:329–338
Ivlev VS (1961) Experimental ecology of the feeding of fishes. Yale University Press, New Have
Jachner A (1991) Food and habitat partitioning among juveniles of three fish species in the pelagial of a mesotrophic lake. Hydrobiologia 226:81–89
Khalil MT (1990) Plankton and primary productivity of Lake Manzala, Egypt. Hydrobiologia 196:201–207
Khalil MT (2019) Fisheries of Egyptian delta coastal wetlands; Burullus Wetland case study. In: Negm A, Bek M, Abdel-Fattah S (eds) Egyptian Coastal Lakes and wetlands: the Handbook of Environmental Chemistry, vol 72. Springer, pp 179–198
Kimmerer WJ (2002) Effects of freshwater flow on abundance of estuarine organisms: physical effects or trophic linkages? Mar Ecol Prog Ser 243:39–55
Kobler A, Klefoth T, Mehner T, Arlinghaus R (2009) Coexistence of behavioural types in an aquatic top predator: a response to resource limitation? Oecologia 161:837–847
Koutrakis E (2016) Biology and ecology of fry and juveniles of Mugilidae. In: Crosetti D, Blaber SJM (eds) Biology, ecology and culture of grey mullet (Mugilidae). CRC Press, Boca Raton, pp 264–292
Krebs C (1998) Ecological methodology. Harper & Row, New York
Laroche JL (1982) Trophic patterns among larvae of five species of sculpins (family: Cottidae) in a Maine estuary [Myoxocephalus aenaeus, Myoxocephalus octodecemspinosus, Myoxocephalus scorpius, Triglops murrayi, Hemitripterus americanus, Damariscotta River]. Fish Bull U S Nat Mar Fish Ser 80:827–840
Last MJ (1980) The food of twenty species of fish larvae in the West-Central North Sea. Ministry of Agriculture, Fisheries and Food, Lowestoft
Lehtiniemi M, Hakala T, Saesmaa S, Viitasalo M (2007) Prey selection by the larvae of three species of littoral fishes on natural zooplankton assemblages. Aquat Ecol 41:85–94
Maes J, Van Damme PA, Taillieu A, Ollevier F (1998) Fish communities along an oxygen-poor salinity gradient (Zeeschelde Estuary, Belgium). J Fish Biol 52:534–546
Maltby E, Ormerod S, Acreman M, Dunbar M, Jenkins A, Maberly S, Ward R (2011) Freshwaters–openwaters, wetlands and floodplains. The UK national ecosystem assessment technical report. UNEP-WCMC, Cambridge, pp 295–360
Marshall S, Elliott M (1998) Environmental influences on the fish assemblage of the Humber estuary, UK. Estuar Coast Shelf Sci 46:175–184
Martino EJ, Able KW (2003) Fish assemblages across the marine to low salinity transition zone of a temperate estuary. Estuar Coast Shelf Sci 56:969–987
McKaye KR, Ryan JD, Stauffer JR Jr, Perez LJ, Vega GI, van den Berghe EP (1995) African Tilapia in Lake Nicaragua. Bioscience 45:406–411
Mehner T, Thiel R (1999) A review of predation impact by 0 + fish on zooplankton in fresh and brackish waters of the temperate northern hemisphere. Environ Biol Fishes 56:169–181
Milstein A, Valdenberg A, Harpaz S (2006) Fish larvae–zooplankton relationships in microcosm simulations of earthen nursery ponds. I. Freshwater system. Aquac Int 14:231–246
Mohammed YM, Abubakar MD, Muhammad AM, Muhammad AS, Umar BL, Hadizat M, Achebe AC (2021) Stomach content of Tillapia zilli and Oreochromis Nilocticus from Wanzun River, Lavun LocalGovernment, Niger State Nigeria. Asian J Fish Aquat 13:15–20
Nordlie FG (2000) Patterns of reproduction and development of selected resident teleosts of Florida salt marshes. Hydrobiologia 434:165–182
Østergaard P, Munk P, Janekarn V (2005) Contrasting feeding patterns among species of fish larvae from the tropical Andaman Sea. Mar Biol 146:595–606
Pattrick P, Weidberg N, Goschen WS, Jackson JM, McQuaid CD, Porri F (2021) Larval fish assemblage structure at coastal fronts and the influence of environmental variability. Front Ecol 9:684502
Penaz M (2001) A general framework of fish ontogeny: a review of the ongoing debate. Folia Zool 50:241–256
Ramdani M, Flower RJ, Elkhiati N, Birks WCH, Kraïem MM, Fathi AA (2001) Zooplankton (Cladocera, Ostracoda), Chironomidae and other benthic faunal remains in sediment cores from nine north African wetland lakes: the CASSARINA Project. Aquat Ecol 35:389–403
Saad AE, Khalil M, El-Shabrawy G, Bcdir T (2006) Ecological studies on zooplankton community of Lake Burullus, Egypt. Egypt J Aquat Biol Fish 10:21–43
Sal’nikov NY, Hussein HA, Amin EM (1980) Some behavioral features and fishing of the European eel, Anguilla anguilla, in Lake Burullus (Egypt). J Ichthyol 20:153–158
Salvarina I, Koutrakis M, Leonardos I (2010) Juvenile feeding habits of Mugilidae species from estuarine systems in North Aegean Sea. Rapports et procès-verbaux des réunions commission internationale pour l’exploration scientifique. de la Mer Méditerranée 39:795
Sanchez-Velasco L (1998) Diet composition and feeding habits of fish larvae of two co-occurring species (Pisces: Callionymidae and Bothidae) in the North-western Mediterranean. ICES J Mar Sci 55:299–308
Shannon CE (1948) A mathematical theory of communication. Bell Syst Tech J 27:379–423
Swalethorp R, Malanski E, Dalgaard AM, Gissel NT, Munk P (2015) Structuring of zooplankton and fish larvae assemblages in a freshwater-influenced Greenlandic fjord: influence from hydrography and prey availability. J Plankton Res 37:102–119
Thiel R, Mehner T, Kopcke B, Kafemann R (1996) Diet Niche Relationships among early life stages of fish in German estuaries. Mar Freshw Res 47:123–136
Torricelli P, Tongiorgi P, Gandolfi G (1988) Feeding habits of mullet fry in the Arno River (Tyrrhenian coast). I. Daily feeding cycle. Ital J Zool 55:161–169
Tosi L, Torricelli P (1988) Feeding habits of mullet fry in the Arno River (Tyrrhenian coast). II. The diet. Ital J Zool 55:171–177
Turner GF (2007) Adaptive radiation of cichlid fish. Curr Biol 17:R827–R831
Ufodike EBC, Wada RK (1991) Feeding habits of tilapia, Sarotherodon Nilotica (Perciformis: Cichlidae) fry in Jos, Nigeria. Rev Biol Trop 39:189–192
Viitasalo M, Flinkman J, Viherluoto M (2001) Zooplanktivory in the Baltic Sea: a comparison of prey selectivity by Clupea harengus and Mysis mixta, with reference to prey escape reactions. Mar Ecol Prog Ser 216:191–200
Watson W, Davis RL (1989) Larval fish diets in shallow coastal waters off San Onofre. Calif Fish Bull 87:569–591
Welker MT, Pierce CL, Wahl DH (1994) Growth and survival of larval fishes: roles of competition and zooplankton abundance. Trans Am Fish Soc 123:703–717
Zaghloul K, Hasheesh W, Marie M, Zahran I (2007) Ecological and biological studies on the Nile tilapia Oreochromis Niloticus along different sites of lake Burullus. Egypt J Aquat Biol Fish 11:57–88
Funding
Open access funding provided by The Science, Technology & Innovation Funding Authority (STDF) in cooperation with The Egyptian Knowledge Bank (EKB). No funding was received for conducting this study.
Author information
Authors and Affiliations
Contributions
Authorship contributions Category 1 Conception and design of study: Nagwa E. Abdel Aziz, Wael S. El-Tohamy, Ahmad E. El-Ghobashyacquisition of data: Ahmad Alzeny, Wael S. El-Tohamyanalysis and/or interpretation of data: Wael S. El-Tohamy, Ahmad Alzeny Category 2 Drafting the manuscript: Ahmad Alzeny, Wael S. El-Tohamy, Nagwa E. Abdel Aziz, Ahmad E. El-Ghobashyrevising the manuscript critically for important intellectual content: Wael S. El-Tohamy, Ahmad Alzeny Category 3 Approval of the version of the manuscript to be published: Ahmad Alzeny, Nagwa E. Abdel Aziz, Ahmad E. El-Ghobashy, Wael S. El-Tohamy Acknowledgements All persons who have made substantial contributions to the work reported in the manuscript (e.g., technical help, writing and editing assistance, general support), but who do not meet the criteria for authorship, are named in the Acknowledgements and have given us their written permission to be named. If we have not included an Acknowledgements, then that indicates that we have not received substantial contributions from non-authors.
Corresponding author
Ethics declarations
Ethical Approval and Consent to Participate
Not applicable.
Human and Animal Ethics
Not applicable.
Consent for Publication
Not applicable.
Competing Interests
The authors declare no competing interests.
Additional information
Publisher’s Note
Springer Nature remains neutral with regard to jurisdictional claims in published maps and institutional affiliations.
Rights and permissions
Open Access This article is licensed under a Creative Commons Attribution 4.0 International License, which permits use, sharing, adaptation, distribution and reproduction in any medium or format, as long as you give appropriate credit to the original author(s) and the source, provide a link to the Creative Commons licence, and indicate if changes were made. The images or other third party material in this article are included in the article's Creative Commons licence, unless indicated otherwise in a credit line to the material. If material is not included in the article's Creative Commons licence and your intended use is not permitted by statutory regulation or exceeds the permitted use, you will need to obtain permission directly from the copyright holder. To view a copy of this licence, visit http://creativecommons.org/licenses/by/4.0/.
About this article
Cite this article
Alzeny, A., Abdel-Aziz, N.E., El-Ghobashy, A.E. et al. Diet Composition and Feeding Habits of Fish Larvae of Five Species in the Burullus Lake, Egypt. Thalassas (2024). https://doi.org/10.1007/s41208-024-00677-3
Received:
Revised:
Accepted:
Published:
DOI: https://doi.org/10.1007/s41208-024-00677-3