Abstract
Purpose
This systematic review aimed to collect published studies concerning intraoperative gamma detection of positron-emitting tracers for radioguided surgery (RGS) applications.
Methods
A systematic literature search of studies published until October 2022 was performed in Pubmed, Web Of Science, Central (Cochrane Library) and Scopus databases, including the following keywords: “Positron Emission Tomography” OR “PET” AND “Gamma” OR “γ” AND “Probe” AND “Radioguided Surgery” OR “RGS”. The included studies had to concern RGS procedures performed in at least 3 patients, regardless of the administered radiopharmaceutical and the field of application.
Results
Among to the 17 selected studies, all published between 2000 and 2022, only 2 investigations were conducted with gallium-68 (68Ga)-labeled somatostatin analogues, with fluorine-18-fluoro-2-deoxyglucose ([18F]FDG) being the most commonly used agent for RGS applications. Almost all studies were performed in oncologic patients, with only one paper also including inflammatory and infectious findings. The analysis showed that the largest part of procedures was performed through the intraoperative use of conventional gamma probes, not specifically designed for the detection of annihilation photons (n = 9), followed by PET gamma probes (n = 5) and with only three studies involving electronic collimation.
Conclusions
Regardless of the intraoperative devices, RGS with positron emitters seems to lead to significant improvements in surgeons’ ability to obtain a complete resection of tumors, even if the nature of photons resulting from positron–electron collision still remains extremely challenging and requires further technical advances.
Similar content being viewed by others
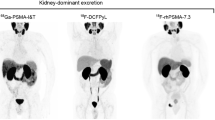
Introduction
Radioguided surgery (RGS) represents an interventional nuclear medicine procedure enabling surgeons to identify lesions at increased radiopharmaceutical concentration through the intraoperative use of radiation detectors [1]. Providing real-time information regarding the location and the extent of disease, allowing for the assessment of surgical resection margins, as well as minimizing the invasiveness of many diagnostic and therapeutic procedures, RGS has gained increasing acceptance over the years, becoming an established discipline within the practice of surgery and revolutionizing the surgical management of many malignancies, as well as the surgical approach to parathyroid disease. From simple Geiger Müller tubes [2, 3] intraoperative detection devices have consequently evolved to sophisticated and ergonomical hand-held probes, providing surgeons numerical, graphical, and acoustical feedback proportionally correlated to radiopharmaceutical concentration and suiting specific surgical applications, including laparoscopic procedures [3]. According to the type of detected radiation, the main categories of intraoperative detectors are represented by gamma probes and beta probes, the formers detecting photon radiation of gamma and X-rays, the latters detecting either positively (β+, positrons) or negatively (β−) charged electrons [4, 5]. In the last decades, there has been increased interest and growth in clinical research concerning the possible use of positron emission tomography (PET) radiopharmaceuticals for RGS applications. In particular, fluorine-18-fluoro-2-deoxyglucose ([18F]FDG) has become an extremely useful tool in oncology and has consequently opened new expectations for radical surgery, becoming the most studied positron-emitting tracer for RGS applications. Positrons emitted from proton-rich/neutron-deficient isotopes travel a short distance of several millimeters within tissues before interacting with negatively charged electrons and annihilating [6], making radio guidance purposes with PET tracers achievable with both beta and gamma probes. The 511-keV photons resulting from annihilation and emitted at a 180° angle from each other, are the basis of coincidence imaging and can be identified with intraoperative photon-sensitive probes, giving a close approximation of the location of positron emission [7]. A hand-held gamma probe for intraoperative detection of positron-emitting radionuclides was first used in 1999 for [18F]FDG radioguided surgery in 14 patients with colorectal cancer by Desai et al. [8], dating back the earliest experiences to over 20 years ago. The detection of 511-keV photons derived from positron–electron annihilation represents an important challenge for gamma detection systems and has been the focus of recent developments specifically intended for the innovative detection of higher energies. Probes are designed to detect differences in radioactivity released from tumor-bearing compared to adjacent normal tissues, providing surgeons a tumor-to-background ratio (TBR) comfortable with target localization [9,10,11]. Due to high-energy photon fluxes, making the achievement of satisfactory TBR extremely challenging, the gamma detection of positron emission for RGS purposes has not found a routine place in cancer surgery and no standard protocol has been proposed, despite the high prevalence and the cornerstone role of PET imaging in the diagnosis, staging, follow-up, surveillance and monitoring of therapies for a wide variety of malignancies [12, 13]. In this background, the literature pertaining to intraoperative gamma detection of positron-emitting isotopes results heterogeneous and the development of novel technologies is still ongoing. This systematic review aims to provide a comprehensive overview of gamma detection of positron-emitting radiopharmaceuticals for RGS applications. Particular attention was paid to the characteristics and performances of different gamma detection systems, by underlining strengths and critical issues that emerged from surgical practice.
Materials and methods
Search strategy and study selection
This systematic review was drawn up according to the Preferred Reporting Items for Systematic reviews and Meta-analyses (PRISMA) guidelines [14]. The literature research was carried out online on Pubmed, Web Of Science, Central (Cochrane Library), and Scopus databases by applying a search strategy based on the following keywords: “Positron Emission Tomography” OR “PET” AND “Gamma” OR “γ” AND “Probe” AND “Radioguided Surgery” OR “RGS”. The search included all papers published until October 2022. Reviews, book chapters, and editorials/letters were excluded. The English language was mandatory for inclusion. Eligible articles had to focus on the role of gamma probe detection of positron-emitting tracers in RGS procedures performed in humans, regardless of the administered radiopharmaceutical and the field of application. Prospective studies, feasibility studies, pilot studies, and case series with a cohort of ≥ 3 patients were included. The reference lists of suitable studies were carefully checked to identify any additional relevant literature.
Data extraction and methodological quality assessment
Data extraction was retrieved for all the selected studies and included authors, location, year of publication, type of study, indication to RGS, sample size, administered radiopharmaceutical, and outcomes. Studies with incomplete technical or clinical data were considered ineligible. The methodological quality assessment was performed using the Critical Appraisal Skills Programme (CASP). Data extraction and subsequent critical appraisal were independently performed by two reviewers and eventual disagreements and discrepancies were resolved by unanimous approval after discussion among researchers.
Results
Search results
A total of 124 articles were found and thus screened by examining each abstract in order to identify potentially suitable studies. From the overall group of 124, 24 reviews, 5 editorials/letters, 3 book chapters, 4 articles not in English language, as well as 60 articles concerning RGS procedures other than positron-emitting radionuclides were excluded. The remaining 28 studies were assessed for eligibility with the exclusion of further 19 papers (6 case reports/case series with less than three patients, 2 dosimetric studies, 3 preclinical studies, 3 retrospective analyses, 4 articles involving β + detection, 1 study with no full text available). 8 relevant manuscripts were added after examining the reference lists of suitable articles, leading to a total of 17 articles ultimately selected for the qualitative analysis of this systematic review. The detailed study selection flow-chart, along with the search strategy and the applied selection criteria, are represented in Fig. 1.
Methodological quality
The quality appraisal of selected studies is represented in Fig. 2. All studies satisfied at least 6 of the 11 domains, with 8 out of 17 studies satisfying 9 domains. 10 studies showed high risk in one or more domains. One of the major concerns with selected studies was the absence of adequate follow-up in most of them, limiting the evaluation of patients’ outcomes. Regarding patient’s selection, RGS requires accurate preoperative assessment, leading to unavoidable patient’s selection, making it not always possible to consecutively enroll subjects. It was found a high concern of applicability in evaluating the possibilities of application of obtained results, as it regards heterogeneous surgical procedures. Cumulatively, the quality appraisal resulted in quite good.
Analysis of the evidence
The 17 selected papers were published from 2000 to 2022. Most studies were conducted by authors from the USA and Europe, with only one study performed by researchers from South Korea. A major limitation of the various studies was represented by the limited number of enrolled patients ranging from 3 to 40, the latter corresponding with the prospective study by Gulec et al. [15]. 9 papers (52.9%) had a cohort < 10 subjects. As subjects were essentially cancer patients, selected studies were about RGS applications in the oncological field, except for only one study performed by Vos et al. including patients with infectious and inflammation diseases [16]. Almost all studies were performed using [18F]FDG, with a minority of investigations (n = 2) conducted with gallium-68 (68Ga)-labeled somatostatin analogues. A positive PET scan before surgery was mandatory for addressing patients with RGS in all studies. From the analysis of the selected papers, we identified 3 main categories of gamma probes for RGS applications with positron-emitting tracers: (1) conventional gamma probes (n = 9), (2) PET gamma probes (n = 5), (3) electronically collimated gamma probes (n = 3). Tables 1 and 2 report the main characteristics of included studies and gamma probes, respectively. The findings of the selected papers for each type of gamma detector are described in the following paragraphs.
Conventional gamma probes
Most published studies concerning RGS procedures with gamma probes for the detection of positron-emitting tracers have been performed through the intraoperative use of conventional gamma probes, not specifically designed for the detection of annihilation photons, including the two selected studies involving a radiopharmaceutical different from [18F]FDG.
Among this heterogeneous group, the most studied malignancy was represented by colorectal cancer, with two studies performed exclusively in this setting of patients and one study also included patients with melanoma in the cohort. In 2000, Desai and colleagues published their work involving 14 patients with primary or recurrent colorectal cancer [17]. Desai et al. reported successful results in all but one patient with recurrent mucin pseudomyxoma-producing tumor and underlined the additional detection of tumor deposits in the abdominal not visualized on preoperative PET imaging obtained in one subject. Their paper also included a preclinical evaluation of probe performances and a phantom study in peritoneal models. In 2007, Sarikaya et al. reported probe detection of 6 foci of less than 1 cm in the omentum and pelvis not seen on preoperative scan, as well as the successful identification of mucinous adenocarcinoma with both techniques, but underlined the superiority of PET imaging in the localization of liver (Fig. 3) and distant metastases [18]. On the other hand, after the in vitro analysis of the gamma probe, in 2001, Richar Essner and coworkers had successfully tested its detection capabilities in six melanoma and two colon carcinoma patients and reported how the probe managed to detect a liver metastasis visualized on ultrasound but not found by preoperative CT or PET and not palpated on inspection [19]. Conventional gamma probe performances have also been evaluated in patients with radioiodine-negative differentiated thyroid carcinoma in two different studies both performed by French Authors. In 2005, Kraeber-Bodéré and colleagues demonstrated the successful identification of all lesions reported on preoperative imaging in all ten enrolled patients but reported how five patients had additional microscopic lymph node metastases with low uptake missed by both preoperative imaging and gamma probe [20]. Similar findings were subsequently reported by Curtet et al., in a comparative study performed with two different conventional gamma probes, one with a bismuth germanate (BGO) crystal, and the other with a thallium-activated caesium iodide (CsI(Tl)) scintillating crystal, both previously tested in vitro and both failing in detecting small additional lesions revealed through histopathologic examination [21]. In 2006, Nwogu and coworkers tested the capability of a conventional gamma probe in identifying metastatic lymph nodes in ten patients with Non-Small Cell Lung Cancer (NSCLC) [22]. In particular, they focused on the role of RGS in detecting micrometastases, thus resulting in upstaging of patients. Three out of five positive findings were negative at routine H&E but resulted positive after ultrastaging, demonstrating the capability of the gamma probe in identifying micrometastasis. However, Nwogu et al. reported three false-positive and two false-negative results, due to inflammation and proximity to the primary tumor, respectively. A case series of three recurrent ovarian cancer patients was published in 2008 by Cohn et al. reported the detection of additional retroperitoneal metastasis obtained with a conventional device in one patient [23]. Two studies were performed in patients with gastroenteropancreatic neuroendocrine tumors (GEP-NETs) using 68Ga-labeled somatostatin analogs. One of the main concerns in this setting of patients is represented by recurrent laparotomies leading to multiple adhesions and altered anatomy, which make extremely challenging the localization of malignant tissues and thus RGS particularly useful. In 2011, Kaemmerer et al. published a pilot study involving nine patients with primary or recurrent GEP-NETs using either [68Ga]Ga-DOTANOC and 68Ga-DOTATATE [24]. Subsequently, Sadowski and colleagues tested the performances of a conventional gamma probe in detecting [68Ga]Ga-DOTATATE avid lesions in a cohort of 14 patients with GEP-NETs [25].
PET gamma probes
Among the overall group of selected studies performed with PET gamma probes, two papers involved patients with heterogeneous malignancies. This category included the prospective study published in 2006 by Gulec et al. and involving 40 patients. The authors, after an accurate in vitro analysis of both sensitivity and spatial resolution, reported the successful identification of all [18F]FDG-PET-positive lesions, along with the detection of additional retroperitoneal foci and demonstrated the usefulness of the device in the re-exploration of the surgical bed after excision [15]. In 2009, Molinaa et al. confirmed the utility of using a PET gamma probe for navigating into scar tissue and for the confirmation of complete disease removal [26]. In 2010, Kim et al. published a pilot study involving 12 patients with differentiated thyroid cancer undergoing RGS with a PET gamma probe. The intraoperative device allowed the detection of all lesions demonstrated by preoperative PET, of additional sites in some patients, and a non-palpable, metastatic lymph node in the deep superior mediastinum not revealed by both PET and neck ultrasonography, after re-exploration of the operative bed [27]. Three patients with breast cancer were submitted to RGS with a PET gamma probe for both localization of primary tumors and evaluation of lymph node metastases by Orsaria and coworkers in a case series published in 2017 [28]. After a same-day PET scan performed before surgery, RGS confirmed preoperative findings in one patient, and localized additional nodal disease in another case, but showed low accuracy in the identification of nodal micrometastasis in the remaining patient. A PET gamma probe specifically designed for the detection of 511-keV photons released from the decay of 18F (Fig. 4) has been recently chosen by Rinehardt and colleagues in a prospective study involving pediatric patients with different cancers and published in 2022 [29]. As thoracic phantom models revealed an extremely low TBR, the PET gamma probe was not used for intraoperative navigation, but only for an external survey ex vivo.
Gamma probes with electronic collimation
In 2010, de Jong and colleagues reported their experience of RGS in three patients with retroperitoneal testicular tumor recurrences [30]. The major concern in this setting of patients is represented by the presence of extensive scar tissue derived from previous surgery and often located in strict proximity to vital structures, making extremely difficult the discrimination between tumor and fibrosis, as well as surgeon’s task. In one out of the three patients, the detector allowed for the localization and subsequent resection of an additional tumor with respect to preoperative imaging.
Subsequently, the use of an electronically collimated device enabled Vos and coworkers to identify and excise all suspicious clinically occult [18F]FDG accumulating lesions in nine consecutive patients with oncological, inflammatory, and infectious diseases [16]. A manuscript published in 2011 by García et al. focused on the role of preoperative [18F]FDG-PET imaging in selecting the better RGS procedure and concluded how in their cohort of patients positron-emitting radioguidance with an electronically collimated gamma probe, represented a valuable tool in case of multiple lesions not easily accessible for radioguided occult lesion localization (ROLL) [31].
Discussion
Surgical resection represents the only curative treatment option for many patients with different malignancies. Unfortunately, a significant number of subjects may have undetected preoperative disease or residual low-volume tumor foci after surgery. Both of these conditions significantly affect complete tumor removal and thus prognosis. In this scenario, RGS offers the possibility of guiding the surgeon to the specific site of disease for targeted excision, enables the verification of complete removal by checking both resection margins and surgical bed, permits ex vivo assessment of disease eradication, and may allow the identification of additional foci not detected on preoperative imaging. Sentinel lymph node biopsy [32], radioguided occult lesion localization (ROLL) [33], minimally invasive radioguided parathyroidectomy (MIRP) [34], detection of neuroendocrine tumors [35], localization of neuroblastomas and pheochromocytomas [36, 37], radioguided seed localization (RSL) and radioimmunoguided surgery (RIGS) procedures [17, 38], represent the most common RGS applications with low and medium-energy gamma-emitting radionuclides. Since the last decades, PET imaging has played a key role in the management of different kinds of malignancies and has continuously evolved following the development of more sensitive detection systems, as well as the evolution of computerized image analysis and the diffusion of new radiopharmaceuticals. Among PET tracers, [18F]FDG represents the most effective imaging agent [39,40,41] having the advantage of being trapped inside malignant cancer cells, thus enhancing its use for tumor localization [42]. The uptake of [18F]FDG in most tumor tissues is based on the increased glycolytic rate. After entering tumor cells through glucose transporter-1 and 3 (GLUT 1 and GLUT 3) and undergoing phosphorylation to [18F]FDG-6-phosphate by hexokinase, [18F]FDG-6-phosphate accumulates due to slow dephosphorylation and to the fact that it cannot be utilized in the metabolic steps of glycolysis. 18F molecule undergoes radioactive decay with slow release of β + particles (short range of 0.5–1.0 cm) and gamma rays which can travel several centimeters through tissue [43]. Different studies have demonstrated the superior sensitivity and specificity of [18F]FDG-PET imaging in comparison with other conventional techniques [44,45,46], making the possibility of extending its applications to RGS extremely appealing. Intraoperative radioguided resection of all [18F]FDG-positive tissue may ensure for more complete removal of the tumor burden as compared to the surgeons’ visual and hands-on approach of assessing and resecting presumed sites of tumor. In addition, intraoperative detection might help overcome the limitation of current generation PET systems in detecting small-volume disease [47]. The capability of a gamma probe to detect a lesion depends on several factors including the tumor avidity, the time from injection to probe survey, the clearance kinetics, the location of the lesion in particular in case of proximity to sites of physiologic uptake, and the technical characteristics of the probe, all ultimately determining surgery results.
The first experiences of RGS with positron emitters date back to 20 years ago. To our knowledge, the most consistent RGS experience has been reported by Povoski et al. in a single-institution retrospective review involving 145 patients submitted to a multimodal imaging and detection approach to [18F]FDG directed surgery for known or suspected malignancies [48]. This work involves the largest cohort of patients until now and thus deserves to be mentioned even if not included in this systematic review due to the lack of specific technical data concerning intraoperative detection devices. Conventional gamma probes have been used for intraoperative detection of annihilation photons, but their performance remains below expectations. The major concern with applying currently available gamma probes for intraoperative localization of positron-emitting tracers is that these devices are not designed for the detection of the high-energy gamma rays derived from positron–electron annihilation (511-keV) but of low or medium-energy gamma rays such as 140–142 keV of technetium-99 m (99mTc), 171 and 247 keV of indium-111 (111In), 159 keV of iodine-123 (123I), and 35 keV of iodine-125 (125I). The greatest obstacle to the use of these devices is theoretically represented by the rapid decay of the 511-keV photons to lower energy species which produces an artifact decreasing the directionality of probes. However, most published studies have demonstrated the usefulness of conventional surgical gamma-ray-sensitive probes for detecting the emission of the decay process of positron emitters, showing capabilities of detecting differences in radioactivity released from tumors and adjacent normal background in most cases and thus identifying most tumors demonstrated by preoperative PET. Moreover, in some papers, the use of conventional devices allowed for the identification of additional lesions, not revealed in preoperative PET scans. Nevertheless, some limitations have emerged from these studies, partially correlated with [18F]FDG properties, and partially to the characteristics of conventional gamma probes. A major limitation of [18F]FDG-PET imaging is represented by the limited sensitivity for the detection of tumors showing a low metabolic activity [49]. As previously reported, Desai et al. failed in the identification of a recurrent mucin pseudomyxoma-producing tumor that is relatively acellular and presents few cells incorporating and metabolizing the [18F]FDG [17]. This tumor had been previously detected on PET imaging, but probably it changed from a cellular form to a mucinous debris one during the time interval between imaging and surgery. Such eventuality, may put the attention on the opportunity of performing preoperative imaging on the same day of surgery or, if not possible, to perform an additional acquisition of the surgical region immediately prior, as performed by Kaemmerer et al. [24]. Sarikaya and coworkers managed the identification of mucinous tumors with low uptake and previously visualized on preoperative PET scan, underlining how the surgeon's ability to position the intraoperative conventional gamma probe in close proximity to sites of suspected tumor recurrence, may ultimately make intraoperative detection more efficient, particularly in such cases [18]. In this regard, it is worth considering that the main advantage of intraoperative gamma probes over preoperative PET imaging is the ability to have the device in close proximity to the suspected site of disease, as demonstrated by Barber et al., who showed that a sodium iodide-based scintillation probe placed within 1 cm of the tumor was more sensitive in detecting small, deep lesions than a gamma camera [50]. Such considerations might explain the detection of a liver metastasis visualized on ultrasound but not found on preoperative imaging and not palpated on inspection, successfully performed by Essner et al. even in presence of high physiologic liver background [19]. Also of note is the fact that [18F]FDG is not cancer-specific, and resultant physiological uptake into benign tissue processes, such as infection and inflammation, may lead to false-positive findings, as shown by Nwogu et al. [22]. The authors also reported the difficulties in differentiating [18F]FDG activity signals of the primary tumor from those of peritumoral lymph nodes, thus leading to false-negative results and consequent understaging, eventually preventing patients from receiving adjuvant therapy. In both studies performed in patients with radioiodine-negative differentiated thyroid carcinoma, additional micrometastases not detected by both PET imaging and intraoperative conventional gamma probes were confirmed after histopathologic examination. Both papers underlined, however, the utility of conventional gamma probes in verifying the complete surgical resection of all detected foci [20, 21]. To further assess the surgical removal of known involved tissues, in their case series of three recurrent ovarian cancer patients, Cohn et al. performed a 10-min PET/CT scan of resected specimens and processed and reviewed images for the presence of hypermetabolic foci, prospecting the usefulness of immediate postoperative patient PET imaging to confirm the absence of residual metabolic foci after resection [23]. As reported by Curtet and colleagues, the spatial resolution obtained with conventional gamma probes when detecting positron emitters is significantly much lower than reported in other studies with lower energy emitters, making it impossible to distinguish two foci located close to one another, specifically less than 2 cm with the conventional gamma probes compared in their study, thus potentially representing a dramatic drawback [5]. One of the major concerns with conventional gamma probes is represented by the achievement of satisfactory TBR, since they have small detectors, that detect only a fraction of 511-keV photons, and are equipped with side and back shieldings not designed to stop 511-keV, making them sensitive to radiation from both adjacent tissues and distant organs with high physiological activity. A minimum TBR of 1.5-to-1 has been suggested to make surgeons comfortable with targeted tissue localization [11, 15, 51]. However, different authors have underlined how it represents an arbitrary and fixed ratio potentially affected by several factors, including tumor tracer uptake, background activity, and type of detection probe system used for making counts per second measurements. Such considerations have lead Kraeber-Bodéré et al. to consider positive a TBR higher than 1.3 in their study for the detection of thyroid carcinoma metastases with a conventional gamma sensitive probe, owing to the high vascular background activity 1–2 h after [18F]FDG injection [20]. In an attempt to overcome the possible limitations of this fixed ratiometric threshold method, the three-sigma statistical threshold criteria for gamma probe positivity, previously used for RIGS by Thurston and Burak [52,53,54], have been recently suggested by Chapman et al. to improve intraoperative in situ identification of [18F]FDG-avid sites [55]. According to this method, all examined areas with counts greater than 3 standard deviations above background counts should be considered abnormal tissue and excised. In a recent paper, Povoski et al. [52] reported the example of a gamma detection probe prototype that can greatly benefit from the three-sigma statistical threshold criteria, the K-alpha probe [56], a device that detects secondary, lower energy gamma emissions resulting when a thin metal foil plate, typically lead, is placed between a cadmium-zinc-telluride crystal and a source of gamma emission, such as [18F]FDG. The three-sigma statistical threshold criteria have also been chosen by Sarikaya and colleagues in their RGS study for the identification of FDG-avid tissues in colorectal cancer patients with a conventional gamma probe [18]. Recently, there has been the appearance of hand-held gamma probes specifically intended for attempting to detect 511-keV photons, generally referred to as PET probes. In their study performed in patients with heterogeneous malignancies, in largest part melanomas, Gulec and colleagues managed to disclose target lesions in 11 patients with negative initial surgical exploration [15], and detect additional retroperitoneal lesions not seen in preoperative PET study through the use of a PET gamma probe. In accordance with most of the literature data, an in situ TBR of 1.5:1 or greater was considered a positive probe-detection criterion. The researchers separately reported the mean in situ TBR for melanoma, colon cancer, lymphoma, and breast cancer lesions as an individual type of tumor may present different [18F]FDG uptake. In 2009, Molina et al. performed an RGS study involving nine patients with different malignancies by successfully using a PET gamma probe to localize and remove [18F]FDG-avid lesions in the head and neck, chest, abdomen, and retroperitoneum [26]. Their results have been confirmed after adequate follow-up. Despite the use of a dedicated PET gamma probe, Kim et al. [27] were not able to establish a precise, meaningful cutoff of TBR and considered a value greater than 1.3 the positive threshold for differentiated thyroid cancer RGS, according to the previous work performed by Kraeber-Bodéré et al. with a conventional device [20]. Kraeber-Bodéré and colleagues also explained the necessity of correctly positioning the PET gamma probe perpendicularly on the suspected lesion to avoid TBR to decrease, thus leading to regrettable false-negative results. The problem of false-positive findings also represents a not negligible drawback in their setting of patients during evaluation of level II lymph nodes which are located close to the submandibular gland and pharynx, having a physiologically high [18F]FDG uptake. In their case series of three patients with breast cancer, Orsaria et al. [28] confirmed low accuracy in the detection of micrometastasis, in line with findings reported with conventional gamma probes [20, 22]. In a recent work, Rinehardt and colleagues reported a mean TBR of 1.07 in their thoracic phantom models, indicating an inability of the PET gamma probe to localize simulated lesions [29]. As in absence of a control arm, the preclinical phantom model was critical for probe validation, in their cohort of eight pediatric patients with primary, recurrent or metastatic cancers, the PET gamma probe was not used for intraoperative navigation, but only for an external survey ex vivo. It is clear that achieving satisfactory TBR remains a major concern, even with PET gamma probes specifically designed for the detection of annihilation photons. Attempts at improving current probe performances by increasing collimation to provide better spatial resolution and by creating crystal geometry of sufficient diameter and thickness to capture a higher percentage of photons would result in cumbersome devices prohibitively large, heavy, and expensive [3, 52, 56]. To overcome these physical barriers, engineering efforts have moved toward alternative directions. Innovative devices with active electronic collimation have been successfully applied to intraoperative hand-held gamma probe designs. Such devices are represented by multi-detector systems able to focus on the target through the parametrization of the count rates of multiple scintillation crystals, not requiring mechanical collimation. In particular, the central crystal preferentially detects the activity of the lesion, while the concentric detector ring principally detects background activity. Thus, the electronic collimation locates the target through special algorithms. An innovative prototype based on these features and designed to overcome the limitations of passive collimators has been developed by the team of Sapienza (Fig. 5) [57] and recently tested for MIRP with positive results [58]. The same team also holds the latest Italian patent for a scintillation probe with active collimator specifically intended for laparoscopic applications (Italian patent application n102021000023963 PCT/IB2022/058698), positively evaluated at European level. In the field of RGS with positron emitters, Vos et al. in 2016 successfully used a multi-detector probe with 5 scintillation crystals to allow a definitive histopathological diagnosis in both oncologic patients and a subject with clinical signs of infection [16]. Previously, in a case series of three patients with retroperitoneal testicular tumor recurrences, De Jong and colleagues had, however, underlined how, despite electronic collimation outperforming traditional mechanical collimated probes, improving surgical resection margins in fibrotic areas remains difficult [30]. Similarly, Garcia et al. in a previous study on phantoms revealed how background interference continues to be the principal disadvantage even with these devices, and did not manage to intraoperatively locate all of the metabolically active lesions seen on PET scan [31]. Garcia and coworkers underlined the limitations of using a fixed TBR not accounting for both the specific study region and the depth of lesions, and put their attention on the timing between injection and intervention. As the metabolism of the [18F]FDG is different between normal tissues and tumors, with the latter presenting greater entrapment, TBR increases with time. However, to have a sufficient number of counts, the time window is 3–4 h in case of injection of 370 MBq, given the 110 min mean half-life time of 18F. The timing of tracer injection relative to surgical access of the target lesion is especially important in the setting of reoperations, as lysis of adhesions can take a long time before arriving at the target and may be particularly critical when using radionuclides having a half-life significantly shorter than 18F. Such considerations have been reported by Sadowski and colleagues in their study performed on patients with GEP-NETs using a conventional gamma probe for the intraoperative detection of 68Ga-labeled somatostatin analogs, due to the 68 min half-life of [68Ga]Ga-DOTA peptides. Sadowski et al. reported high correct identification of gastric and small bowel neuroendocrine tumors, including mesenteric lymph nodes, but found a lower detection rate for primary pancreatic lesions and peripancreatic lymph nodes, as well as liver metastases [25]. Similarly, Kaemmerer and coworkers, successfully identified small lesions of 0.5 cm and more tumor foci as compared to both preoperative PET imaging and surgical palpation, using a conventional gamma probe for radioguidance [24]. Both studies underlined the usefulness of RGS in patients GEP-NETs presenting with scars and fibrosis from previous surgery but recognized some limitations in the detection capability due to high physiologic retention of radiopharmaceutical in the liver, kidney, spleen, and pancreas. At present, most literature data concerning RGS with positron emitters, regard procedures involving [18F]FDG, with only a limited experience with 68Ga-labeled somatostatin analogues. However, as PET with 68Ga-labeled somatostatin analogs has shown to be more accurate than other agents for detecting GEP-NETs and has gained an important role in the clinical management of GEP-NETs patients [59, 60], the possibility of using this radiotracer for RGS is extremely promising. Similarly, RGS procedures based on [18F]Fluorodihydroxyphenylalanine ([18F]FDOPA) uptake could represent an additional weapon against medullary thyroid carcinoma recurrences, as reported by Evangelista et al. [61] and subsequently shown in a case report by López-Gómez et al. [62]. We could assume that improved tissue specificity by novel radiolocalizing agents could provide highly specific intraoperative guidance. Overall, published studies demonstrated a consistent performance of intraoperative gamma probe detection over broadly dispersed tumor histologies, variable anatomic locations, including cervical, intra-abdominal, and intra-thoracic operations, different settings of patients, from reoperations to pediatric subjects, and different intraoperative gamma detectors, from conventional devices, to PET gamma probes and electronically collimated prototypes.
Conclusion
Combining PET imaging with intraoperative radioguided approaches to detect positron-emitting radiopharmaceuticals should lead to significant improvements in surgeons’ ability to obtain a complete resection of primary, recurrent or residual tumors. However, due to the nature of photons resulting from a positron–electron collision, acquiring a focused signal with gamma probes still remains extremely challenging and presents several critical issues. Despite the encouraging and favorable results, published studies have not provided sufficiently optimal evidence and RGS based on positron-emitting tracers has not gained a widespread use, being performed in only a scarcity of centers throughout the world. Changing PET tracers with gamma-emitting radionuclides [63] suitable for intraoperative radioguidance through low or medium-energy gamma probes represents a viable alternative option to correctly harvest pathologic tissue.
In the upcoming future, conducting further studies in larger cohorts, randomizing patients to operations with and without RGS, as well as performing long-term follow-up, could definitively determine the true value of gamma probe detection of positron emitters. Moreover, as advances in medicine are strictly related to advances in technology, technical improvements might determine if RGS with positron-emitting tracers will gain a routine established role in surgical practice.
Data availability
Not applicable.
Abbreviations
- RGS:
-
Radioguided surgery
- PET:
-
Positron emission tomography
- 18F:
-
Fluorine-18
- FDG:
-
Fluoro-2-deoxyglucose
- TBR:
-
Tumor-to-background ratio
- PRISMA:
-
Preferred Reporting Items for Systematic reviews and Meta-analyses
- CASP:
-
Critical Appraisal Skills Programme
- 68Ga:
-
Gallium-68
- ROLL:
-
Radioguided occult lesion localisation
- GLUT 1 and GLUT 3:
-
Glucose transporter-1 and 3
- 99mTc:
-
Technetium-99m
- 111In:
-
Indium-111
- 123I:
-
Iodine-123
- 125I:
-
Iodine-125
- GEP-NETs:
-
Gastroenteropancreatic neuroendocrine tumors
- FDOPA:
-
Fluorodihydroxyphenylalanine
References
Rietbergen DD, Vano MN, Kleinjan GH, Brouwer OR, Valdes-Olmos RA, Vanl FW, Buckle T (2021) Interventional nuclear medicine: a focus on radioguided intervention and surgery. Q J Nucl Med Mol Imaging 65(1):4–19. https://doi.org/10.23736/s1824-4785.21.03286-6
Selverstone B, Sweet WH, Robinson CV (1949) The clinical use of radioactive phosphorus in the surgery of brain tumors. Ann Surg 130(4):643–651
Povoski SP, Neff RL, Mojzisik CM, O’Malley DM, Hinkle GH, Hall NC, Murrey DA Jr, Knopp MV, Martin EW Jr (2009) A comprehensive overview of radioguided surgery using gamma detection probe technology. World J Surg Oncol 7:11. https://doi.org/10.1186/1477-7819-7-11
Hoffman EJ, Tornai MP, Janecek M, Patt BE, Iwanczyk JS (1999) Intraoperative probes and imaging probes. Eur J Nucl Med 26(8):913–935. https://doi.org/10.1007/s002590050468
Zanzonico P, Heller S (2000) The intraoperative gamma probe: basic principles and choices available. Semin Nucl Med 30(1):33–48. https://doi.org/10.1016/s0001-2998(00)80060-4
Bai B, Ruangma A, Laforest R, Tai YC, Leahy RM (2003) Positron range modeling for statistical PET image reconstruction. In: 2003 IEEE Nuclear Science Symposium. Conference Record (IEEE Cat. No.03CH37515), 19–25 Oct. 2003, vol 2504, pp 2501–2505. https://doi.org/10.1109/NSSMIC.2003.1352400
Kwo DP, Barber HB, Barrett HH, Hickernell TS, Woolfenden JM (1991) Comparison of NaI(T1), CdTe, and HgI2 surgical probes: effect of scatter compensation on probe performance. Med Phys 18(3):382–389. https://doi.org/10.1118/1.596683
Desai D, Arnold M, Saha S, Hinkle G, Soble D, Frye J, DePalatis L, Mantil J, Satter M, Martin E (1999) Intraoperative gamma detection of FDG distribution in colorectal cancer. Clin Positron Imaging 2(6):325. https://doi.org/10.1016/s1095-0397(99)00085-0
Kuhn JA, Corbisiero RM, Buras RR, Carroll RG, Wagman LD, Wilson LA, Yamauchi D, Smith MM, Kondo R, Beatty JD (1991) Intraoperative gamma detection probe with presurgical antibody imaging in colon cancer. Arch Surg 126(11):1398–1403. https://doi.org/10.1001/archsurg.1991.01410350092013
Bertsch DJ, Burak WE Jr, Young DC, Arnold MW, Martin EW Jr (1996) Radioimmunoguided surgery for colorectal cancer. Ann Surg Oncol 3(3):310–316. https://doi.org/10.1007/bf02306288
Gulec SA, Hoenie E, Hostetter R, Schwartzentruber D (2007) PET probe-guided surgery: applications and clinical protocol. World J Surg Oncol 5:65. https://doi.org/10.1186/1477-7819-5-65
Maldonado A, González-Alenda FJ, Alonso M, Sierra JM (2007) PET-CT in clinical oncology. Clin Transl Oncol 9(8):494–505. https://doi.org/10.1007/s12094-007-0093-5
Poeppel TD, Krause BJ, Heusner TA, Boy C, Bockisch A, Antoch G (2009) PET/CT for the staging and follow-up of patients with malignancies. Eur J Radiol 70(3):382–392. https://doi.org/10.1016/j.ejrad.2009.03.051
Liberati A, Altman DG, Tetzlaff J, Mulrow C, Gøtzsche PC, Ioannidis JP, Clarke M, Devereaux PJ, Kleijnen J, Moher D (2009) The PRISMA statement for reporting systematic reviews and meta-analyses of studies that evaluate health care interventions: explanation and elaboration. PLoS Med 6(7):e1000100. https://doi.org/10.1371/journal.pmed.1000100
Gulec SA, Daghighian F, Essner R (2016) PET-probe: evaluation of technical performance and clinical utility of a handheld high-energy gamma probe in oncologic surgery. Ann Surg Oncol 23(Suppl 5):9020–9027. https://doi.org/10.1245/aso.2006.05.047
Vos CG, Hartemink KJ, Muller S, Oosterhuis JW, Meijer S, van den Tol MP, Comans EF (2012) Clinical applications of FDG-probe guided surgery. Acta Chir Belg 112(6):414–418. https://doi.org/10.1080/00015458.2012.11680864
Desai DC, Arnold M, Saha S, Hinkle G, Soble D, Fry J, DePalatis LR, Mantil J, Satter M, Martin EW (2000) Correlative whole-body FDG-PET and intraoperative gamma detection of FDG distribution in colorectal cancer. Clin Positron Imaging 3(5):189–196. https://doi.org/10.1016/s1095-0397(00)00052-2
Sarikaya I, Povoski SP, Al-Saif OH, Kocak E, Bloomston M, Marsh S, Cao Z, Murrey DA, Zhang J, Hall NC, Knopp MV, Martin EW Jr (2007) Combined use of preoperative 18F FDG-PET imaging and intraoperative gamma probe detection for accurate assessment of tumor recurrence in patients with colorectal cancer. World J Surg Oncol 5:80. https://doi.org/10.1186/1477-7819-5-80
Essner R, Hsueh EC, Haigh PI, Glass EC, Huynh Y, Daghighian F (2001) Application of an [(18)F]fluorodeoxyglucose-sensitive probe for the intraoperative detection of malignancy. J Surg Res 96(1):120–126. https://doi.org/10.1006/jsre.2000.6069
Kraeber-Bodéré F, Cariou B, Curtet C, Bridji B, Rousseau C, Dravet F, Charbonnel B, Carnaille B, Le Néel JC, Mirallié E (2005) Feasibility and benefit of fluorine 18-fluoro-2-deoxyglucose-guided surgery in the management of radioiodine-negative differentiated thyroid carcinoma metastases. Surgery 138(6):1176–1182. https://doi.org/10.1016/j.surg.2005.08.028. (discussion 1182)
Curtet C, Carlier T, Mirallié E, Bodet-Milin C, Rousseau C, Barbet J, Kraeber-Bodéré F (2007) Prospective comparison of two gamma probes for intraoperative detection of 18F-FDG: in vitro assessment and clinical evaluation in differentiated thyroid cancer patients with iodine-negative recurrence. Eur J Nucl Med Mol Imaging 34(10):1556–1562. https://doi.org/10.1007/s00259-007-0393-6
Nwogu C, Fischer G, Tan D, Glinianski M, Lamonica D, Demmy T (2006) Radioguided detection of lymph node metastasis in non-small cell lung cancer. Ann Thorac Surg 82(5):1815–1820. https://doi.org/10.1016/j.athoracsur.2006.05.104. (discussion 1820)
Cohn DE, Hall NC, Povoski SP, Seamon LG, Farrar WB, Martin EW Jr (2008) Novel perioperative imaging with 18F-FDG PET/CT and intraoperative 18F-FDG detection using a handheld gamma probe in recurrent ovarian cancer. Gynecol Oncol 110(2):152–157. https://doi.org/10.1016/j.ygyno.2008.04.026
Kaemmerer D, Prasad V, Daffner W, Haugvik SP, Senftleben S, Baum RP, Hommann M (2012) Radioguided surgery in neuroendocrine tumors using Ga-68-labeled somatostatin analogs: a pilot study. Clin Nucl Med 37(2):142–147. https://doi.org/10.1097/RLU.0b013e3182291de8
Sadowski SM, Millo C, Neychev V, Aufforth R, Keutgen X, Glanville J, Alimchandani M, Nilubol N, Herscovitch P, Quezado M, Kebebew E (2015) Feasibility of radio-guided surgery with 68Gallium-DOTATATE in patients with gastro-entero-pancreatic neuroendocrine tumors. Ann Surg Oncol 22(Suppl 3):S676-682. https://doi.org/10.1245/s10434-015-4857-9
Molina MA, Goodwin WJ, Moffat FL, Serafini AN, Sfakianakis GN, Avisar E (2009) Intra-operative use of PET probe for localization of FDG avid lesions. Cancer Imaging 9(1):59–62. https://doi.org/10.1102/1470-7330.2009.0009
Kim WW, Kim JS, Hur SM, Kim SH, Lee SK, Choi JH, Kim S, Choi JY, Lee JE, Kim JH, Nam SJ, Yang JH, Choe JH (2011) Radioguided surgery using an intraoperative PET probe for tumor localization and verification of complete resection in differentiated thyroid cancer: a pilot study. Surgery 149(3):416–424. https://doi.org/10.1016/j.surg.2010.08.004
Orsaria P, Chiaravalloti A, Fiorentini A, Pistolese C, Vanni G, Granai AV, Varvaras D, Danieli R, Schillaci O, Petrella G, Buonomo OC (2017) PET probe-guided surgery in patients with breast cancer: proposal for a methodological approach. In Vivo 31(1):101–110. https://doi.org/10.21873/invivo.11031
Rinehardt HN, Longo S, Gilbert R, Shoaf JN, Edwards WB, Kohanbash G, Malek MM (2022) Handheld PET probe for pediatric cancer surgery. Cancers (Basel). https://doi.org/10.3390/cancers14092221
de Jong JS, van Ginkel RJ, Slart RH, Lemstra CL, Paans AM, Mulder NH, Hoekstra HJ (2010) FDG-PET probe-guided surgery for recurrent retroperitoneal testicular tumor recurrences. Eur J Surg Oncol 36(11):1092–1095. https://doi.org/10.1016/j.ejso.2010.08.129
García JR, Fraile M, Soler M, Bechini J, Ayuso JR, Lomeña F (2011) PET/CT-guided salvage surgery protocol. Results with ROLL technique and PET probe. Rev Esp Med Nucl 30(4):217–222. https://doi.org/10.1016/j.remn.2011.02.011
Alex JC, Krag DN (1993) Gamma-probe guided localization of lymph nodes. Surg Oncol 2(3):137–143. https://doi.org/10.1016/0960-7404(93)90001-f
Bowles H, Sánchez N, Tapias A, Paredes P, Campos F, Bluemel C, Valdés Olmos RA, Vidal-Sicart S (2017) Radioguided surgery and the GOSTT concept: From pre-operative image and intraoperative navigation to image-assisted excision. Rev Esp Med Nucl Imagen Mol 36(3):175–184. https://doi.org/10.1016/j.remn.2016.09.004
Norman J, Chheda H (1997) Minimally invasive parathyroidectomy facilitated by intraoperative nuclear mapping. Surgery 122(6):998–1003. https://doi.org/10.1016/s0039-6060(97)90201-4. (discussion 1003–1004)
Ahlman H, Tisell LE, Wängberg B, Nilsson O, Fjälling M, Forssell-Aronsson E (1994) Somatostatin receptors on neuroendocrine tumors—a way to intraoperative diagnosis and localization. Yale J Biol Med 67(3–4):215–221
Filippi L, Valentini FB, Gossetti B, Gossetti F, De Vincentis G, Scopinaro F, Massa R (2005) Intraoperative gamma probe detection of head and neck paragangliomas with 111In-pentetreotide: a pilot study. Tumori 91(2):173–176. https://doi.org/10.1177/030089160509100213
Ricard M, Tenenbaum F, Schlumberger M, Travagli JP, Lumbroso J, Revillon Y, Parmentier C (1993) Intraoperative detection of pheochromocytoma with iodine-125 labelled meta-iodobenzylguanidine: a feasibility study. Eur J Nucl Med 20(5):426–430. https://doi.org/10.1007/bf00209002
Pouw B, de Wit-van der Veen LJ, Stokkel MP, Loo CE, Vrancken Peeters MJ, Valdés Olmos RA (2015) Heading toward radioactive seed localization in non-palpable breast cancer surgery? A meta-analysis. J Surg Oncol 111(2):185–191. https://doi.org/10.1002/jso.23785
Kole AC, Pruim J, Nieweg OE, van Ginkel RJ, Hoekstra HJ, Schraffordt Koops H, Vaalburg W (1997) PET with L-[1-carbon-11]-tyrosine to visualize tumors and measure protein synthesis rates. J Nucl Med 38(2):191–195
Inoue T, Kim EE, Wong FC, Yang DJ, Bassa P, Wong WH, Korkmaz M, Tansey W, Hicks K, Podoloff DA (1996) Comparison of fluorine-18-fluorodeoxyglucose and carbon-11-methionine PET in detection of malignant tumors. J Nucl Med 37(9):1472–1476
Higashi K, Clavo AC, Wahl RL (1993) Does FDG uptake measure proliferative activity of human cancer cells? In vitro comparison with DNA flow cytometry and tritiated thymidine uptake. J Nucl Med 34(3):414–419
Checkley S (1986) The brain, biochemistry and behavior. Proceedings of the Sixth Arnold O Beckman Conference in Clinical Chemistry. J Neurol Neurosurg Psychiatry 49(7):848. https://doi.org/10.1136/jnnp.49.7.848-a
Wahl RL, Hutchins GD, Buchsbaum DJ, Liebert M, Grossman HB, Fisher S (1991) 18F-2-deoxy-2-fluoro-D-glucose uptake into human tumor xenografts. Feasibility studies for cancer imaging with positron-emission tomography. Cancer 67 (6):1544–1550. doi:https://doi.org/10.1002/1097-0142(19910315)67:6<1544::aid-cncr2820670614>3.0.co;2-0
Valk PE, Abella-Columna E, Haseman MK, Pounds TR, Tesar RD, Myers RW, Greiss HB, Hofer GA (1999) Whole-body PET imaging with [18F]fluorodeoxyglucose in management of recurrent colorectal cancer. Arch Surg 134(5):503–511. https://doi.org/10.1001/archsurg.134.5.503. (discussion 511-503)
Gritters LS, Francis IR, Zasadny KR, Wahl RL (1993) Initial assessment of positron emission tomography using 2-fluorine-18-fluoro-2-deoxy-D-glucose in the imaging of malignant melanoma. J Nucl Med 34(9):1420–1427
Damian DL, Fulham MJ, Thompson E, Thompson JF (1996) Positron emission tomography in the detection and management of metastatic melanoma. Melanoma Res 6(4):325–329. https://doi.org/10.1097/00008390-199608000-00008
Yamamoto S, Higashi T, Matsumoto K, Senda M (2006) Development of a positron-imaging detector with background rejection capability. Ann Nucl Med 20(10):655–662. https://doi.org/10.1007/bf02984676
Povoski SP, Hall NC, Murrey DA Jr, Chow AZ, Gaglani JR, Bahnson EE, Mojzisik CM, Kuhrt MP, Hitchcock CL, Knopp MV, Martin EW Jr (2011) Multimodal imaging and detection approach to 18F-FDG-directed surgery for patients with known or suspected malignancies: a comprehensive description of the specific methodology utilized in a single-institution cumulative retrospective experience. World J Surg Oncol 9:152. https://doi.org/10.1186/1477-7819-9-152
Whiteford MH, Whiteford HM, Yee LF, Ogunbiyi OA, Dehdashti F, Siegel BA, Birnbaum EH, Fleshman JW, Kodner IJ, Read TE (2000) Usefulness of FDG-PET scan in the assessment of suspected metastatic or recurrent adenocarcinoma of the colon and rectum. Dis Colon Rectum 43(6):759–767. https://doi.org/10.1007/bf02238010. (discussion 767–770)
Barber HB, Barrett HH, Woolfenden JM, Myers KJ, Hickernell TS (1989) Comparison of in vivo scintillation probes and gamma cameras for detection of small, deep tumours. Phys Med Biol 34(6):727–739. https://doi.org/10.1088/0031-9155/34/6/008
Gulec SA (2007) PET probe-guided surgery. J Surg Oncol 96(4):353–357. https://doi.org/10.1002/jso.20862
Povoski SP, Chapman GJ, Murrey DA Jr, Lee R, Martin EW Jr, Hall NC (2013) Intraoperative detection of 18F-FDG-avid tissue sites using the increased probe counting efficiency of the K-alpha probe design and variance-based statistical analysis with the three-sigma criteria. BMC Cancer 13:98. https://doi.org/10.1186/1471-2407-13-98
Martin EW Jr, Thurston MO (1996) The use of monoclonal antibodies (MAbs) and the development of an intraoperative hand-held probe for cancer detection. Cancer Invest 14(6):560–571. https://doi.org/10.3109/07357909609076901
Burak WE Jr, Boso M, Thurston MO, Martin EW (1996) Surgical applications of gamma-detecting probes. Surg Technol Int 5:259–264
Chapman GJ, Povoski SP, Hall NC, Murrey DA Jr, Lee R, Martin EW Jr (2014) Comparison of two threshold detection criteria methodologies for determination of probe positivity for intraoperative in situ identification of presumed abnormal 18F-FDG-avid tissue sites during radioguided oncologic surgery. BMC Cancer 14:667. https://doi.org/10.1186/1471-2407-14-667
Martin EW, Chapman GJ, Subramaniam VV, Povoski SP (2010) Intraoperative detection of gamma emissions using K-alpha X-ray fluorescence. Expert Rev Med Devices 7(4):431–434. https://doi.org/10.1586/erd.10.33
Pani R, Pellegrini R, Cinti MN, Longo M, Donnarumma R, D’Alessio A, Borrazzo C, Pergola A, Ridolfi S (2016) De Vincentis G Development of a novel gamma probe for detecting radiation direction. J Instrumen. https://doi.org/10.1088/1748-0221/11/01/C01002
Bononi M, Viviana F, De Feo MS, Sollaku S, Pani A, Falconi R, Pani R, Cavallaro G, Brozzetti S, De Vincentis G (2021) Gonioprobe, an innovative gamma-probe to guide parathyroid radio-guided surgery: First clinical experiences with navigator and lock-on-target functions. Curr Radiopharm 14(2):161–169. https://doi.org/10.2174/1874471013666200721013903
Falconi M, Bartsch DK, Eriksson B, Klöppel G, Lopes JM, O’Connor JM, Salazar R, Taal BG, Vullierme MP, O’Toole D (2012) ENETS Consensus Guidelines for the management of patients with digestive neuroendocrine neoplasms of the digestive system: well-differentiated pancreatic non-functioning tumors. Neuroendocrinology 95(2):120–134. https://doi.org/10.1159/000335587
Yang J, Kan Y, Ge BH, Yuan L, Li C, Zhao W (2014) Diagnostic role of Gallium-68 DOTATOC and Gallium-68 DOTATATE PET in patients with neuroendocrine tumors: a meta-analysis. Acta Radiol 55(4):389–398. https://doi.org/10.1177/0284185113496679
Evangelista L, Farsad M, Piotto A, Pelizzo MR (2014) 18F-DOPA and 18F-FDG PET/CT, scintigraphic localization and radioguided surgery of recurrent medullary thyroid cancer: two case reports. Curr Radiopharm 7(2):133–137. https://doi.org/10.2174/1874471007666141027151130
López-Gómez J, Medina-Ornelas S, Salazar-Álvarez MA, Álvarez-Bojórquez M, Zaragoza-Cruz N, Melchor-Ruan J, Álvarez-Cano A (2018) Radioguided hepatic resection with (18)F-DOPA in a patient with metastatic medullary thyroid carcinoma. Rev Esp Med Nucl Imagen Mol (Engl Ed) 37(4):244–249. https://doi.org/10.1016/j.remn.2017.12.003
Filippi L, Palumbo B, Frantellizzi V, Nuvoli S, De Vincentis G, Spanu A, Schillaci O (2022) Prostate-specific membrane antigen-directed imaging and radioguided surgery with single-photon emission computed tomography: state of the art and future outlook. Expert Rev Med Devices 19(11):815–824. https://doi.org/10.1080/17434440.2022.2146999
Funding
Open access funding provided by Università degli Studi di Roma La Sapienza within the CRUI-CARE Agreement. No funding has been received for this paper.
Author information
Authors and Affiliations
Contributions
DFMS: literature search, literature review, and manuscript writing. FV: manuscript writing, editing and content planning. DSL: editing and content planning. FA: editing and content planning. DVG: editing and content planning. PR: editing and content planning.
Corresponding author
Ethics declarations
Conflict of interest
Maria Silvia De Feo, Viviana Frantellizzi, Luciano De Sio, Alessio Farcomeni, Giuseppe De Vincentis and Roberto Pani declare that have no conflict of interest.
Ethical standards
This article does not contain any studies with human participants or animal subjects performed by any of the authors.
Additional information
Publisher's Note
Springer Nature remains neutral with regard to jurisdictional claims in published maps and institutional affiliations.
Rights and permissions
Open Access This article is licensed under a Creative Commons Attribution 4.0 International License, which permits use, sharing, adaptation, distribution and reproduction in any medium or format, as long as you give appropriate credit to the original author(s) and the source, provide a link to the Creative Commons licence, and indicate if changes were made. The images or other third party material in this article are included in the article's Creative Commons licence, unless indicated otherwise in a credit line to the material. If material is not included in the article's Creative Commons licence and your intended use is not permitted by statutory regulation or exceeds the permitted use, you will need to obtain permission directly from the copyright holder. To view a copy of this licence, visit http://creativecommons.org/licenses/by/4.0/.
About this article
Cite this article
De Feo, M.S., Frantellizzi, V., De Sio, L. et al. Role of PET gamma detection in radioguided surgery: a systematic review. Clin Transl Imaging 11, 567–585 (2023). https://doi.org/10.1007/s40336-023-00559-3
Received:
Accepted:
Published:
Issue Date:
DOI: https://doi.org/10.1007/s40336-023-00559-3