Abstract
Purpose of Review
We explore the challenges of managing solid organ transplant recipients (SOTRs) during the COVID-19 pandemic, with a focus on prolonged viral detection in immunosuppressed individuals.
Recent Findings
SOTR guidelines recommend three mRNA vaccine doses with additional booster dosing and continued protective post-vaccination measures. COVID-19 therapies are similar for SOTRs and non-SOTRs, although drug-drug interactions limit the use of some such as nirmatrelvir/ritonavir (NIM-RTV). Inpatient treatment options include remdesivir and steroids; outpatient antiviral options include NIM-RTV or remdesivir. Whereas molnupiravir has not been withdrawn in the USA, it is no longer available in Europe due to safety and efficacy concerns, along with selection mutagenesis. Prolonged viral replication in immunosuppressed patients presents the risk of future variant generation and concern for transmission.
Summary
SOTR COVID-19 guidelines emphasize vaccination and protective measures; persistently positive cases remain a challenge. Medications promoting selection mutagenesis are ill-advised for those already at risk of incubating variants capable of immunologic escape.
Similar content being viewed by others
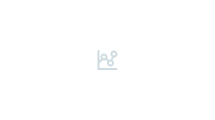
Introduction
The SARS-CoV-2/COVID-19 pandemic continues to be a source of significant morbidity and mortality in the solid organ transplant (SOT) patient population. Persistent SARS-CoV-2 positivity and indolent viral replication in immunosuppressed patients have been identified in multiple case series and present challenges for clinicians providing ongoing SOT care while attempting to protect other patients in their cohorts [1••, 2, 3]. Additionally, prolonged detection of viral RNA by reverse-transcriptase polymerase chain reaction (RT-PCR) in body fluids suggests a possibility of a latent reservoir of virus capable of undetected continuous mutation. Here, we present a case of a prolonged period of SARS-CoV-2 positivity and mutational drift in a lung transplant recipient to illustrate several clinical and scientific challenges for solid organ transplant recipient (SOTR) care in the COVID-19 era.
Case Report
A 65-year-old man underwent bilateral lung transplantation for interstitial lung disease in 2020, prior to the availability of vaccines against SARS-CoV-2. Non-ablative immune induction upon transplantation included basiliximab (monoclonal antibody against CD25, the α-subunit of the IL2 receptor), along with 1000 mg of intravenous (IV) mycophenolate mofetil (MMF) and 1000 mg of IV methylprednisolone. Initial maintenance oral immunosuppression included triple-drug therapy, consisting of MMF 1000 mg twice daily (up to 8 months following transplant), tacrolimus with goal serum troughs of 8–12 ng/ml during the first year, and prednisone. MMF was reduced to 500 mg twice daily at 8 months following transplant due to leukopenia. His post-transplant course was complicated by grade 2 primary graft dysfunction requiring prolonged mechanical ventilation and tracheostomy. Following liberation from mechanical ventilation, he was discharged home 29 days following the transplant. The patient’s forced expiratory volume in the first second (FEV1) improved, and surveillance transbronchial biopsies performed at 1- and 3-months post-transplantation demonstrated no evidence of acute cellular rejection (ACR), and asymptomatic, minimal (A1, B0) ACR that did not require additional therapy, respectively.
Four months after the transplantation, he developed mild sinus congestion and a new cough; he had a positive nasopharyngeal swab for SARS-CoV-2 tested by a commercial RT-PCR kit (TaqPath™ COVID-19 Combo Kit, Thermo Fisher Scientific, Inc, Waltham, MA) with a lower limit of detection of 25 copies/ml. Neutralizing monoclonal antibody bamlanivimab was infused on the third day of known infection without any additional therapy or need for hospitalization. Despite symptom resolution, the patient’s nasopharyngeal RT-PCR, tested as part of the standard infection-control measures at the time for the institution, remained persistently positive beyond 1 year (Table 1). Viral sequencing of samples confirmed an acquisition of serial mutations of an unnamed B.1.2 variant (clade 20G, Table 2).
Despite persistently positive nasopharyngeal RT-PCR results, his transplanted lungs remained uninvolved clinically and radiologically (Fig. 1). The patient was vaccinated against SARS-CoV-2 with the mRNA-1273 anti-SARS-CoV-2 vaccine (Moderna) 6 months following the initial SARS-CoV-2 positive test. Surveillance transbronchial biopsies at 6 months and 1-year post-transplant showed no evidence of rejection. Imaging remained without new pulmonary infiltrates. At the time of publication, the patient has remained free of donor-specific HLA antibodies (DSA) that were tested by Luminex-based single antigen beads (One Lambda, Inc., A Thermo Fisher Company, Canoga Park, CA). The patient was also tested for SARS-CoV-2 IgG antibodies using a multi-target commercial Luminex-based kit (LABScreen COVID Plus Antibody Testing, Thermo Fisher Scientific, Inc, Waltham, MA). While the patient had detectable anti-SARS-CoV-2 IgG antibodies against spike, RBD, S1, and S2 antigens (indicating a specific humoral response to SARS-CoV-2), the anti-nucleocapsid antibody remained negative (Table 1) consistent with defective humoral antiviral response. The subject has done well from a pulmonary perspective now 2 years after transplant.
Representative images from CT scan of the chest at 16 months post-transplantation from the upper (A), middle (B), and lower (C) lung zones illustrating no significant parenchymal abnormality or pneumonia despite persistent COVID-19 positive testing. Left sided pleural thickening and small effusion with associated atelectasis (C) are noted, which has been chronic and stable for this patient
Impact of COVID-19 Pandemic on SOT Recipients
The SARS-CoV-2 virus, responsible for the COVID-19 global pandemic, has persisted since its zoonotic jump in late 2019. On May 5, 2023, the World Health Organization (WHO) announced that the disease was no longer classified as a public health emergency of international concern (PHEIC). However, this did not mark the end of the pandemic but rather indicated a shift towards national responsibility for the long-term management of the virus [4].
Disruption of Solid Organ Transplant Care
By May 2020, SARS-CoV-2 had spread globally, presenting with a spectrum of manifestations, from asymptomatic cases to severe illnesses, straining hospitals worldwide [5, 6]. Certain comorbidities, such as CKD, liver and lung diseases, immunosuppression, diabetes mellitus, obesity, hypertension, pregnancy, and age over 50, were associated with higher morbidity and mortality [7]. Consequently, many transplant centers scaled back or halted operations, impacting transplant rates and altering patient management. Boyarsky et al. assessed 88 centers from March to May 2020, revealing significant disruptions across organ transplants [8]. Living donor kidney transplant (LDKT) saw major impacts, with 30% suspending entirely and 51% facing restrictions like limited organ recovery and equipment challenges. Similarly, 51% of deceased donor kidney transplant (DDKT) programs faced restrictions. Living donor liver transplant (LDLT) halted in 52% of centers, while 57% of deceased donor liver transplant (DDLT) programs operated unhindered. Only 36% of heart and 29% of lung transplant programs remained unrestricted.
Mandatory RT-PCR testing for both donors and recipients became standard. Most transplant centers initially halted surgeries if either party tested positive for COVID-19 [8] until nuanced risk assessments distinguished between lung and non-lung SOTs [9]. Cholankeril et al. found a 23.3% drop in waitlist additions and transplant surgeries due to the pandemic, and a 26.2% increase in waitlist deaths [10]. Single-center ICU studies in 2020 revealed SOTRs faced higher severe disease risks and mortality rates than non-SOTRs (39% vs. 6.1% respectively for poor outcomes and 24% vs. 1.4%–4.3% respectively for mortality). Non-SOTRs were defined as patients in the cohort without medical history of solid organ transplantation [11,12,13]. Outcomes and immunosuppression adjustments depended on organ type, symptom severity [11,12,13], and provider discretion, with limited guiding data. Some studies found similar outcomes independent of transplant status [14,15,16,17]. A 2021 study revealed mortality rates in hospitalized SOTRs for COVID-19 declined from 19.6 to 13.7% between early and late 2020, mirroring trends in the broader population [18]. Other findings suggested therapeutic improvements benefited both SOTRs and the general population, as hospital mortality rates dropped from 25.6 in March 2020 to 7.6% in August 2020 [12,13,14, 19].
Persistent Positivity of COVID-19 RT-PCR
Recent 2023 data indicates that COVID-19 exhibits peak viral loads in the respiratory system 2–3 days after symptom onset [20, 98]. However, some experts suggest peak viral load may occur even before symptom onset or within 1–2 days of symptoms [99]. The alpha variant’s median shedding duration ranges from 8 to 15 days, with some cases exceeding 100 days [21,22,23,24]. Current data on continuously positive RT-PCR test results are limited, mostly consisting of case reports and small retrospective analyses. Factors for persistent nasopharyngeal positive results include male gender, age, hypertension, immunosuppression, severe COVID-19, and respiratory failure requiring mechanical ventilation [25, 26]. Persistent viral shedding is presumably frequent in immunocompromised individuals, occasionally extending beyond a year. To our knowledge, the patient presented in this case has the longest reported duration of RT-PCR positivity by the same viral variant [27, 28].
The Emergence of Medical Therapies and Vaccines for COVID-19
Remdesivir, Dexamethasone, Tocilizumab, and Baricitinib
The Food and Drug Administration (FDA) granted emergency use authorization (EUA) for the first effective COVID-19 antiviral treatment, remdesivir, in May 2020, which later received full approval [29]. Initially prescribed for hospitalized patients with lower respiratory infections [29,30,31], the regimen of a 200 mg loading dose followed by 100 mg daily over 10 days showed faster median recovery (10 vs. 15 days) and reduced mortality (14.3% vs. 15.2%) in the ACTT-1 trial compared to standard care [29, 32]. Subsequently, a 5-day course of the same drug was found to be non-inferior [31]. Notably, recent data indicates that remdesivir reduces mortality in immunocompromised patients, a benefit persisting throughout the pandemic’s duration [33].
The RECOVERY trial in July 2020 showed that dexamethasone (6 mg daily for 10 days) reduced mortality rates (from 25.7 to 22.9%) in hospitalized COVID-19 patients with hypoxemia; there was no benefit and a trend towards harm in patients without hypoxemia [34]. While anti-IL6 antibodies like tocilizumab had mixed results [35, 36], leading to a weaker recommendation in IDSA guidelines [37], oral baricitinib consistently showed positive outcomes [38, 39]. The baricitinib dose is renally adjusted and used for up to 14 days. The Center for Disease Control and Prevention (CDC), American Society of Transplantation (AST), National Institute of Health (NIH), American Association for the Study of Liver Diseases (AASLD), and the International Society for Heart and Lung Transplantation (ISFLT) agree that there is no evidence to support managing moderate to severe COVID-19 differently in SOTRs [40,41,42, 43••, 44, 45]. For SOTRs, special considerations are for drug interactions and possible consideration of interruption of immunosuppression (mycophenolate mofetil, mTOR inhibitors, and azathioprine) when patients are admitted with moderate/severe disease to decrease the risk of superinfection, though data are limited [40,41,42, 43••, 44, 45].
The Rise and Fall of Monoclonal Antibodies
During the pandemic, the FDA issued now-revoked EUAs for several SARS-CoV-2 targeting monoclonal antibodies, including bamlanivimab (November 2020), casirivimab plus imdevimab (November 2020), bamlanivimab plus etesevimab (February 2021), sotrovimab (May 2021), and bebtelovimab (February 2022) [46, 47]. Concerns about their efficacy in SOTRs given their immunosuppressed stats were evaluated [48,49,50]. Klein and colleagues’ analysis showed that 15% of SOTRs given these antibodies required hospitalization or emergency department visits, compared to 76% who did not receive them (p < 0.001), and no deaths were observed in the treated group while there was 8% mortality in those untreated [50]. In a study involving 73 SOTRs with mild to moderate COVID-19 who received monoclonal antibody therapy, favorable outcomes were observed, including low hospitalization rates or advanced respiratory support requirements, and zero deaths or allograft rejection (p < 0.05) [48]. A study on the efficacy and safety of bamlanivimab and casirivimab-imdevimab in SOTRs with mild to moderate COVID-19 found a 30-day hospitalization rate of 8.7%, and no graft losses or deaths; a comparison with SOT recipients who did not receive monoclonal antibody treatment suggested a potentially lower hospitalization rate (odds ratio 0.49 [95% confidence interval 0.18–1.32], p = 0.16) [49]. Nevertheless, clinical use of monoclonal antibody therapies was short-lived given viral evolution and immunologic escape. Our patient received the contemporaneously authorized bamlanivimab, without requiring hospitalization or additional therapy. While symptoms resolved, the persistent positivity in our patient underscores the complexity of monoclonal antibodies in clearing the virus, particularly when both humoral and cellular immunity are impaired and when mutations can still accrue over time, leading to new SARS-CoV-2 isolates [47,48,49,50,51].
The monoclonal antibody combination tixagevimab/cilgavimab received EUA in December 2021 for pre-exposure prophylaxis in immunocompromised patients, but by January 2023, the EUA was rescinded given the lack of efficacy in neutralizing the SARS-CoV-2 variants circulating since that time [39]. Therefore, there are currently no prophylactic medical interventions outside of vaccination and exposure avoidance for high-risk patients [42, 44, 45, 52].
Current Guidelines for Mild COVID-19 Infection Management in SOTRs
According to the National Institutes of Health COVID-19 Treatment Guidelines [53], high-risk non-hospitalized adults should receive a 5-day course of oral nirmatrelvir (300 mg) combined with ritonavir (100 mg) (NIM-RTV) twice daily or a 3-day IV remdesivir treatment course if there are no limiting or unmanageable drug-drug interactions. If these are unavailable or contraindicated, a 5-day course of oral molnupiravir was recommended previously but is now de-emphasized in 2023 NIH treatment guideline updates with remdesivir now preferred (BIIa recommendation) when NIM-RTV is not an option; molnupirivir is relegated to an option as an alternative therapy (CIIa recommendation) [54]. For those with eGFR ≥ 30 but < 60 ml/min/1.73 m2, the NIM component dosage should be halved, and it is not recommended for eGFR below 30 ml/min/1.73 m2. NIM-RTV has significant drug interactions, especially with some immunosuppressant therapies like calcineurin inhibitors and with anticoagulation [55]. The PINETREE trial showed that remdesivir’s efficacy is on par with NIM-RTV [56•]. Given its consistent performance in studies focused on immunocompromised patients [57, 58] and its absence of drug interactions, IV remdesivir is often preferred for SOTRs; however, only a small fraction of eligible patients receive it due to the difficulty of arranging and having patients agree to 3 days of outpatient infusions [30, 41, 56•, 59]. The FDA updated remdesivir’s label, with remedesivir use now on-label for every level of renal function [60].
Although the viral mutagen molnupiravir has not yet been removed from NIH recommendations [61] and remains available in the USA when NIM-RTV cannot be given and if a 3-day outpatient course of remdesivir is not available, the marketing authorization application for molnupiravir has been withdrawn in Europe [62••]. Molnupiravir is a pro-drug of the degenerate base, N-hydroxycytidine, that once incorporated, can tautomerize between a cytosine (C) or uracil (U)-like hydrogen bond acceptor inducing transition mutations. Although this viral mutagenesis is intended to generate “error catastrophe” [66], it demonstrably increases viral evolution and escape [65•], as was anticipated ab initio. Based on the relative inefficacy of molnupiravir and the public health hazard posed by the acceleration of mutational escape via in vivo saturation mutagenesis across the SARS-CoV-2 genome [63, 64, 65•, 66, 67], we strongly discourage its use. For these problematic mutagenesis concerns and due to insufficient evidence of efficacy in the SOTR population, molnupiravir is not recommended for SOTRs by the AST, NIH, AASLD, and ISHLT [40,41,42, 43••, 53, 61]. Considering its mechanism of action (viral mutagenesis) and the lack of evidence for substantial benefit, including the negative PANAROMIC trial [64], the use of molnupiravir should be discouraged; it is no longer approved for use in most European countries [62••].
The Role of Vaccination and Prophylaxis Against COVID-19
A breakthrough in the management of the COVID-19 pandemic was achieved in December of 2020, with the EUA of mRNA vaccines. Initially studied as a two-dose vaccine series, the Pfizer-BioNTech and Moderna mRNA vaccine regimens (30 µg per dose, 21 days apart, and 100 µg per dose, 28 days apart, respectively) showed a comparable 94–95% efficacy in preventing symptomatic COVID-19 infection [68, 69]. Both vaccine trials reached primary safety endpoints, and common side effects were managed with over-the-counter analgesia and antipyretics [68, 69]. Following the vaccine rollout in the USA, cases of “breakthrough” SARS-CoV-2 infection were reported to the CDC by May 2021 [70], suggesting that immunity from vaccination or past infection could wane over time.
Initial vaccine label-generating trials excluded SOT recipients due to their immunosuppressive status [68, 69]. Subsequent vaccine trials post-FDA approval demonstrated efficacy and safety data concerning SOTRs. These trials showed strong efficacy in immunocompetent individuals, but immunocompromised patients, such as SOTRs, had attenuated immune responses as is the case for other vaccines. Initial data indicated a higher breakthrough infection rate in SOTRs post-two mRNA vaccine doses, highlighting reduced protection against infection [71,72,73,74]. An 82-fold increased risk of infection was observed in a study of 18,215 vaccinated SOTRs [70, 71]. Immunosuppressive drugs, specifically mycophenolate (MPA), affecting B and T-cell activation, were associated with lower post-vaccination seroconversion rates [72, 73]. Induction therapies (high doses of immunosuppression given in the peri-operative period of SOT) were also associated with reduced vaccine response (measured by antibody titers) [75, 76]. Interrupting MPA might enhance booster dose outcomes [76, 77]. Short-term interruption of immunosuppression in autoimmune disease patients has resulted in short-lived enhanced antibody titers to the booster dose, with no intervention-related serious adverse events [79]. Similar approaches of holding antimetabolites in kidney transplant recipients around the fourth vaccine dose administration have yielded higher rates of seroconversion [78, 79]. However, no consensus exists for adjusting or holding immunosuppression during vaccination for SOTRs—a potentially high-risk alteration of care given the potential for organ rejection [74]. Consequently, current AST guidelines advise against altering immunosuppression solely to improve vaccine response [45, 80].
In observational cohort studies measuring humoral response via antibody titers against the spike protein of SARS-CoV-2, SOTRs displayed limited positive antibody responses after one and two mRNA vaccine doses. Yet, a third dose significantly increased the antibody titer, with manageable side effects and heightened protection from severe COVID-19. Only 17% of SOTRs mounted a positive antibody response after the first dose of the mRNA vaccine. After the second dose, a significant proportion (54%) had detectable antibody responses, although titers were generally lower than in immunocompetent individuals [72,73,74, 81,82,83]. Enhanced T-cell responses after a third dose led the CDC to advocate for an additional dose for immunocompromised individuals [52]. The Novavax protein-conjugate vaccine remains an option under EUA for patients as an alternative to mRNA vaccines, as the mRNA vaccines have progressed to full regulatory approval and are being updated on an annual basis. Test-negative, case–control studies confirm that while the vaccine efficacy (VE) had somewhat decreased as the pandemic progressed, the vaccines remain effective compared to other widely adopted vaccines [84].
The most recent guidelines from the CDC, AST, AASLD, and ISLHT advise three initial mRNA vaccine doses and at least one bivalent (or subsequent updated monovalent) booster 2 or more months after the initial series [42, 43••, 52, 80]. When feasible, vaccination should be completed prior to transplantation or 1–3 months post-transplantation to avoid high-dose immunosuppression’s potential attenuation of a vaccine response [52]. Transplant recipients are also advised to continue protective behaviors (such as masking) post-vaccination [42, 80, 85].
Isolation Practices in COVID-19
According to guidance from the CDC [86], individuals who are infected with COVID-19 but do not show symptoms or have mild symptoms are advised to self-isolate for a minimum of 5 days. Day 0 is considered the day symptoms first appeared or when the positive test sample was collected for asymptomatic individuals. Additionally, these individuals are advised to wear masks until day 10. However, if a test-based strategy is employed and the results are negative, they may discontinue masking earlier. For individuals with moderate or severe COVID-19, the isolation period should be extended to at least day 10; in cases of severe COVID-19, it is extended for up to 20 days. The same document recommends that individuals who are moderately or severely immunocompromised should self-isolate for a minimum of 20 days, undergo serial testing, and seek guidance from an infectious disease specialist before ending the isolation period. These guidelines fail to address the problem of persistent positivity. It remains unclear whether our patient could have potentially spread the virus during his extended period of positive testing, though we are not aware of any evidence of spread to his contacts (Table 1).
The AST suggests that candidates with active COVID-19 be deferred transplantation, wait until complete symptom resolution, and at least until one negative RT-PCR test for SARS-CoV-2 [45]. When dealing with donors who have resolved clinical COVID-19 but still test positive by RT-PCR, the decision to proceed with transplantation is made on a case-by-case basis [45, 87]. There are a growing number of cases where non-lung organ transplantation from donors with recent SARS-CoV-2 infection has occurred without viral transmission and with positive short-term patient and graft outcomes [88,89,90,91,92,93]. For non-lung donors who have been exposed to SARS-CoV-2 but test negative, the safety of organ donation is no longer a concern.
Our patient acquired the COVID-19 infection 4 months following the transplantation and received the two-dose mRNA-1273 vaccine 6 months following the initial positive test. This would have been considered late in light of the guidelines discussed, though in his case vaccination timing was affected by vaccine availability. Current guidelines do not fully address the approach to vaccination in persistently positive cases since there is limited to no evidence of vaccine effectiveness in this unique patient population.
Management of Prolonged SARS-COV-2 Viral Shedding in Immunosuppressed Patients
Several case studies have reported persistent viral replication in immunosuppressed patients [1••, 2, 3, 94, 95]. This appears to be the case even in those treated with remdesivir. Hettle and colleagues identified six patients who had persistent SARS-CoV-2 infection [95]. All of them had hypogammaglobulinemia and underlying hematological malignancies. Previous attempts to clear the virus with alternative treatments had been unsuccessful. Additionally, serial analysis of viral load and sequencing in these patients was indicative of ongoing viral replication, as evidenced by cycle threshold values, the accrual of mutations associated with variants of concern, and the viral lineages being maintained throughout the course of infections [2, 3, 94, 96]. They also reviewed the literature and found 60 confirmed cases of persistent infection, along with an additional 31 probable cases. Among all of these cases, 80% of patients treated with monoclonal antibodies were able to clear the SARS-CoV-2 infection based on repeat RT-PCR testing, and there were no reported deaths [2, 3, 94,95,96].
However, some SARS-CoV-2 viruses in immunocompromised patients treated with convalescent plasma have experienced evolution and decreased sensitivity to neutralizing antibodies while maintaining a similar level of infectivity as the wild-type virus [96]. Genotypic variations have also been shown to increase in cases of longer positivity with consistently high viral load, as illustrated by genetic sequencing showing an increase in mutations during periods of increased viral load. As the immune response wanes and viral load increases, so does genetic diversity—especially in cases of particularly prolonged positivity [1••, 2, 3, 96]. Most concerningly, some variants identified in persistently positive patients show a high percentage of polymorphisms present in the spike protein-coding region consistent with adaptive evolution that could facilitate evasion of vaccine or exposure-related immunity. Corey et al. and Choi et al. reported that the majority of the mutations discovered via genetic sequencing in their respective patients were present in the spike gene and the receptor binding domain [1••, 2]. These areas are the target of many therapeutic neutralizing antibodies; therefore, mutations are indicative of adaptive evolution. Correspondingly, the consecutive samples from our patient revealed multiple mutations in the spike and the nucleocapsid genes (Table 2.), raising concerns about an adaptive variant that could potentially resist immune responses, thereby becoming more prone to spread.
In conclusion, some immunocompromised patients may test positive longer than the general population (at least 20 days after initial infection), but recommendations are limited to continued social distancing with frequent re-testing until two consecutive negative tests have been obtained [42, 43••, 86]. The CDC also recommends serial sequencing to identify variants of interest, but guidelines do not specify how to address the issue of continued viral replication [86]. Additionally, the correct approach for patients who remain persistently positive, as was the case in our patient, especially with respect to the need for procedures, pulmonary function testing, and other parts of their routine SOT care remains unclear. Virally stimulated T-cells to therapeutically target SARS-CoV-2 may hold promise for those unable to clear the virus [97].
Back to the Case
Our patient experienced a relatively mild case of COVID-19, limited to upper respiratory involvement alone, which had no measurable impact on transplant-related outcomes (Fig. 1). He was administered neutralizing monoclonal antibodies (bamlanivimab), which may have aided symptom management but did not stop continuous viral replication for at least a year. Over time, the patient developed a virus-specific immune response against viral antigens, but not the nucleocapsid; it is unclear if the partial seroconversion represents a humoral response to the vaccine or stimulation by the virus itself. Nevertheless, a degree of anergy is evident (Table 1). Also, due to persistent positivity and contemporary local infection control guidelines, the patient could not undergo aerosolizing procedures (spirometry) until they had two consecutive negative PCR-based tests. Ultimately, exceptions were made in his case to obtain spirometry (which was done specifically in a negative pressure room and with additional PPE provision for the staff) given the duration of his positive test results. To differentiate whether this patient’s positive testing reflected the same virus (as opposed to repeated infection with SARS-CoV-2), his virus was sequenced, allowing us to determine that his tests reflected the same variant undergoing mutational drift (Table 2). Despite concerns about continuous viral shedding throughout this time period and potential exposure for his contacts, there was no objective evidence of disease transmission despite close contact with family starting 3 weeks after his COVID-19 diagnosis. Due to the risk of rejection and recency of lung transplantation, the patient’s immunosuppression was not interrupted during his clinical course.
Conclusion
SARS-CoV-2 presents challenges for SOT patients given their increased risk of severe disease and the decreased efficacy of prophylactic and treatment interventions. Viral replication may be prolonged in some SOTRs, leading to persistently positive RT-PCR testing and the acquisition of private mutations from ongoing viral replication and immunologic escape. The COVID-19 pandemic highlights the risk that highly transmissible infectious diseases pose for immunocompetent and immunocompromised patients alike. Therapeutic and prophylactic interventions can decrease mortality, and infection control measures are more complex in these patients. Persistent viral shedding has been repeatedly documented in immunocompromised patients such as ours and is of particular concern given the potential for viral adaptation over time. Though morbidity and mortality of COVID-19 infection have waned over time as dominant variants have changed and therapies have improved, and while governmental declarations of emergency have ended allowing social practices to normalize for most of the world, COVID-19 remains a persistent clinical challenge, especially for SOTRs.
Data Availability
Anonymized data is available for review upon reasonable request.
References
Papers of particular interest, published recently, have been highlighted as: • Of importance •• Of major importance
•• Corey L, Beyrer C, Cohen MS, Michael NL, Bedford T, Rolland M. SARS-CoV-2 variants in patients with immunosuppression. n engl j med. 2021. This study examines several case studies of viral evolution of SARS-CoV-2 in immunosuppressed individuals and emphasizes the importance of surveillance to protect these individuals and the public from viral adaptation.
Choi B, Choudhary MC, Regan J, Sparks JA, Padera RF, Qiu X, et al. Persistence and evolution of SARS-CoV-2 in an immunocompromised host. New England Journal of Medicine. 2020;383:2291–3. This study
Sepulcri C, Dentone C, Mikulska M, Bruzzone B, Lai A, Fenoglio D, et al. The longest persistence of viable SARS-CoV-2 with recurrence of viremia and relapsing symptomatic COVID- 19 in an immunocompromised patient-a case study. Open Forum Infect Dis. 2022;8.
International Health Regulations Emergency Committee. Statement on the fifteenth meeting of the IHR (2005) emergency committee on the COVID-19 pandemic [Internet]. 2023 [cited 2023 Sep 14]. Available from: https://www.who.int/news/item/05-05-2023-statement-on-the-fifteenth-meeting-of-the-international-health-regulations-(2005)-emergency-committee-regarding-the-coronavirus-disease-(covid-19)-pandemic
Holshue ML, DeBolt C, Lindquist S, Lofy KH, Wiesman J, Bruce H, et al. First case of 2019 novel coronavirus in the United States. N Engl J Med. 2020;382:929–36.
Burke RM, Killerby ME, Newton S, Ashworth CE, Berns AL, Brennan S, et al. Morbidity and mortality weekly report symptom profiles of a convenience sample of patients with COVID-19-United States, January-April 2020. 2020.
Centers for Disease Control and Prevention. Underlying medical conditions associated with higher risk for severe COVID-19: information for healthcare professionals [Internet]. 2023 [cited 2023 Jul 27]. Available from: https://www.cdc.gov/coronavirus/2019-ncov/hcp/clinical-care/underlyingconditions.html
Boyarsky BJ, Ruck JM, Chiang TPY, Werbel WA, Strauss AT, Getsin SN, et al. Evolving impact of COVID-19 on transplant center practices and policies in the United States. Clin Transplant. 2020;34.
Heldman MR, Kates OS, Safa K, Kotton CN, Georgia SJ, Steinbrink JM, et al. COVID-19 in hospitalized lung and non-lung solid organ transplant recipients: a comparative analysis from a multicenter study. Am J Transplant. 2021;21:2774–84.
Cholankeril G, Podboy A, Alshuwaykh OS, Kim D, Kanwal F, Esquivel CO, et al. Early impact of COVID-19 on solid organ transplantation in the United States. Transplantation. 2020;2221–4.
Rodrigues B, Daoud D, Li G, Segraves J, Hemmersbach-Miller M, Loor G, et al. COVID-19 in lung-transplant recipients: a descriptive study. J Heart Lung Transplant. 2021;40:S315.
Pereira MR, Mohan S, Cohen DJ, Husain SA, Dube GK, Ratner LE, et al. COVID-19 in solid organ transplant recipients: initial report from the US epicenter. Am J Transplant. 2020;20:1800–8.
Banerjee D, Popoola J, Shah S, Ster IC, Quan V, Phanish M. COVID-19 infection in kidney transplant recipients. Kidney Int. Elsevier B.V.; 2020. p. 1076–82.
Molnar MZ, Bhalla A, Azhar A, Tsujita M, Talwar M, Balaraman V, et al. Outcomes of critically ill solid organ transplant patients with COVID-19 in the United States. Am J Transplant. 2020;20:3061–71.
Chaudhry ZS, Williams JD, Vahia A, Fadel R, Parraga Acosta T, Prashar R, et al. Clinical characteristics and outcomes of COVID-19 in solid organ transplant recipients: a cohort study. Am J Transplant. 2020;20:3051–60.
Maggiore U, Riella LV, Azzi J, Cravedi P. Mortality in solid organ transplant recipients with COVID-19: more than meets the eye. American Journal of Transplantation. John Wiley and Sons Inc; 2022. p. 1496–7.
Mendoza MA, Raja M, Villavicencio A, Anjan S, Natori Y. Is the outcome of SARS-CoV-2 infection in solid organ transplant recipients really similar to that of the general population? American Journal of Transplantation. Blackwell Publishing Ltd; 2021. p. 1670–1.
Heldman MR, Kates OS, Safa K, Kotton CN, Georgia SJ, Steinbrink JM, et al. Changing trends in mortality among solid organ transplant recipients hospitalized for COVID-19 during the course of the pandemic. Am J Transplant. 2022;22:279–88.
Horwitz LI, Jones SA, Cerfolio RJ, Francois F, Greco J, Rudy B, et al. Trends in COVID-19 risk-adjusted mortality rates. J Hosp Med. 2021;16:90–2.
Yang Y, Guo L, Yuan J, Xu Z, Gu Y, Zhang J, et al. Viral and antibody dynamics of acute infection with SARS-CoV-2 omicron variant (B.1.1.529): a prospective cohort study from Shenzhen, China. Lancet Microbe. 2023;4:e632–41.
Da LW, Chang SY, Wang JT, Tsai MJ, Hung CC, Hsu CL, et al. Prolonged virus shedding even after seroconversion in a patient with COVID-19. J Infect. 2020;81:318.
Li J, Zhang L, Liu B, Song D. Case report: viral shedding for 60 days in a woman with COVID-19. Am J Trop Med Hyg. 2020;102:1210–3.
van Kampen JJA, van de Vijver DAMC, Fraaij PLA, Haagmans BL, Lamers MM, Okba N, et al. Duration and key determinants of infectious virus shedding in hospitalized patients with coronavirus disease-2019 (COVID-19). Nat Commun. 2021;12.
Joukar F, Yaghubi Kalurazi T, Khoshsorour M, Taramian S, Mahfoozi L, Balou HA, et al. Persistence of SARS-CoV-2 RNA in the nasopharyngeal, blood, urine, and stool samples of patients with COVID-19: a hospital-based longitudinal study. Virol J. 2021;18.
Xu K, Chen Y, Yuan J, Yi P, Ding C, Wu W, et al. Factors associated with prolonged viral RNA shedding in patients with coronavirus disease 2019 (COVID-19). Clin Infect Dis. 2020;71:799–806.
Genuardi MV, Moss N, Najjar SS, Houston BA, Shore S, Vorovich E, et al. Coronavirus disease 2019 in heart transplant recipients: risk factors, immunosuppression, and outcomes. J Heart Lung Transplant. 2021;40:926–35.
Cunha M dos P, Vilela APP, Molina CV, Acuña SM, Muxel SM, Barroso V de M, et al. Atypical prolonged viral shedding with intra-host SARS-CoV-2 evolution in a mildly affected symptomatic patient. Front Med (Lausanne). 2021;8:760170.
Avanzato VA, Matson MJ, Seifert SN, Pryce R, Williamson BN, Anzick SL, et al. Case study: prolonged infectious SARS-CoV-2 shedding from an asymptomatic immunocompromised individual with cancer. Cell. 2020;183:1901-1912.e9.
Beigel JH, Tomashek KM, Dodd LE, Mehta AK, Zingman BS, Kalil AC, et al. Remdesivir for the treatment of Covid-19 — final report. N Engl J Med. 2020;383:1813–26.
Spinner CD, Gottlieb RL, Criner GJ, Arribas López JR, Cattelan AM, Soriano Viladomiu A, et al. Effect of remdesivir vs standard care on clinical status at 11 days in patients with moderate COVID-19: a randomized clinical trial. JAMA. 2020;324:1048–57.
Goldman JD, Lye DCB, Hui DS, Marks KM, Bruno R, Montejano R, et al. Remdesivir for 5 or 10 days in patients with severe Covid-19. N Engl J Med. 2020;383:1827–37.
Chokkalingam AP, Hayden J, Goldman JD, Li H, Asubonteng J, Mozaffari E, et al. Association of remdesivir treatment with mortality among hospitalized adults with COVID-19 in the United States. JAMA Netw Open. 2022;5:E2244505.
Mozaffari E, Chandak A, Gottlieb RL, Chima-Melton C, Read SH, Jiang H, Chiang M, Lee E, Gupta R, Berry M, Kalil AC. Remdesivir reduced mortality in immunocompromised patients hospitalized for COVID-19 across variant waves: Findings from routine clinical practice. Clinical Infectious Diseases. 2023;77(12):1626-34. Available from: https://doi.org/10.1093/cid/ciad460
Abani O, Abbas A, Abbas F, Abbas M, Abbasi S, Abbass H, et al. Tocilizumab in patients admitted to hospital with COVID-19 (RECOVERY): a randomised, controlled, open-label, platform trial. Lancet [Internet]. 2021 [cited 2023 Sep 14];397:1637–45. Available from: https://pubmed.ncbi.nlm.nih.gov/33933206/
Salama C, Han J, Yau L, Reiss WG, Kramer B, Neidhart JD, et al. Tocilizumab in patients hospitalized with Covid-19 pneumonia. N Engl J Med. 2021;384:20–30.
Rosas IO, Diaz G, Gottlieb RL, Lobo SM, Robinson P, Hunter BD, et al. Tocilizumab and remdesivir in hospitalized patients with severe COVID-19 pneumonia: a randomized clinical trial. Intensive Care Med. 2021;47:1258–70.
Bhimraj A, Morgan RL, Shumaker AH, Baden L, Cheng VC-C, Edwards KM, et al. IDSA Guidelines on the treatment and management of patients with COVID-19 [Internet]. 2023 [cited 2023 Sep 14]. Available from: https://www.idsociety.org/practice-guideline/covid-19-guideline-treatment-and-management/
Kalil AC, Patterson TF, Mehta AK, Tomashek KM, Wolfe CR, Ghazaryan V, et al. Baricitinib plus remdesivir for hospitalized adults with Covid-19. N Engl J Med. 2021;384:795–807.
Marconi VC, Ramanan AV, de Bono S, Kartman CE, Krishnan V, Liao R, et al. Efficacy and safety of baricitinib for the treatment of hospitalised adults with COVID-19 (COV-BARRIER): a randomised, double-blind, parallel-group, placebo-controlled phase 3 trial. Lancet Respir Med. 2021;9:1407–18.
National Institutes of Health. Therapeutic management of hospitalized adults with COVID-19 [Internet]. 2022 Aug. Available from: https://www.covid19treatmentguidelines.nih.gov
Kumar D, Humar A, Ison MG, Kaul D, Blumberg E, Theodoropoulos N, et al. AST statement on oral antiviral therapy for COVID-19 for organ transplant recipients. 2022 Jan.
Fix OK, Fontana RJ, Chu J, Chung RT, Goacher EK, Hameed B, et al. AASLD expert panel consensus statement: COVID-19 clinical best practice advice for hepatology and liver transplant providers [Internet]. Alexandria, VA; 2022 Oct. Available from: https://www.aasld.org
•• Aslam S, Danziger-Isakov L, Luong M-L, Husain S, Silveira FP, Adler E, et al. Guidance from the International Society of Heart and Lung Transplantation regarding the SARS CoV-2 pandemic. 2021 Feb.
Centers for Disease Control and Prevention. Transplants | COVID-19 treatment guidelines [Internet]. 2023 [cited 2023 Sep 14]. Available from: https://www.covid19treatmentguidelines.nih.gov/special-populations/transplant/
American Society of Transplantation. 2019-nCoV (Coronavirus): FAQs for organ transplantation. 2023 [cited 2023 Jul 26]; Available from: https://www.myast.org
U.S. Food & Drug Administration. Coronavirus (COVID-19) | Drugs [Internet]. 2023 [cited 2023 Jul 27]. Available from: https://www.fda.gov/drugs/emergency-preparedness-drugs/coronavirus-covid-19-drugs
Dougan M, Azizad M, Chen P, Feldman B, Frieman M, Igbinadolor A, et al. Bebtelovimab, alone or together with bamlanivimab and etesevimab, as a broadly neutralizing monoclonal antibody treatment for mild to moderate, ambulatory COVID-19. medRxiv. 2022;2022.03.10.22272100.
Yetmar ZA, Beam E, O’Horo JC, Ganesh R, Bierle DM, Brumble L, et al. Monoclonal antibody therapy for COVID-19 in solid organ transplant recipients. Open Forum Infect Dis. 2021;8.
Sarrell BA, Bloch K, El Chediak A, Kumm K, Tracy K, Forbes RC, et al. Monoclonal antibody treatment for COVID-19 in solid organ transplant recipients. Transplant Infectious Disease. 2022;24.
Klein EJ, Hardesty A, Vieira K, Farmakiotis D. Use of anti-spike monoclonal antibodies in kidney transplant recipients with COVID-19: efficacy, ethnic and racial disparities. Am J Transplant. 2022;22:640–5.
National Institutes of Health. Anti-SARS-CoV-2 monoclonal antibodies [Internet]. 2023 Mar. Available from: https://www.covid19treatmentguidelines.nih.gov
Centers for Disease Control and Prevention. COVID-19 vaccines for people who are moderately or severely immunocompromised [Internet]. 2023 [cited 2023 Jul 27]. Available from: https://www.cdc.gov/coronavirus/2019-ncov/vaccines/recommendations/immuno.html#
National Institutes of Health. Therapeutic management of nonhospitalized adults with COVID-19 [Internet]. 2022 Dec. Available from: https://www.covid19treatmentguidelines.nih.gov
Saravolatz LD, Depcinski S, Sharma M. Molnupiravir and nirmatrelvir-ritonavir: oral coronavirus disease 2019 antiviral drugs. Clin Infect Dis. 2023;76:165–71.
Zijp TR, Toren-Wielema ML, Nannan Panday PV, Kosterink JGW, Berger SP, Touw DJ. Important interactions of immunosuppressants with experimental therapies for novel coronavirus disease (COVID-19): how to act. Ther Drug Monit. Lippincott Williams and Wilkins; 2020. p. 652–3.
• Gottlieb RL, Vaca CE, Paredes R, Mera J, Webb BJ, Perez G, et al. Early remdesivir to prevent progression to severe Covid-19 in outpatients. New England Journal of Medicine. 2022;386:305–15. This is a randomized, double-blind, placebo-controlled trial involving high risk patients, including those who are immunocompromised. This study demonstrated that early administration of a 3-day course of remdesivir resulted in a 87% reduction in the risk of hospitalizations or death thereby preventing progression to severe disease.
Rajme-López S, Martinez-Guerra BA, Zalapa-Soto J, Román-Montes CM, Tamez-Torres KM, González-Lara MF, et al. Early outpatient treatment with remdesivir in patients at high risk for severe COVID-19: a prospective cohort study. Open Forum Infect Dis. 2022;9.
Solera JT, Árbol BG, Bahinskaya I, Marks N, Humar A, Kumar D. Short-course early outpatient remdesivir prevents severe disease due to COVID-19 in organ transplant recipients during the omicron BA.2 wave. Am J Transplant. 2023;23:78–83.
Buxeda A, Arias-Cabrales C, Pérez-Sáez MJ, Cacho J, Cabello Pelegrin S, Melilli E, et al. Use and safety of remdesivir in kidney transplant recipients with COVID-19. Kidney Int Rep. 2021;6:2305.
U.S. Food and Drug Administration. Highlights of prescribing information, 2.4 [Internet]. 2023 [cited 2023 Sep 14]. p. 4–4. Available from: https://www.accessdata.fda.gov/
National Institutes of Health. COVID-19 treatment guidelines | Molnupiravir [Internet]. 2023 [cited 2023 Jul 27]. Available from: https://www.covid19treatmentguidelines.nih.gov/therapies/antivirals-including-antibody-products/molnupiravir/
•• European Medicines Agency. Lagevrio: withdrawn application [Internet]. [cited 2023 Sep 14]. Available from: https://www.ema.europa.eu/en/medicines/human/withdrawn-applications/lagevrio
Jayk Bernal A, Gomes da Silva MM, Musungaie DB, Kovalchuk E, Gonzalez A, Delos Reyes V, et al. Molnupiravir for oral treatment of Covid-19 in nonhospitalized patients. New England Journal of Medicine. 2022;386:509–20.
Butler CC, Hobbs FDR, Gbinigie OA, Rahman NM, Hayward G, Richards DB, et al. Molnupiravir plus usual care versus usual care alone as early treatment for adults with COVID-19 at increased risk of adverse outcomes (PANORAMIC): an open-label, platform-adaptive randomised controlled trial. The Lancet. 2023;401:281–93.
• Sanderson T, Hisner R, Ah Donovan-Banfield I’, Hartman H, Løchen A, Peacock TP, et al. Ahead of print – identification of a molnupiravir-associated mutational signature in SARS-CoV-2 sequencing databases. medRxiv. 2023; This study identified genetic mutations in the SARS-CoV-2 virus directly linked to molnupiravir. This study underscores the need for medical professionals to update treatment guidelines to discourage the use of this drug to treat COVID-19 in order to prevent new variants with potential immune evasion.
Kabinger F, Stiller C, Schmitzová J, Dienemann C, Kokic G, Hillen HS, et al. Mechanism of molnupiravir-induced SARS-CoV-2 mutagenesis. Nat Struct Mol Biol. 2021;28:740–6.
Zhou S, Hill CS, Sarkar S, Tse LV, Woodburn BMD, Schinazi RF, et al. β-d-N4-hydroxycytidine Inhibits SARS-CoV-2 through lethal mutagenesis but is also mutagenic to mammalian cells. J Infect Dis. 2021;224:415–9.
Baden LR, El Sahly HM, Essink B, Kotloff K, Frey S, Novak R, et al. Efficacy and safety of the mRNA-1273 SARS-CoV-2 vaccine. N Engl J Med. 2021;384:403–16.
Polack FP, Thomas SJ, Kitchin N, Absalon J, Gurtman A, Lockhart S, et al. Safety and efficacy of the BNT162b2 mRNA Covid-19 vaccine. N Engl J Med. 2020;383:2603–15.
Birhane M, Bressler S, Chang G, Clark T, Dorough L, Fischer M, et al. COVID-19 vaccine breakthrough infections reported to CDC — United States, January 1–April 30, 2021. MMWR Morb Mortal Wkly Rep. 2021;70:792–3.
Qin CX, Moore LW, Anjan S, Rahamimov R, Sifri CD, Ali NM, et al. Risk of breakthrough SARS-CoV-2 infections in adult transplant recipients. Transplantation. Lippincott Williams and Wilkins; 2021. p. E265–6.
Boyarsky BJ, Werbel WA, Avery RK, Tobian AAR, Massie AB, Segev DL, et al. Immunogenicity of a single dose of SARS-CoV-2 messenger RNA vaccine in solid organ transplant recipients. JAMA - Journal of the American Medical Association. American Medical Association; 2021. p. 1784–6.
Boyarsky BJ, Werbel WA, Avery RK, Tobian AAR, Massie AB, Segev DL, et al. Antibody response to 2-dose SARS-CoV-2 mRNA vaccine series in solid organ transplant recipients. JAMA - Journal of the American Medical Association. American Medical Association; 2021. p. 2204–6.
Werbel WA, Boyarsky BJ, Ou MT, Massie AB, Tobian AAR, Garonzik-Wang JM, et al. Safety and immunogenicity of a third dose of SARS-CoV-2 vaccine in solid organ transplant recipients: a case series. Ann Intern Med. American College of Physicians; 2021. p. 1330–2.
Marion O, Del Bello A, Abravanel F, Faguer S, Esposito L, Laure Hebral A, et al. Predictive factors for humoral response after 2-dose SARS-CoV-2 vaccine in solid organ transplant patients. Transplant Direct. 2022;8:E1248.
Schrezenmeier E, Rincon-Arevalo H, Jens A, Stefanski A-L, Hammett C, Osmanodja B, et al. Temporary antimetabolite treatment hold boosts SARS-CoV-2 vaccination-specific humoral and cellular immunity in kidney transplant recipients. 2022; Available from: https://doi.org/10.1172/jci.insight.157836DS1
Kho MML, Messchendorp AL, Frölke SC, Imhof C, Koomen VJ, Malahe SRK, et al. Alternative strategies to increase the immunogenicity of COVID-19 vaccines in kidney transplant recipients not responding to two or three doses of an mRNA vaccine (RECOVAC): a randomised clinical trial. Lancet Infect Dis. 2023;23:307–19.
Durez P, Combe B. Should we stop methotrexate or not for vaccination? RMD Open: BMJ Publishing Group; 2023.
Abhishek A, Boyton RJ, Peckham N, McKnight Á, Coates LC, Bluett J, et al. Effect of a 2-week interruption in methotrexate treatment versus continued treatment on COVID-19 booster vaccine immunity in adults with inflammatory conditions (VROOM study): a randomised, open label, superiority trial. Lancet Respir Med. 2022;10:840–50.
American Society of Transplantation. COVID-19 vaccine FAQ sheet (updated 1/12/2023). 2023.
Hall VG, Ferreira VH, Ku T, Ierullo M, Majchrzak-Kita B, Chaparro C, et al. Randomized trial of a third dose of mRNA-1273 vaccine in transplant recipients. N Engl J Med. 2021;385:1244–6.
Villanego F, Cazorla JM, Vigara LA, Garcia T, Trujillo T, Montiel N, et al. Protecting kidney transplant recipients against SARS-CoV-2 infection: a third dose of vaccine is necessary now. American Journal of Transplantation. John Wiley and Sons Inc; 2022. p. 1275–6.
Kumar D, Ferreira VH, Hall VG, Hu Q, Samson R, Ku T, et al. Neutralization of SARS-CoV-2 variants in transplant recipients after two and three doses of mRNA-1273 vaccine. Ann Intern Med. 2022;175:226–33.
DeCuir J, Surie D, Zhu Y, Gaglani M, Ginde AA, Douin DJ, et al. Effectiveness of monovalent mRNA COVID-19 vaccination in preventing COVID-19–associated invasive mechanical ventilation and death among immunocompetent adults during the omicron variant period — IVY network, 19 U.S. States, February 1, 2022–January 31, 2023. MMWR Morb Mortal Wkly Rep. 2023;72:463–8.
The International Society of Heart & Lung Transplantation. COVID-19 vaccination and pre-exposure prophylaxis in heart and lung transplant candidates and recipients [Internet]. 2022 Oct. Available from: https://www.ishlt.org
Centers for Disease Control and Prevention. Ending isolation and precautions for people with COVID-19: interim guidance summary of recent changes [Internet]. 2022 Aug. Available from: https://www.cdc.gov/coronavirus/2019-ncov/hcp/duration-isolation.html#print
Organ Procurement and Transplantation Network. Summary of current evidence and information-donor SARS-CoV-2 testing & organ recovery from donors with a history of COVID-19 aim [Internet]. 2022 Aug. Available from: https://optn.transplant.hrsa.gov/media/kkhnlwah/sars-cov-2-summary-of-evidence.pdf
Kates OS, Fisher CE, Rakita RM, Reyes JD, Limaye AP. Use of SARS-CoV-2-infected deceased organ donors: should we always “just say no?” Am J Transplant. 2020;20:1787–94.
Kaul DR, Valesano AL, Petrie JG, Sagana R, Lyu D, Lin J, et al. Donor to recipient transmission of SARS-CoV-2 by lung transplantation despite negative donor upper respiratory tract testing. Am J Transplant. 2021;21:2885–9.
Koval CE, Poggio ED, Lin YC, Kerr H, Eltemamy M, Wee A. Early success transplanting kidneys from donors with new SARS-CoV-2 RNA positivity: a report of 10 cases. Am J Transplant. 2021;21:3743–9.
Kute VB, Godara S, Guleria S, Ray DS, Aziz F, Hegde U, et al. Is it safe to be transplanted from living donors who recovered from COVID-19? Experience of 31 kidney transplants in a multicenter cohort study from India. Transplantation. 2021;105:842–50.
La Hoz RM, Danziger-Isakov LA, Klassen DK, Michaels MG. Risk and reward: balancing safety and maximizing lung donors during the COVID-19 pandemic. Am J Transplant. 2021;21:2635–6.
Kumar D, Humar A, Keshavjee S, Cypel M. A call to routinely test lower respiratory tract samples for SARS-CoV-2 in lung donors. Am J Transplant. 2021;21:2623–4.
Purpura LJ, Chang M, Annavajhala MK, Mohri H, Liu L, Shah J, et al. Prolonged severe acute respiratory syndrome coronavirus 2 persistence, attenuated immunologic response, and viral evolution in a solid organ transplant patient. Am J Transplant. 2022;22:649–53.
Hettle D, Hutchings S, Muir P, Moran E. Persistent SARS-CoV-2 infection in immunocompromised patients facilitates rapid viral evolution: retrospective cohort study and literature review. Clin Infect Pract. 2022;16.
Kemp SA, Collier DA, Datir RP, Ferreira IATM, Gayed S, Jahun A, et al. SARS-CoV-2 evolution during treatment of chronic infection. Nature. 2021;592:277–82.
Martits-Chalangari K, Spak CW, Askar M, Killian A, Fisher TL, Atillasoy E, et al. ALVR109, an off-the-shelf partially HLA matched SARS-CoV-2-specific T cell therapy, to treat refractory severe COVID-19 pneumonia in a heart transplant patient: case report. Am J Transplant. 2022;22:1261–5.
Puhach O, Meyer B, Eckerle I. SARS-CoV-2 viral load and shedding kinetics. Nat Rev Microbiol. Nature Research; 2023. p. 147–61.
Rao R, Musante CJ, Allen R. A quantitative systems pharmacology model of the pathophysiology and treatment of COVID-19 predicts optimal timing of pharmacological interventions. NPJ Syst Biol Appl. 2023;9.
Author information
Authors and Affiliations
Contributions
KC and SKM wrote the main manuscript text with significant edits from RLG and TG; KC, RLG, LP, JW, MA prepared Tables 1-2; SKM prepared the figure. All authors reviewed and approved the manuscript.
Corresponding authors
Ethics declarations
Conflicts of Interest
Dr. Gottlieb has received consulting fees from AbbVie, Astra Zeneca, Eli Lilly, Gilead Sciences, GSK Pharmaceuticals, and Roche, all related to COVID-19. He has served on a steering committee for a Roivant Sciences COVID-19 clinical trial and acted as the coordinating investigator for a Johnson and Johnson COVID-19 clinical trial. Both roles are outside the scope of the current work. Additionally, Dr. Gottlieb has received a gift-in-kind for Baylor Scott and White Research Institute to support the unrelated academic-sponsored clinical trial (NCT03383419). Dr. Askar is a member of the scientific advisory board for One Lambda (a subsidiary of Thermo Fisher). Dr. Mathai reports participation as an investigator on a COVID-19 clinical trial funded by Foresee Pharmaceuticals (NCT04750278); she is an investigator on other trials outside the scope of this work funded by Boehringer Ingelheim, the National Institutes of Health, Cumberland Pharmaceuticals, ATyr Pharma, and the US Department of Defense. She has received fees for medical advisory activities for Natera and Verona Pharmaceuticals and for Data Safety and Monitoring Board participation from Mt. Sinai School of Medicine outside the scope of this work. Dr. Grazia reports receiving grants from Gates Grubstakes Grant (GGF014-18–13). He also reports associations with several other initiatives outside the scope of this work, including Renovion RVN-001—ARINA-1 in Bilateral Lung Transplant, Alberta Transplant Applied Genomics Center in collaboration with Natera, Sanofi, Pulmocide Ltd, and with Zambon Pharmaceuticals: BOSTON-1, BOSTON-2, and BOSTON-3. Additionally, Dr. Grazia holds a U.S. Patent (CU TTO File No. CU2425H-PPA1, U.S. Patent Application No. 13/394,625 issued) and a European Patent (covering Britain, France, and Germany; no. 2475379 issued). All other authors, namely, CAN, CWS, LP, JW, KC, KV, and TEY, have declared no relevant conflicts of interest concerning this manuscript.
Human and Animal Rights and Informed Consent
All reported studies/experiments with human or animal subjects performed by the authors have been previously published and complied with all applicable ethical standards (including the Helsinki Declaration and its amendments, institutional/national research committee standards, and international/national/institutional guidelines). The retrospective data was gathered via two existing retrospective protocols for lung transplant and SARS-CoV-2 approved by the Baylor Scott and White Research Institute Institutional Review Board.
Additional information
Publisher's Note
Springer Nature remains neutral with regard to jurisdictional claims in published maps and institutional affiliations.
Rights and permissions
Open Access This article is licensed under a Creative Commons Attribution 4.0 International License, which permits use, sharing, adaptation, distribution and reproduction in any medium or format, as long as you give appropriate credit to the original author(s) and the source, provide a link to the Creative Commons licence, and indicate if changes were made. The images or other third party material in this article are included in the article's Creative Commons licence, unless indicated otherwise in a credit line to the material. If material is not included in the article's Creative Commons licence and your intended use is not permitted by statutory regulation or exceeds the permitted use, you will need to obtain permission directly from the copyright holder. To view a copy of this licence, visit http://creativecommons.org/licenses/by/4.0/.
About this article
Cite this article
Castro, K., Naik, C.A., Spak, C.W. et al. Management of SARS-CoV-2 and Persistent Viral Detection in Solid Organ Transplant Recipients. Curr Pulmonol Rep 13, 26–37 (2024). https://doi.org/10.1007/s13665-024-00338-z
Accepted:
Published:
Issue Date:
DOI: https://doi.org/10.1007/s13665-024-00338-z