Abstract
Slow light devices with buffering capability play a critical role in all-optical signal processing. In this paper, multiple slow light phenomena are implemented based on plasmon-induced transparency (PIT) in our device. The device mainly consists of dual tooth cavities coupled with stub resonators, respectively. Temporal coupled-mode theory model illustrates that the triple PIT phenomena can be achieved based on different formation mechanisms. The simulation results calculated by the finite-difference time-domain method reveal that significant slow light response occurs at two wavelength regions. In addition, the parameters of structure have an important influence on PIT response and slow light characteristics. Moreover, the separate manipulation of wavelength, transmission and group index at transparency peak can be achieved in different slow light channels by adjusting the structural parameters. This plasmonic device is of great significance for the design of optical networks on chips.







Similar content being viewed by others
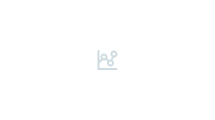
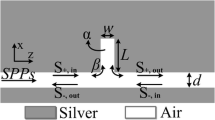
Data Availability
All data generated or analyzed during this study are included in this article.
References
Ye J, Wang F, Liang R, Wei Z, Meng H, Zhong J, Jiang L (2016) Plasmon induced transparency in loop-stub resonator-coupled waveguide systems. Opt Commun 370:36–42
Wang G, Zhang W, Gong Y, Liang J (2015) Tunable slow light based on plasmon-induced transparency in dual-stub-coupled waveguide. IEEE Photonics Technol Lett 27(1):89–92
Zafar R, Salim M (2015) Achievement of large normalized delay bandwidth product by exciting electromagnetic-induced transparency in plasmonic waveguide. IEEE J Quantum Electron 51(10):7200306
Li X, Xie R, Li W, Li Z, Gu E, Niu L, Guo S (2019) Adjustable electromagnetically induced transparency effect based on graphene surface plasmon. Superlattices Microstruct 128:342–348
Wang Q, Meng H, Huang B, Wang H, Zhang X, Yu W, Tan C, Huang X, Li S (2016) Dual coupled-resonator system for plasmon-induced transparency and slow light effect. Opt Commun 380:95–100
Lu H, Liu X, Mao D (2012) Plasmonic analog of electromagnetically induced transparency in multi-nanoresonator-coupled waveguide systems. Phys Rev A 85(5):053803
Liu D, Sun S, Yin X, Sun B, Sun J, Liu Y, Li W, Zhu N, Li M (2019) Large-capacity and low-loss integrated optical buffer. Opt Express 27(8):11585–11593
Liu W, Romeira B, Li M, Guzzon RS, Norberg EJ, Parker JS, Coldren LA, Yao JP (2016) A wavelength tunable optical buffer based on self-pulsation in an active microring resonator. J Lightwave Technol 34(14):3466–3472
Zhou L, Wang X, Lu L, Chen J (2018) Integrated optical delay lines: a review and perspective. Chin Opt Lett 16(10):101301
Wheeler NV, Light PS, Couny F, Benabid F (2010) Slow and superluminal light pulses via EIT in a 20-m acetylene-filled photonic microcell. J Lightwave Technol 28(6):870–875
Lukin MD, Imamoglu A (2001) Controlling photons using electromagnetically induced transparency. Nature 413(6853):273–276
Gu J, Singh R, Liu X, Zhang X, Ma Y, Zhang S, Maier SA, Tian Z, Azad AK, Chen HT, Taylor AJ, Han J, Zhang W (2012) Active control of electromagnetically induced transparency analogue in terahertz metamaterials. Nat Commun 3:1151
Shi X, Su X, Yang Y (2015) Enhanced tunability of plasmon induced transparency in graphene strips. J Appl Phys 117(14):143101
Chai Z, Hu X, Zhu Y, Sun S, Yang H, Gong Q (2014) Ultracompact chip-integrated electromagnetically induced transparency in a single plasmonic composite nanocavity. Adv Opt Mater 2(4):320–325
Yao G, Ling F, Yue J, Luo Q, Yao J (2016) Dynamically tunable graphene plasmon-induced transparency in the terahertz region. J Lightwave Technol 34(16):3937–3942
Xie Y, Ye Y, Liu Y, Wang S, Zhang J, Liu Y (2018) Synchronous slow and fast light based on plasmon-induced transparency and absorption in dual hexagonal ring resonators. IEEE Trans Nanotechnol 17(3):552–558
Zhang ZD, Wang RB, Zhang ZY, Tang J, Zhang WD, Xue CY, Yan SB (2017) Electromagnetically induced transparency and refractive index sensing for a plasmonic waveguide with a stub coupled ring resonator. Plasmonics 12(4):1007–1013
Barnes WL, Dereux A, Ebbesen TW (2003) Surface plasmon subwavelength optics. Nature 424:824–830
Armaghani S, Khani S, Danaie M (2019) Design of all-optical graphene switches based on a mach-zehnder interferometer employing optical kerr effect. Superlattices Microstruct 135:106244
Gramotnev DK, Bozhevolnyi SI (2010) Plasmonics beyond the diffraction limit. Nat Photonics 4(2):83–91
Genet C, Ebbesen TW (2007) Light in tiny holes. Nature 445(7123):39–46
Zhan S, Li H, Cao G, He Z, Li B, Yang H (2014) Slow light based on plasmon-induced transparency in dual-ring resonator-coupled MDM waveguide system. J Phys D: Appl Phys 47(20):205101
Huang B, Meng H, Wang Q, Wang H, Zhang X, Yu W, Tan C, Huang X, Wang F (2016) Plasmonic-induced transparency and slow-light effect based on stub waveguide with nanodisk resonator. Plasmonics 11(2):543–550
Yun B, Hu G, Cong J, Cui Y (2014) Plasmon induced transparency in metal-insulator-metal waveguide by a stub coupled with F-P resonator. Mater Res Express 1(3):036201
Zhang Z, Yang J, He X, Han Y, Zhang J, Huang J, Chen D, Xu S (2018) Plasmon-induced transparency based on aperture-coupled cascade resonators without gap. Superlattices Microstruct 123:138–143
Hosseini A, Massoud Y (2007) Nanoscale surface plasmon based resonator using rectangular geometry. Appl Phys Lett 90(18):181102
Rakhshani MR, Mansouri-Birjandi MA (2017) High sensitivity plasmonic refractive index sensing and its application for human blood group identification. Sens Actuators B 249:168–176
Zhai X, Wang L, Wang LL, Li XF, Huang WQ, Wen SC, Fan DY (2013) Tuning bandgap of a double-tooth-shaped MIM waveguide filter by control widths of the teeth. J Opt 15(5):055008
Wang G, Lu H, Liu X, Mao D, Duan L (2011) Tunable multi-channel wavelength demultiplexer based on MIM plasmonic nanodisk resonators at telecommunication regime. Opt Express 19(4):3513–3518
Han Z, Bozhevolnyi SI (2011) Plasmon-induced transparency with detuned ultracompact Fabry-Perot resonators in integrated plasmonic devices. Opt Express 19(4):3251–3257
Wang Y, Xie Y, Ye Y, Du Y, Liu B, Zheng W, Liu Y (2018) Exploring a novel approach to manipulating plasmon-induced transparency. Opt Commun 427:505–510
Feng Y, Liu Y, Wang X, Dong D, Shi Y, Hua S, Zhang H, Tang L (2016) Compact nanofilters based on plasmonics waveguide with archimedes’ spiral nanostructure. IEEE Photonics J 8(5):4802908
Lu H, Liu X, Gong Y, Mao D, Wang G (2011) Analysis of nanoplasmonic wavelength demultiplexing based on metal-insulator-metal waveguides. J Opt Soc Amer B 28(7):1616–1621
Lu H, Liu X, Wang G, Mao D (2012) Tunable high-channel-count bandpass plasmonic filters based on an analogue of electromagnetically induced transparency. Nanotechnol 23(44):444003
Funding
This work was supported by the Natural Science Foundation of Chongqing City under Grant cstc2016jcyjA0581, by the Postdoctoral Science Foundation of China under Grant 2016M590875, by the Fundamental Research Funds for the Central Universities under Grant XDJK2018B012.
Author information
Authors and Affiliations
Contributions
Yiyuan Xie contributed to conceptualization, supervision and writing—review. Junxiong Chai provided methodology and software and performed writing—original draft, and writing—editing. Yichen Ye, Tingting Song, Bocheng Liu, Liangyi Zhang, Yunchao Zhu and Yong Liu performed writing—review.
Corresponding author
Ethics declarations
Conflicts of Interest
The authors declare that they have no conflict of interest.
Consent to Participate
Informed consent was obtained from all participants.
Consent to Publish
Informed consent for publication was obtained from all authors.
Additional information
Publisher’s Note
Springer Nature remains neutral with regard to jurisdictional claims in published maps and institutional affiliations.
Rights and permissions
About this article
Cite this article
Xie, Y., Chai, J., Ye, Y. et al. A Tunable Slow Light Device with Multiple Channels Based on Plasmon-Induced Transparency. Plasmonics 16, 1809–1816 (2021). https://doi.org/10.1007/s11468-020-01367-5
Received:
Accepted:
Published:
Issue Date:
DOI: https://doi.org/10.1007/s11468-020-01367-5