Abstract
In order to obtain the reaction mechanism and thermodynamic parameters on tin oxide surface chemistry, which provide theoretical data for atomic layer deposition equipment optimization. The reaction mechanism of preparation of SnO2 pathway for TDMASn and H2O half reaction by atomic layer deposition (ALD) was studied by Density Function Theory. The study employed the average local ion energy (ALIE) and electrostatic potential (ESP) to predict potential reaction sites. These reaction sites were identified by investigating the ALIE extremes and the distribution of ESP intervals between the reactants. The results revealed that the primary reaction sites resided between the nitrogen (N) atoms within three distinct dimethylamino moieties and the outer region of tin (Sn) atoms. Transition state calculations were subsequently performed to ascertain the activation energies of the reaction steps, which resulted in TS1 (11.75 kcal mol−1), TS2 (7.16 kcal mol−1), TS3 (6.49 kcal mol−1), and TS4 (5.76 kcal mol−1). The interaction region indicator analysis was employed to verify the reaction mechanism, and the breakage and reformation of chemical bonds during the reaction were analyzed. The reaction rate constant (k) was calculated by the transition state theory. The Wigner tunneling factor also was used for correction to determine the reaction rate constants at both the standard temperature (298.15 K) and the reaction temperature (413.15 K). Meanwhile, the thermodynamic parameters of each reactant and product were calculated. These parameters can provide theoretical data to computational fluid dynamics model for the design and optimization of equipment for the preparation of SnO2 thin film using ALD.








Similar content being viewed by others
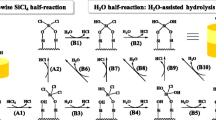
References
Fu QM, Peng JL, Yao ZC, Zhao HY, Ma ZB, Tao H, Tu YF, Tian Y et al (2020) Highly sensitive ultraviolet photodetectors based on ZnO/SnO2 core-shell nanorod arrays. Appl Sur Sci 527:146923. https://doi.org/10.1016/j.apsusc.2020.146923
Martinez-Gazoni RF, Allen MW, Reeves RJ (2018) Conductivity and transparency limits of Sb-doped SnO2 grown by molecular beam epitaxy. Phys Rev B 98:155308. https://doi.org/10.1103/PhysRevB.98.155308
Giraldi TR, Ribeiro C, Escote MT, Conti TG, Chiquito AJ, Leite ER, Longo E, Varela JA (2006) Deposition of controlled thickness ultrathin SnO2: Sb films by spin-coating. J Nanosci Nanotechnol 6:3849–3853. https://doi.org/10.1166/jnn.2006610
Dalapati GK, Sharma H, Guchhait A, Chakrabarty N, Bamola P, Liu Q, Saianand G, Krishna AMS et al (2021) Tin oxide for optoelectronic, photovoltaic and energy storage devices: a review. J Mater Chem A 9:16621–16684. https://doi.org/10.1039/D1TA01291F
Liu J, Zhang H, Li Y, Shen H, Ding Y, Su N, Shao L, Jin G et al (2021) Enhanced Vis-NIR light absorption and thickness effect of Mo-modified SnO2 thin films: a first principle calculation study. Results Phys 23:103997. https://doi.org/10.1016/j.rinp.2021.103997
Shen C, Yin Z, Collins F, Pinna N (2022) Atomic layer deposition of metal oxides and chalcogenides for high performance transistors. Adv Sci 9:2104599. https://doi.org/10.1002/advs.202104599
Zhao C, Tan C, Lien DH, Song X, Amani M, Hettick M, Nyein HYY, Yuan Z et al (2020) Evaporated tellurium thin films for p-type field-effect transistors and circuits. Nat Nanotechnol 15:53–58. https://doi.org/10.1038/s41565-019-0585-9
Huang S, Li P, Wang J, Huang JC, Xue Q, Fu N (2022) Modification of SnO2 electron transport layer: Brilliant strategies to make perovskite solar cells stronger. Chem Eng J 439:135687. https://doi.org/10.1016/j.cej.2022.135687
Johnson RW, Hultqvist A, Bent SF (2014) A brief review of atomic layer deposition: from fundamentals to applications. Mater Today 17:236–246. https://doi.org/10.1016/j.mattod.2014.04.026
George SM (2010) Atomic layer deposition: an overview. ACS Chem Rev 110:111–131. https://doi.org/10.1021/cr900056b
Raza A, Clemmen S, Wuytens P, Muneeb M, Daele MV, Dendooven J, Detavernier C, Skirtach A et al (2018) ALD assisted nanoplasmonic slot waveguide for on-chip enhanced Raman spectroscopy. APL Photonics 3:116105. https://doi.org/10.1063/1.5048266
Li MJ, Breeden M, Wang V, Linn NMK, Winter CH, Kummel A, Bakir MS (2021) Characterization of low-temperature selective cobalt atomic layer deposition (ALD) for chip bonding. IEEE Int Interconnect Technol Conf. https://doi.org/10.1109/IITC51362.2021.9537353
Rönn J, Zhang W, Autere A, Lerous X, Pakarinen L, Ramos CA, Säynätjoki A, Lipsanen H (2019) Ultra-high on-chip optical gain in erbium-based hybrid slot waveguides. Nat Commun 10:1–9. https://doi.org/10.1038/s41467-019-08369-w
Sheng J, Lee J, Choi W, Hong T, Kim M, Seong J (2018) Atomic layer deposition for oxide semiconductor thin film transistors: advances in research and development. J Vac Sci Technol A 36:060801. https://doi.org/10.1116/1.5047237
Du X, Du Y, George SM (2005) In situ examination of tin oxide atomic layer deposition using quartz crystal microbalance and fourier transform infrared techniques. J Vac Sci Technol A 23:581–588. https://doi.org/10.1116/1.1914810
Tokoro K, Saito S, Kanomata K, Miura M, Ahmmad B, Kubota S, Hirose F (2018) Room-temperature atomic layer deposition of SnO2 using tetramethyltin and its application to TFT fabrication. IEICE Trans Electron 101:317–322. https://doi.org/10.1587/transele.E101.C.317
Choi DW, Park JS (2014) Highly conductive SnO2 thin films deposited by atomic layer deposition using tetrakis-dimethyl-amine-tin precursor and ozone reactant. Surf Coat Technol 259:238–243. https://doi.org/10.1016/j.surfcoat.2014.02.012
Nwanna EC, Imoisili PE, Jen TC (2022) Synthesis and characterization of SnO2 thin films using metal organic precursors. J King Saud Univ Sci. https://doi.org/10.1016/j.jksus.2022.102123
Tanskanen JT, Bent SF (2013) Insights into the surface chemistry of tin oxide atomic layer deposition from quantum chemical calculations. ACS J Phys Chem C 117:19056–19062. https://doi.org/10.1021/jp4063324
Rohnacher V, Ullrich F, Eggers H, Schackmar F, Hell S, Salazar A, Huck C, Hernandez-Sosa G (2021) Analytical study of solution-processed tin oxide as electron transport layer in printed perovskite solar cells. Adv Mater Technol 6:2000282. https://doi.org/10.1002/admt.202000282
Lee DK, Wan Z, Bae JS, Lee HBR, Ahn JH, Kim SD, Kim J, Kwon SH (2016) Plasma-enhanced atomic layer deposition of SnO2 thin films using SnCl4 and O2 plasma. Mater Lett 166:163–166. https://doi.org/10.1016/j.matlet.2015.12.049
Hirose F, Tokoro K, Kanomata K, Miura M, Ahmmad B, Kubota S (2016) Infrared study of room temperature atomic layer deposition of SnO2 using Sn(CH3)4 and plasma excited humidified argon. ECS Meet Abstr. https://doi.org/10.1149/MA2016-02/28/1875
Elam JW, Baker DA, Hryn AJ, Martinson ABF, Pellin MJ, Hupp JT (2008) Atomic layer deposition of tin oxide films using tetrakis (dimethylamino) tin. J Vac Sci Technol A 26:244–252. https://doi.org/10.1116/1.2835087
Baena JPC, Steier L, Tress W, Saliba M, Neutzner S, Matsui T, Giordano F, Jacobsson TJ (2015) Highly efficient planar perovskite solar cells through band alignment engineering. Energy Environ Sci 8:2928–2934. https://doi.org/10.1039/C5EE02608C
Gu Y, Tao JJ, Wang T, Liu YH, Peng BF, Zhu LY, Lu HL (2021) Nonlinear growth of zinc tin oxide thin films prepared by atomic layer deposition. Ceram Int 47:22760–22767. https://doi.org/10.1016/j.ceramint.2021.04.294
Gong SH, Lim HJ, Lee JH, Yoo Y, Yu S, Lim HD, Jung HW, Ko JS et al (2023) Electrochemical assessment of highly reversible SnO2–coated Zn metal anodes prepared via atomic layer deposition for aqueous Zn-ion batteries. Appl Sur Sci 611:155633. https://doi.org/10.1016/j.apsusc.2022.155633
Li Y, Janik MJ (2019) Recent progress on first-principles simulations of voltammograms. Curr Opin Electrochem 14:124–132. https://doi.org/10.1016/j.coelec.2019.01.005
Car R, Parrinello M (1985) Unified approach for molecular dynamics and density-functional theory. Phys Rev Lett 55:2471. https://doi.org/10.1103/PhysRevLett.55.2471
Han JK, Song DS, Lim YR, Agbenyeke RE, Castelli IE, Esposito V, Kim SY, Bu SD et al (2021) Nonlinear photoelectric properties by strained MoS2 and SnO2 core-shell nanotubes for flexible visible light photodetectors. Adv Mater Technol 6:2001105. https://doi.org/10.1002/admt.202001105
Lee JH, Yoo M, Kang DH, Lee HM, Choi W, Park JW, Yi Y, Kim HY et al (2018) Selective SnOx atomic layer deposition driven by oxygen reactants. ACS Appl Mater Interfaces 10:33335–33342. https://doi.org/10.1021/acsami.8b12251
Nguyen VH, Akbari M, Sekkat A, Ta HTT, Resende J, Jiménezet C, Musselman KP, Rojas DM (2022) Atmospheric atomic layer deposition of SnO2 thin films with tin (II) acetylacetonate and water. Dalton Trans 51:9278–9290. https://doi.org/10.1039/D2DT01427K
Han JH, Kim DY, Lee S, Yang HL, Park BH, Park JS (2021) A study on the growth mechanism and gas diffusion barrier property of homogeneously mixed silicon–tin oxide by atomic layer deposition. Ceram Int 47:34774–34782. https://doi.org/10.1016/j.ceramint.2021.09.016
Frisch MJ, Trucks GW, Schlegel HB, Scuseria GE, Robb MA, Cheeseman JR, Scalmani G, Barone V, et al. (2016) Gaussian, Inc., Wallingford CT, 2016
Pritchard BP, Altarawy D, Didier B, Gibson TD, Windus TL (2019) New basis set exchange: an open, up-to-date resource for the molecular sciences community. ACS J Chem Inf Model 59:4814–4820. https://doi.org/10.1021/acs.jcim.9b00725
Yanai T, Tew DP, Handy NC (2004) A new hybrid exchange–correlation functional using the Coulomb-attenuating method (CAM-B3LYP). Chem Phys Lett 393:51–57. https://doi.org/10.1016/j.cplett.2004.06.011
Tomasi J, Mennucci B, Cammi R (2005) Quantum mechanical continuum solvation models. Chem Rev 105:2999–3094. https://doi.org/10.1021/cr9904009
Kargar H, Fallah-Mehrjardi M, Behjatmanesh-Ardakani R, Bahadori M, Moghadam M, Ashfaq M, Munawar KS, Tahir MN (2022) Spectroscopic investigation, molecular structure, catalytic activity with computational studies of a novel Pd (II) complex incorporating unsymmetrical tetradentate Schiff base ligand. Inorg Chem Commun 142:109697. https://doi.org/10.1016/j.inoche.2022.109697
Kulichenko M, Fedik N, Boldyrev A, Muñoz-Castro A (2020) Expansion of magnetic aromaticity criteria to multilayer structures: magnetic response and spherical aromaticity of matryoshka-like cluster [Sn@Cu12@Sn20]12−. Chem Eur J 26:2263–2268. https://doi.org/10.1002/chem.201905088
Lu T, Chen Q (2021) Interaction region indicator: a simple real space function clearly revealing both chemical bonds and weak interactions. Chem Methods 1:231–239. https://doi.org/10.1002/cmtd.202100007
Murray JS, Politzer P (2011) The electrostatic potential: an overview. Wiley Interdiscip. Rev.: Comput. Mol Sci 1:153–163. https://doi.org/10.1002/wcms.19
Politzer P, Murray JS (2002) The fundamental nature and role of the electrostatic potential in atoms and molecules. Theor Chem Acc 108:134–142. https://doi.org/10.1007/s00214-002-0363-9
Canneaux S, Bohr F, Henon E (2014) KiSThelP: a program to predict thermodynamic properties and rate constants from quantum chemistry results. J Comput Chem 35:82–93. https://doi.org/10.1002/jcc.23470
Fang M, Wu K, Ma X, Yao X, Guo Y, Yu L, Wu Q, Zeng Z et al (2022) Alkali metals modified porous carbon for enhanced methanol and acetone selective adsorption: a theoretical study. Appl Surf Sci 602:154271. https://doi.org/10.1016/j.apsusc.2022.154271
Lu T, Chen F (2012) Multiwfn: a multifunctional wavefunction analyzer. J Comput Chem 33:580–592
Lu T, Chen Q (2018) Revealing molecular electronic structure via analysis of valence electron density. Acta Phys Chim Sin 34:503–513. https://doi.org/10.3866/PKU.WHXB201709252
Lu T, Manzetti S (2014) Wavefunction and reactivity study of benzo [a] pyrene diol epoxide and its enantiomeric forms. Struct Chem 25:1521–1533. https://doi.org/10.1007/s11224-014-0430-6
Humphrey W, Dalke A, Schulten K (1996) VMD: visual molecular dynamics. J Mol Graphics 14:33–38. https://doi.org/10.1016/0263-7855(96)00018-5
Politzer P, Murray JS, Bulat FA (2010) Average local ionization energy: a review. J Mol Model 16:1731–1742. https://doi.org/10.1007/s00894-010-0709-5
Lu T, Chen F (2012) Quantitative analysis of molecular surface based on improved Marching Tetrahedra algorithm. J Mol Graphics Modell 38:314–323. https://doi.org/10.1016/j.jmgm.2012.07.004
Eyring H (1935) The activated complex in chemical reactions. J Chem Phys 3:107–115. https://doi.org/10.1063/1.1749604
Eyring H (1935) The activated complex and the absolute rate of chemical reactions. ACS Chem Rev 17:65–77. https://doi.org/10.1021/cr60056a006
Wynne-Jones WFK, Eyring H (1935) The absolute rate of reactions in condensed phases. J Chem Phys 3:492–502. https://doi.org/10.1016/B978-0-08-012344-8.50008-8
Merrick JP, Moran D, Radom L (2007) An evaluation of harmonic vibrational frequency scale factors. ACS J Phys Chem A 111:11683–11700. https://doi.org/10.1021/jp073974n
Garrett BC, Truhlar DG (1979) Accuracy of tunneling corrections to transition state theory for thermal rate constants of atom transfer reactions. J Phys Chem 83:200–203. https://doi.org/10.1021/j100464a026
Wigner E (1937) Calculation of the rate of elementary association reactions. J Chem Phys 5:720–725. https://doi.org/10.1063/1.1750107
Qiu L, Xiao H, Gong X, Ju X, Zhu W (2007) Crystal density predictions for nitramines based on quantum chemistry. J Hazard Mater 141:280–288. https://doi.org/10.1016/j.jhazmat.2006.06.135
Rice BM, Hare JJ, Byrd EFC (2007) Accurate predictions of crystal densities using quantum mechanical molecular volumes. ACS J Phys Chem A 111:10874–10879. https://doi.org/10.1021/jp073117j
Mortazavi B, Javvaji B, Shojaei F, Rabczuk T, Shapeev AV, Zhuang X (2021) Exceptional piezoelectricity, high thermal conductivity and stiffness and promising photocatalysis in two-dimensional MoSi2N4 family confirmed by first-principles. Nano Energy 82:105716. https://doi.org/10.1016/j.nanoen.2020.105716
Mortazavi B, Silani M, Podryabinkin EV, Rabczuk T, Zhuang X, Shapeev AV (2021) First-principles multiscale modeling of mechanical properties in graphene/borophene heterostructures empowered by machine-learning interatomic potentials. Adv Mater 33:2102807. https://doi.org/10.1002/adma.202102807
Acknowledgements
This work was supported by National Key Research and Development Program of China (No. 2022YFB3404401) and National Natural Science Foundation of China (No. 51975594). This work was supported in part by the High Performance Computing Center of Central South University.
Author information
Authors and Affiliations
Contributions
PW contributed to investigation, modeling calculation, and writing of the original draft. YW was involved in the investigation and conceptualization. TW and JX contributed to analysis and revision. WX and YP were involved in the resources and analysis. JL contributed to revision, resources, and project administration.
Corresponding author
Ethics declarations
Conflict of interest
The authors declare that they have no known competing financial interests or personal relationships that could have appeared to influence the work reported in this paper.
Additional information
Handling Editor: Pedro Camargo.
Publisher's Note
Springer Nature remains neutral with regard to jurisdictional claims in published maps and institutional affiliations.
Rights and permissions
Springer Nature or its licensor (e.g. a society or other partner) holds exclusive rights to this article under a publishing agreement with the author(s) or other rightsholder(s); author self-archiving of the accepted manuscript version of this article is solely governed by the terms of such publishing agreement and applicable law.
About this article
Cite this article
Wang, P., Wu, Y., Wu, T. et al. Theoretical study on tin oxide surface chemistry mechanism and thermodynamic properties for atomic layer deposition equipment optimization. J Mater Sci 58, 15634–15646 (2023). https://doi.org/10.1007/s10853-023-09007-z
Received:
Accepted:
Published:
Issue Date:
DOI: https://doi.org/10.1007/s10853-023-09007-z