Abstract
The formation of silaspiropentane from addition of singlet silacyclopropylidene 1 and silacyclopropylidenoid 8 to ethylene has been investigated separately at the B3LYP, X3LYP, WB97XD, and M05–2X theories using the 6–31+G(d,p) basis set. The silacycloproylidenoid addition follows a stepwise route. In contrast, a concerted mechanism occurs for silacyclopropylidene addition. Moreover, the intramolecular rearrangements of silaspiropentane 9 to methylenesilacyclobutane 11 and 2-silaallene + ethylene 12 have been studied extensively. The required energy barrier for the isomerization of 9 to 10 was determined to be 44.0 kcal mol−1 at the B3LYP/6–31+G(d,p) level. After formation of 10, the rearrangement to methylenesilacyclobutane 12 is highly exergonic by −15.9 kcal mol−1, which makes this reaction promising. However, the conversion of 9 to 11 is calculated to be quite endergonic, by 26.5 kcal mol−1.


Similar content being viewed by others
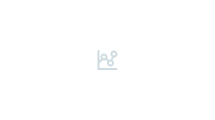
References
Gaspar P, West R (1998) The chemistry of organic silicon compounds. In: Rappoport Z, Apeloig Y (eds) Silylenes. Wiley, New York, pp 2463–2568
West R (1989) The chemistry of organic silicon compounds. In: Patai S, Rappoport Z (eds) Polysilanes. Wiley, Chichester, pp 1207–1240
Miller RD, Michl J (1989) Polysilane high polymers. Chem Rev 89:1359–1410. doi:10.1021/cr00096a006
Lee VY, Sekiguchi A (2011) Novel organometallic reagents: geminal dianionic derivatives of the heavy group 14 elements. Inorg Chem 50:12303–12314. doi:10.1021/ic2006106
Qi Y, Geng B, Chen Z (2012) The insertion reactions of the p–complex silylenoid H 2SiLiF with Si–X (X = F, Cl, Br, O, N) bonds. J Mol Model 18:1015–1021. doi:10.1007/s00894-011-1129-x
Weidenbruch M (2006) A stable silylenoid and a donor–stabilized chlorosilylene: low–coordinate silicon compounds—a never-ending story? Angew Chem Int Ed 45:4241–4242. doi:10.1002/anie.200601414
Clark T, Schleyer PR (1980) The isomeric structures of SiH2LiF. J Organomet Chem 191:347–353. doi:10.1016/S0022-328X(00)81063-3
Lee M, Hyeon M, Lim Y, Choi J, Park C, Jeong S, Lee U (2004) Syntheses and reactivities of stable halosilylenoids, (Tsi)X2SiLi (Tsi = C(SiMe3)3, X = Br, Cl). Chem Eur J 10:377–381. doi:10.1002/chem.200305151
Molev G, Bravo–Zhivotovakii D, Karni M, Tumanskii B, Botoshansky M, Apeloig Y (2006) Synthesis, molecular structure, and reactivity of the isolable silylenoid with a tricoordinate silicon. J Am Chem Soc 128:2784–2785. doi:10.1021/ja0575880
Tokitoh N, Hatano K, Sadahiro T, Okazaki R (1999) Generation and reactions of an overcrowded diaryldilithiosilane. Chem Lett 28:931–932. doi:10.1246/cl.1999.931
Hatano K, Tokitoh N, Takagi N, Nagase S (2000) The first stable heteracyclopropabenzene: synthesis and crystal structure of a silacyclopropabenzene. J Am Chem Soc 122:4829–4830. doi:10.1021/ja000501k
Feng DC, Feng SY, Deng CH (1996) A theoretical study on the addition reaction of silylenoid H2SiLiF and ethylene. Chem J Chin Univ 17:1108–1111
Zhang M, Li W, Li O, Cheng J (2015) A new exploration of the addition reaction of the silylenoid H2SiLiF with ethylene. J Mol Model 21:202–207. doi:10.1007/s00894-015-2753-7
Nefedow O, Manakow M (1964) Entstehung und reaktionen des dimethylsilylens, eines siliciumanalogons der carbene. Angew Chem 76:270–271. doi:10.1002/ange.19640760605
Gilman H, Peterson D (1965) Reaction of diphenyldichlorosilane with lithium. J Am Chem Soc 87:2389–2394. doi:10.1021/ja01089a016
Qi YH, Ma J, Xu CJ, Geng B, He MX (2014) Computational investigations on the electronic and structural properties of the unsaturated silylenoid HP = SiLiF. J Mol Model 20:2213–2218. doi:10.1007/s00894-014-2213-9
Flock M, Marschner C (2005) Silyl anions or silylenoids?—A DFT study of silyllithium compounds with π-donating substituents. Chem Eur J 11:4635–4642. doi:10.1002/chem.200401353
Xie J, Feng DC, Feng SY (2007) Theoretical study on the substitution reactions of silylenoid H2SiLiF with CH4, NH3, H2O, HF, SiH4, PH3, H2S, and HCl. J Phys Chem A 111:8475–8481. doi:10.1021/jp070773a
Li WZ, Yan BF, Xiao CP, Li QZ, Cheng JB (2014) Novel formation of silicon–germanium bond: Insertion reactions of H2SiLiF with GeH3X (X = F, Cl, Br). J Org Chem 750:112–116. doi:10.1016/j.jorganchem.2013.11.018
Yildiz CB, Azizoglu A (2016) Substituent effects on the ring–opening mechanism of gem–dibromospiropentanes to related allenes: a theoretical study. J Phys Org Chem 29:63–68. doi:10.1002/poc.3487
Azizoglu A, Balci M, Mieusset JL, Brinker UH (2008) Substituent effect on the ring opening mechanism of lithium bromocyclopropylidenoids to allenes. J Org Chem 73:8182–8186. doi:10.1021/jo8011144
Christl M, Brauen M, Fischer H, Groetsch S, Müller G, Leusser D, Deurlein S, Stalke D, Arnone M, Engels B (2006) The stereochemical course of the generation and interception of a six-membered cyclic allene: 3δ2-1H-Naphthalene (2,3-Didehydro-1,2-dihydronaphthalene). Eur J Org Chem 2006:5045–5058. doi:10.1002/ejoc.200600443
Azizoglu A, Demirkol O, Kilic T, Yildiz YK (2007) Incorporatian of an allene unit into 1, 4-dihydronapthaline: generation of 1,2-benzo-1,4,5-cycloheptatriene and its dimerization. Tetrahedron 63:2409–2413. doi:10.1016/j.tet.2007.01.017
Sedenkova KN, Averina EB, Grishin YK, Rybakov VB, Kuznetzova TS, Zefirov NS (2010) Cationic carbenoid rearrangement of 2-phenyl substituted gem-dihalogenospiropentanes. Eur J Org Chem 2010:4145–4150. doi:10.1002/ejoc.201000427
Sigal N, Apeloig Y (2001) Are disilacyclopropylidenes and their carbenoids good precursors for the unknown 1,3–disilaallenes? J Organomet Chem 636:148–156. doi:10.1016/S0022-328X(01)00996-2
Azizoglu A, Yildiz CB (2010) Ring–opening mechanism of lithium bromosilacyclopropylidenoids to silaallenes. Organometallics 29:6739–6743. doi:10.1021/om100868b
Azizoglu A, Yildiz CB (2012) Ring-opening mechanism of disilacyclopropylidenoids and trisilacyclopropylidenoid: a theoretical study. J Organomet Chem 715:19–25. doi:10.1016/j.jorganchem.2012.05.007
Yildiz CB, Azizoglu A (2012) Theoretical study on the structures and stabilities of silacyclopropylidenoids. Struct Chem 33:1777–1784. doi:10.1007/s11224-012-9981-6
Baldwin JE, Fleming RH (1973) Thermal rearrangements of methylenecyclobutanes. Degenerate rearrangement of optically active 1-(z)-(1-deuterioethylidene)-2-methyl-trans-3,4,4-trideuteriocyclobutane. J Am Chem Soc 95:5261–5266. doi:10.1021/ja00797a028
Gajewski JJ, Burka LT (1971) Stereochemistry of and alteration in the mechanism of the spiropentane to methylenecyclobutane thermal rearrangement by polar substituents. J Am Chem Soc 93:4952–4953. doi:10.1021/ja00748a072
Carpenter BK, Pittner J, Veis L (2009) Ab initio calculations on the formation and rearrangement of spiropentane. J Phys Chem A 113:10557–10563. doi:10.1021/jp905368b
Su KJ, Mieusset JL, Arion VB, Knoll W, Brecker L, Brinker UH (2010) Efforts toward distorted spiropentanes. J Org Chem 75:7494–7497. doi:10.1021/jo1017298
Maki A, Price JE, Harzan J, Nibler JW, Weber A, Masiello T, Blake TA (2015) Analysis of several high–resolution infrared bands of spiropentane, C5H8. J Mol Spectrosc 312:68–77. doi:10.1016/j.jms.2015.03.013
Frey H, Jackson GE, Smith RA, Walsh R (1975) Reaction of singlet methylene with methylenecyclopropane. Part 1. Evidence for multistep collisional deactivation of chemically activated spiropentane. J Chem Soc Faraday Trans 71:1991–2006. doi:10.1039/F19757101991
Gordon MS, Boudjouk P (1985) Molecular and electronic structures of metallaspiropentanes. J Am Chem Soc 107:1439–1440. doi:10.1021/ja00291a070
Skancke PN (1994) Fluoride affinities of some cyclic silanes. A theoretical study. J Phys Chem 98:3154–3160. doi:10.1021/j100063a018
Maier G, Reisenauer HP, Egenolf H (1998) Reaction of silicon atoms with acetylene and ethylene: generation and matrix–spectroscopic identification of C2H2Si and C2H4Si isomers. Eur J Org Chem 7:1313–1317. doi:10.1002/(SICI)1099-0690(199807)1998:7
Frisch MJ, Trucks GW, Schlegel HB, Scuseria GE, Robb MA, Cheeseman JR, Montgomery JA Jr, Vreven T, Kudin KN, Burant JC, Millam JM, Iyengar SS, Tomasi J, Barone V, Mennucci B, Cossi M, Scalmani G, Rega N, Petersson GA, Nakatsuji H, Hada M, Ehara M, Toyota K, Fukuda R, Hasegawa J, Ishida M, Nakajima T, Honda Y, Kitao O, Nakai H, Klene M, Li X, Knox JE, Hratchian HP, Cross JB, Adamo C, Jaramillo J, Gomperts R, Stratmann RE, Yazyev O, Austin AJ, Cammi R, Pomelli C, Ochterski JW, Ayala PY, Morokuma K, Voth GA, Salvador P, Dannenberg JJ, Zakrzewsk VG, Dapprich S, Daniels AD, Strain MC, Farkas O, Malick DK, Rabuck AD, Raghavachari K, Foresman JB, Ortiz JV, Cui Q, Baboul AG, Clifford S, Cioslowski J, Stefanov BB, Liu G, Liashenko A, Piskorz P, Komaromi I, Martin RL, Fox DJ, Keith T, Al–Laham MA, Peng CY, Nanayakkara A, Challacombe M, Gill PMW, Johnson B, Chen W, Wong MW, Gonzalez C, Pittsburgh PA, Pople JA (2009) Gaussian 09, revision A02. Gaussian Inc, Wallingford
Becke AD (1993) Density‐functional thermochemistry. III. The role of exact exchange. J Chem Phys 98:5648–5652. doi:10.1063/1.464913
Lee C, Yang W, Parr RG (1998) Development of the Colle-Salvetti correlation-energy formula into a functional of the electron density. Phys Rev B 37:785–789. doi:10.1103/PhysRevB.37.785
Xu X, Goddard WA III (2004) The X3LYP extended density functional for accurate descriptions of nonbond interactions, spin states, and thermochemical properties. Proc Natl Acad Sci USA 101:2673–2677. doi:10.1073/pnas.0308730100
Chai JD, Head–Gordon M (2008) Long–range corrected hybrid density functionals with damped atom–atom dispersion corrections. Phys Chem Chem Phys 10:6615–6620. doi:10.1039/B810189B
Zhao Y, Schultz NE, Truhlar DG (2006) Design of density functionals by combining the method of constraint satisfaction with parametrization for thermochemistry, thermochemical kinetics, and noncovalent interactions. J Chem Theory Comput 2:364–382. doi:10.1021/ct0502763
Anslyn EV, Dougherty DA (2006) Modern physical organic chemistry. University Science Books, Sausalito, pp 461–463
Ruff F, Csizmadia IG (1994) Organic reactions: equilibria, kinetics and mechanism. Elsevier, Amsterdam, pp 290–291
Fukui K (1981) The path of chemical reactions—the IRC approach. Acc Chem Res 14:363–368. doi:10.1021/ar00072a001
Gonzalez C, Schlegel HB (1991) Improved algorithms for reaction path following: higher‐order implicit algorithms. J Chem Phys 95:5853–5860. doi:10.1063/1.461606
Wiberg KB (1968) Application of the pople–santry–segal CNDO method to the cyclopropylcarbinyl and cyclobutyl cation and to bicyclobutane. Tetrahedron 24:1083–1096. doi:10.1016/0040-4020(68)88057-3
Dennington RII, Keith T, Millam J, Eppinnett K, Hovell WL, Gilliland R (2009) GaussView v.5.0.9 Visualizer and Builder. Gaussian 09. Wallingford, CT
Gillbert JC (1969) Geometric isomerization of spiropentane. Tetrahedron 7:1459–1466. doi:10.1016/S0040-4020(01)82717-4
Acknowledgments
Financial support by the Aksaray University (BAP2016–024) is gratefully acknowledged. We are very grateful to the reviewers for valuable comments that improved our manuscript.
Author information
Authors and Affiliations
Corresponding author
Electronic supplementary material
Below is the link to the electronic supplementary material.
ESM 1
(DOCX 583 kb)
Rights and permissions
About this article
Cite this article
Yildiz, C.B., Azizoglu, A. A mechanistic investigation on the formation and rearrangement of silaspiropentane: A theoretical study. J Mol Model 22, 158 (2016). https://doi.org/10.1007/s00894-016-3016-y
Received:
Accepted:
Published:
DOI: https://doi.org/10.1007/s00894-016-3016-y