- 1Key Laboratory of Livestock Infectious Diseases in Northeast China, Ministry of Education, College of Animal Science and Veterinary Medicine, Shenyang Agricultural University, Shenyang, China
- 2Tecon Biological Co. Ltd., Urumqi, China
- 3Beijing Advanced Innovation Center for Soft Matter Science and Engineering, Beijing University of Chemical Technology, Beijing, China
- 4Brucellosis Prevention and Treatment Engineering Technology Research Center of Inner Mongolia Autonomous Region, Inner Mongolia University for Nationalities, Tongliao, China
- 5School of Public Health, Sun Yat-sen University, Guangzhou, China
Brucella abortus is an important zoonotic pathogen that causes severe economic loss to husbandry and poses a threat to human health. The B. abortus A19 live vaccine has been extensively used to prevent bovine brucellosis in China. However, it is difficult to distinguish the serological response induced by A19 from that induced by natural infection. In this study, a novel genetically marked vaccine, A19ΔvirB12, was generated and evaluated. The results indicated that A19ΔvirB12 was able to provide effective protection against B. abortus 2308 (S2308) challenge in mice. Furthermore, the safety and protective efficacy of A19ΔvirB12 have been confirmed in natural host cattle. Additionally, the VirB12 protein allowed for serological differentiation between the S2308 challenge/natural infection and A19ΔvirB12 vaccination. However, previous studies have found that the accuracy of the serological detection based on VirB12 needs to be improved. Therefore, we attempted to identify potential supplementary antigens with differential diagnostic functions by combining label-free quantitative proteomics and protein chip technology. Twenty-six proteins identified only in S2308 were screened; among them, five proteins were considered as potential supplementary antigens. Thus, the accuracy of the differential diagnosis between A19ΔvirB12 immunization and field infection may be improved through multi-antigen detection. In addition, we explored the possible attenuation factors of Brucella vaccine strain. Nine virulence factors were downregulated in A19ΔvirB12. The downregulation pathways of A19ΔvirB12 were significantly enriched in quorum sensing, ATP-binding cassette transporter, and metabolism. Several proteins related to cell division were significantly downregulated, while some proteins involved in transcription were upregulated in S2308. In conclusion, our results contribute to the control and eradication of brucellosis and provide insights into the mechanisms underlying the attenuation of A19ΔvirB12.
Introduction
Brucellosis is an important reemerging zoonosis that causes tremendous economic losses in animal husbandry and presents a significant risk to public health. The pathogen of brucellosis is Brucella, a small gram-negative coccobacillus lacking spores. Several Brucella species have been found to be pathogenic to humans, including B. melitensis, B. abortus, B. suis, and B. canis. Transmission can occur through direct contact with body fluids of infected animals, ingestion of contaminated dairy products, and aerosol (1–3). In the absence of an effective vaccine protecting humans from Brucella infection, the research and development of animal vaccines will be beneficial to both animals and humans (4, 5).
The B. abortus 2308 strain A19 vaccine has been widely employed in China and offers a high level of protection for cattle. However, the antibody response induced by the O-side chain of the A19 vaccine interferes with serological diagnosis, which hardly distinguishes between vaccinated and infected animals (6). Undoubtedly, this shortcoming of traditional vaccines impairs efforts to control and eradicate infectious diseases. Fortunately, the development and application of gene-deleted vaccines can overcome this limitation (7, 8). It is well known that the use of gene-deleted vaccines, such as gE-null marker strains, has led to great progress in the eradication of Pseudorabies virus in some areas (9).
Therefore, we generated the genetically marked vaccine A19ΔvirB12 by knocking out virB12 gene in this study. The VirB12 protein, a component of the type IV secretion system (T4SS), has been shown to be a potential marker for the serological diagnosis of brucellosis (10). The results indicated that A19ΔvirB12 has similar virulence to that of its parent strain in mice. Immunization of mice with A19ΔvirB12 conferred protection against challenge with the virulent B. abortus 2308 (S2308). Furthermore, the safety and protective efficacy of A19ΔvirB12 were confirmed in cattle. VirB12-based iELISA can differentiate the vaccination from S2308 challenge/natural infections. However, previous studies have shown that the sensitivity and specificity of serological detection based on VirB12 needs to be improved (10, 11). In this regard, new antigens should be identified and combined with VirB12 to improve the accuracy of differential diagnosis. Proteomics has become an indispensable tool for comparing the differences of protein expression profiles between virulent and attenuated strains (12–14). Moreover, protein microarrays are an effective method for discovering antigen-specific antibody responses and identifying novel antigens. The two methods were combined in this study to screen for supplementary differential diagnosis antigens. In addition, based on the difference of proteomics between A19ΔvirB12 and S2308, we explored factors that may lead to significant differences in virulence between wild-type and vaccine strains.
Materials and Methods
Ethics Statement
BALB/c female mice (age, 7 weeks) were acquired from Tecon Biological Co., Ltd. (License number: SCXK2003-0002, Urumqi, China) and were housed in microisolator cages containing wood shavings in laminar flow racks. Mice inoculated with live attenuated Brucella vaccine strains were maintained under biosafety level-2 (BSL-2) containment and those inoculated with S2308 were maintained under BSL-3 containment. All mice were acclimated to the facility for 5 days prior to vaccination or infection, and were maintained on a 12-h/12-h light-dark cycle with free access to food and filtered water. Female Xinjiang Brown cattle (age, 3-8 months) with no prior infection with Brucella were selected for analysis in this study and housed in an outdoor and restricted access isolation facility. One to two weeks prior to the challenge, the cattle were transferred to a BSL-3 containment facility, where they were housed throughout the study duration. All animal experiments were strictly performed in accordance with the Experimental Animal Regulation Ordinances (2017) formulated by the China National Science and Technology Commission. The protocol was approved by the Committee on Ethics and Welfare of Experimental Animals of Tecon biological Co., Ltd.
Cells, Plasmids and Bacterial Strains
Escherichia coli (E. coil) DH5α and BL21 (DE3) cells were purchased from Transgen (Beijing, China). The plasmid pUC19-SacB was obtained from the Changchun Veterinary Research Institute (Changchun, China). The plasmid pET-28a was purchased from Transgen (Beijing, China). The virulent strains of S2308 and B. melitensis M28, and A19 vaccine strain were obtained from Tecon Biological Co., Ltd. (Urumqi, China). The A19ΔvirB12 mutant was constructed as previously described, with some modifications (15). Briefly, the upstream and downstream sequences of virb12 were amplified from S2308 genomic DNA. Subsequently, the two products were ligated to one another via overlapping PCR, and the ligated product was inserted into pUC19-SacB to generate the pUC19-SacB-ΔvirB12 plasmid. Competent A19 was subjected to electroporation with pUC19-SacB-ΔvirB12, and then A19ΔvirB12 was isolated, identified, and used in this study.
Evaluation of Virulence of A19ΔvirB12 Mutant in BALB/c Mice
Groups of female BALB/c mice (age, 7 weeks; n=4 per group) were injected intraperitoneally (i.p.) with 5.0×105 colony formation units (CFU) of A19 or A19ΔvirB12 in 0.2 ml of phosphate-buffered saline (PBS; pH 7.2). The PBS-inoculated group served as a control. At 2, 4, 6, 8, and 10 weeks post-infection (wpi), mice were sacrificed, the spleens were removed, weighed, and then homogenized. Tissue homogenates were serially diluted with PBS, plated on tryptic soy agar (Becton, Dickinson and Company, USA) and incubated for 3 to 5 days at 37°C. The number of CFUs per spleen was measured.
Protective Efficacy Evaluation and Cytokine Response Detection in BALB/C Mice
To evaluate the protective efficacy of A19ΔvirB12, groups of four female BALB/c mice were inoculated i.p. with 5.0 x 105 CFU A19ΔvirB12 or 5.0 x 105 CFU A19. Mice inoculated with PBS served as control. At 9 weeks post-vaccination (wpv), the mice were challenged i.p. with 5.0 x 105 CFU (200 µl) of virulent S2308 per mouse. Three weeks after virulent challenge, all mice were euthanized by CO2 asphyxiation, and the spleens were collected and weighed. In addition, the levels of gamma interferon (IFN-γ) and interleukin-4 (IL-4) in mice serum were determined at 2, 4, 6, 8, 10 wpv and 3 weeks post-challenge(wpc) using the mouse IL-4 ELISA kit and the IFN-γ ELISA kit, according to the manufacturer’s instructions (Cloud-Clone, Corp., USA). Briefly, the ELISA plate had been pre-coated with antibodies specific to IL-4/IFN-γ. Standards or samples were then added to the wells with a biotin-conjugated antibody specific to IL-4/IFN-γ. Then, Avidin conjugated to Horseradish Peroxidase was added to each well and incubated. Subsequently, TMB substrate solution was added and the reaction was terminated by the addition of sulphuric acid solution. The concentration of IL-4/IFN-γ was then measured according to the standard curve.
Evaluation of A19ΔvirB12 in Cattle
Groups of 12 female Xinjiang Brown cattle (age, 3-8 months) with no prior infection with Brucella were vaccinated subcutaneously with 6.0×1010 CFU of A19ΔvirB12 or 6.0×1010 A19. Control unimmunized cattle were injected with equivalent PBS. The rectal temperature of all the cattle was measured at the same time before and after immunization. At 5 weeks post-immunization, groups of five cattle were intraconjuctivally challenged with B. melitensis M28 at 109 CFU (50 μl of inoculum per eye). Sixty days later, the cattle were euthanized and their submandibular lymph nodes, anterior shoulder lymph nodes, inguinal lymph nodes, and spleen were removed (0.3-0.5g) and washed with sterile PBS 3-5 times. The weighed tissues were placed into a sterile tube with two steel balls and 1 ml PBS, and then homogenized. The tissue homogenate (100 μl) was spread on Brucella selective medium dish and incubated in a 37°C incubator under 5% CO2 for 3-5 days. The Brucella genus-specific gene bcsp31-based PCR method (16) was used to detect the suspected Brucella colonies on the petri dish. The positive colonies amplified via bcsp31-PCR were further analyzed through classical AMOS-PCR, which was previously developed to identify and differentiate specific Brucella species. The typical B. melitensis yielded a specific band of 731 bp, and B. abortus yielded a specific band of 498 bp (17). To evaluate the safety of A19ΔvirB12 in pregnant cattle, A19ΔvirB12 immunization was carried out at the end of May in three local experimental pastures, and the calving situation of pregnant cattle was recorded in detail from March to November in the same year. The pregnancy status of the cattle was determined through rectal palpation.
VirB12-Based iELISA in BALB/c Mice and Cattle
The recombinant protein VirB12 was produced as previously described with some modifications (15). Open reading frames (ORFs) of VirB12 were amplified through PCR using S2308 as a template (14). Subsequently, the amplified DNA fragments were cloned into the pET-28a vector and expressed in E. coli BL21 (DE3) cells (Transgen, Beijing). The recombinant protein VirB12 was confirmed through SDS-PAGE and western blotting. Then, the recombinant protein VirB12 was purified using affinity chromatography. The concentration of purified proteins was determined using a BCA kit (Thermo Fisher Scientific, Waltham, MA, USA) according to the manufacturer’s instructions. After determining the concentration, VirB12 served as coating antigen for indirect enzyme-linked immunosorbent assay (iELISA). Serum samples used for antibody detection were obtained from mice inoculated with S2308 or A19ΔvirB12, and cattle with natural infection or A19ΔvirB12 vaccination. The iELISA procedure was carried out as previously described (18).
Collection of Sera and Fabrication of Protein Chip
Nine female Xinjiang Brown cattle (age, 3-8 months) were randomly assigned to either the immunized group (n=4) or the challenged group (n=5). The immunized group was subcutaneously injected with 6.0×1010 CFU of A19ΔvirB12, while the challenged group was intraconjuctivally inoculated with 1.0×109 of S2308 (50 μl of inoculum per eye). Immunized sera were collected at 7, 14, 21, 28, 35, 42, 49, 56, and 63 days post-vaccination, and challenged sera were collected at 7, 14, 21, and 45 days post-infection.
The fabrication of the protein chip was performed as previously described with some modifications. Briefly, the ORFs of selected proteins were obtained using GenBank sequences NC_007618.1"> and NC_007624.1"> and amplified using gene-specific primers containing a 33 bp “adapter” sequence, which is homologous to the cloning sites of the linearized pXT7 vector. The genomic DNA of S2308 served as a template, and PCR products were visualized via agarose gel electrophoresis. A mixture containing linearized pXT7 vector and PCR-generated ORF fragment was transformed into E. coli DH5α cells and incubated overnight at 37°C. Subsequently, the recombinant plasmids were extracted using a QIAprep 96 Turbo Kit (Qiagen). The recombinant plasmids were expressed in E. coli using an in vitro transcription-translation (IVTT) reaction system (RTS Kit, Roche) according to the manufacturer’s instructions. The reaction of the negative control was performed in the absence of DNA. The reaction solution was printed onto nitrocellulose coated glass FAST slides. Slides were blocked for 30 min and then incubated with diluted serum samples at 4°C overnight. The slides were then washed and incubated with labeled secondary antibodies. The slides were scanned using a confocal laser, and the signal intensity of individual spots was quantified using QuantArray software.
Protein Sample Preparation for Mass Spectrometry (MS) Analysis
Whole cell protein samples of virulent S2308 and A19ΔvirB12 strain were used for proteome analysis. All operations related to live Brucella were carried out in BSL-3 labs, and each sample had 3 biological replicates. Protein samples were prepared as described previously with some modifications (19). Briefly, bacteria were cultured to logarithmic and stationary phases. The logarithmic and stationary phase of the virulent S2308 were named Logw and Staw, respectively, while the logarithmic and stationary-phase A19ΔvirB12 vaccine strain were named Logv and Stav, respectively. The pH values of all culture media were adjusted to 7.0. Cells were harvested and centrifuged at 7,000×g for 15 min at 4°C and washed three times with sterile PBS buffer. The bacteria were resuspended in lysis buffer and lysed by ultrasonication. The remaining debris were removed by centrifugation at 1,2000×g for 30 min at 4°C, and then the supernatant was collected and stored at −80°C before trypsin digestion.
The BCA kit (Thermo Fisher Scientific, Waltham, USA) was used to measure protein concentration. Dithiothreitol (DDT) was added as a reductant to a final concentration of 5 mM and the sample was incubated at 56°C for 30 min. After reduction, the solution was alkylated with 11 mM iodoacetamide for 15 min at room temperature in the dark. Trypsin was added to the alkylated protein solution at a ratio of 1:50 (trypsin: protein, w/w) at 37°C overnight. Finally, trypsin was added at a ratio of 1:100 (trypsin: protein, w/w) for another 4 h.
HPLC Separation and LC–MS/MS Analysis
Peptides produced by trypsin digestion were separated by high pH reversed-phase HPLC using an Agilent 300 Extend C18 column (5 μm particle size, 4.6 mm ID, 250 mm length). Peptides were fractionated with a gradient of 2% to 60% acetonitrile under alkaline conditions (pH =9.0) into 60 fractions. These fractions were then merged into four fractions and dried under vacuum.
Peptides were dissolved using buffer A (containing 0.1% formic acid and 2% acetonitrile) and then separated via EASY-nLC 1200 ultra HPLC system. The gradient comprised an increase from 8% to 16% of buffer B (0.1% formic acid in 98% acetonitrile) for approximately 30 min, 18% to 32% for 25 min, and to 80% in 2 min, and hold at 80% for the last 3 min. The flow rate was maintained at 400 nl/min. After separation, peptides were subjected to NSI source and then analyzed using tandem MS/MS in Q ExactiveTM Plus (Thermo Fisher Scientific, Waltham, USA). The electrospray voltage applied was set to 2.0 kV. The m/z scan range of the full scan was set from 350 to 1,800, and intact peptides were detected in the Orbitrap with a resolution of 70,000. Peptides were selected for MS/MS using the NCE setting 28 and the fragments were detected in the Orbitrap with a resolution of 17,500. A data-dependent procedure that alternated between one MS scan followed by 20 MS/MS scans with 15.0s dynamic exclusion. Automatic gain control was set at 5E4. The fixed first mass was set as 100 m/z.
Database Searching
A Maxquant search engine (v.1.5.2.8) was employed for processing the resulting MS/MS data. Tandem mass spectra were searched against the UniProt B. abortus (3,023 sequences) concatenated with a reverse decoy database. Trypsin/P was designated as the cleavage enzyme, allowing up to 2 missing cleavages. The mass tolerance for precursor ions was set as 20 ppm in the first search and 5 ppm in the main search, and the mass tolerance for fragment ions was set as 0.02 Da. Alkylation of cysteine was set as a fixed modification and variable modifications were oxidation of methionine, acetylation of the N-terminal of protein, and deamidation of asparagine. The FDR was adjusted to <1%.
Bioinformatics Analyses
Functional annotation of proteins was carried out using various bioinformatics procedures, including Gene Ontology (GO), Kyoto Encyclopedia of Genes and Genomes (KEGG) and The Clusters of Orthologous Groups (COG). GO annotation proteome was derived from the UniProt-GOA database (http://www.ebi.ac.uk/GOA/). Firstly, the identified protein ID was converted to UniProt ID and then mapped to GO IDs by protein ID. If the identified proteins were not annotated by UniProt-GOA database, the InterProScan soft would be used to annotated protein’s GO functional based on protein sequence alignment method. KEGG connects known information on molecular interaction networks (20). Firstly, the protein’s KEGG database description was annotated using KEGG online service tools KAAS. Then the annotation result was mapped to the KEGG pathway database using KEGG online service tools KEGG mapper. COG (http://www.ncbi.nlm.nih.gov/COG/) system software program was used to determine the functional distribution of proteins (21).
Statistical Analysis
Statistical analysis was performed using GraphPad Prism 7.0 (GraphPad Software, San Diego, CA, USA) and SPSS software (version 22.0, IBM, Armonk, NY, USA). Statistical analysis was performed by a two-tailed Student’s t test, Fisher’s exact test or Mann-Whitney U test; a P-value less than 0.05 was considered statistically significant.
Results
Evaluation of Virulence and Immune Responses of A19ΔvirB12 in Mice
To evaluate the virulence of A19ΔvirB12 in vivo, BALB/c mice were infected with A19ΔvirB12 or its parental strain A19. As shown in Figure 1A, there was no significant difference in colonization levels in the spleen between A19ΔvirB12 and A19 (P>0.05) at 2, 4, 6, 8, or 10 wpi. The change of spleen weight in infected mice is closely related to the virulence of Brucella and the level of inflammatory response (22). Mice injected with A19ΔvirB12 showed significantly increased spleen weights compared with mice inoculated with A19 at 4 and 10 wpi (Figure 1B). Nevertheless, spleen weight tended to be normal in mice injected with A19ΔvirB12 or A19.
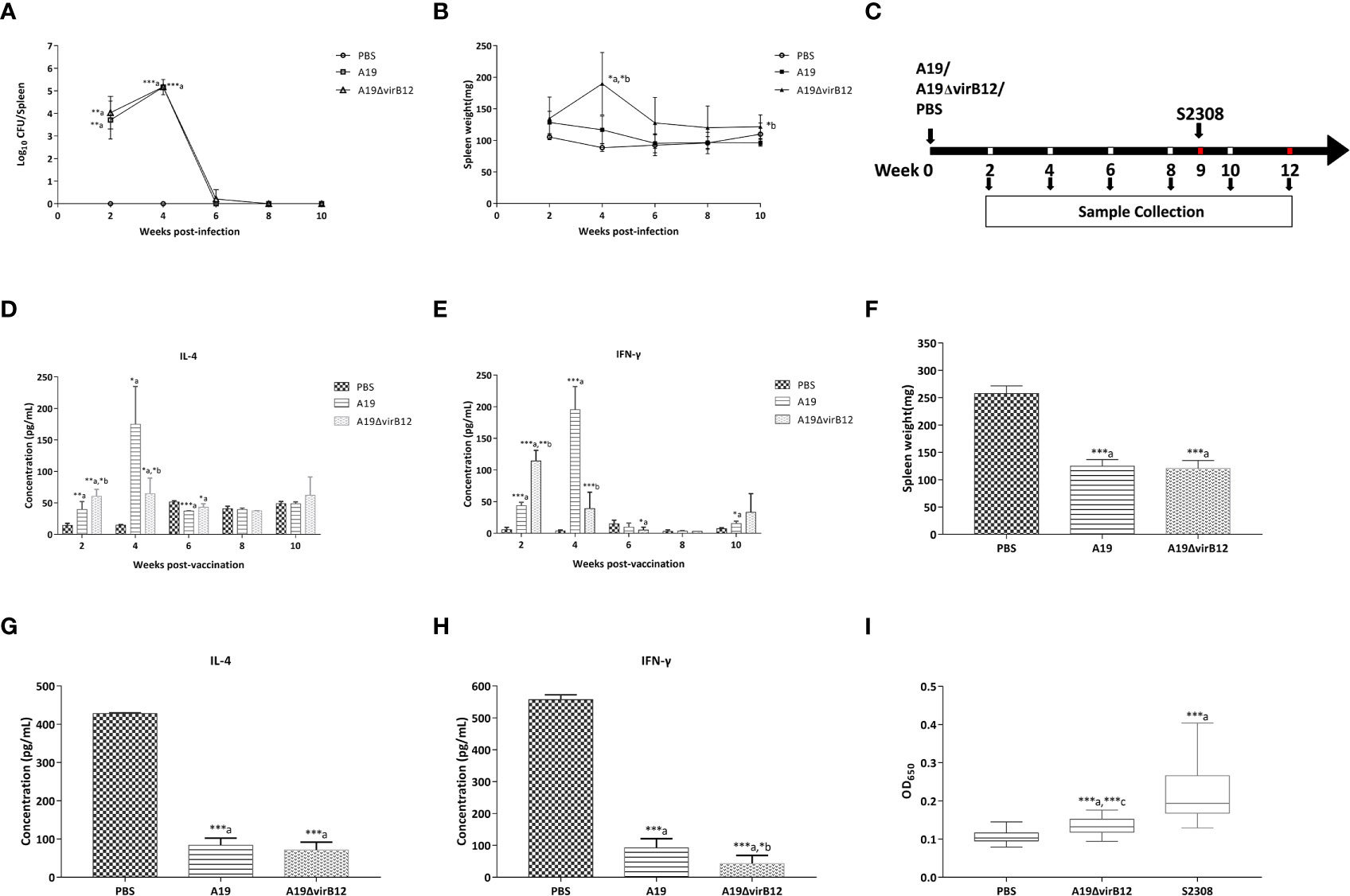
Figure 1 Characteristics of A19ΔvirB12 vaccination. To determine the virulence of A19ΔvirB12, female BALB/c mice were i.p. injected with 5 × 105 CFU of A19ΔvirB12, A19, or PBS. Colonization levels (A) and spleen weights (B) were assessed at 2, 4, 6, 8, and 10 weeks post-infection. To determine the protective efficacy of immunization with A19ΔvirB12, female BALB/c mice were immunized i.p. with 5 × 105 CFU of A19ΔvirB12 or A19 while the control group received 0.2 ml of PBS (C). At 9 weeks post-vaccination, mice (n=5 per group) were challenged with 5 × 105 CFU of virulent S2308 (C). The levels of IL-4 (D) and IFN-γ (E) were measured at 2, 4, 6, 8, and 10 weeks post-vaccination. At 3 weeks post-challenge, the spleen weight (F), and the levels of IL-4 (G) and IFN-γ (H) were measured. Immune response of VirB12 to A19ΔvirB12-immunized sera and challenged sera was shown in the (I). Values was expressed as the means ± standard deviation (SD). Differences were determined by the two-tailed Student's t test or Mann-Whitney U test. The letter a in the (A–I) indicates statistically significant differences between A19 or A19ΔvirB12 and the PBS group; The letter b in the (A–H) indicates statistically significant differences between A19 and A19ΔvirB12; The letter c in the (I) indicates statistically significant differences between A19ΔvirB12 and S2308 (*P < 0.05; **P < 0.01; ***P < 0.001).
In order to determine the protection efficiency of A19ΔvirB12, the mice were vaccinated i.p. with A19ΔvirB12 or A19, and the PBS-inoculated group served as the control (Figure 1C). At 9 wpv, the mice were challenged i.p. with S2308 (Figure 1C). Compared with the PBS-inoculated group, the levels of IL-4 in A19 and A19ΔvirB12 vaccinated groups were significantly increased at 2,4 and 6 wpv (P<0.05; Figure 1D). As for IFN-γ, we obtained results similar to those of IL-4 at 2 and 4 wpv (Figure 1E). These results indicated that vaccination with the strain A19ΔvirB12 can induce obvious cellular immune response in mice. As shown in Figure 1F, mice immunized with A19ΔvirB12 or A19 exhibited lighter spleen weights compared with the PBS-inoculated group at 3 wpc (P<0.05). In addition, the concentrations of IL-4 and IFN-γ in mice vaccinated with A19ΔvirB12 or A19 were significantly lower than those of the PBS-inoculated control group (P<0.5; Figures 1G, H). These results indicated that compared to the PBS-inoculated group, the immunized mice may eliminate the wild-type strains more quickly. In addition, we compared the cytokine levels and spleen weights of mice at 3 wpc with those at 10 wpv (Table S1). The levels of IL-4 and IFN-γ in the A19-inoculation group at 3 wpc were higher than those at 10 wpv (P<0.05). In contrast, the cytokine levels and spleen weights of the A19ΔvirB12-immunized group at 3 wpc were similar to those at 10 wpv. Therefore, mice immunized with A19ΔvirB12 could recover to the pre-challenge level more quickly after challenge, suggesting that A19ΔvirB12 may have a better protective effect than A19 in mice.
Evaluation of Differential Diagnosis Using VirB12 as a Test Antigen in Mice
To determine whether the VirB12 protein can be used as a diagnostic marker antigen, iELISA was employed to detect antibodies in A19ΔvirB12- and S2308-inoculated mice sera using the recombinant purified protein VirB12 as the coating antigen. As shown in Figure 1I, the levels of antibodies against VirB12 in the sera of B. abortus 2308-inoculated mice were significantly higher than those of A19ΔvirB12-inoculated mice (P<0.05). Therefore, VirB12 may be used to differentiate vaccination from a natural infection.
Evaluation of A19ΔvirB12 in Cattle and Field Application
The results of mice experiments indicated that A19ΔvirB12 is a potential live attenuated vaccine with a differential diagnostic function. Subsequently, the safety and protective efficacy of A19ΔvirB12 in cattle were evaluated. After vaccination, the temperature of the cattle fluctuated within the normal temperature range (38-40°C; Figure 2A). At 1 and 3 days post-immunization, there was a slight increase in body temperature of the immunized groups. In general, the body temperature of cattle increased after vaccination, but soon returned to the pre-immunization level. The most dominant Brucella species in China was B. melitensis, which is the important pathogen responsible for sheep and cow abortion (23, 24). Thus, A19-immunized and A19ΔvirB12-immunized cattle were challenged with virulent B. melitensis M28 at 35 days post-immunization to evaluate the protective efficacy. At 60 days post-challenge, the clearance of M28 within the submandibular lymph nodes, anterior shoulder lymph nodes, inguinal lymph nodes, and spleen was evaluated. Compared with the control group, M28 was effectively eliminated in the A19ΔvirB12-immunized group (Table 1), suggesting that A19ΔvirB12 immunization can protect cattle against wild-type infection. In addition, the safety of A19ΔvirB12 was evaluated in large-scale pregnant cattle. Previous epidemiological studies have shown that the occurrence of cattle abortion is caused by multiple factors (25). In the same pasture, the occurrence of cattle abortion is mainly related to seasonal variation. Winter is the season with the lowest incidence of cattle abortion, and the incidence begins to increase in March and reaches a peak in summer (26, 27). In this study, we also observed an extremely high incidence of cattle abortion in spring. Fortunately, A19ΔvirB12 vaccination did not cause deterioration (Figure 2B), which may have played a role in curbing the increase in the incidence of cattle abortion, indicating that A19ΔvirB12 is safe in pregnant cattle. Similarly, the sera of naturally infected cattle and A19ΔvirB12-immunized cattle were analyzed through ELISA using VirB12 as a coating antigen. The results showed that the level of anti-VirB12 antibody in naturally infected cattle serum was significantly higher than that in A19ΔvirB12-immunized serum (Figure 2C). Therefore, the differential diagnostic function of the A19ΔvirB12 vaccine is supported by data from both mice and cattle.
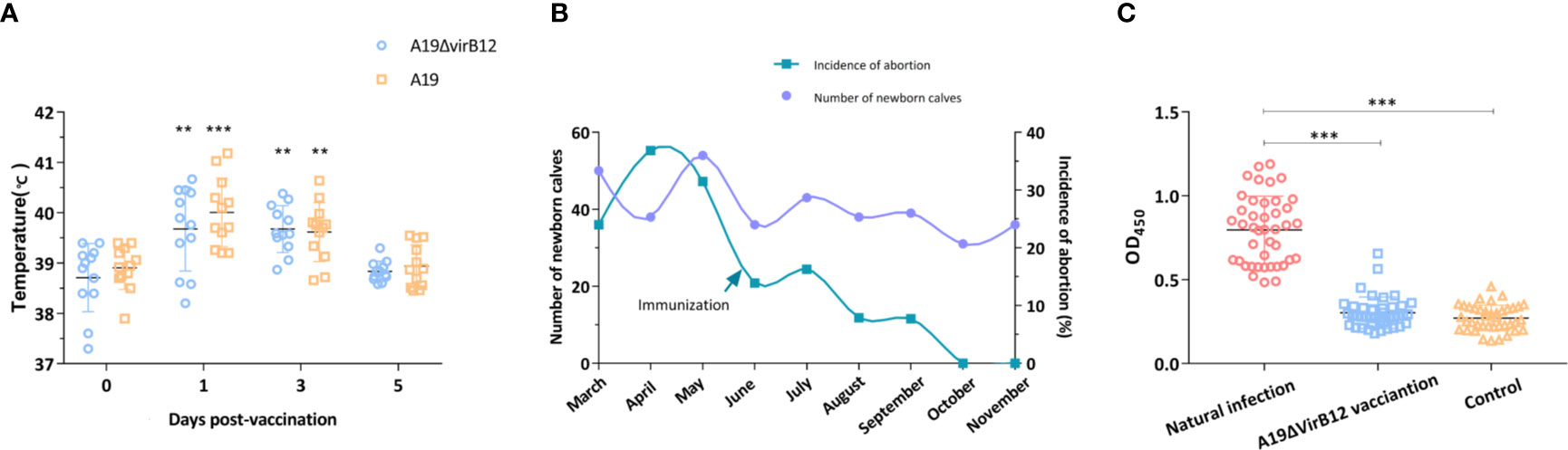
Figure 2 Evaluation of A19ΔvirB12 vaccination in cattle. (A) Changes of body temperature after immunization. (B) Incidence of cattle abortion by month before and after A19ΔvirB12 immunization. (C) Immune response of VirB12 to sera with A19ΔvirB12 immunization or natural infection. The arrow in (B) represents the time point of A19ΔvirB12 immunization. * in the (A) indicates statistically significant differences between the groups of A19ΔvirB12/A19 vaccination and pre-vaccination. Differences were determined by the two-tailed Student’s t test or Mann-Whitney U test (**P < 0.01; ***P < 0.001).
Overview of Proteomics and Screening of Differentially Expressed Proteins Involved in Virulence
In order to discover specific proteins of S2308, a label-free proteomics approach was used to compared the protein expression profiles of S2308 and A19ΔvirB12. The screening process of target antigens is shown in Figure S1. First, whole proteins were extracted from A19ΔvirB12 and S2308 in the logarithmic and stationary phases, digested with trypsin, followed by LC–MS/MS analysis. In total, 2185 proteins were identified, of which 1999 were quantifiable (Table S2). The proteins were divided into two groups for analysis: Log w/v (Logw vs Logv) and Sta w/v (Staw vs Stav). With ratios of 1.50 and 0.67 as the cutoffs for differential up and downregulated expression, the expression levels of 154 proteins were found to increase in the logarithmic-phase S2308, while 121 proteins showed a decrease. In the stationary period, 66 upregulated proteins and 59 downregulated proteins were observed in S2308 (Table S3). The analysis of COG and GO for the DEPs is shown in Figure S2, Tables S4 and S5.
Brucella has high pathogenicity to human and animals; however, spontaneous attenuated strains have low virulence and could provide immune protection for vaccinated animals against natural infection (28). In this study, we compared the protein expression profiles of S2308 and A19ΔvirB12 in order to explore the potential molecular mechanisms causing the virulence attenuation. We screened the upregulated virulence factors identified in S2308 using the Victors database (http://www.phidias.us/victors), an online database for virulence factors of pathogens (29). We observed that 8 and 3 virulence-related proteins were downregulated in the logarithmic and stationary phase of A19ΔvirB12, respectively (Table 2). Subcellular localization showed that most of these virulence proteins were located in the cytoplasm, followed by the cytoplasmic membrane. The results of COG functional analysis showed that these virulence proteins were mainly related to amino acid metabolism, transport, and transcription, followed by transcription. Both VjbR (encoded by vjbR) and urease subunit alpha 2(ureC2) were downregulated during the growth of A19ΔvirB12.
KEGG Enrichment of DEPs
The KEGG enrichment results of DEPs in the log w/v and Sta w/v groups are shown in Table 3. The upregulated pathways in the log w/v group were mainly enriched for ATP-binding cassette (ABC) transporters, quorum sensing (QS), and metabolism of multiple substances. The important role of ABC transporters and QS system in microbial virulence has been revealed (30, 31). In addition, there is also an important link between metabolism and virulence in Brucella (32). As for stationary-phase DEPs, the upregulated DEPs were also found to be enriched in the metabolism of multiple substances, including branched chain amino acids (BCAA; valine, leucine, and isoleucine), tryptophan, atrazine, and propanoate. The downregulated DEPs were found to be enriched in amino acid biosynthesis. This suggests that there are differences in degradation and synthesis of substances between the two strains in the stationary phase.
Potential Supplementary Antigens for Differential Diagnosis
To find supplementary differential diagnostic antigens, we searched for proteins that were expressed only in S2308. A total of 26 proteins were identified and they are listed in Table S6. These proteins are located on the cytoplasmic membrane (10 proteins) or cytoplasm (9). Notably, three of them were T4SS proteins, including VirB4 (virB4), VirB9 (virB9), and VirB11 (virB11). We speculate that the absence of VirB12 may implicate the expression of other T4SS proteins. Subsequently, these specific proteins were constructed into protein arrays and interacted with cattle sera. The L7/L12(rplL) protein, known as Brucella antigen (33) was employed as a positive control, which showed no difference in the expression between the two strains in this study. Compared with immunized sera, a total of five proteins produced a stronger response intensity to challenged sera (P<0.05; Figure 3). The lower IgG responses of immunized sera suggested that these five proteins may have the potential for differential diagnosis between A19ΔvirB12 vaccination and natural infection. Subsequently, the IgG antibody kinetics of the cattle sera against these proteins were analyzed (Figure 4). The heat map showed directly that the challenged sera induced an obvious IgG response against these proteins, compared with the immunized sera. However, there seemed to be no regularity in the response of challenged sera to these proteins at different time points.
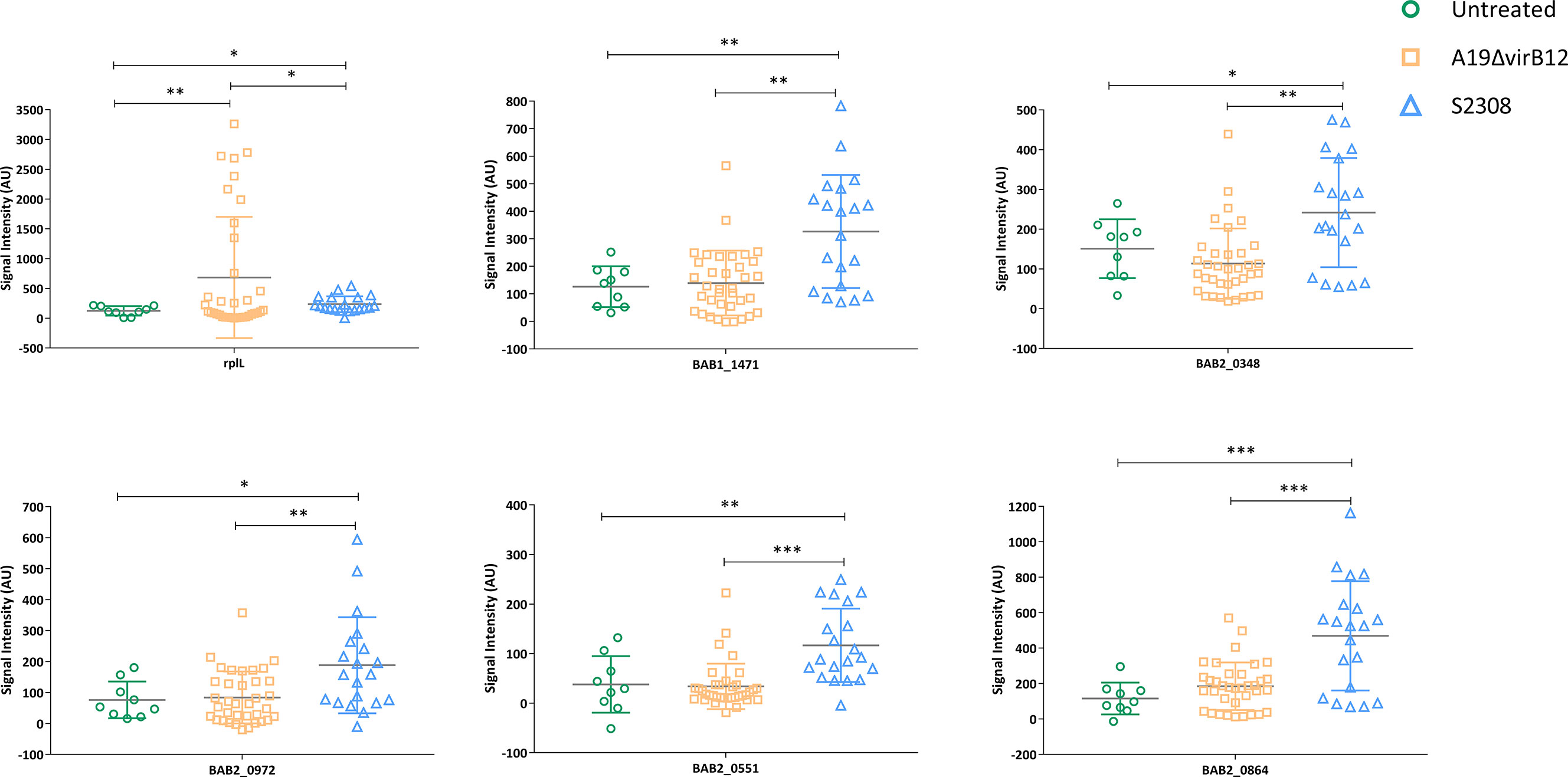
Figure 3 IgG response of 5 proteins obtained from sera of cattle inoculated with A19ΔvirB12 or S2308. Values were expressed as the means ± (SD). Differences were determined by two-tailed Student’s t test (*P < 0.05; **P < 0.01; ***P < 0.001).
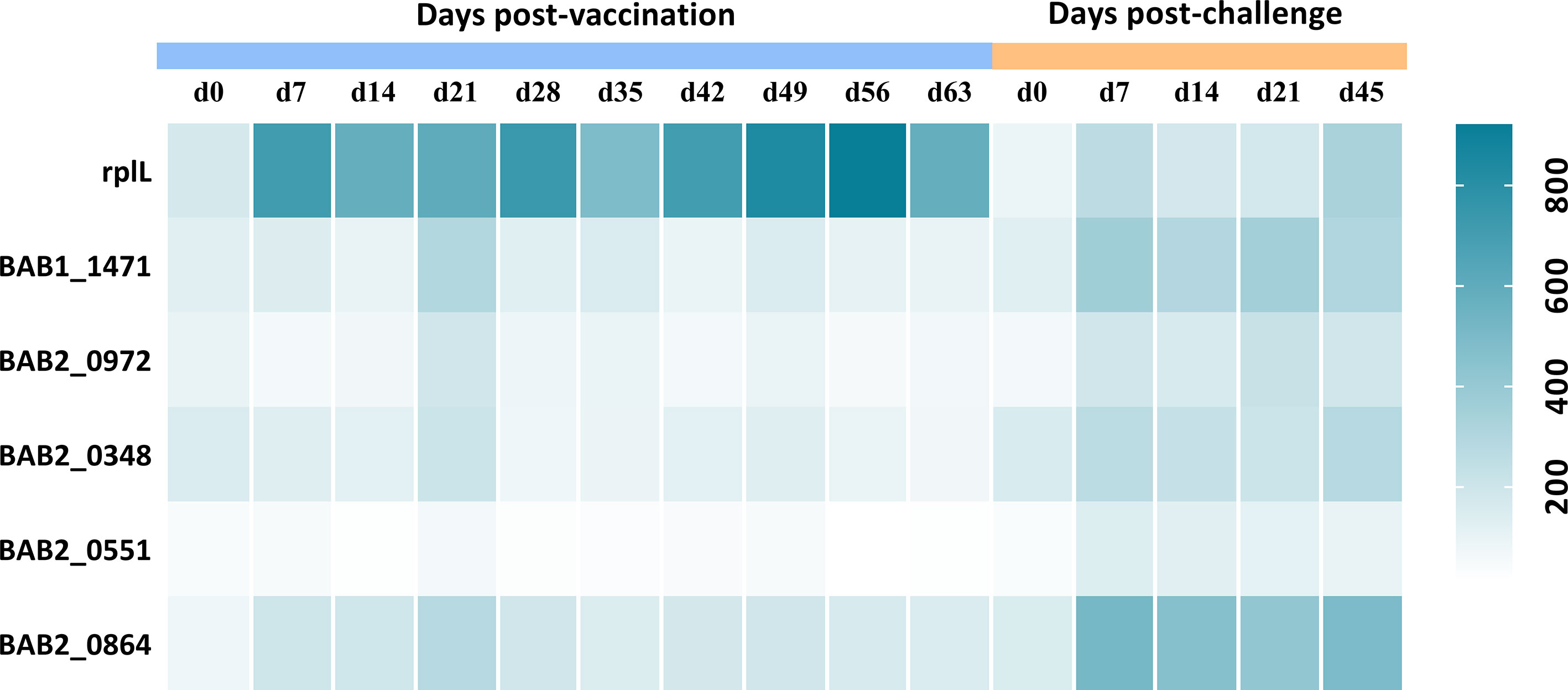
Figure 4 IgG response of 5 proteins obtained from sera of cattle inoculated with A19ΔvirB12 or S2308 at different time points. Values were expressed as average signal intensity of IgG response.
Discussion
B. abortus infection causes rapid activation of innate immunity, including phagocytosis by professional phagocytes, such as macrophages, dendritic cells, and neutrophils. The activation of pattern recognition receptors by pathogen-associated pattern molecules and the secretion of cytokines are key factors in the elimination of Brucella (34, 35). IFN-γ is required for resistance to brucellosis and is closely related to the elimination of Brucella. On the contrary, IL-4 antagonizes IFN-γ mediated antibacterial activity, and promotes chronic infection. This may be due to the interaction between host and Brucella (36). Brucella infection has caused great economic losses, affecting many livestock, wildlife and human. Vaccine immunization is still a necessary strategy for the prevention and control of brucellosis. The licensed Brucella vaccines are live attenuated vaccines, which retain the invasion process similar to that of the wild-type strains, but are easier to be eliminated by the immune system and can induce the production of efficient humoral and cellular immune responses in immunized animals (37). However, these vaccines have obvious limitations in the control and eradication of brucellosis (28). At present, the development of gene-deleted vaccines allows for serological differential diagnosis between vaccination and field infections (38). Here, the virB12 mutant was derived from B. abortus 2308 strain A19. Firstly, the deletion of virB12 gene did not affect the replication ability of A19 within the mice. In addition, vaccination with A19ΔvirB12 could elicit obvious cellular immune response and provide good protection efficacy against a challenge with S2308 in mice. A19ΔvirB12 vaccinated mice induce production of IFN-γ and IL-4. However, low levels of IL-4 were induced in mice vaccinated with another commonly used vaccine, B. abortus RB51, indicating that different vaccine strains cause different protective mechanism. Furthermore, the safety and protection efficiency of A19ΔvirB12 were confirmed in natural host cattle. Brucella infection in cows and ewes is a public health issue and B. melitensis has become the dominant species in China. Although goats and sheep are the preferential host for B. melitensis, this pathogen has become a cause for Brucella outbreaks in cattle (24, 39, 40). Our results showed that vaccination with A19ΔvirB12 can confer effective protection in cattle against the challenge with B. melitensis M28 and is safe for pregnant cattle. What’s more, VirB12 allowed for the serological differentiation between S2308 challenge/natural infection and A19ΔvirB12 vaccination. Recently, A19ΔvirB12 was approved and became the first brucellosis genetically marked vaccine in China (41).
However, the sensitivity and specificity of differential diagnosis based on a single protein need to be improved. We believe that detection of multiple antigens may improve the accuracy of differential diagnosis. Therefore, we attempted to identify novel antigens that could induce differential responses to A19ΔvirB12 vaccination and natural infection. Using a proteomics approach, the protein expression profiles of S2308 and A19ΔvirB12 were compared, and 26 proteins were identified only in S2308. Currently, most proteomics studies focus on differential regulatory proteins. In this study, we focused on the proteins only expressed in the virulent strain, which may have the potential for differential diagnosis. Protein-chip technology was used to further confirm the immune response of these proteins; the IgG response level of challenged sera against five proteins was higher than that of immunized sera in cattle. This suggests that A19ΔvirB12 immunization and field infection may be more accurately distinguished by detecting multiple antigens. Differential vaccines combined with companion diagnostic methods facilitate the control and eradication of epidemic diseases (38). Future research should focus on the development of corresponding diagnostic approaches. In general, we innovatively combined the proteomics approach with the protein array technology in this study, which provides a reference strategy for screening target proteins for the differential diagnosis of other pathogens.
In addition, virulence attenuation mechanisms of vaccine strains are the focus of many studies (42–44). There was no significant difference at the genomic level between the S2308 and A19 vaccine strains (45, 46). Therefore, proteomics studies may contribute to the understanding of the mechanisms causing great differences in virulence between the virulent and attenuated strains. In this study, potential attenuation mechanisms of A19ΔvirB12 were analyzed as follows.
First, some downregulated virulence factors in A19ΔvirB12 were identified (Table 2). VjbR protein (vjbR), a member of the LuxR-type QS regulators, was induced in both the logarithmic and stationary phase of S2308. Previous studies have shown that VjbR is involved in regulating the expression of virulence factors during the early stage of intracellular infection (47, 48). Urease, another factor associated with the survival of Brucella was also affected. In the process of gastrointestinal tract infection, the presence of urease, a nickel-binding protein, enhances the survival capability of pathogenic microorganisms in acidic environments, including Brucella (49–51). Brucella contains two urease operons, ure1 and ure2, and the latter contains the genes of ure ABCEFGDT involved in the transport of urea and nickel (50, 52). In this study, ureC2 and ureG2, contained in the ure2 operon, were more abundant at the protein level in the logarithmic phase of S2308, while ureC2 and ureA2 were found to be induced in the stationary phase of S2308 (52). In addition, several virulence gene products were upregulated in the logarithmic phase of S2308, including cydB, bacA, recA, pgm, and leuA. cydB is responsible for encoding cytochrome bd oxidase. The absence of cydB increases the sensitivity of Brucella to environmental stresses and affects its intracellular survival (53). Similarly, the bacteroid development protein BacA(bacA) is required for Brucella to establish chronic infection in mice (54). Intracellular pathogens inevitably encounter a series of adverse conditions during their life cycle. Timely repair of DNA damage is particularly vital for the intracellular survival of Brucella (55). RecA protein (recA) is an important protein involved in DNA repair pathways. There is no doubt that the lack of RecA reduces the ability of Brucella to deal with DNA damage (56). pgm is responsible for the production of phosphoglucomutase, catalyzing the conversion of glucose-6-phosphate to glucose-1-phosphate. Mutation of pgm that is related to the loss of O-antigen generates a rough phenotype that is usually less virulent (57). Another metabolic enzyme considered to be a virulence factor of Brucella was 2-isopropylmalate synthase (leuA), which is essential for the survival of Brucella within macrophages (29).
The QS pathway was found to be enriched significantly in the logarithmic phase of S2308 compared with that of A19ΔvirB12 (Table 3). QS is a cell-cell communication system involved in monitoring bacterial populations. First, bacteria produce small extracellular signaling molecules that accumulate gradually as the number of bacteria increases. When the concentration of signaling molecules reaches the threshold of detection limit, bacteria respond and synchronously regulate the expression of many genes. QS systems are involved in multiple bacterial processes that are beneficial for survival, such as biofilm formation, virulence factor secretion, and bioluminescence production (58, 59). In view of the role of the QS system in the regulation of virulence factors, it has become a new therapeutic target for some bacterial diseases (60). The significant enrichment of the QS pathway in S2308 may be one of the main reasons for the significant differences in virulence and intracellular survival between the marked vaccine strain and the virulent strain.
In addition, some proteins identified as being downregulated were associated with ABC transporters in the logarithmic phase of A19ΔvirB12 (Table 3). ABC transporters, a large group of membrane protein complexes, are responsible for transmembrane transport of multiple nutrients, accompanied by the hydrolysis of ATP (61). Previous studies have indicated that ABC transporters are related to virulence in some bacteria by transporting various nutrients and metal ions as well as promoting adhesion (62–64). Additionally, a previous study has indicated that the absence of an ABC transporter leads to increased susceptibility of B. melitensis to polymyxin B (65). In this work, the upregulated ABC transporters were mainly involved in the uptake of amino acids, especially BCAA (valine, leucine, and isoleucine). Amino acids not only provide energy for protein synthesis, but are also precursors of many important metabolites, such as ammonia, ketone bodies, and glucose (66). Moreover, BCAA uptake is closely related to the pathogenicity of pathogenic bacteria (67). Some intracellular pathogens rely on BCAA transport proteins to support their growth within host cells (68–70). Thus, decreased BCAA utilization may lead to lower virulence in A19ΔvirB12.
This group of proteins was associated with cell division. Interestingly, some key proteins participating in cell division were observed to be more abundant in A19ΔvirB12 in vitro culture (Figure S2 and Table S4). Cell division is a complex process that requires the participation and coordination of a large number of proteins. The cell division protein ZapA is involved in the formation of a septum called Z-ring in the middle of the cell (71). Min proteins (MinE, MinC, and MinD) play a role in the right placement of Z-ring for equal cell distribution (72). The cytoplasmic ATPase FtsE is involved in promoting septal ring constriction. The cell division coordinator CpoB is required for the coordination of peptidoglycan synthesis and outer membrane constriction (73). Tyrosine recombinase XerD facilitates the disaggregation of chromosome dimers (74). In the logarithmic phase, ZapA, XerD, and CpoB were downregulated in S2308 compared with A19ΔvirB12 at the protein level. In addition, decreased expression levels of MinE and FtsE were observed in the stationary phase of S2308. We assumed that A19ΔvirB12 may have a higher growth rate. Indeed, higher growth rate leads to more material consumption. Especially in the stationary growth period, accompanied by resource depletion and increased levels of toxic metabolites, limiting reproduction level is a wise choice. Notably, in the early stage of the intracellular life cycle, growth arrest was observed in S2308 within the endosomal Brucella-containing vacuole (75). Thus, it may be an important survival strategy for S2308 to limit the level of cell reproduction when necessary.
Based on the COG analysis, 13 and 7 upregulated proteins related to transcription were found in the logarithmic and stationary phase of S2308, respectively. Most of them were bacterial regulatory proteins belonging to different families, such as ArsR, AsnC, and MerR families. Within the group of transcriptional regulators, one protein with σ70 factor activity was induced in S2308 during the logarithmic phase. σ factors are required for initiation of transcription at promoters, and most σ factors belong to the σ70-family. The existence of σ factor is important for bacteria to sense and respond to environmental changes in time (76). Thus, active transcriptional regulation, timely response, and adaptation to environmental changes may be key factors in the pathogenicity of virulent Brucella strains.
Conclusion
In the present study, A19ΔvirB12 was successfully generated. The safety and protective efficacy of A19ΔvirB12 have been confirmed in mice and cattle. VirB12 protein has potential as a diagnostic antigen for the differentiation of A19ΔvirB12-immunization from field infection. We screened for supplementary antigens by combining proteomics and protein chip technology to optimize the accuracy of the differential diagnosis of the VirB12-based method. Among the 26 proteins identified only in S2308, five proteins induced a higher IgG response to challenged sera than immune sera. In addition, we analyzed virulence-related proteins and pathways between S2308 and A19ΔvirB12. The upregulation of virulence factors and QS system, active intake of nutrients, control of reproduction, timely response to environmental change, and induction of virulence factors may mediate the high pathogenicity of S2308. In other words, the difference in virulence is caused by multiple factors. The exploration of Brucella virulence will provide some potential targets for the treatment of brucellosis.
Data Availability Statement
The datasets presented in this study can be found in online repositories. The names of the repository/repositories and accession number(s) can be found below: https://www.ebi.ac.uk/pride/archive/, PXD022031.
Ethics Statement
The animal study was reviewed and approved by Committee on Ethics and Welfare of Experimental Animals of Tecon biological Co., Ltd.
Author Contributions
ZC, SH, and BL conceived and designed the experiments and ML, HZhao, LR, FD and DW performed the experiments. XH, HZhan and CH carried out revision of manuscript. JY analyzed the data and draft the manuscript. All authors contributed to the article and approved the submitted version.
Funding
This work was supported by the State Key Program of National Natural Science of China (U1808202), NSFC International (regional) cooperation and exchange program (31961143024), National Key Research and Development Program Projects (2017YFD0500901, 2017YFD0500305, 2016YFC1200100), the National Key Program for Infectious Disease of China (2018ZX10101002-002), Key Program of Inner Mongolia (2019ZD006).
Conflict of Interest
Authors CH, ML, HZ, LR, DW, and SH were employed by Tecon Biological Co., Ltd.
The remaining authors declare that the research was conducted in the absence of any commercial or financial relationships that could be construed as a potential conflict of interest.
Acknowledgments
We would like to thank the lab members for their efforts, PTM BioLab, Inc for technical assistance with the LC–MS/MS analysis as well as Editage (www.editage.cn) for English language editing.
Supplementary Material
The Supplementary Material for this article can be found online at: https://www.frontiersin.org/articles/10.3389/fimmu.2021.679560/full#supplementary-material
Supplementary Figure 1 | A brief screening process of supplementary differential diagnosis antigens for A19ΔvirB12. First, whole proteins were extracted from A19ΔvirB12 and S2308 in the logarithmic and stationary phases, digested with trypsin, followed by LC–MS/MS analysis. The protein expression profiles of A19ΔvirB12 and S2308 were compared and analyzed. Subsequently, the proteins identified only in S2308 were screened. Finally, protein chip technology was used to find the proteins that have the potential to distinguish immunized and challenged sera of cattle.
Supplementary Figure 2 | COG and GO analysis of DEPs. (A) COG analysis for DEPs in the group of Log w/v. (B) COG analysis for DEPs in the group of Sta w/v. (C) GO analysis for DEPs in the group of Log w/v. (D) GO analysis for DEPs in the group of Sta w/v.
Supplementary Table 1 | Comparison of cytokine level and spleen weight of mice before and after challenge. Differences were determined by the two-tailed Student’s t test.
Supplementary Table 2 | Identified results of mass spectrometry.
Supplementary Table 3 | Differentially expressed proteins in the groups of Log w/v and Sta w/v.
Supplementary Table 4 | COG analysis for DEPs in the groups of Log w/v and Sta w/v.
Supplementary Table 5 | GO analysis for DEPs in the groups of Log w/v and Sta w/v.
Supplementary Table 6 | Specific proteins identified only in S2308.
References
1. Galińska EM, Zagórski J. Brucellosis in Humans–Etiology, Diagnostics, Clinical Forms. Ann Agric Environ Med (2013) 20(2):233–8.
2. Rossetti CA, Arenas-Gamboa AM, Maurizio E. Caprine Brucellosis: A Historically Neglected Disease With Significant Impact on Public Health. PloS Negl Trop Dis (2017) 11(8):e0005692. doi: 10.1371/journal.pntd.0005692
3. Kneipp C, Malik R, Mor SM, Wiethoelter AK. Commentary: Retrospective and Prospective Perspectives on Zoonotic Brucellosis. Front Microbiol (2019) 10:1859. doi: 10.3389/fmicb.2019.01859
4. Zamri-Saad M, Kamarudin MI. Control of Animal Brucellosis: The Malaysian Experience. Asian Pac J Trop Med (2016) 9(12):1136–40. doi: 10.1016/j.apjtm.2016.11.007
5. Xie J, Wang J, Li Z, Wang W, Pang Y, He Y. Ontology-Based Meta-Analysis of Animal and Human Adverse Events Associated With Licensed Brucellosis Vaccines. Front Pharmacol (2018) 9:503. doi: 10.3389/fphar.2018.00503
6. Perkins SD, Smither SJ, Atkins HS. Towards a Brucella Vaccine for Humans. FEMS Microbiol Rev (2010) 34(3):379–94. doi: 10.1111/j.1574-6976.2010.00211.x
7. Weiss M, Brum MC, Anziliero D, Weiblen R, Flores EF. A Glycoprotein E Gene-Deleted Bovine Herpesvirus 1 as a Candidate Vaccine Strain. Braz J Med Biol Res (2015) 48(9):843–51. doi: 10.1590/1414-431x20154243
8. Lin Y, Cao C, Shi W, Huang C, Zeng S, Sun J, et al. Development of a Triplex Real-Time PCR Assay for Detection and Differentiation of Gene-Deleted and Wild-Type African Swine Fever Virus. J Virol Methods (2020) 280:113875. doi: 10.1016/j.jviromet.2020.113875
9. Pomeranz LE, Reynolds AE, Hengartner CJ. Molecular Biology of Pseudorabies Virus: Impact on Neurovirology and Veterinary Medicine. Microbiol Mol Biol Rev (2005) 69(3):462–500. doi: 10.1128/MMBR.69.3.462-500.2005
10. Rolán HG, den Hartigh AB, Kahl-McDonagh M, Ficht T, Adams LG, Tsolis RM. VirB12 Is a Serological Marker of Brucella Infection in Experimental and Natural Hosts. Clin Vaccine Immunol (2008) 15(2):208–14. doi: 10.1128/CVI.00374-07
11. Mirkalantari S, Zarnani AH, Nazari M, Irajian GR, Amirmozafari N. Brucella Melitensis VirB12 Recombinant Protein Is a Potential Marker for Serodiagnosis of Human Brucellosis. Ann Clin Microbiol Antimicrob (2017) 16(1):8. doi: 10.1186/s12941-017-0182-4
12. Du XJ, Han R, Li P, Wang S. Comparative Proteomic Analysis of Cronobacter Sakazakii Isolates With Different Virulences. J Proteomics (2015) 128:344–51. doi: 10.1016/j.jprot.2015.08.013
13. Li Y, Zou Y, Xia Y, Bai J, Wang X, Jiang P. Proteomic and Transcriptomic Analyses of Swine Pathogen Erysipelothrix Rhusiopathiae Reveal Virulence Repertoire. PloS One (2016) 11(8):e0159462. doi: 10.1371/journal.pone.0159462
14. Li S, Fang L, Liu W, Song T, Zhao F, Zhang R, et al. Quantitative Proteomic Analyses of a Pathogenic Strain and Its Highly Passaged Attenuated Strain of Mycoplasma Hyopneumoniae. BioMed Res Int (2019) 2019:4165735. doi: 10.1155/2019/4165735
15. Li Z, Zhang J, Zhang KE, Fu Q, Wang Z, Li T, et al. Brucella Melitensis 16mΔTcfSR as a Potential Live Vaccine Allows for the Differentiation Between Natural and Vaccinated Infection. Exp Ther Med (2015) 10(3):1182–8. doi: 10.3892/etm.2015.2619
16. Bricker BJ. PCR as a Diagnostic Tool for Brucellosis. Vet Microbiol (2002) 90(1-4):435–46. doi: 10.1016/s0378-1135(02)00228-6
17. Bricker BJ, Halling SM. Differentiation of Brucella Abortus Bv. 1, 2, and 4, Brucella Melitensis, Brucella Ovis, and Brucella Suis Bv. 1 by PCR. J Clin Microbiol (1994) 32(11):2660–6. doi: 10.1128/JCM.32.11.2660-2666.1994
18. Ge J, Cui X, Shi Y, Zhao L, Wei C, Wen S, et al. Development and Application of an Indirect Enzyme-Linked Immunosorbent Assay Based on Recombinant Capsid Protein for the Detection of Mink Circovirus Infection. BMC Vet Res (2018) 14(1):29. doi: 10.1186/s12917-018-1337-z
19. Zai X, Yang Q, Liu K, Li R, Qian M, Zhao T, et al. A Comprehensive Proteogenomic Study of the Human Brucella Vaccine Strain 104 M. BMC Genomics (2017) 18(1):402. doi: 10.1186/s12864-017-3800-9
20. Kanehisa M, Furumichi M, Tanabe M, Sato Y, Morishima K. KEGG: New Perspectives on Genomes, Pathways, Diseases and Drugs. Nucleic Acids Res (2017) 45(D1):D353–61. doi: 10.1093/nar/gkw1092
21. Galperin MY, Makarova KS, Wolf YI, Koonin EV. Expanded Microbial Genome Coverage and Improved Protein Family Annotation in the COG Database. Nucleic Acids Res (2015) 43(Database issue):D261–9. doi: 10.1093/nar/gku1223
22. Roset MS, Ibañez AE, de Souza Filho JA, Spera JM, Minatel L, Oliveira SC, et al. Brucella Cyclic β-1,2-glucan Plays a Critical Role in the Induction of Splenomegaly in Mice. PloS One (2014) 9(7):e101279. doi: 10.1371/journal.pone.0101279
23. Tian GZ, Cui BY, Piao DR, Zhao HY, Li LY, Liu X, et al. Multi-Locus Variable-Number Tandem Repeat Analysis of Chinese Brucella Strains Isolated From 1953 to 2013. Infect Dis Poverty (2017) 6(1):89. doi: 10.1186/s40249-017-0296-0
24. Zhang H, Deng X, Cui B, Shao Z, Zhao X, Yang Q, et al. Abortion and Various Associated Risk Factors in Dairy Cow and Sheep in Ili, China. PloS One (2020) 15(10):e0232568. doi: 10.1371/journal.pone.0232568
25. Deresa B, Tulu D, Deressa FB. Epidemiological Investigation of Cattle Abortion and Its Association With Brucellosis in Jimma Zone, Ethiopia. Vet Med (Auckl) (2020) 11:87–98. doi: 10.2147/VMRR.S266350
26. Carpenter TE, Chrièl M, Andersen MM, Wulfson L, Jensen AM, Houe H, et al. An Epidemiologic Study of Late-Term Abortions in Dairy Cattle in Denmark, July 2000-August 2003. Prev Vet Med (2006) 77(3-4):215–29. doi: 10.1016/j.prevetmed.2006.07.005
27. Norman HD, Miller RH, Wright JR, Hutchison JL, Olson KM. Factors Associated With Frequency of Abortions Recorded Through Dairy Herd Improvement Test Plans. J Dairy Sci (2012) 95(7):4074–84. doi: 10.3168/jds.2011-4998
28. Schurig GG, Sriranganathan N, Corbel MJ. Brucellosis Vaccines: Past, Present and Future. Vet Microbiol (2002) 90(1-4):479–96. doi: 10.1016/S0378-1135(02)00255-9
29. Sayers S, Li L, Ong E, Deng S, Fu G, Lin Y, et al. Victors: A Web-Based Knowledge Base of Virulence Factors in Human and Animal Pathogens. Nucleic Acids Res (2019) 47(D1):D693–700. doi: 10.1093/nar/gky999
30. Lewis VG, Ween MP, McDevitt CA. The Role of ATP-binding Cassette Transporters in Bacterial Pathogenicity. Protoplasma (2012) 249(4):919–42. doi: 10.1007/s00709-011-0360-8
31. Castillo-Juárez I, Maeda T, Mandujano-Tinoco EA, Tomás M, Pérez-Eretza B, García-Contreras SJ, et al. Role of Quorum Sensing in Bacterial Infections. World J Clin cases (2015) 3(7):575–98. doi: 10.12998/wjcc.v3.i7.575
32. Barbier T, Nicolas C, Letesson JJ. Brucella Adaptation and Survival At the Crossroad of Metabolism and Virulence. FEBS Lett (2011) 585(19):2929–34. doi: 10.1016/j.febslet.2011.08.011
33. Senevirathne A, Hewawaduge C, Lee JH. Live Vaccine Consisting of Attenuated Salmonella Secreting and Delivering Brucella Ribosomal Protein L7/L12 Induces Humoral and Cellular Immune Responses and Protects Mice Against Virulent Brucella Abortus 544 Challenge. Vet Res (2020) 51(1):6. doi: 10.1186/s13567-020-0735-y
34. Gomes MT, Campos PC, de Almeida LA, Oliveira FS, Costa MM, Marim FM, et al. The Role of Innate Immune Signals in Immunity to Brucella Abortus. Front Cell Infect Microbiol (2012) 2:130. doi: 10.3389/fcimb.2012.00130
35. Copin R, De Baetselier P, Carlier Y, Letesson JJ, Muraille E. MyD88-Dependent Activation of B220-CD11b+LY-6C+ Dendritic Cells During Brucella Melitensis Infection. J Immunol (2007) 178(8):5182–91. doi: 10.4049/jimmunol.178.8.5182
36. Dorneles EM, Teixeira-Carvalho A, Araújo MS, Sriranganathan N, Lage AP. Immune Response Triggered by Brucella Abortus Following Infection or Vaccination. Vaccine (2015) 33(31):3659–66. doi: 10.1016/j.vaccine.2015.05.057
37. Olsen SC. Recent Developments in Livestock and Wildlife Brucellosis Vaccination. Rev Sci Tech (2013) 32(1):207–17. doi: 10.20506/rst.32.1.2201
38. Henderson LM. Overview of Marker Vaccine and Differential Diagnostic Test Technology. Biologicals (2005) 33(4):203–9. doi: 10.1016/j.biologicals.2005.08.006
39. Zhu X, Zhao Z, Ma S, Guo Z, Wang M, Li Z, et al. Brucella Melitensis, a Latent “Travel Bacterium,” Continual Spread and Expansion From Northern to Southern China and Its Relationship to Worldwide Lineages. Emerg Microbes Infect (2020) 9(1):1618–27. doi: 10.1080/22221751.2020.1788995
40. Alvarez J, Sáez JL, García N, Serrat C, Pérez-Sancho M, González S, et al. Management of an Outbreak of Brucellosis Due to B. Melitensis in Dairy Cattle in Spain. Res Vet Sci (2011) 90(2):208–11. doi: 10.1016/j.rvsc.2010.05.028
41. Ministry of Agriculture and Rural Affairs of the People's Republic of China. Announcement of the Ministry of Agriculture and Rural Affairs of the People’s Republic of China. Available at: http://www.moa.gov.cn/govpublic/xmsyj/202007/t20200721_6348993.htm [Accessed July 21, 2020].
42. Hsu T, Hingley-Wilson SM, Chen B, Chen M, Dai AZ, Morin PM, et al. The Primary Mechanism of Attenuation of Bacillus Calmette-Guerin Is a Loss of Secreted Lytic Function Required for Invasion of Lung Interstitial Tissue. Proc Natl Acad Sci USA (2003) 100(21):12420–5. doi: 10.1073/pnas.1635213100
43. Bull TJ, Schock A, Sharp JM, Greene M, McKendrick IJ, Sales J, et al. Genomic Variations Associated With Attenuation in Mycobacterium Avium Subsp. Paratuberculosis Vaccine Strains. BMC Microbiol (2013) 13:11. doi: 10.1186/1471-2180-13-11
44. Yu D, Hui Y, Zai X, Xu J, Liang L, Wang B, et al. Comparative Genomic Analysis of Brucella Abortus Vaccine Strain 104M Reveals a Set of Candidate Genes Associated With Its Virulence Attenuation. Virulence (2015) 6(8):745–54. doi: 10.1080/21505594.2015.1038015
45. Crasta OR, Folkerts O, Fei Z, Mane SP, Evans C, Martino-Catt S, et al. Genome Sequence of Brucella Abortus Vaccine Strain S19 Compared to Virulent Strains Yields Candidate Virulence Genes. PloS One (2008) 3(5):e2193. doi: 10.1371/journal.pone.0002193
46. Wang S, Wang W, Sun K, Bateer H, Zhao X. Comparative Genomic Analysis Between Newly Sequenced Brucella Abortus Vaccine Strain A19 and Another Brucella Abortus Vaccine S19. Genomics (2019) 112(2):1444–53. doi: 10.1016/j.ygeno.2019.08.015
47. Uzureau S, Lemaire J, Delaive E, Dieu M, Gaigneaux A, Raes M, et al. Global Analysis of Quorum Sensing Targets in the Intracellular Pathogen Brucella Melitensis 16 M. J Proteome Res (2010) 9(6):3200–17. doi: 10.1021/pr100068p
48. Kleinman CL, Sycz G, Bonomi HR, Rodríguez RM, Zorreguieta A, Sieira R. ChIP-seq Analysis of the LuxR-type Regulator VjbR Reveals Novel Insights Into the Brucella Virulence Gene Expression Network. Nucleic Acids Res (2017) 45(10):5757–69. doi: 10.1093/nar/gkx165
49. Clyne M, Dolan B, Reeves EP. Bacterial Factors That Mediate Colonization of the Stomach and Virulence of Helicobacter Pylori. FEMS Microbiol Lett (2007) 268(2):135–43. doi: 10.1111/j.1574-6968.2007.00648.x
50. Sangari FJ, Seoane A, Rodríguez MC, Agüero J, García Lobo JM. Characterization of the Urease Operon of Brucella Abortus and Assessment of Its Role in Virulence of the Bacterium. Infect Immun (2007) 75(2):774–80. doi: 10.1128/IAI.01244-06
51. Zhou C, Bhinderwala F, Lehman MK, Thomas VC, Chaudhari SS, Yamada KJ, et al. Urease Is an Essential Component of the Acid Response Network of Staphylococcus Aureus and Is Required for a Persistent Murine Kidney Infection. PloS Pathog (2019) 15(1):e1007538. doi: 10.1371/journal.ppat.1007538
52. Bandara AB, Contreras A, Contreras-Rodriguez A, Martins AM, Dobrean V, Poff-Reichow S, et al. Brucella Suis Urease Encoded by Ure1 But Not Ure2 Is Necessary for Intestinal Infection of BALB/c Mice. BMC Microbiol (2007) 7:57. doi: 10.1186/1471-2180-7-57
53. Endley S, McMurray D, Ficht TA. Interruption of the CydB Locus in Brucella Abortus Attenuates Intracellular Survival and Virulence in the Mouse Model of Infection. J Bacteriol (2001) 183(8):2454–62. doi: 10.1128/JB.183.8.2454-2462.2001
54. LeVier K, Phillips RW, Grippe VK, Roop RM 2nd, Walker GC. Similar Requirements of a Plant Symbiont and a Mammalian Pathogen for Prolonged Intracellular Survival. Science (2000) 287(5462):2492–3. doi: 10.1126/science.287.5462.2492
55. Roop RM 2nd, Gaines JM, Anderson ES, Caswell CC, Martin DW. Survival of the Fittest: How Brucella Strains Adapt to Their Intracellular Niche in the Host. Med Microbiol Immunol (2009) 198(4):221–38. doi: 10.1007/s00430-009-0123-8
56. Tatum FM, Morfitt DC, Halling SM. Construction of a Brucella Abortus RecA Mutant and Its Survival in Mice. Microb Pathog (1993) 14(3):177–85. doi: 10.1006/mpat.1993.1018
57. Ugalde JE, Czibener C, Feldman MF, Ugalde RA. Identification and Characterization of the Brucella Abortus Phosphoglucomutase Gene: Role of Lipopolysaccharide in Virulence and Intracellular Multiplication. Infect Immun (2000) 68(10):5716–23. doi: 10.1128/IAI.68.10.5716-5723.2000
58. Ng WL, Bassler BL. Bacterial Quorum-Sensing Network Architectures. Annu Rev Genet (2009) 43:197–222. doi: 10.1146/annurev-genet-102108-134304
59. Papenfort K, Bassler BL. Quorum Sensing Signal-Response Systems in Gram-negative Bacteria. Nat Rev Microbiol (2016) 14(9):576–88. doi: 10.1038/nrmicro.2016.89
60. Jiang Q, Chen J, Yang C, Yin Y, Yao K. Quorum Sensing: A Prospective Therapeutic Target for Bacterial Diseases. BioMed Res Int (2019) 2019:2015978. doi: 10.1155/2019/2015978
61. Jones PM, George AM. The ABC Transporter Structure and Mechanism: Perspectives on Recent Research. Cell Mol Life Sci (2004) 61(6):682–99. doi: 10.1007/s00018-003-3336-9
62. Dassa E, Bouige P. The ABC of ABCS: A Phylogenetic and Functional Classification of ABC Systems in Living Organisms. Res Microbiol (2001) 152(3-4):211–29. doi: 10.1016/S0923-2508(01)01194-9
63. Tamura GS, Nittayajarn A, Schoentag DL. A Glutamine Transport Gene, glnQ, Is Required for Fibronectin Adherence and Virulence of Group B Streptococci. Infect Immun (2002) 70(6):2877–85. doi: 10.1128/IAI.70.6.2877-2885.2002
64. Tanaka KJ, Song S, Mason K, Pinkett HW. Selective Substrate Uptake: The Role of ATP-binding Cassette (ABC) Importers in Pathogenesis. Biochim Biophys Acta Biomembr (2018) 1860(4):868–77. doi: 10.1016/j.bbamem.2017.08.011
65. Wang Z, Bie P, Cheng J, Lu L, Cui B, Wu Q. The ABC Transporter YejABEF Is Required for Resistance to Antimicrobial Peptides and the Virulence of Brucella Melitensis. Sci Rep (2016) 6:31876. doi: 10.1038/srep31876
66. Wu G. Amino Acids: Metabolism, Functions, and Nutrition. Amino Acids (2009) 37(1):1–17. doi: 10.1007/s00726-009-0269-0
67. Kaiser JC, Heinrichs DE. Branching Out: Alterations in Bacterial Physiology and Virulence Due to Branched-Chain Amino Acid Deprivation. mBio (2018) 9(5):e01188–18. doi: 10.1128/mBio.01188-18
68. Bange FC, Brown AM, Jacobs WR Jr. Leucine Auxotrophy Restricts Growth of Mycobacterium Bovis BCG in Macrophages. Infect Immun (1996) 64(5):1794–9. doi: 10.1128/IAI.64.5.1794-1799.1996
69. Atkins T, Prior RG, Mack K, Russell P, Nelson M, Oyston PC, et al. A Mutant of Burkholderia Pseudomallei, Auxotrophic in the Branched Chain Amino Acid Biosynthetic Pathway, Is Attenuated and Protective in a Murine Model of Melioidosis. Infect Immun (2002) 70(9):5290–4. doi: 10.1128/IAI.70.9.5290-5294.2002
70. Brenner M, Lobel L, Borovok I, Sigal N, Herskovits AA. Controlled Branched-Chain Amino Acids Auxotrophy in Listeria Monocytogenes Allows Isoleucine to Serve as a Host Signal and Virulence Effector. PloS Genet (2018) 14(3):e1007283. doi: 10.1371/journal.pgen.1007283
71. Galli E, Gerdes K. Spatial Resolution of Two Bacterial Cell Division Proteins: ZapA Recruits ZapB to the Inner Face of the Z-Ring. Mol Microbiol (2010) 76(6):1514–26. doi: 10.1111/j.1365-2958.2010.07183.x
72. Weiss DS. Bacterial Cell Division and the Septal Ring. Mol Microbiol (2004) 54(3):588–97. doi: 10.1111/j.1365-2958.2004.04283.x
73. Gray AN, Egan AJ, Van’t Veer IL, Verheul J, Colavin A, Koumoutsi A, et al. Coordination of Peptidoglycan Synthesis and Outer Membrane Constriction During Escherichia Coli Cell Division. Elife (2015) 4:e07118. doi: 10.7554/eLife.07118
74. Midonet C, Das B, Paly E, Barre FX. XerD-Mediated FtsK-Independent Integration of TLCϕ Into the Vibrio Cholerae Genome. Proc Natl Acad Sci USA (2014) 111(47):16848–53. doi: 10.1073/pnas.1404047111
75. Deghelt M, Mullier C, Sternon JF, Francis N, Laloux G, Dotreppe D, et al. G1-Arrested Newborn Cells Are the Predominant Infectious Form of the Pathogen Brucella Abortus. Nat Commun (2014) 5:4366. doi: 10.1038/ncomms5366
Keywords: protein chip technology, B. abortus, A19ΔvirB12, proteomics, differential diagnosis, attenuation mechanism
Citation: Yang J, He C, Zhang H, Liu M, Zhao H, Ren L, Wu D, Du F, Liu B, Han X, He S and Chen Z (2021) Evaluation and Differential Diagnosis of a Genetic Marked Brucella Vaccine A19ΔvirB12 for Cattle. Front. Immunol. 12:679560. doi: 10.3389/fimmu.2021.679560
Received: 12 March 2021; Accepted: 10 May 2021;
Published: 07 June 2021.
Edited by:
Pedro A. Reche, Complutense University of Madrid, SpainReviewed by:
Paulo Bettencourt, Catholic University of Portugal, PortugalHan Sang Yoo, Seoul National University, South Korea
Copyright © 2021 Yang, He, Zhang, Liu, Zhao, Ren, Wu, Du, Liu, Han, He and Chen. This is an open-access article distributed under the terms of the Creative Commons Attribution License (CC BY). The use, distribution or reproduction in other forums is permitted, provided the original author(s) and the copyright owner(s) are credited and that the original publication in this journal is cited, in accordance with accepted academic practice. No use, distribution or reproduction is permitted which does not comply with these terms.
*Correspondence: Zeliang Chen, zeliangchen@yahoo.com; Xiaohu Han, hxh8849@163.com; Sun He, hesun@tecon-bio.com; Baoshan Liu, lbslgy@syau.edu.cn
†These authors have contributed equally to this work