Abstract
Chitosan is a polysaccharide that has been extensively studied in the field of biomedicine, especially its water-soluble degraded products called chitooligosaccharides (COS). In this study, COS were produced by the degradation of chitosan hydrogel dispersed in a dilute solution (i.e., 1.55 mM) of various kinds of carboxylic acids using a non-thermal plasma technology called solution plasma (SP). The degradation rates of chitosan were influenced by the type of carboxylic acids, depending on the interaction between chitosan and each carboxylic acid. After SP treatment, the water-soluble degraded products containing COS could be easily separated from the water-insoluble residue of chitosan hydrogel by centrifugation. The production yields of the COS were mostly higher than 55%. Furthermore, the obtained COS products were evaluated for their inhibitory effect as well as their selectivity against human lung cancer cells (H460) and human lung normal cells (MRC-5).
Export citation and abstract BibTeX RIS
1. Introduction
Biopolymers are polymers produced by living organisms such as animals, plants, fungi, and bacteria.1,2) Biopolymers contain monomeric units that are covalently bonded together to form polymeric chain structures. For example, polysaccharides have monosaccharides as repeating units linked together through glycosidic bonds.3,4) Owning to their biocompatibility and biodegradability, their uses for eco-commodities, food supplements, cosmetics, and especially biomedical applications have been growing during the past decade.5)
Chitosan is one of the polysaccharides that has attracted much interest from both academic and industrial viewpoints. Chitosan is a derivative of chitin, which can be found as a structural component in shells of crustaceans, the cuticle of insects, and cell walls of fungi. Chitosan contains d-glucosamine as a major monomeric unit randomly connected with N-acetyl-d-glucosamine units by β-(1 → 4) glycosidic linkages.6) Their chemical structures are shown in Fig. 1. Chitosan consists of reactive functional groups including hydroxyl groups at the C3 and C6 positions and an amino or acetamido group at the C2 position.7) The presence of amino groups that cause positively charged character of chitosan is the main reason that chitosan differs from other polysaccharides, which have a neutral or negatively charged character. The positively charged nature of chitosan correlates to its metal chelation, flocculation, as well as biological properties.7) Therefore, chitosan has been intensively investigated in several applications, especially in biomedical fields such as drug and gene delivery,8,9) tissue engineering,10) wound dressings,11,12) and biosensors.13)
Fig. 1. Chemical structures of chitin and chitosan.
Download figure:
Standard image High-resolution imageUnfortunately, native chitosan generally has a high molecular weight together with intra- and intermolecular hydrogen bond networks due to the abundance of hydroxyl groups, resulting in its insolubility in water and poor solubility in most organic solvents.14) Therefore, many studies relating to chitosan have focused on a water-soluble form of chitosan, such as chitooligosaccharides (COS), which, moreover, broadens the biological applications of chitosan. The biological properties of both chitosan and COS according to previous studies are summarized in supplementary data (Table I).
Table I. Mw (Da) and PDI of chitosan solution at a concentration of 0.2% dissolved in 1 and 2 M acetic acid solutions and chitosan hydrogel at a solid content of 0.2% (dry weight) dispersed in 1.55 mM acetic acid solution after the degradation via conventional heat treatment and SP treatment for different reaction times in this study in comparison with the previous works.
SP treatment time (min) | Mw (PDI) | ||||
---|---|---|---|---|---|
Heat at 75 °C | SP | ||||
Chitosan hydrogel | Chitosan hydrogel | Chitosan solution | Chitosan solution29) | Chitosan solution40) | |
0 | 4.8 × 105 (2.35) | 4.8 × 105 (2.35) | 4.8 × 105 (2.35) | 1.1 × 106 (4.10) | 2.1 × 105 (4.10) |
15 | — | 7.3 × 104 (4.20) | — | 4.3 × 105 (5.40) | — |
30 | 4.2 × 105 (4.35) | 2.2 × 104 (3.35) | 1.1 × 105 (5.21) | 2.0 × 105 (5.30) | 6.2 × 104 (2.70) |
45 | — | 1.1 × 104 (2.74) | — | — | — |
60 | 4.0 × 105 (4.24) | 6.6 × 103 (2.35) | 2.1 × 104 (5.42) | 1.0 × 105 (2.35) | 5.6 × 104 (2.70) |
Cancer is one of the major causes of death worldwide.15) The current treatments for cancer, such as radiation therapy and chemotherapy, still cause high risks of harming normal cells and lead to long-term and short-term side effects in patients.16,17) Therefore, many researchers have attempted to find alternative ways of treating cancer minimizing negative effects on healthy cells, such as by using natural products. COS are natural products that have been widely studied and show potential as an alternative choice for the treatment of cancer.18–20) Some examples relating to the anticancer activity of COS are presented in supplementary data (Table II).
Table II. Chemical structures, acid dissociation constants, number of carboxylic groups, and number of methylene groups on the backbone of the carboxylic acids used in this study.
Acid | Chemical structure | pKa54) | Number of carboxylic groups | Number of –CH2– groups on backbone |
---|---|---|---|---|
Acetic acid | ![]() |
4.76 | 1 | — |
Oxalic acid | ![]() |
1.23, 4.19 | 2 | 0 |
Malonic acid | ![]() |
2.83, 5.76 | 2 | 1 |
Succinic acid | ![]() |
4.19, 5.48 | 2 | 2 |
Glutaric acid | ![]() |
4.34, 5.42 | 2 | 3 |
Adipic acid | ![]() |
4.42, 5.41 | 2 | 4 |
Pimelic acid | ![]() |
4.48, 5.42 | 2 | 5 |
Azelaic acid | ![]() |
4.55, 5.41 | 2 | 7 |
Citric acid | ![]() |
3.09, 4.75, 5.41 | 3 | — |
COS can be prepared by the degradation of chitosan. This degradation has been used to produce low-molecular-weight products of not only chitosan but also other polysaccharides, leading to the improvement of their water solubility and absorbability in order to exert their biological effects. In general, there are three main methods applied for the degradation of polysaccharides: chemical degradation,21–23) enzymatic degradation,24–26) and physical degradation.27,28) Degradation by using strong chemicals has been widely used on an industrial scale, but the use of a large amount of hazardous chemicals leads to difficulty in handling the generated waste and may cause environmental pollution.29) Using enzymes to degrade chitosan has drawn much attention because they were found to be able to region-selectively degrade chitosan under mild conditions.30) However, high-quality enzymes and the operating setup are expensive, while the production yield has been reported to be relatively low.6) Recently, physical methods, applying an energy, such as microwaves,31–34) ultraviolet,35) sonication,36,37) gamma rays,27) and plasma,38) have been extensively studied because they can enhance the degradation of chitosan with low chemical requirements.
Non-thermal plasma discharge under a liquid phase, called solution plasma (SP), is one of the energy-impact methods that has been recently utilized as a tool for the degradation of polysaccharides such as chitosan29,39,40) and alginate.41) The term SP was proposed by Takai.42) In early research, SP was used for the synthesis of noble-metal nanoparticles43,44) and the decomposition of organic compounds in water treatment.45) SP has the ability to produce highly active species such as hydroxyl radicals (OH), hydroperoxyl radicals (
HO2), free electrons (e−), superoxide anions (O2−), and atomic oxygen anions (O−) under atmospheric pressure and room temperature.29,46) These radicals play a part in the enhancement of the degradation of polymers. For instance, in the degradation of chitosan to produce COS by SP treatment, it has been reported that the plasma-induced
OH attacked the chitosan chain and caused scission at the glycosidic bonds.29)
Since COS are high-valued natural substances possessing potential anticancer activity, their production processes, including not only degradation but also separation and purification, are very important. Currently, the production of COS still uses a lot of chemicals and needs some complicated separation and purification systems. For example, it has been reported that the degradation of chitosan by 35% HCl was effectively conducted within 30 min to produce COS with a degree of polymerization (DP) of 8, but owing to the use of strong acid, further neutralization by NaOH as well as separation and purification to remove contaminants were required.25) Even though, methods such as microwave and SP treatment have been claimed to realize the green degradation of chitosan, chitosan still has to be dissolved in an acetic acid solution with a concentration of approximately 1–2 M in order to obtain chitosan solution prior to the degradation.19,29,32,39,40) The degradation of chitosan solution usually leads to some difficulty in separating COS from the high-molecular-weight chitosan. This is because they are dissolved together in the solution. Therefore, the heterogeneous degradation of chitosan in an undissolved form, such as chitosan hydrogel, will probably ease the separation of the COS products from the high-molecular-weight chitosan. A hydrogel is generally defined as a hydrophilic polymer capable of holding a large amount of water in its three-dimensional networks.47) In this study, COS were produced by applying plasma technology in order to reduce the use of chemicals in the degradation reaction. Furthermore, simple separation and purification processes to attain COS should be accomplished by conducting the heterogeneous degradation of chitosan hydrogel dispersed in dilute acid solution. Chitosan hydrogel was prepared by the reprecipitation method, which resulted in the reduction of the crystallinity of chitosan.48) Various carboxylic acids, such as di- and tricarboxylic acids, were added to the suspension of chitosan hydrogel in order to examine the influences of distinct carboxylic acids, other than acetic acid, which is a monocarboxylic acid, in relation to the degradation of chitosan. Finally, the obtained COS products were evaluated for their anticancer activity as well as their selectivity to cancer cells in comparison with normal cells.
2. Experimental methods
2.1. Materials
Chitosan was obtained from the deacetylation of chitin, which was prepared from the shells of Metapenaeus dobsoni shrimp, provided by Surapon Foods Public. Glacial acetic acid (CH3COOH, 99.9%), hydrochloric acid (HCl, 37.0%), sodium chloride (NaCl, 99.0%), and sodium hydroxide (NaOH, 97.0%) pellets were purchased from RCI Labscan. A 50% (w/v) NaOH solution was supplied by the Chemical Enterprise. Oxalic acid dihydrate [(COOH)2 2H2O, 98.0%] and succinic acid [C2H4(COOH)2, 99.5%] were purchased from Ajax Finechem. Malonic acid [CH2(COOH)2, 99.0%], glutaric acid [C3H6(COOH)2, 99.0%], adipic acid [C4H8(COOH)2, 99.0%], pimelic acid [C5H10(COOH)2, 98.0%], and azelaic acid [C7H14(COOH)2, 98.0%] were supplied by Sigma-Aldrich. Citric acid [C3H5O(COOH)3, 99.5–102.0%] was purchased from Loba Chemie. Sodium borohydride (NaBH4) and potassium bromide (KBr) were obtained from Carlo Erba Reagents and Wako, respectively.
2.2. Preparation of chitosan hydrogel from the shrimp shell
M. dobsoni shrimp shells were washed and dried under sunlight before grinding into small flakes. The flakes of dried shrimp shell were demineralized by soaking in a 1 M HCl solution for 24 h. The demineralization step to remove calcium carbonate in shrimp shells was repeated by adding a fresh 1 M HCl solution and soaking for another 24 h before the flakes were removed and washed with distilled water until neutral. Subsequently, the demineralized shrimp shell flakes were deproteinized by immersion in a 4% (w/v) NaOH solution at 80 °C with vigorous stirring for 4 h. The flakes were filtered and washed with distilled water until the eluent reached a pH of 7.0. Then, the deproteinized product, known as chitin, was obtained. A 50% (w/v) NaOH solution containing 0.5% (w/v) NaBH4 was added to the chitin flakes before the suspension was heated in an autoclave at 110 °C for 75 min. After filtration, the solid fraction from the deacetylation process was washed with distilled water until the pH became neutral. The deacetylation process was repeated twice to achieve the required degree of deacetylation (%DD) of chitosan (i.e., 90%), calculated by following an equation of Sabnis and Block.49) The solid-to-liquid ratio used in all processes was . After each process, water in the product was removed by drying in an oven at 60 °C before continuing further steps or use.
Chitosan solution was prepared by dissolving chitosan flakes (2 g) into 200 ml of 2% (v/v) acetic acid solution. The obtained chitosan solution was then poured dropwise into 400 ml of 5% (w/v) NaOH solution with vigorous stirring.48) The gel-like precipitate was dialyzed against distilled water in a dialysis tube with a molecular weight cutoff of 12,132 Da (Sigma-Aldrich) until neutral to attain chitosan hydrogel.
2.3. Degradation of chitosan hydrogel
A suspension of chitosan hydrogel for the degradation by the SP treatment was prepared by adding 6 g of chitosan hydrogel (having dry weight ∼0.1 g) to the SP reactor and then 50 ml of dilute acid solution was added to the suspension with an acid-to-chitosan mole ratio of or equivalent to 1.55 mM. The suspension was stirred at room temperature (30 °C) for 5 min. The SP reactor was made of a glass beaker having two tungsten electrodes (purity 99.9%, Nilaco) with a diameter of 1 mm inserted in it. The SP system used in this study has been described in previous works40,42,46) and is schematically shown in Fig. 2. The operation parameters of the typical voltage, pulse frequency, pulse width, and electrode distance were 1.6 kV, 23 kHz, 2 µs, and 0.75 mm, respectively. The reaction temperature during the plasma operation was approximately 60–70 °C.
Fig. 2. Schematic diagram of SP setup.
Download figure:
Standard image High-resolution image2.4. Separation of degraded chitosan product
After the degradation of chitosan hydrogel by the SP treatment, the suspension was centrifuged to collect the remaining solid residue assigned as the solid fraction, which was water-insoluble chitosan that was then dried in an oven at 60 °C. The supernatant was further freeze-dried and assigned as the solution fraction, which should contain water-soluble COS. All fractions were weighed and the percentage yield of each fraction was calculated using the following equation:

where W0 is the initial dry weight of chitosan in the system and Wi is the dry weight of the degraded chitosan fraction, while i refers to either the solid fraction or solution fraction.
2.5. Characterization
The crystallinity of native chitosan and chitosan hydrogel before and after the SP treatment was characterized by using a wide-angle X-ray diffraction (WAXD) analyzer (Rigaku SmartLab) operated in a continuous mode with a scan speed of 5° min−1 and a scattering angle (2θ) from 5 to 60° using Cu Kα as an X-ray source.
The morphology of the chitosan flakes and dried chitosan hydrogel was examined by using field-emission scanning electron microscopy (FE-SEM; JEOL JSM-7610F).
The changes in the average molecular weight and the molecular weight distribution of chitosan during the degradation via the SP treatment were determined using gel permeation chromatography (GPC; Shimadzu CTO-10A) apparatus equipped with a refractive index (RI) detector. An ultrahydrogel linear column (Waters 600E) for separation of the molecular weight in the range of 1.0 × 103 to 2.0 × 107 Da was connected in series with a guard column equipped in an oven at the operating temperature of 40 °C. The mobile phase was a 0.5 M acetate buffer solution at pH 4.0 and the flow rate was set at 0.6 ml·min−1. The injection volume of a sample was 40 µl, comprising 3 mg·ml−1 chitosan in the acetate buffer solution. Pullulans with molecular weight in the range of 2.17 × 103 to 8.05 × 105 Da were used as standard samples.
The investigation of any changes in the chemical structures of chitosan after the degradation via the SP treatment was carried out by using Fourier transform infrared (FT-IR) spectrometry (Thermo Fisher Scientific Nicolet iS5). FT-IR spectra with wavenumbers from 4000 to 400 cm−1 were taken on KBr pellets at a resolution of 4 cm−1 using 64 scans with correction for atmospheric carbon dioxide (CO2).
2.6. Biological assay
2.6.1. Cell culture.
H460 cells (human lung cancer cell line) and MRC-5 cells (human lung normal cell line) were cultured by using Roswell Park Memorial Institute (RPMI) 1640 Medium with l-glutamine (Biowest), and Eagle's Minimum Essential Medium (EMEM) with sodium bicarbonate, non-essential amino acids, l-glutamine, and sodium pyruvate (Corning). Before the cell cultivation, both media were supplemented with 10% fetal bovine serum (Biowest), 100 U·ml−1 penicillin, and 100 µg·ml−1 streptomycin (Life Technologies Gibco). The cells were incubated in a 5% CO2 humidified incubator at 37 °C.
2.6.2. Cytotoxicity assay.
A test on the in vitro cytotoxicity of the chitosan sample was performed by MTT assay against both cancer and normal cells. Firstly, cells were seeded into 96-well microliter plates (104 cells/well) and incubated in a 5% CO2 humidified incubator at 37 °C for 24 h. After that, different concentrations of chitosan samples, diluted with sterile water, were added into the wells to make the desired final concentration (0.1 to 5 mg·ml−1) and further incubated in an incubator at 37 °C and 5% CO2. After 24 h incubation, the medium was discarded and replaced with phosphate buffer saline solution (PBS) containing 0.05% (w/v) of 3-(4,5-dimethylthiazol-2-yl)-2,5-diphenyltetrazolium bromide (MTT) solution with further incubation for 3 h. Then, the solution was discarded and DMSO was added to dissolve the formed formazan crystals and the absorbance (A) of each well was measured at 570 nm using a microplate reader (Thermo Fisher Scientific Varioskan™ Flash Multimode Reader). The cell viability was calculated using the following equation:

The percentage cell viability was plotted versus the concentration of the COS sample to determine the mean growth-inhibitory concentration (IC50) value. All experiments were repeated five times. Doxorubicin was used as a positive control.
3. Results and discussion
3.1. Degradation of chitosan hydrogel
A comparison of the morphology of native chitosan flakes and freeze-dried chitosan hydrogel was performed by using FE-SEM, and the FE-SEM images are shown in Fig. 3(a). The FE-SEM image revealed that the chitosan flakes were composed of well-packed multilayered sheets. After dissolving chitosan flakes in the acetic acid solution, followed by reprecipitation in the NaOH solution to form chitosan hydrogel, intra- and intermolecular hydrogen bonding interactions within the structure of native chitosan were disrupted. The dissolution and regeneration of chitosan hydrogel resulted in the loss of the former well-packed multilayered structure, leading to a more amorphous region in chitosan hydrogel compared with native chitosan.50)
Download figure:
Standard image High-resolution imageFig. 3. (a) FE-SEM images and (b) WAXD spectra of native chitosan, freeze-dried, and wet chitosan hydrogel samples.
Download figure:
Standard image High-resolution imageMoreover, the change in the crystalline structure of native chitosan and chitosan hydrogel was examined by WAXD analysis, as shown in Fig. 3(b). The WAXD spectrum of native chitosan showed that the main characteristic peaks of native chitosan appeared at 2θ = 9.8 and 19.9°. As reported in the previous works,51,52) the reflection of 2θ around 9–10.5° indicated that chitosan had a hydrate polymorph. In the hydrate tendon crystal, the chain conformation appeared as a 2-fold helix stabilized by a hydrogen bond (O3•••O5) with the orientation of O6. Hydrogen bonds also occurred between adjacent chains (via N2•••O6) to form a sheet structure. These sheets stacked together in a parallel fashion and could be stabilized by water molecules that presented inside the chitosan structure.52) Upon the transformation of native chitosan to hydrogel, the crystalline structure in native chitosan was changed. The WAXD result of chitosan hydrogel exhibited clearly broader peaks at 2θ = 9.8°. The characteristic peak at 2θ = 19.9° disappeared, while two new broad peaks at 2θ = 28.1 and 35.0° could be observed. The WAXD result suggested that the crystalline structure of native chitosan was destroyed. The peaks of 2θ at 28.1 and 35.0° indicated the formation of chitosan acetate salt as reported by Modrzejewska et al.53) According to this evidence, the chitosan hydrogel had lower crystallinity than native chitosan and should be more susceptible to degradation by the SP treatment.
The degradation of chitosan hydrogel dispersed in the dilute acetic acid by applying the SP treatment and the conventional heat treatment were conducted under the same condition. The chitosan hydrogel was found to disperse well in the dilute acetic acid solutions. It was found that the weight-average molecular weight (Mw) of chitosan hydrogel after SP treatment dramatically decreased, while the slight reduction of Mw for chitosan hydrogel by the conventional heating treatment occurred, as shown in Table I. Furthermore, the degradation of chitosan hydrogel dispersed in the dilute acetic acid by the SP treatment was performed for comparison with the degradation of chitosan solution dissolved in 1 M acetic acid by SP treatment, which has been studied in some previous works.29,39,40) It was found that the Mw values of both types of chitosan samples were greatly reduced after the SP treatment. From GPC measurement, the degradation of chitosan hydrogel dispersed in the dilute acetic acid by applying the SP treatment for 60 min could reduce Mw for chitosan by approximately 98%. On the other hand, Mw for chitosan in the chitosan solution was decreased by 95% under the same condition. Therefore, it might be concluded that chitosan hydrogel dispersed in the dilute acetic acid was readily degraded by the SP treatment. Moreover, it also indicated that the SP treatment is an effective tool for promoting the degradation of chitosan to produce COS, even though only a dilute organic acid solution was present in the reaction.
Figure 4 shows flow charts giving a comparison of the experimental procedures for the degradation of chitosan solution and chitosan hydrogel to produce COS by SP treatment employed in this study and other previous works.19,29) At a high concentration of acetic acid (pH ≈ 4.0), chitosan can dissolve well because most NH2 groups at the C2 position in the structure of chitosan are protonated by protons from the dissociation of acetic acid and become soluble under an acidic environment.36) However, at a very low concentration (pH ≈ 6.5) of acetic acid, only a small number of NH2 groups in chitosan can be protonated due to the lack of protons and consequently, the chitosan hydrogel still remains as a suspension in the dilute acetic acid solution. Accordingly, the water-soluble degraded product of COS obtained from the SP treatment of the chitosan hydrogel dispersed in the dilute acetic acid could be easily separated from the remaining solid fractions by using simple centrifugation. The COS dissolving in the solution was collected as a supernatant, while the solid fraction that contained water-insoluble, high-molecular-weight chitosan was separated as a precipitate after centrifugation. On the other hand, the degraded product from the degradation of the chitosan solution in the presence of a high concentration of acetic acid by the SP treatment required neutralization by using NaOH in order to separate the water-insoluble, high-molecular-weight chitosan from the water-soluble, low-molecular-weight product that contained COS. Furthermore, the addition of a non-solvent such as ethanol or acetone was necessary for separation of the COS products from the solution as a precipitate. According to the flow charts in Fig. 4, the production of COS from chitosan hydrogel was simpler and less time-consuming than that obtained from chitosan solution.
Fig. 4. Comparison of the experimental procedures for the degradation of chitosan solution and chitosan hydrogel to produce COS by SP treatment.
Download figure:
Standard image High-resolution imageIn addition to acetic acid, various types of other carboxylic acids were also used to disperse chitosan hydrogel in order to study their influence on the degradation of chitosan hydrogel by applying the SP treatment. The various types of other carboxylic acids including chemical structures, acid dissociation constants,54) number of carboxylic groups, and number of methylene groups on the backbone are shown in Table II. According to GPC analysis, the results on molecular weight reduction as a function of the SP treatment time are shown in Table III. Mw for the original chitosan hydrogel before the SP treatment was 4.8 × 105 Da. Mw was found to decrease for all types of carboxylic acids with increasing SP treatment time. For the addition of oxalic acid, the dramatic reduction of Mw was observed and the scale of molecular weight reduction was comparable to that obtained from acetic acid. Mw for the degraded products obtained from the systems containing acetic acid and oxalic acid was approximately 6 × 103 Da after 60 min of SP treatment. It was expected that the degraded products would contain COS having Mw less than 5 kDa30) as a major component. In the systems with the addition of malonic acid, succinic acid, glutaric acid, and pimelic acid, the SP treatment could reduce Mw for the chitosan hydrogel from 4.8 × 105 Da to the range of 7.1 × 103–1.3 × 104 Da, while by the addition of azelaic acid and citric acid, Mw for the degraded chitosan was equal to approximately 2 × 104 Da after the SP treatment for 60 min.
Table III. Mw (Da) and PDI of chitosan hydrogel at a solid content of 0.2% (dry weight) dispersed in various carboxylic acid solutions with a concentration of 1.55 mM after degradation via SP treatment for different reaction times.
SP treatment time (min) | Mw (PDI) | ||||||||
---|---|---|---|---|---|---|---|---|---|
Acetic acid | Oxalic acid | Malonic acid | Succinic acid | Glutaric acid | Adipic acid | Pimelic acid | Azelaic acid | Citric acid | |
0 | 4.8 × 105 (2.35) | 4.8 × 105 (2.35) | 4.8 × 105 (2.35) | 4.8 × 105 (2.35) | 4.8 × 105 (2.35) | 4.8 × 105 (2.35) | 4.8 × 105 (2.35) | 4.8 × 105 (2.35) | 4.8 × 105 (2.35) |
15 | 7.1 × 104 (4.20) | 7.1 × 104 (4.95) | 1.0 × 105 (5.64) | 1.0 × 105 (4.97) | 8.9 × 104 (5.82) | 8.9 × 104 (4.20) | 1.2 × 105 (7.50) | 1.4 × 105 (6.06) | 1.8 × 105 (3.76) |
30 | 2.5 × 104 (3.35) | 2.6 × 104 (3.67) | 3.9 × 104 (4.55) | 4.1 × 104 (4.50) | 3.4 × 104 (4.92) | 2.7 × 104 (3.35) | 4.0 × 104 (5.34) | 5.8 × 104 (6.72) | 1.2 × 105 (5.48) |
45 | 1.0 × 104 (2.74) | 1.0 × 103 (2.59) | 1.7 × 104 (3.68) | 1.8 × 104 (3.46) | 1.2 × 104 (3.13) | 1.6 × 104 (2.74) | 2.0 × 104 (4.03) | 2.3 × 104 (4.73) | 5.7 × 104 (4.20) |
60 | 6.7 × 103 (2.35) | 5.8 × 103 (2.22) | 9.2 × 103 (3.00) | 8.7 × 103 (2.62) | 7.1 × 103 (2.65) | 7.8 × 103 (2.35) | 1.3 × 104 (3.58) | 1.7 × 104 (4.75) | 2.5 × 104 (3.68) |
The rate constant of the degradation reaction of chitosan hydrogel was analyzed on the basis of the following linear relationship between inverse molecular weight (1/Mw) and reaction time (t) in previous reports:29,55)

where Mt is Mw for the sample at the reaction time (t), M0 is the initial Mw for the sample, M is the molecular weight of the monomer, k (min−1) or k' (mol g−1 min−1) is the rate constant, and t is the reaction time. The k values are shown in Table IV. The k value obtained by the system with the addition of oxalic acid was equal to 3.67 × 10−4 min−1, which was slightly higher than that for the addition of acetic acid (k = 3.48 × 10−4 min−1). Meanwhile, the addition of the other dicarboxylic acids led to lower k values than that obtained by the system with the addition of acetic acid. The obtained k values for the systems using dicarboxylic acids ranged from 1.36 × 10−4 to 3.67 × 10−4 min−1, which seemed to vary, depending on the number of methylene groups on the backbone. For the tricarboxylic acid, citric acid, the k value was the lowest among all the studied carboxylic acids. Nevertheless, the k values obtained in this study from the degradation of chitosan hydrogel dispersed in dilute carboxylic acids by applying the SP plasma treatment were comparable to those obtained from the degradation of chitosan solution by using concentrated H2O2 or using various types of energy with the k values ranging from 10−4 to 10−2 min−1.32,39,55,56)
Table IV. Rate constants (k) of the degradation reactions of chitosan hydrogel at a solid content of 0.2% (dry weight) dispersed in 1.55 mM carboxylic acid solutions by SP treatment and production yield of water-soluble COS produced by the degradation of chitosan hydrogel dispersed in various carboxylic acids at a concentration of 1.55 mM by applying SP treatment as well as their Mw, Mn, and PDI.
Acid | k × 10−4 (min−1) | COS | |||
---|---|---|---|---|---|
Yield (%) | Mn (Da) | Mw (Da) | PDI | ||
Acetic acid | 3.48 ± 0.05 | 68.3 ± 2.36 | 1861 | 2969 | 1.59 |
Oxalic acid | 3.67 ± 0.20 | 60.3 ± 6.85 | 1944 | 3344 | 1.72 |
Malonic acid | 2.37 ± 0.26 | 53.3 ± 7.17 | 2053 | 3482 | 1.69 |
Succinic acid | 2.39 ± 0.08 | 60.9 ± 4.71 | 2321 | 4484 | 1.93 |
Glutaric acid | 3.09 ± 0.01 | 63.1 ± 3.12 | 2181 | 4033 | 1.84 |
Adipic acid | 2.75 ± 0.02 | 60.3 ± 3.54 | 2008 | 3395 | 1.69 |
Pimelic acid | 1.80 ± 0.04 | 64.1 ± 6.56 | 2216 | 4032 | 1.81 |
Azelaic acid | 1.36 ± 0.03 | 40.0 ± 2.04 | 2133 | 4515 | 1.93 |
Citric acid | 0.75 ± 0.02 | 2.5 ± 0.21 | 1538 | 2330 | 1.51 |
For further investigation, the chemical structures of chitosan hydrogel before and after degradation by applying the SP treatment in the presence of various types of dilute carboxylic acids were examined by using FT-IR, and the FT-IR results are shown in Fig. 5. The absorption peaks of chitosan hydrogel before the SP treatment at the wavenumber of approximately 3450 cm−1 were assigned to the N–H and hydrogen bonded O–H stretching vibration and the peaks at the wavenumbers of 1650 and 1600 cm−1 were referred to the C=O stretching of the amide bond and the N–H bending vibrations of the secondary amide, respectively.6,15,57,58) The peaks at around 1420 and 1375 cm−1 were attributed to –CH2– bending with the orientation of the primary hydroxyl group in the polysaccharides and methyl group.59,60) The peak at 1070 cm−1 was assigned to the stretching of C–O–C.6) According to the FT-IR results, the overall chemical structures of the degraded chitosan hydrogel dispersed in various dilute carboxylic acids did not change after the SP treatment. For the degraded chitosan hydrogel obtained from the system with the addition of acetic acid, strong absorption at 1650 cm−1 was observed. This might indicate the ionic interaction between the protonated amino groups of chitosan and the carboxylate anion (–COO−) of the carboxylic acid.61) Normally, protons (H+) from the dissociation of acetic acid (CH3COOH) can protonate amino groups (–NH2) of chitosan to form ammonium groups (–NH3+).50) Then, acetate anions (CH3COO−), which have relatively small size and low steric hindrance, should be able to penetrate into the structure of chitosan and undergo ionic interaction with –NH3+. After that, these interactions could lead to an electrostatic repulsion between chitosan chains.19) Consequently, the structure of chitosan may freely expand and distribute in the solution, as demonstrated later in Fig. 7(a), leading to an increasing probability that radicals generated by plasma discharge could penetrate to the β-(1,4)-glycosidic linkages of chitosan and greatly promote the Mw reduction of chitosan hydrogel. For the addition of oxalic acid, the FT-IR spectra of the degraded product showed that the absorption at 1650 cm−1 became sharp and stronger than that of the degraded product obtained from the system with the addition of acetic acid. In the case of oxalic acid, which is a dicarboxylic acid with no methylene group, it was reported that its dissociation could be influenced by the phenomenon called the mesomeric effect.62) The mesomeric effect might lead to intramolecular hydrogen bonding between the first dissociated carboxylic group and the OH part of the undissociated group in the oxalic acid, which bears a partial positive charge. Similar to acetic acid, oxalic acid could interact with chitosan, but the interaction might be slightly stronger, leading to a slightly higher rate of degradation than that of acetic acid. Meanwhile, the FT-IR spectra of the degraded product from the system with the addition of other dicarboxylic acids and the tricarboxylic acid exhibited the characteristic peak at 1600 cm−1, which slightly shifted to lower wavenumbers, and the absorption at 1650 cm−1 became obvious but had lower intensity than that obtained by the addition of oxalic acid. According to the pKa values in Table II, all dicarboxylic acids and the tricarboxylic acid are able to dissociate in the water and can lead to the protonation of –NH2 in chitosan, similar to acetic acid and oxalic acid. The result might indicate that there were ionic interactions between the –NH3+ groups of chitosan and the –COO− groups of the carboxylic acids. However, since dicarboxylic acid and tricarboxylic acid contain two and three groups of –COO−, respectively, they could undergo ionic crosslinking or complexation with some adjacent chitosan chains, as reported in some previous studies.63–65) These ionic crosslinking or complexation might be the reason for the slower Mw reduction of chitosan hydrogel. Moreover, the result showed that the rate of the degradation of chitosan depended on the number of methylene groups in the structures of dicarboxylic acid and tricarboxylic acid.
Fig. 5. FT-IR spectra of the chitosan hydrogel before the SP treatment and the degraded chitosan hydrogel dispersed in various carboxylic acid solutions at a concentration of 1.55 mM after applying the SP treatment for 60 min.
Download figure:
Standard image High-resolution imageFig. 6. WAXD spectra of the chitosan hydrogel before SP treatment and the degraded chitosan hydrogel dispersed in various carboxylic acid solutions at a concentration of 1.55 mM after applying SP treatment for 60 min.
Download figure:
Standard image High-resolution imageFig. 7. (a) Possible ionic interaction and ionic crosslink between chitosan hydrogel and different types of carboxylic acids including monocarboxylic, dicarboxylic, and tricarboxylic acids. (b) Possible degradation mechanism of chitosan hydrogel dispersed in dilute acid solution by SP treatment.
Download figure:
Standard image High-resolution imageFigure 6 shows the WAXD patterns of chitosan hydrogel before the SP treatment and the degraded chitosan hydrogel after applying the SP treatment in the presence of various types of carboxylic acid solutions at a concentration of 1.55 mM. The presence of acetic acid during the SP treatment resulted in a degraded product with an amorphous structure and, in the case of oxalic acid, very low intensity of the peaks at 2θ = 19.6 and 22.2° was observed. This may imply that the destruction of the crystalline structure of chitosan was facilitated by the presence of acetic acid and oxalic acid during the SP treatment. Meanwhile, the addition of other dicarboxylic acids and the tricarboxylic acid gave peaks with relatively stronger intensity at 2θ = 19.6 and 22.2°. According to a previous study, the broad peak at around 2θ = 19 and 23° might be ascribed to the diffraction of the plane of the crystal region in the chitosan carboxylate.66) The chitosan carboxylate was induced by the formation of ionic interactions between the –NH3+ groups of chitosan and –COO− groups of the carboxylic acid. In the case of the dicarboxylic acids and tricarboxylic acid, they could undergo ionic crosslinking or complexation with some adjacent chitosan chains, leading to stabilization of the crystal structure of chitosan and, consequently, these two peaks could be clearly observed. In particular, the relatively high crystallinity of the degraded chitosan products from the systems with the addition of azelaic acid and citric acid was evidence that indicated some extent of crosslinking within the structure of degraded chitosan. Therefore, the WAXD results were consistent with the FT-IR results and could also be used to explain the reason that different rates of degradation were obtained when the chitosan hydrogel dispersed in the different carboxylic acids was degraded by SP treatment.
A schematic drawing of a possible degradation mechanism of chitosan hydrogel dispersed in various carboxylic acid solutions by applying SP treatment is shown in Fig. 7(b). The reaction solution containing only molecules of H2O, carboxylic acids, and chitosan was subjected to SP treatment. The major component in the system was H2O molecules. The plasma discharge can result in the excitation and ionization of H2O molecules.67) Electrons are emitted as a result of ionization (i.e., H2O → H2O+ + e−) and then continuously collide with the surrounding H2O molecules (i.e., e− + H2O → OH + −H) to produce radicals. In addition, the excitation of H2O molecules also directly leads to the formation of radicals (i.e., H2O* →
OH +
H). Both the ionization and excitation of H2O molecules by SP treatment rapidly produce
OH, which is necessary for the degradation of chitosan.60,68) The generated
OH could lead to the chain scission of the chitosan at β-1,4-glycosidic linkage which have been reported in the previous studies.29,55,60) The breakdown of the β-1,4-glycosidic linkage leads to the reduction of the molecular weight of chitosan.60) Moreover, the electron from the plasma discharge can also directly collide with a chitosan molecule, leading to the chain scission.
The degraded chitosan hydrogel obtained by SP treatment in the presence of various dilute carboxylic acids for 60 min was centrifuged in order to separate the degraded products into water-soluble and water-insoluble degraded products. The solid fraction consisted of water-insoluble chitosan, while the liquid fraction contained the water-soluble COS. Further purification after the centrifugation was unnecessary because a very low concentration of carboxylic acids was used in the reaction. Moreover, carboxylic acids are generally found in many natural products and are safe for use in food and drug production.69) After centrifugation, in most cases of the studied carboxylic acids, the production yield of water-soluble COS was in the range of 50 to 70% with the exception of azelaic acid and citric acid, where the production yields of water-soluble COS were 40% and less than 5%, respectively. The production yield in this study was comparable to that obtained by different methods in other previous studies, as shown in supplementary data (Table III). For further investigation of the molecular weight reduction, Mw, number-average molecular weight (Mn), and PDI of the water-soluble COS were determined by GPC, as shown in Table IV. On the basis of Mn, the major oligosaccharides in the water-soluble COS could be estimated for each carboxylic acid, as shown in Table V. Degradation of chitosan hydrogel dispersed in the dilute solution of citric acid could produce COS with the major oligosaccharide of 10-mers, while the addition of acetic acid, oxalic acid, and adipic acid resulted in the COS containing mainly oligosaccharides of 12-mers. The addition of malonic acid, glutaric acid, and azelaic acid could give the major oligosaccharides of 13-mers and the COS with the major oligosaccharide of 14-mers could be produced by the addition of succinic acid and pimelic acid. Therefore, it could be concluded that the degradation of chitosan hydrogel dispersed in the dilute solution of various carboxylic acids by applying SP treatment could produce COS with a DP ranging from 10 to 14, which are difficult to produce by enzymatic degradation.6) Besides, since the carboxylate anions still remained in the degraded products, they should undergo ionic interactions with the COS leading to the formation of COS-carboxylates.
Table V. Possible major oligosaccharides in the water-soluble COS-carboxylate samples with the evaluation of their anticancer activity.
COS sample | Possible major oligosaccharide | Evaluation of anticancer activity | ||
---|---|---|---|---|
IC50a) (mg·ml−1) | SIb) | |||
H460 | MRC-5 | |||
COS-acetate | 12 | 2.9 | 5.0 | 1.72 |
COS-oxalate | 12 | 2.6 | 3.7 | 1.42 |
COS-malonate | 13 | 2.0 | 4.5 | 2.25 |
COS-succinate | 14 | 1.6 | 5.0 | 3.13 |
COS-glutarate | 13 | 2.2 | 4.0 | 1.81 |
COS-adipate | 12 | 2.0 | 2.4 | 1.20 |
COS-pimelate | 14 | 1.6 | 3.5 | 2.19 |
COS-azalate | 13 | 2.4 | 3.7 | 1.54 |
COS-citrate | 10 | — | — | — |
COSc) | 13 | 5.0 | 6.5 | 1.30 |
a) IC50 is the concentration required to kill 50% of the cell population.
b) SI is the selectivity index (SI = IC50 of pure compound in a normal cell line/IC50 of the same pure compound in a cancer cell line).70,71)
c) COS was obtained from the precipitation of the degraded chitosan solution in 1 M acetic acid after SP treatment under the corresponding condition.
3.2. Evaluation of anticancer activity
To evaluate the anticancer activity and to determine the concentrations of the COS-carboxylate samples that could kill 50% of the cell population (IC50), H460 and MRC-5 were used as representatives of the cancer cell line and the normal cell line, respectively. The COS-carboxylate samples were tested at final concentrations ranging from 1 to 5 mg·ml−1. IC50 of the COS-carboxylate and the normal COS samples against cancer cells (H460) and normal cells (MRC-5) are shown in Table V. The morphology of H460 cells untreated and treated with the COS-carboxylate samples at a concentration of 5 mg·ml−1 is also shown in the online supplementary data at http://stacks.iop.org/JJAP/57/0102B5/mmedia (Fig. S1). The normal COS was obtained by following the protocol as reported in a previous work,19) using SP treatment of chitosan solution. For comparing the COS-carboxylate and the COS, the result suggested that the presence of carboxylate in the structure of the COS could enhance the anticancer activity. Among the studied COS-carboxylate samples, it was found that the COS-carboxylate samples with the majority of 14-mers, which were obtained from the degradation of chitosan hydrogel dispersed in succinic acid and pimelic acid, exhibited the best inhibitory effect on the growth of H460 cells (IC50 = 1.6 mg·ml−1). For cytotoxicity against MRC-5 cells, the COS-acetate and COS-succinate samples had the highest IC50 value at 5 mg·ml−1. Moreover, the selectivity index (SI) of the COS-carboxylate and COS samples could be determined by the IC50 ratio of a compound tested against cancer cells and normal cells. SI implies the difference in the cytotoxic activity of the tested sample against cancer and normal cells.70,71) Therefore, a high SI value indicates a higher selectivity for cytotoxic activity against H460 cells than MRC-5 cells. Among the studied COS-carboxylate samples, the SI of the COS-succinate had the highest value of 3.1. The COS-succinate was also found to have better selectivity to cancer cells than COS. Since the last decade, succinate has been extensively studied for the treatment of cancer, because it was found to be a key metabolic factor in the cancer-immune and may lead to cancer immunotherapies.72,73) Previous studies reported that vitamin E succinate showed an inhibitory effect on cancer cells and tumors.74,75) Therefore, the combination of COS and succinate, which exhibited better selectivity against cancer cells, could be recommended for further development as a potential anticancer agent. In addition, according to Table VI, the cell viability obtained for the COS-succinate was comparable to that of the COS prepared by enzymatic degradation. From this evidence, it was proved that SP treatment can be used to reduce the molecular weight of chitosan in order to obtain COS without changing the biological properties such as anticancer activity, which naturally belongs to COS.
Table VI. Anticancer activity of COS and COS derivatives prepared by different methods.
COS and COS derivatives | Preparation method | Cell lines | Cell viability (%) | SIa) | Ref. | ||
---|---|---|---|---|---|---|---|
At ∼0.5 mg·ml−1 | At ∼1.5 mg·ml−1 | At ∼15 mg·ml−1 | |||||
COS (DP1–5) | Enzymatic | HeLa | 48 | — | — | — | 20 |
COS (DP3–9) | Enzymatic | SMMC- | 80 | 65 | — | — | 76 |
7721 | |||||||
COS (DP2–9) | SP | HeLa | — | 95 | 45 | 1.5 | 19 |
MRC-5b) | — | 100 | 70 | ||||
COS-succinate | SP | H460 | 80 | 50 | — | 3.1 | This work |
(DP∼14) | MRC-5b) | 89 | 75 | — |
a) SI is the selectivity index (SI = IC50 of pure compound in a normal cell line/IC50 of the same pure compound in a cancer cell line).70,71)
b) Normal cell line.
4. Conclusions
Chitosan hydrogel was found to be a suitable form of chitosan to produce water-soluble COS via a heterogeneous degradation reaction by using solution plasma treatment. As a result of the further disruption of the crystalline structure of chitosan hydrogel, the molecular weight reduction of chitosan hydrogel was enhanced by the presence of a small amount of carboxylic acids, especially acetic acid and oxalic acid, during the degradation of chitosan hydrogel under the discharge of plasma. Since a dilute concentration of carboxylic acids was used and chitosan hydrogel still remained as an undissolved hydrogel, the separation process to remove water-soluble degraded products containing COS from the remaining chitosan hydrogel could be simply achieved by centrifugation. Owning to the application of simple processes with less chemical use, the production cost of COS can be significantly reduced. Although the addition of carboxylic acids to the suspension of chitosan hydrogel results in the formation of COS-carboxylate products, the presence of succinate anions in COS could enhance the inhibitory effect towards cancer cells, resulting in a higher value of selectivity index. In this study, we proposed a novel, simple process to produce COS with anticancer activity. The proposed process not only gives a high production yield of COS but can also reduce the production cost of COS.
Acknowledgments
CC would like to express thank to the Thailand Research Fund (TRF) for financial support through a Royal Golden Jubilee Ph.D. program (RGJ-Ph.D.: Grant Number PHD/0179/2556). This work was financially supported by the Thailand Research Fund (TRF) under contract number BRG5480008, the National Research Funding from the Government of Thailand given to Chulalongkorn University, and Japan Science and Technology Agency: Core Research for Evolutional Science and Technology (JST/CREST: Grant Number GJPMJCR12L1). Moreover, the authors would like to acknowlege Surapon Foods Public Co., Ltd. (Thailand) for providing the shrimp shells and Nagoya University (Japan) for providing solution plasma equipment. In addition, the authors would like to thank the Drug Discovery and Development Center at Thammasat University (Thailand) for providing all facilities that were used for the biological tests.
Biographies
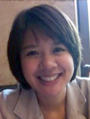
Chayanaphat Chokradjaroen is a Ph.D. student at the faculty of polymer science at the Petroleum and Petrochemical College, Chulalongkorn University, Thailand. She holds the bachelor's degree in chemical engineering from the Sirindhorn International Institute of Technology, Thammasat University, Thailand. For her further study, she continued and completed her master's degree at Kanazawa University, Japan. Currently, her research focuses on the degradation and the modification of chitin and chitosan, and the evaluation of biological activities of the obtained products.
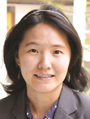
Sewan Theeramunkong is a lecture of faculty of pharmacy at Thammasat University, one of high ranked universities in Thailand. She holds the bachelor's degree in pharmacy, a master's degree in pharmaceutical chemistry from Thailand and a Ph.D. in medicinal chemistry from Italy. Prior to studying master's degree she had over 8 years of industrial quality control experience in Bangkok. During her Ph.D. period, she got internship in CEA-Saclay in France. Currently, her research focuses on the synthesis of novel anticancer agents and find out the mechanism of them.
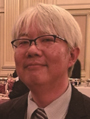
Nagahiro Saito is the research director for "Deepening of the Precise Reaction Field in Solution Plasma and Development of Advanced Carbon Catalyst" in JST/CREST research area; Establish of Molecular Technology towards the Creation of New Functions by Research Supervisor, Hisashi Yamamoto, Ph.D. The author is venturing into the novel and new organic reactions through solution plasma.
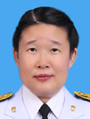
Ratana Rujiravanit is an associate professor of the Polymer Science Program at the Petroleum and Petrochemical College, Chulalongkorn University, Bangkok, Thailand. She received her master's and doctorate degrees from Hokkaido University, Japan. After that she earned post-doctoral fellowship at Japan Advanced Institute of Science and Technology (JAIST). Ratana's main research interest is the development of biopolymer-derived functionalized materials. Her published research works have been cited more than 2,000 times with the h-index of 27. One of her publication in Carbohydrate Polymers became one of the Top Ten Cited Articles in the year 2008–2010. In addition, her invention on bio-cellulose composite wound dressing received the Gold Medal Award from the 45th International Exhibition of Inventions, Geneva, Switzerland.