Recent Advances in the Discovery of Biomarkers for Canine Osteosarcoma
- 1Department of Biomedical Sciences, Ontario Veterinary College, University of Guelph, Guelph, ON, Canada
- 2Department of Pathobiology, Ontario Veterinary College, University of Guelph, Guelph, ON, Canada
Canine osteosarcoma (OSA) is an aggressive malignancy that frequently metastasizes to the lung and bone. Not only has there been essentially no improvement in therapeutic outcome over the past 3 decades, but there is also a lack of reliable biomarkers in clinical practice. This makes it difficult to discriminate which patients will most benefit from the standard treatment of amputation and adjuvant chemotherapy. The development of reliable diagnostic biomarkers could aid in the clinical diagnosis of primary OSA and metastasis; while prognostic, and predictive biomarkers could allow clinicians to stratify patients to predict response to treatment and outcome. This review summarizes biomarkers that have been explored in canine OSA to date. The focus is on molecular biomarkers identified in tumor samples as well as emerging biomarkers that have been identified in blood-based (liquid) biopsies, including circulating tumor cells, microRNAs, and extracellular vesicles. Lastly, we propose future directions in biomarker research to ensure they can be incorporated into a clinical setting.
Introduction
Osteosarcoma (OSA) is an aggressive neoplasm of the bone that is characterized by the production of osteoid (1). In canines, OSA accounts for 85% of tumors that arise in the bone and has an incidence rate of 13.9/100,000 per year, compared to 1.2/100,000 per year in humans (2, 3). Large breed (>40 kg) dogs that are middle-age and older are most affected, with a median age at diagnosis of 7 years (4). Most cases present in the appendicular skeleton, with the forelimb being more commonly impacted than the hindlimb. Dogs often present with lameness, swelling at the site, and pain. Diagnosis is based on a physical examination and radiographs of the lesion. Pre-operative histological analysis of the lesion offers a definitive diagnosis and can be performed on bone biopsies obtained through open incisional, closed needle or trephine biopsy techniques (1, 5). However, this procedure is quite invasive and increases the risk of pathological fracture. As such, fine needle aspirate of the bone lesion offers a less invasive diagnostic alternative (6–8). The initial work-up also involves thoracic radiographs to determine the presence of lung metastasis (9).
Treatment for canine OSA involves removal of the primary tumor through either limb-spare or limb amputation surgery. Patients that undergo surgery alone have a short median survival time of 101–177 days (~6 months) due to the development of lung metastases, the most common site of OSA metastasis (10–14). Other reported metastatic sites include bones, lymph nodes, skin and subcutaneous tissues (4, 15, 16). Although most canine OSA patients do not present with radiographically detectable metastatic disease at diagnosis, ~90–95% of canine OSA patients have micrometastases as is evident through the formation of macrometastases after removal of the primary tumor (17). Adjuvant chemotherapy treatment with carboplatin, cisplatin and doxorubicin post-surgery has been helpful in extending the median survival time to ~247–366 days, depending on the agent used (10, 11, 13, 14, 18–24). Despite this treatment approach, a majority of OSA patients will develop metastasis, a clinical problem in both human and canine OSA (25, 26).
Potential Utility of Biomarkers
The variability in the time it takes dogs to develop detectable metastasis after treatment, as well as variability in the overall survival (OS) time, suggests that patients respond differently to treatment; yet current practice effectively lumps all these patients into the same category of “poor prognosis.” Biomarkers could be particularly informative in this regard and are defined by the NIH Biomarkers Definitions Working Group as a “characteristic that can be objectively measured and evaluated as indicator of biological processes, pathogenic processes, or pharmacologic responses to a therapeutic intervention” (27). Biomarkers could be used as a diagnostic tool, to determine the extent of disease, to indicate disease prognosis, and to predict or monitor the clinical response to a particular intervention (Figure 1). This could benefit both the client and clinician as it would better predict a patient's disease course, allowing more informed decisions. Biomarkers could also specify the molecular features of a tumor and potentially permit a personalized treatment approach.
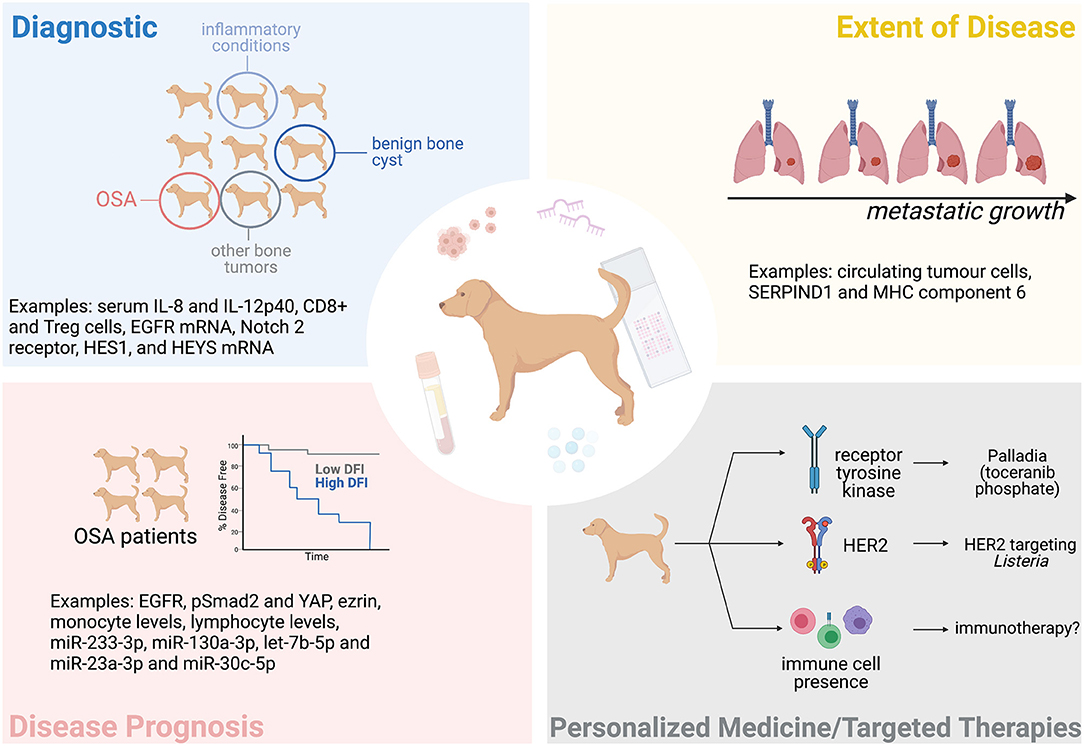
Figure 1. The potential of biomarkers in the clinical management of canine OSA. Tissue and blood-based (liquid) biopsies could help diagnose OSA, determine the extent of a patient's disease (metastatic progression), the patient's prognosis, and help personalize treatment. Some examples mentioned in this manuscript are indicated here. Figure created with BioRender.com.
Alkaline phosphatase (ALP) status, body weight, and location of the primary tumor are examples of proposed biomarkers. Greater weight at the time of diagnosis has been associated with metastasis; while primary lesions in the proximal humerus, distal femur, or proximal tibia and age are associated with an increased mortality (28). Two grading systems have also been developed by Kirpensteijn et al. (29) and Loukopoulos and Robinson (30), which was modified based on features described by Straw et al. (31). However, when both systems were evaluated in 85 canine appendicular OSA tumor samples that received standard of care (SOC) treatment (amputation and adjuvant chemotherapy), neither were effective at predicting disease outcomes (32). This lack of clinically relevant biomarkers may reflect the biological complexity of OSA, but can also be attributed to underpowered studies, lack of consistent treatment and other inclusion criteria, and poor-quality data on time of actual metastasis vs. euthanasia due to comorbidities such as cardiovascular and respiratory disease or osteoarthritis (33). This review summarizes literature that has explored the diagnostic and prognostic use of various tissue-based and blood-based (liquid biopsy) markers, see Table 1.
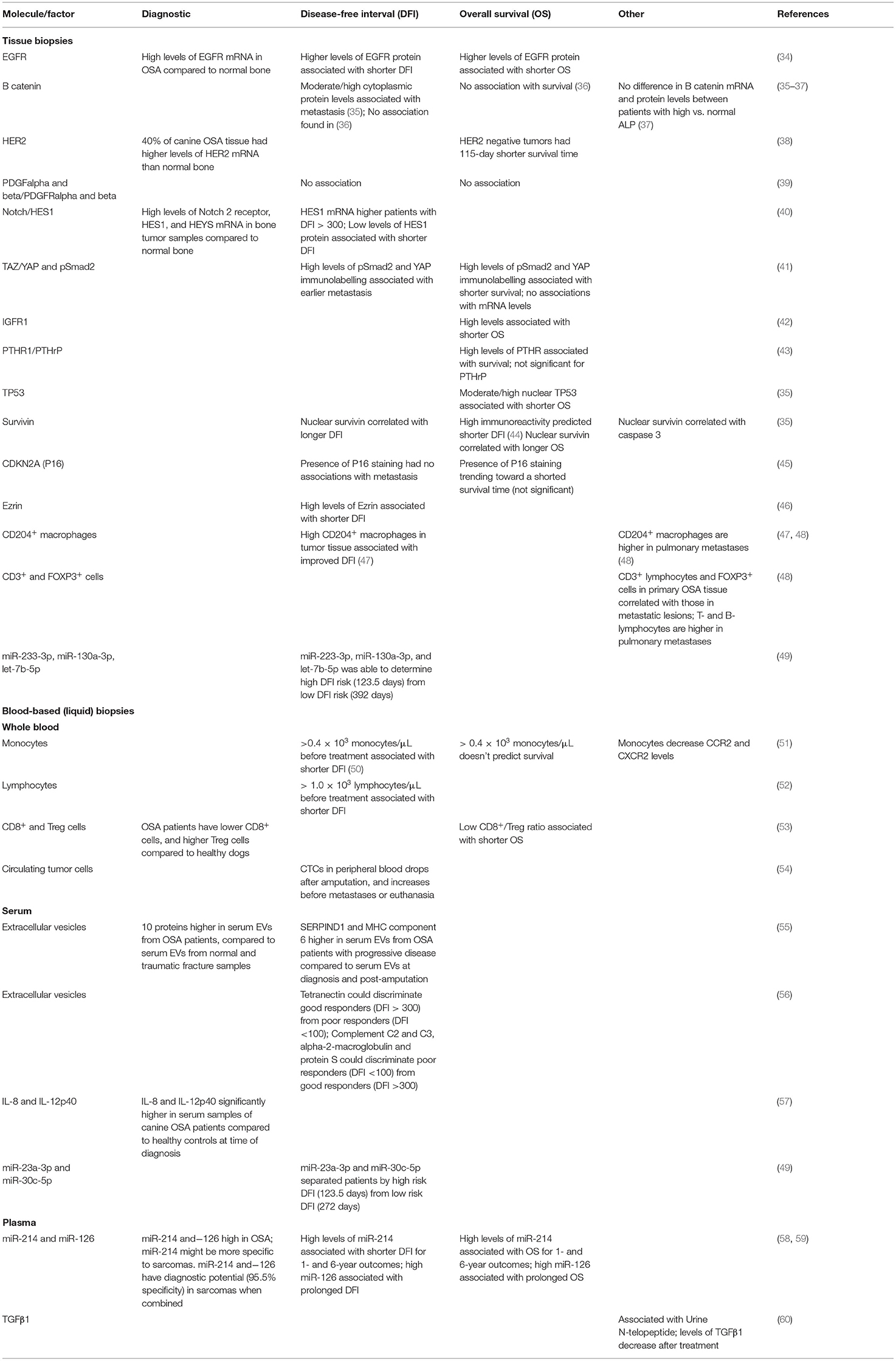
Table 1. Summary of biomarkers explored in canine appendicular OSA using tissue and blood-based (liquid) biopsies.
Tissue-Biopsy Based Markers
Molecular profiling of tumor tissue is an invaluable way to understand what signaling pathways and genes may be contributing to cancer progression. Gene expression microarray analysis found 51 genes to be differentially expressed between short survivors (<6 months) and long survivors (≥6 months). A majority of these genes were upregulated in the short survivors group and mediate drug resistance, proliferation, or metastasis. Pathways that were overactive in short survivors included Wnt, chemokine/cytokine, and integrin signaling (61). A similar analysis was completed by O'Donoghue et al., but they explored gene expression differences for dogs with disease free interval (DFI) <100 days, and > 300 days (62). Pathway analysis identified genes involved in cell adhesion, cytoskeletal remodeling, immune response, cAMP/protein kinase A (PKA) signaling, oxidative phosphorylation and bone development to be differentially expressed. Given the role of these pathways and cellular processes in promoting OSA progression, related molecules have been investigated in subsequent studies at the mRNA and protein level (see below) and found to correlate with patient outcomes.
Cell Signaling Pathways
The hyperactivation of several pathways have been suggested to promote the growth of OSA cells. The increased expression of receptor tyrosine kinases (RTK) can lead to enhanced downstream signaling and pro-tumourigenic phenotypes. OSA tumors have significantly higher levels of epidermal growth factor receptor (EGFR) mRNA compared to normal bone tissue. Analysis in a tissue microarray (TMA) found that patients with high levels of EGFR protein had shorter OS and DFI, compared to patients with low or negative levels (34). In the same family of EGFR is human epidermal growth factor receptor-2 (HER2 or ErbB2), a well-known proto-oncogene in mammary carcinomas. A small study involving 10 canine OSA tissue samples found that HER2 is significantly overexpressed in 40% of tumors compared to their normal counterparts. The sample size was not conducive for a robust statistical analysis in terms of patient outcome, but the authors noted that dogs that were HER2 negative had a 115-day longer survival time post-amputation (38). However, the antibody used to detect HER2 in this study is discontinued and there was no validation of specificity to canine HER2 conducted, so it is not clear that HER2 protein is expressed in canine OSA. Indeed, specificity of antibodies raised against human proteins and applied to canine proteins has been an issue in other studies. Burrai et al. tested a different HER2 antibody in canine mammary cancers and found unexpected cytoplasmic immunolabelling on immunohistochemistry (IHC), several bands of inappropriate molecular weights on Western blots, and no HER2 detection by mass spectrometry in any canine mammary tumors that were positive on IHC (63).
Insulin growth factor receptor 1 (IGFR1) and platelet derived growth factor (PDGF) signaling promote cancer cell proliferation and survival by activating PI3K/AKT and mitogen-activated kinase (MAPK) signaling. High levels of IGFR1 were found to be associated with reduced OS but no association was observed between IGFR1 levels and DFI (42). Neither PDGF ligands nor receptors were found to associate with DFI or OS (39). Notch signaling, mediated by a non-kinase receptor, has also been examined in canine OSA. Tumor tissue has increased mRNA expression of Notch2, and downstream mediators hairy ears, Y-linked (HEY), and hairy and enhancer of split 1 (HES1) compared to normal bone. However, when comparing patients with DFI <100 and DFI > 300 days, HES1 mRNA was significantly higher in the DFI > 300 cohort. This trend was also seen at the protein level, as higher HES1 protein immunoreactivity associated with a longer DFI (40).
Bone Development Pathways
Wnt signaling is involved in bone development, and its overactivation has been observed in OSA (64). Stein et al. found that a majority of canine primary OSA tumors were positive for β-catenin, but found no association with patient outcome (36). Others observed that β-catenin mRNA and protein levels did not differ between patients with elevated vs. normal ALP levels (37). The localization of β-catenin may be more reflective of OSA aggressiveness, as moderate/high levels of cytoplasmic β-catenin was predictive of metastasis in another study (35). Other well-known mediators of bone development are transforming growth factor beta (TGFβ) and Hippo signaling (65–67). Our findings suggest that TGFβ and Hippo signaling cooperate to promote OSA progression via activated SMAD2 and yes-associated protein (YAP), as high levels of phosho-SMAD2 and YAP in canine OSA tissue associated with reduced DFI and OS (41). Parathyroid hormone receptor 1 (PTHR1) is another known regulator of bone formation (68) and functions through cAMP/PKA signaling (69), the activity of which was shown to mediate OSA development in a p53 mutant model of OSA (70). High levels of PTHR1 in canine OSA tissue was associated with decreased OS (43).
Tumor Suppressors, Cell Cycle, and Cell Survival Mediators
The levels and localization of tumor suppressors and cell cycle mediators have also been explored in OSA tumors. The protein levels of TP53, PTEN, RB1, and CDKN2A (P16), which were previously found to be mutated in OSA, were assessed in a canine OSA TMA comprised of 150 cases (71). Differences in localization (nuclear, cytoplasmic or both), staining positivity (presence of staining), and staining intensity (darkness of staining) were demonstrated between tissue cores, although associations with outcome were not explored. Another study found that patients with high nuclear TP53 expression have a shorter OS (35). P16 expression was also evaluated in another study but did not consider localization. Though not statistically significant, patients with tumors with any immunoreactivity to P16 trended toward a shorter OS, compared to patients whose tumors had no immunoreactivity (45). To increase cell division and survival, cancer cells may upregulate the expression of survivin, an inhibitor of apoptosis (72). Surprisingly, a significant positive correlation was found between nuclear survivin levels and pro-caspase 3 levels, and DFI and OS (35). These results contrast those from another study that found patient with high levels of survivin immunoreactivity had a shorter median DFI of 173 days, compared to 331 days when immunoreactivity was low (44).
Immune Response
Previous studies have shown that developing an infection post-limb-spare surgery is a positive predictor for recurrence and survival, suggesting that an effective immune response could provide an anti-tumor effect (73, 74). However, this association between wound site infection and survival was not seen in patients undergoing amputation (75).
When considering dogs that had undergone limb-amputation and chemotherapy, high levels of CD204+ macrophages within the primary tumor associated with a longer DFI (47). This is an interesting finding given that CD204 is considered a marker of M2 tumor promoting macrophages, whose enrichment associates with poor prognosis in some epithelial tumors (76, 77). To determine how immune cell populations differ between the primary and metastatic lesion, Withers et al. compared the expression of various immune cell markers in 21 paired samples. It was found that CD3+ (T-lymphocytes), and FOXP3+ (T-regulatory) cells were positively correlated between the two sites. To determine if there were significant differences in immune cell infiltrates between the primary and metastatic lesion, CD3+, PAX-5 (B cell) and CD204+ (macrophage) levels were compared. All three cell types were significantly increased at the metastatic lesion, compared to the primary (48). These results suggest that the primary tumor could provide indication of the immune environment at the metastatic lesion. It should be noted that almost all the metastatic lesions were taken at necropsy. Thus, these results only represent advanced metastases, complicating their potential use in guiding immunotherapy approaches in the micrometastasis setting.
Metastasis
Metastasis is a complex process, and its success depends on many different factors [recently reviewed in (78)]. Of the proteins proposed to play a role in OSA metastasis, the cytoskeleton-to-membrane molecule, ezrin, is the best characterized. High ezrin expression in the primary tumor is an indicator of a short DFI, most likely due to ezrin's ability to facilitate lung colonization (46). Matrix metalloproteinases-2 and−9 (MMP-2/-9) are also expressed in primary OSA tissue, but no associations with outcome has been reported (50, 79, 80).
The aforementioned studies demonstrate the importance of various molecules in OSA progression in a patient relevant context and provide justification for the development and use of certain targeted therapies [i.e., tyrosine kinase receptor inhibitors (81, 82) and HER2-targeting Listeria (83)]. However, tissue biopsies only provide a static view of the tumor and do not account for tumor heterogeneity and evolution. Liquid biopsies could be particularly advantageous in this regard.
Blood-Based (Liquid) Biopsies
Liquid biopsies allow for the dynamic evaluation of pathological processes in bodily fluids such as blood and urine. They offer some advantages over tissue biopsies as they are minimally invasive and allow for serial monitoring throughout the course of a patient's treatment [see review in (84) for more comparisons]. This could be helpful in OSA since most canine patients undergo limb amputation, and tissue samples from secondary lung lesions are not easily accessible. As OSA predominately metastasizes via the blood stream (85), and blood serves as a conduit for both cells and cell products, many of the studies focus on blood as the source. The section below summarizes the limited literature in this field.
Immune Cells and Immune Cell Modulators
The blood contains a plethora of cells which can provide insight on the disease status of a patient. As complete blood counts are routinely completed during medical visits, evaluating the quantity of immune cells in circulation could be informative and a feasible way to monitor a patient over time. Sottnick et al. observed that high levels of monocytes (>0.4 × 103/μL) and lymphocytes (> 1 × 103/μL) before SOC treatment was associated with a significantly shorter DFI (52). This >0.4 × 103/μL monocyte cut off was unfortunately unable to predict OS in another study, possibly due to different inclusion criteria of patient cohorts (51).
When evaluating T lymphocytes specifically, it was found that the number of CD8+ cells was significantly lower, while the number of T regulatory cells was significantly higher in the blood of canine OSA patients compared to healthy controls. When considering both cell populations together, a low CD8+/T regulatory cell ratio was associated with a significantly shorter OS (53).
Aside from levels and ratios of specific number of immune cells, the levels of specific cytokines may also be informative. A preliminary study showed that serum levels of IL-8 and IL-12p40 was significantly higher in canine OSA patients compared to healthy controls (57). The plasma levels of TGFβ1, one of the three ligands that activate TGFβ signaling, was demonstrated to correlate with urine N-telopeptide, a marker of bone resorption that is significantly higher in OSA patients (86). TGFβ1 plasma levels were also lower after ionizing radiation and zolendronate treatment, suggesting that TGF β1 may be a good marker of bone resorption (60).
microRNAs
microRNAs are non-coding RNAs that are typically 18–25 nucleotides in length. miRNAs bind to target mRNAs to induce their degradation or inhibit their translation, thus modulating protein levels and cell behavior (87). miRNAs can be useful as circulating biomarkers as they enter circulation inside extracellular vesicles, like exosomes (88), and by forming complexes with proteins like Argonaute2 (89, 90).
Heishima et al. found that miR-214 and miR-126 are significantly elevated in the plasma of canine sarcoma patients when compared to controls. These microRNAs may be particularly relevant in OSA, as circulating levels were deemed high when compared to other malignancies explored in the study. Thus, miR-214 and miR-126 could potentially be a sensitive and accurate diagnostic marker when considered in combination, but this will have to be confirmed for OSA specifically (58). A later follow-up study evaluated miR-214 and miR-126 levels in the pre-treatment plasma of canine OSA patients receiving SOC. Univariate analysis found that high miR-214 levels associated with a shorter DFI and OS for 1- and 6-year outcomes. The trend was not replicated for miR-126, as high levels were associated with a prolonged DFI and OS, but only when considering 1-year outcomes. An integrated analysis, which combined both miRNAs and serum ALP status, was more effective at predicting DFS and OS compared to ALP alone (59). A recent study evaluated miRNA expression in canine OSA tumor tissue and serum to identify associations with patient outcome. A three-miRNA model, miR-233-3p, miR-130a-3p, and let-7b-5p, was able to predict high risk (DFI = 123.5 days) from low risk (DFI = 392 days) patients when evaluated in tumor tissue. Serum miR-23a-3p and miR-30c-5p combined were also identified to have prognostic value and separated high risk (DFI = 123.5 days) from low risk (DFI = 272 days) patients (49). These are the only studies that reported associations between circulating miRNAs and outcome in canine OSA. Another study explored a miRNA-associated signature in OSA tumor tissue. It found that patients with an increased expression of a gene signature associated with miR-382 had a shorter OS than those that had a decreased expression (91).
Circulating Tumor Cells
CTCs are cells released from the primary lesion, either through active intravasation or passive shedding. CTCs can then enter the blood stream individually, or in clumps, where they have to survive and eventually arrest at metastatic sites or get stuck in capillary beds (92). The appearance of CTCs in the peripheral blood of canines with metastatic mammary carcinoma is associated with poor survival outcomes (93).
In OSA, Wright et al. demonstrated that CTCs can be detected in the blood by flow cytometry. A majority of canine OSA CTCs are positive for collagen I (COLI) and weakly positive for osteocalcin (OCN). In an analysis of 3 patients, it was found that the number of CTCs declined after amputation but increased before metastatic disease or euthanasia (54).
Extracellular Vesicles
Extracellular vesicles, or EVs, is a broad term that applies to membrane-bound vesicles released by cells into the extracellular environment. The cargo of EVs varies in biomolecule type and differs between cancers (94). These two features, as well as the high abundance of EVs in the blood, ~1010 EVs/1 mL of plasma (95), makes them extremely attractive cancer biomarkers.
To date, only two studies have explored the biomarker potential of circulating EVs in canine OSA. In one study, authors compared the protein content in a specific type of EV, known as exosomes, from the sera of dogs with OSA, dogs that suffered a fracture, and normal donors. From this analysis, 10 proteins were successfully able to distinguish OSA from the other groups with an 85% accuracy. To determine if the exosomal protein content changed throughout OSA progression, the sera of five dogs were compared pre-amputation, 2 weeks post-amputation and upon detection of progressive disease. SERPIND1 and major histocompatibility complex (MHC) class III component C6 were both able to distinguish the OSA by their various stages with 77% accuracy (55). In relation to outcome, a recent study evaluated exosomes in the sera of 10 canine OSA patients that were defined as poor responders (DFI <100 days) and good responders (DFI > 300 days). Exosomal tetranectin predicted a good prognosis, while the complement C2 and C3, alpha-2-macroglobulin and protein S predicted a poor prognosis (56). These results are encouraging but preliminary and warrant further investigation in larger patient cohorts.
Looking Forward: Barriers to Overcome to Improve Clinical Applicability
The studies reviewed herein provided valuable information on OSA biology, but multiple barriers prevent the clinical application of the proposed biomarkers. Some of the studies compared only a handful of patients, leading to problems with statistical power (96). Almost all the findings described above are based on single studies, each using different quantification methods for their molecule of interest and creating different cut-offs for their desired clinical comparison. Altogether, this makes it extremely difficult to determine which biomarker candidates are most promising, extrapolate these findings to larger cohorts of OSA patients, and precludes their clinical use. Increased multi-institutional collaborative studies would increase patient recruitment (leading to larger sample sizes), determine the replicability of previous findings, and maximize the use of patient samples by screening multiple tissue-based and blood-based markers within the same patient.
The feasibility of evaluating some of the above markers in a clinical setting will also need to be improved. Isolating miRNA and EVs and detecting CTCs and blood cells require specialized equipment and reagents which makes the pipeline from sample acquisition to data acquisition cumbersome. Certain technologies, such as microfluidic devices (97) or synthetic peptides (98) would allow a more rapid isolation of EVs and multi-omic profiling (99). However, this will first require antibody validation or cross-species molecular approaches to permit the rapid detection of biomarkers in canine samples. These validation efforts need to also extend to antibodies used in cytokine studies. Human and canine cytokine amino acid sequences share 49–96% homology, but proper validation is necessary to confirm antibody cross-reactivity between species (100). Canine-specific cytokine assays may be advantageous as diagnostic tools but would also require appropriate and rigorous validation.
Given these obstacles, it is imperative that future biomarker research follows a critical discovery, validation, and clinical translation pipeline. The study populations included in the discovery and validation studies need to be well-documented, ideally with genetic background of the patient's tumor (given the chaotic nature of OSA), adequate history of treatments received, and complete dates of defined clinical endpoints. If the biomarker proves useful, focus should then be directed to developing technologies to allow its use in a clinic setting. As blood-based (liquid) biopsies are easier to access, permit serial sampling, and potentially have an easier clinical translation, future studies should focus on blood-based (liquid) biomarkers.
Conclusion
Canine OSA lacks reliable diagnostic, prognostic, and predictive biomarkers. Several tissue-based and liquid-based biomarkers have been proposed in the literature. These studies showcase the potential of biomarkers to predict patient response to therapy, determine the presence of metastasis, predict patient outcomes, and allow for personalized medicine. Confirming the results in larger cohorts through collaboration and increasing the feasibility of evaluating these molecules in a diagnostic laboratory setting will bring biomarkers one step closer to becoming a clinical reality for canine OSA patients.
Author Contributions
AL and AV-P conceived the ideas to develop the manuscript. AL drafted the manuscript and prepared all figures and tables. AV-P and GW revised the manuscript, figure, and table. All authors edited and approved the final manuscript.
Funding
AV-P studies on canine OSA biomarkers are funded by OVC Pet Trust Grant #053666. GW studies on canine OSA biomarkers are funded by OVC Pet Trust Grant # 053527.
Conflict of Interest
The authors declare that the research was conducted in the absence of any commercial or financial relationships that could be construed as a potential conflict of interest.
Publisher's Note
All claims expressed in this article are solely those of the authors and do not necessarily represent those of their affiliated organizations, or those of the publisher, the editors and the reviewers. Any product that may be evaluated in this article, or claim that may be made by its manufacturer, is not guaranteed or endorsed by the publisher.
Acknowledgments
We thank members of the OVC Dog Osteosarcoma Group – Biomarkers of Neoplasia (DOGBONe) for fruitful discussions that encourage the research leading to this manuscript.
References
1. Straw RC, Withrow SJ, Powers BE. Management of canine appendicular osteosarcoma. Vet Clin North Am Small Anim Pract. (1990) 20:1141–61. doi: 10.1016/S0195-5616(90)50089-3
2. Alexander JW, Patton CS. Primary tumors of the skeletal system. Vet Clin North Am Small Anim Pract. (1983) 13:181–95. doi: 10.1016/S0195-5616(83)50011-9
3. Rowell JL, McCarthy DO, Alvarez CE. Dog models of naturally occurring cancer. Trends Mol Med. (2011) 17:380–8. doi: 10.1016/j.molmed.2011.02.004
4. Dernell W, Ehrhart N, Straw R, Vail D. Tumors of the skeletal system. In: Withrow & MacEwen's Small Animal Clinical Oncology. Amstrdam: Elsevier. p. 540–82.
5. Berg J. Canine Osteosarcoma. Vet Clin North Am Small Anim Pract. (1996) 26:111–21. doi: 10.1016/S0195-5616(96)50010-0
6. Sabattini S, Renzi A, Buracco P, Defourny S, Garnier-Moiroux M, Capitani O, et al. Comparative assessment of the accuracy of cytological and histologic biopsies in the diagnosis of canine bone lesions. J Vet Intern Med. (2017) 31:864–71. doi: 10.1111/jvim.14696
7. Chun R, de Lorimier LP. Update on the biology and management of canine osteosarcoma. Vet Clin North Am Small Anim Pract. (2003) 33:491–516. doi: 10.1016/S0195-5616(03)00021-4
8. Britt T, Clifford C, Barger A, Moroff S, Drobatz K, Thacher C, et al. Diagnosing appendicular osteosarcoma with ultrasound-guided fine-needle aspiration: 36 cases. J Small Anim Pract. (2007) 48:145–50. doi: 10.1111/j.1748-5827.2006.00243.x
9. Endicott M. Principles of treatment for osteosarcoma. Clin Tech Small Anim Pract. (2003) 18:110–4. doi: 10.1053/svms.2003.36626
10. Thompson JP, Fugent MJ. Evaluation of survival times after limb amputation, with and without subsequent administration of cisplatin, for treatment of appendicular osteosarcoma in dogs: 30 cases (1979-1990). J Am Vet Med Assoc. (1992) 200:531–3.
11. Shapiro W, Fossum TW, Kitchell BE, Couto CG, Theilen GH. Use of cisplatin for treatment of appendicular osteosarcoma in dogs. J Am Vet Med Assoc. (1988) 192:507–11.
12. Spodnick GJ, Berg J, Rand WM, Schelling SH, Couto G, Harvey HJ, et al. Prognosis for dogs with appendicular osteosarcoma treated by amputation alone: 162 cases (1978-1988). J Am Vet Med Assoc. (1992) 200:995–9.
13. Mauldin GN, Matus RE, Withrow SJ, Patnaik AK. Canine osteosarcoma. Treatment by amputation versus amputation and adjuvant chemotherapy using doxorubicin and cisplatin. J Vet Intern Med. (1988) 2:177–80. doi: 10.1097/00003086-196901000-00008
14. Straw RC, Withrow SJ, Richter SL, Powers BE, Klein MK, Postorino NC, et al. Amputation and cisplatin for treatment of canine osteosarcoma. J Vet Intern Med. (1991) 5:205–10. doi: 10.1111/j.1939-1676.1991.tb00950.x
15. Hillers KR, Dernell WS, Lafferty MH, Withrow SJ, Lana SE. Incidence and prognostic importance of lymph node metastases in dogs with appendicular osteosarcoma: 228 cases (1986-2003). J Am Vet Med Assoc. (2005) 226:1364–7. doi: 10.2460/javma.2005.226.1364
16. Parachini-Winter C, Curran KM, Pellin M, Laver T, Hanot C, Vernier TH, et al. Cutaneous and subcutaneous metastasis of appendicular osteosarcoma in dogs: 20 cases. J Vet Intern Med. (2019) 33:2200–8. doi: 10.1111/jvim.15557
17. MacEwen EG, Kurzman ID. Canine osteosarcoma. Amputation and chemoimmunotherapy. Vet Clin North Am Small Anim Pract. (1996) 26:123–33. doi: 10.1016/s0195-5616(96)50011-2
18. Lane A, Black M, Wyatt K. Toxicity and efficacy of a novel doxorubicin and carboplatin chemotherapy protocol for the treatment of canine appendicular osteosarcoma following limb amputation. Aust Vet J. (2012) 90:69–74. doi: 10.1111/j.1751-0813.2011.00878.x
19. Berg J, Weinstein MJ, Schelling SH, Rand WM. Treatment of dogs with osteosarcoma by administration of cisplatin after amputation or limb-sparing surgery: 22 cases (1987-1990). J Am Vet Med Assoc. (1992) 200:2005–8.
20. Phillips B, Powers BE, Dernell WS, Straw RC, Khanna C, Hogge GS, et al. Use of single-agent carboplatin as adjuvant or neoadjuvant therapy in conjunction with amputation for appendicular osteosarcoma in dogs. J Am Anim Hosp Assoc. (2015) 45:33–8. doi: 10.5326/0450033
21. Bergman PJ, MacEwen EG, Kurzman ID, Henry CJ, Hammer AS, Knapp DW, et al. Amputation and carboplatin for treatment of dogs with osteosarcoma: 48 cases (1991 to 1993). J Vet Intern Med. (1996) 10:76–81. doi: 10.1111/j.1939-1676.1996.tb02031.x
22. Frimberger AE, Chan CM, Moore AS. Canine osteosarcoma treated by post-amputation sequential accelerated doxorubicin and carboplatin chemotherapy: 38 cases. J Am Anim Hosp Assoc. (2016) 52:149–56. doi: 10.5326/JAAHA-MS-6315
23. Berg J, Weinstein MJ, Springfield DS, Rand WM. Results of surgery and doxorubicin chemotherapy in dogs with osteosarcoma. J Am Vet Med Assoc. (1995) 206:1555–60.
24. Chun R, Garrett LD, Henry C, Wall M, Smith A, Azene NM. Toxicity and efficacy of cisplatin and doxorubicin combination chemotherapy for the treatment of canine osteosarcoma. J Am Anim Hosp Assoc. (2005) 41:382–7. doi: 10.5326/0410382
25. LeBlanc AK, Mazcko CN. Improving human cancer therapy through the evaluation of pet dogs. Nat Rev Cancer. (2020) 20:727–42. doi: 10.1038/s41568-020-0297-3
26. Selmic LE, Burton JH, Thamm DH, Withrow SJ, Lana SE. Comparison of carboplatin and doxorubicin-based chemotherapy protocols in 470 dogs after amputation for treatment of appendicular osteosarcoma. J Vet Intern Med. (2014) 28:554–63. doi: 10.1111/jvim.12313
27. Biomarkers Definitions Working Group. Biomarkers and surrogate endpoints: preferred definitions and conceptual framework. Clin Pharmacol Ther. (2001) 69:89–95. doi: 10.1067/mcp.2001.113989
28. Schmidt AFF, Nielen M, Klungel OHH, Hoes AWW, de Boer A, Groenwold RH, et al. Prognostic factors of early metastasis and mortality in dogs with appendicular osteosarcoma after receiving surgery: an individual patient data meta-analysis. Prev Vet Med. (2013) 112:414–22. doi: 10.1016/j.prevetmed.2013.08.011
29. Kirpensteijn J, Kik M, Rutteman GR, Teske E. Prognostic significance of a new histologic grading system for canine osteosarcoma. Vet Pathol. (2002) 39:240–6. doi: 10.1354/vp.39-2-240
30. Loukopoulos P, Robinson WF. clinicopathological relevance of tumour grading in canine osteosarcoma. J Comp Pathol. (2007) 136:65–73. doi: 10.1016/j.jcpa.2006.11.005
31. Straw R, Powers B, Klausner J, Henderson R, Morrison W, McCaw D, et al. Canine mandibular osteosarcoma: 51 cases (1980-1992). J Am Anim Hosp Assoc. (1996) 32:257–62. doi: 10.5326/15473317-32-3-257
32. Schott CR, Tatiersky LJ, Foster RA, Wood GA. Histologic grade does not predict outcome in dogs with appendicular osteosarcoma receiving the standard of care. Vet Pathol. (2018) 55:202–11. doi: 10.1177/0300985817747329
33. Meuten D, Munday JS, Hauck M. Time to standardize? Time to validate? Vet Pathol. (2018) 55:195–9. doi: 10.1177/0300985817753869
34. Selvarajah GT, Verheije MH, Kik M, Slob A, Rottier PJM, Mol JA, et al. Expression of epidermal growth factor receptor in canine osteosarcoma: association with clinicopathological parameters and prognosis. Vet J. (2012) 193:412–9. doi: 10.1016/j.tvjl.2012.02.009
35. Bongiovanni L, Mazzocchetti F, Malatesta D, Romanucci M, Ciccarelli A, Buracco P, et al. Immunohistochemical investigation of cell cycle and apoptosis regulators (Survivin, β-Catenin, P53, Caspase 3) in canine appendicular osteosarcoma. BMC Vet Res. (2012) 8:78. doi: 10.1186/1746-6148-8-78
36. Stein TJ, Holmes KE, Muthuswamy A, Thompson V, Huelsmeyer MK. Characterization of β-catenin expression in canine osteosarcoma. Vet Comp Oncol. (2011) 9:65–73. doi: 10.1111/j.1476-5829.2010.00236.x
37. Piskun CM, Muthuswamy A, Huelsmeyer MK, Thompson V, Stein TJ. Wnt/β-catenin expression does not correlate with serum alkaline phosphatase concentration in canine osteosarcoma patients. PLoS ONE. (2011) 6:e26106. doi: 10.1371/journal.pone.0026106
38. Flint AF, U'Ren L, Legare ME, Withrow SJ, Dernell W, Hanneman WH. Overexpression of the erbB-2 proto-oncogene in canine osteosarcoma cell lines and tumors. Vet Pathol. (2004) 41:291–6. doi: 10.1354/vp.41-3-291
39. Maniscalco L, Iussich S, Morello E, Martano M, Biolatti B, Riondato F, et al. PDGFs and PDGFRs in canine osteosarcoma: new targets for innovative therapeutic strategies in comparative oncology. Vet J. (2013) 195:41–7. doi: 10.1016/j.tvjl.2012.05.003
40. Dailey DD, Anfinsen KP, Pfaff LE, Ehrhart E, Charles J, Bønsdorff TB, et al. HES1, a target of Notch signaling, is elevated in canine osteosarcoma, but reduced in the most aggressive tumors. BMC Vet Res. (2013) 9:130. doi: 10.1186/1746-6148-9-130
41. Luu AK, Schott CR, Jones R, Poon AC, Golding B, Hamed R, et al. An evaluation of TAZ and YAP crosstalk with TGFβ signalling in canine osteosarcoma suggests involvement of hippo signalling in disease progression. BMC Vet Res. (2018) 14:365. doi: 10.1186/s12917-018-1651-5
42. Maniscalco L, Iussich S, Morello E, Martano M, Gattino F, Miretti S, et al. Increased expression of insulin-like growth factor-1 receptor is correlated with worse survival in canine appendicular osteosarcoma. Vet J. (2015) 205:272–80. doi: 10.1016/j.tvjl.2014.09.005
43. Al-Khan AA, Nimmo JS, Tayebi M, Ryan SD, Simcock JO, Tarzi R, et al. Parathyroid hormone receptor 1 (PTHR1) is a prognostic indicator in canine osteosarcoma. Sci Rep. (2020) 10:1564. doi: 10.1038/s41598-020-58524-3
44. Shoeneman JK, Ehrhart EJ, Eickhoff JC, Charles JB, Powers BE, Thamm DH. Expression and function of survivin in canine osteosarcoma. Cancer Res. (2012) 72:249–59. doi: 10.1158/0008-5472.CAN-11-2315
45. Murphy BG, Mok MY, York D, Rebhun R, Woolard KD, Hillman C, et al. Evaluation of P16 expression in canine appendicular osteosarcoma. BMC Vet Res. (2017) 13:189. doi: 10.1186/s12917-017-1113-5
46. Khanna C, Wan X, Bose S, Cassaday R, Olomu O, Mendoza A, et al. The membrane-cytoskeleton linker ezrin is necessary for osteosarcoma metastasis. Nat Med. (2004) 10:182–6. doi: 10.1038/nm982
47. Withers SS, Skorupski KA, York D, Choi JW, Woolard KD, Laufer-Amorim R, et al. Association of macrophage and lymphocyte infiltration with outcome in canine osteosarcoma. Vet Comp Oncol. (2019) 17:49–60. doi: 10.1111/vco.12444
48. Withers SS, York D, Choi JW, Woolard KD, Laufer-Amorim R, Sparger EE, et al. Metastatic immune infiltrates correlate with those of the primary tumour in canine osteosarcoma. Vet Comp Oncol. (2019) 17:242–52. doi: 10.1111/vco.12459
49. Dailey DD, Hess AM, Bouma GJ, Duval DL. MicroRNA expression changes and integrated pathways associated with poor outcome in canine osteosarcoma. Front Vet Sci. (2021) 8:637622. doi: 10.3389/fvets.2021.637622
50. Gebhard C, Fuchs-Baumgartinger A, Razzazi-Fazeli E, Miller I, Walter I. Distribution and activity levels of matrix metalloproteinase 2 and 9 in canine and feline osteosarcoma. Can J Vet Res. (2016) 80:66–73.
51. Tuohy JL, Lascelles BDX, Griffith EH, Fogle JE. Association of canine osteosarcoma and monocyte phenotype and chemotactic function. J Vet Intern Med. (2016) 30:1167–78. doi: 10.1111/jvim.13983
52. Sottnik JL, Rao S, Lafferty MH, Thamm DH, Morley PS, Withrow SJ, et al. Association of blood monocyte and lymphocyte count and disease-free interval in dogs with osteosarcoma. J Vet Intern Med. (2010) 24:1439–44. doi: 10.1111/j.1939-1676.2010.0591.x
53. Biller BJ, Guth A, Burton JH, Dow SW. Decreased ratio of CD8+ T cells to regulatory T cells associated with decreased survival in dogs with osteosarcoma. J Vet Intern Med. (2010) 24:1118–23. doi: 10.1111/j.1939-1676.2010.0557.x
54. Wright T, Brisson BA, Wood GA, Oblak M, Mutsaers AJ, Sabine V, et al. Flow cytometric detection of circulating osteosarcoma cells in dogs. Cytom Part A. (2019) 95:997–1007. doi: 10.1002/cyto.a.23847
55. Brady JV, Troyer RM, Ramsey SA, Leeper H, Yang L, Maier CS, et al. A preliminary proteomic investigation of circulating exosomes and discovery of biomarkers associated with the progression of osteosarcoma in a clinical model of spontaneous disease. Transl Oncol. (2018) 11:1137–46. doi: 10.1016/j.tranon.2018.07.004
56. Weinman MA, Ramsey SA, Leeper HJ, Brady JV, Schlueter A, Stanisheuski S, et al. Exosomal proteomic signatures correlate with drug resistance and carboplatin treatment outcome in a spontaneous model of canine osteosarcoma. Cancer Cell Int. (2021) 21:1–13. doi: 10.1186/s12935-021-01943-7
57. Allende C, Higgins B, Johns J. Comparison of serum cytokine concentrations between healthy dogs and canine osteosarcoma patients at the time of diagnosis. Vet Immunol Immunopathol. (2020) 227:110084. doi: 10.1016/j.vetimm.2020.110084
58. Heishima K, Ichikawa Y, Yoshida K, Iwasaki R, Sakai H, Nakagawa T, et al. Circulating microRNA-214 and−126 as potential biomarkers for canine neoplastic disease. Sci Rep. (2017) 7:2301. doi: 10.1038/s41598-017-02607-1
59. Heishima K, Meuten T, Yoshida K, Mori T, Thamm DH. Prognostic significance of circulating microRNA-214 and−126 in dogs with appendicular osteosarcoma receiving amputation and chemotherapy. BMC Vet Res. (2019) 15:39. doi: 10.1186/s12917-019-1776-1
60. Portela RF, Fadl-Alla BA, Pondenis HC, Byrum ML, Garrett LD, Wycislo KL, et al. Pro-tumorigenic effects of transforming growth factor beta 1 in canine osteosarcoma. J Vet Intern Med. (2014) 28:894–904. doi: 10.1111/jvim.12348
61. Selvarajah GT, Kirpensteijn J, van Wolferen ME, Rao NA, Fieten H, Mol JA. Gene expression profiling of canine osteosarcoma reveals genes associated with short and long survival times. Mol Cancer. (2009) 8:72. doi: 10.1186/1476-4598-8-72
62. O'Donoghue LE, Ptitsyn AA, Kamstock DA, Siebert J, Thomas RS, Duval DL. Expression profiling in canine osteosarcoma: identification of biomarkers and pathways associated with outcome. BMC Cancer.(2010) 10:506. doi: 10.1186/1471-2407-10-506
63. Burrai GP, Tanca A, De Miglio MR, Abbondio M, Pisanu S, Polinas M, et al. Investigation of HER2 expression in canine mammary tumors by antibody-based, transcriptomic and mass spectrometry analysis: is the dog a suitable animal model for human breast cancer? Tumor Biol. (2015) 36:9083–91. doi: 10.1007/s13277-015-3661-2
64. Day TF, Guo X, Garrett-Beal L, Yang Y. Wnt/Beta-catenin signaling in mesenchymal progenitors controls osteoblast and chondrocyte differentiation during vertebrate skeletogenesis. Dev Cell. (2005) 8:739–50. doi: 10.1016/j.devcel.2005.03.016
65. Yang J-Y, Cho SW, An JH, Jung JY, Kim SW, Kim SY, et al. Osteoblast-targeted overexpression of TAZ increases bone mass in vivo. PLoS ONE. (2013) 8:e56585. doi: 10.1371/journal.pone.0056585
66. Hong J-H, Yaffe MB. TAZ a beta-catenin-like molecule that regulates mesenchymal stem cell differentiation. Cell Cycle. (2005) 5:176–9. doi: 10.4161/cc.5.2.2362
67. Janssens K. ten Dijke P, Janssens S, Van Hul W. Transforming growth factor-beta1 to the bone. Endocr Rev. (2005) 26:743–74. doi: 10.1210/er.2004-0001
68. Martin TJ. Parathyroid hormone-related protein, its regulation of cartilage and bone development, and role in treating bone diseases. Physiol Rev. (2016) 96:831–71. doi: 10.1152/physrev.00031.2015
69. Swarthout JT, Tyson DR, Jefcoat SC, Partridge NC. Induction of transcriptional activity of the cyclic adenosine monophosphate response element binding protein by parathyroid hormone and epidermal growth factor in osteoblastic cells. J Bone Miner Res. (2002) 17:1401–7. doi: 10.1359/jbmr.2002.17.8.1401
70. Walia MK, Ho PM, Taylor S, Ng AJ, Gupte A, Chalk AM, et al. Activation of PTHrP-cAMP-CREB1 signaling following p53 loss is essential for osteosarcoma initiation and maintenance. Elife. (2016) 5:e13446. doi: 10.7554/eLife.13446
71. Russell DS, Jaworski L, Kisseberth WC. Immunohistochemical detection of p53, PTEN, Rb, and p16 in canine osteosarcoma using tissue microarray. J Vet Diagnostic Investig. (2018) 30:504–9. doi: 10.1177/1040638718770239
72. Ambrosini G, Adida C, Altieri DC. A novel anti-apoptosis gene, survivin, expressed in cancer and lymphoma. Nat Med. (1997) 3:917–21. doi: 10.1038/nm0897-917
73. Lascelles BDX, Dernell WS, Correa MT, Lafferty M, Devitt CM, Kuntz CA, et al. Improved survival associated with postoperative wound infection in dogs treated with limb-salvage surgery for osteosarcoma. Ann Surg Oncol. (2005) 12:1073–83. doi: 10.1245/ASO.2005.01.011
74. Culp WTN, Olea-Popelka F, Sefton J, Aldridge CF, Withrow SJ, Lafferty MH, et al. Evaluation of outcome and prognostic factors for dogs living greater than one year after diagnosis of osteosarcoma: 90 cases (1997–2008). J Am Vet Med Assoc. (2014) 245:1141–6. doi: 10.2460/javma.245.10.1141
75. Hans EC, Pinard C, van Nimwegen SA, Kirpensteijn J, Singh A, MacEachern S, et al. Effect of surgical site infection on survival after limb amputation in the curative-intent treatment of canine appendicular osteosarcoma: a Veterinary Society of Surgical Oncology retrospective study. Vet Surg. (2018) 47:E88–96. doi: 10.1111/vsu.13105
76. Kawachi A, Yoshida H, Kitano S, Ino Y, Kato T, Hiraoka N. Tumor-associated CD204 + M2 macrophages are unfavorable prognostic indicators in uterine cervical adenocarcinoma. Cancer Sci. (2018) 109:863–70. doi: 10.1111/cas.13476
77. Hirayama S, Ishii G, Nagai K, Ono S, Kojima M, Yamauchi C, et al. Prognostic impact of CD204-positive macrophages in lung squamous cell carcinoma: possible contribution of Cd204-positive macrophages to the tumor-promoting microenvironment. J Thorac Oncol. (2012) 7:1790–7. doi: 10.1097/JTO.0b013e3182745968
78. Wilk SS, Zabielska-Koczywas KA. Molecular mechanisms of canine osteosarcoma metastasis. Int J Mol Sci. (2021) 22:3639. doi: 10.3390/ijms22073639
79. Loukopoulos P, Mungall BA, Straw RC, Thornton JR, Robinson WF. Matrix metalloproteinase-2 and−9 involvement in canine tumors. Vet Pathol. (2003) 40:382–94. doi: 10.1354/vp.40-4-382
80. Lana SE, Ogilvie GK, Hansen RA, Powers BE, Dernell WS, Withrow SJ. Identification of matrix metalloproteinases in canine neoplastic tissue. Am J Vet Res. (2000) 61:111–4. doi: 10.2460/ajvr.2000.61.111
81. London CA. Tyrosine kinase inhibitors in veterinary medicine. Top Companion Anim Med. (2009) 24:106–12. doi: 10.1053/j.tcam.2009.02.002
82. London CA, Malpas PB, Wood-Follis SL, Boucher JF, Rusk AW, Rosenberg MP, et al. Multi-center, placebo-controlled, double-blind, randomized study of oral toceranib phosphate (SU11654), a receptor tyrosine kinase inhibitor, for the treatment of dogs with recurrent (either local or distant) mast cell tumor following surgical excision. Clin Cancer Res. (2009) 15:3856–65. doi: 10.1158/1078-0432.CCR-08-1860
83. Mason NJ, Gnanandarajah JS, Engiles JB, Gray F, Laughlin D, Gaurnier-Hausser A, et al. Immunotherapy with a HER2-targeting listeria induces HER2-specific immunity and demonstrates potential therapeutic effects in a phase i trial in canine osteosarcoma. Clin Cancer Res. (2016) 22:4380–90. doi: 10.1158/1078-0432.CCR-16-0088
84. Poulet G, Massias J, Taly V. Liquid biopsy: general concepts. Acta Cytol. (2019) 63:449–55. doi: 10.1159/000499337
85. Fan TM, Roberts RD, Lizardo MM. Understanding and modeling metastasis biology to improve therapeutic strategies for combating osteosarcoma progression. Front Oncol. (2020) 10:1–27. doi: 10.3389/fonc.2020.00013
86. Lacoste H, Fan TM, De Lorimier LP, Charney SC. Urine N-telopeptide excretion in dogs with appendicular osteosarcoma. J Vet Intern Med. (2006) 20:335–41. doi: 10.1892/0891-6640(2006)20[335:uneidw]2.0.co;2
87. Filipowicz W, Bhattacharyya SN, Sonenberg N. Mechanisms of post-transcriptional regulation by microRNAs: are the answers in sight? Nat Rev Genet. (2008) 9:102–14. doi: 10.1038/nrg2290
88. Gallo A, Tandon M, Alevizos I, Illei GG. The majority of microRNAs detectable in serum and saliva is concentrated in exosomes. PLoS ONE. (2012) 7:e30679. doi: 10.1371/journal.pone.0030679
89. Arroyo JD, Chevillet JR, Kroh EM, Ruf IK, Pritchard CC, Gibson DF, et al. Argonaute2 complexes carry a population of circulating microRNAs independent of vesicles in human plasma. Proc Natl Acad Sci. (2011) 108:5003–8. doi: 10.1073/pnas.1019055108
90. Turchinovich A, Weiz L, Langheinz A, Burwinkel B. Characterization of extracellular circulating microRNA. Nucleic Acids Res. (2011) 39:7223–33. doi: 10.1093/nar/gkr254
91. Sarver AL, Thayanithy V, Scott MC, Cleton-Jansen A-M, Hogendoorn PC, Modiano JF, et al. MicroRNAs at the human 14q32 locus have prognostic significance in osteosarcoma. Orphanet J Rare Dis. (2013) 8:7. doi: 10.1186/1750-1172-8-7
92. Pantel K, Speicher MR. The biology of circulating tumor cells. Oncogene. (2016) 35:1216–24. doi: 10.1038/onc.2015.192
93. Marconato L, Facchinetti A, Zanardello C, Rossi E, Vidotto R, Capello K, et al. Detection and prognostic relevance of circulating and disseminated tumour cell in dogs with metastatic mammary carcinoma: a pilot study. Cancers (Basel). (2019) 11:163. doi: 10.3390/cancers11020163
94. Hoshino A, Kim HS, Bojmar L, Gyan KE, Cioffi M, Hernandez J, et al. Extracellular vesicle and particle biomarkers define multiple human cancers. Cell. (2020) 182:1044–61.e18. doi: 10.1016/j.cell.2020.07.009
95. Johnsen KB, Gudbergsson JM, Andresen TL, Simonsen JB. What is the blood concentration of extracellular vesicles? Implications for the use of extracellular vesicles as blood-borne biomarkers of cancer. Biochim Biophys Acta Rev Cancer. (2019) 1871:109–16. doi: 10.1016/j.bbcan.2018.11.006
96. Boracchi P, Roccabianca P, Avallone G, Marano G. Kaplan-Meier curves, cox model, and P-values are not enough for the prognostic evaluation of tumor markers: statistical suggestions for a more comprehensive approach. Vet Pathol. (2021) 58:795–808. doi: 10.1177/03009858211014174
97. Zhao Z, Fan J, Hsu Y-MS, Lyon CJ, Ning B, Hu TY. Extracellular vesicles as cancer liquid biopsies: from discovery, validation, to clinical application. Lab Chip. (2019) 19:1114–40. doi: 10.1039/C8LC01123K
98. Ghosh A, Davey M, Chute IC, Griffiths SG, Lewis S. Rapid isolation of extracellular vesicles from cell culture and biological fluids using a synthetic peptide with specific affinity for heat shock proteins. PLoS ONE. (2014) 9:110443. doi: 10.1371/journal.pone.0110443
99. Roy JW, Taylor CA, Beauregard AP, Dhadi SR, Ayre DC, Fry S, et al. A multiparametric extraction method for Vn96-isolated plasma extracellular vesicles and cell-free DNA that enables multi-omic profiling. Sci Rep. (2021) 11:8085. doi: 10.1038/s41598-021-87526-y
100. Moreira ML, Dorneles EMS, Soares RP, Magalhães CP, Costa-Pereira C, Lage AP, et al. Cross-reactivity of commercially available anti-human monoclonal antibodies with canine cytokines: establishment of a reliable panel to detect the functional profile of peripheral blood lymphocytes by intracytoplasmic staining. Acta Vet Scand. (2015) 57:51. doi: 10.1186/s13028-015-0142-y
Keywords: canine osteosarcoma, biomarker, liquid biopsy, personalized medicine, microRNA (miRNA), extracellular vesicles, tissue biopsies
Citation: Luu AK, Wood GA and Viloria-Petit AM (2021) Recent Advances in the Discovery of Biomarkers for Canine Osteosarcoma. Front. Vet. Sci. 8:734965. doi: 10.3389/fvets.2021.734965
Received: 01 July 2021; Accepted: 03 September 2021;
Published: 01 October 2021.
Edited by:
Kristina Meichner, University of Georgia, United StatesReviewed by:
Labrini V. Athanasiou, University of Thessaly, GreeceSara Connolly, University of Illinois at Urbana-Champaign, United States
Copyright © 2021 Luu, Wood and Viloria-Petit. This is an open-access article distributed under the terms of the Creative Commons Attribution License (CC BY). The use, distribution or reproduction in other forums is permitted, provided the original author(s) and the copyright owner(s) are credited and that the original publication in this journal is cited, in accordance with accepted academic practice. No use, distribution or reproduction is permitted which does not comply with these terms.
*Correspondence: Alicia M. Viloria-Petit, aviloria@uoguelph.ca