Thiamine Alleviates High-Concentrate-Diet-Induced Oxidative Stress, Apoptosis, and Protects the Rumen Epithelial Barrier Function in Goats
- Laboratory of Metabolic Manipulation of Herbivorous Animal Nutrition, College of Animal Science and Technology, Yangzhou University, Yangzhou, China
High-concentrate diets are continually used in ruminants to meet the needs of milk yield, which can lead to the occurrence of subacute rumen acidosis in ruminants. This study investigated the protective effects of dietary thiamine supplementation on the damage of the ruminal epithelium barrier function in goats fed a high-concentrate diet. Twenty-four healthy Boer goats (live weight of 35.62 ± 2.4 kg; age, 1 year) were randomly assigned into three treatments, with eight goats in each treatment, consuming one of three diets: a low-concentrate diet (CON; concentrate/forage, 30:70), a high-concentrate diet (HC; concentrate/forage, 70:30), or a high-concentrate diet with 200 mg of thiamine/kg of dry matter intake (HCT; concentrate/forage, 70:30) for 12 weeks. The additional dose of thiamine was based on our previous study wherein thiamine ameliorates inflammation. Compared with HC treatment, the HCT treatment had markedly higher concentrations of glutathione, superoxide dismutase, and glutathione peroxidase and total antioxidant capacity (P < 0.05) in plasma and rumen epithelium. The results showed that the apoptosis index was lower (P < 0.05) in the HCT treatment than in that of the HC treatment. Compared with the HC treatment, permeability and the electrophysiology parameter short circuit current for ruminal epithelial tissue were significantly decreased (P < 0.05) in the HCT treatment. The immunohistochemical results showed that the expression distribution of tight junctions including claudin-1, claudin-4, occludin, and zonula occludin-1 (ZO-1) was greater (P < 0.05) in the HCT treatments than in the HC treatment. The mRNA expression in the rumen epithelium of ZO-1, occludin, claudin-1, B-cell lymphoma/leukemia 2, nuclear factor erythroid-2 related factor 2 (Nrf2), superoxide dismutase 2 (SOD2), glutathione peroxidase 1, and the phase II metabolizing enzymes quinone oxidoreductase and heme oxygenase in the HCT group was significantly increased in comparison with the HC diet treatment (P < 0.05), whereas the mRNA expression of caspase 3, caspase 8, caspase 9, bcl-2 associated X protein, lipopolysaccharide binding protein, toll-like receptor 4, nuclear factor kappa-B (NFκB), tumor necrosis factor alpha, interleukin-1β, interleukin, and tumor necrosis factor receptor-associated factor 6 decreased significantly in the HCT treatment (P < 0.05). Compared with the HC treatment, the HCT diet significantly increased the protein expression of ZO-1, occludin, claudin-1, NQO1, HO-1, SOD2, serine/threonine kinase, p-Akt, Nrf2, and p-Nrf2; conversely, the expression of NFκB-related proteins p65 and pp65 was significantly decreased (P < 0.05). In addition, thiamine relieved the damage on the ruminal epithelium caused by the HC diet. The results show that dietary thiamine supplementation improves the rumen epithelial barrier function by regulating Nrf2–NFκB signaling pathways during high-concentrate-diet feeding.
Introduction
In the intensive ruminant production industry, high-concentrate diets are used to feed goats or dairy to meet the global meat and milk demand (1). Although the richness in fermentable carbohydrate diets can improve ruminant production efficiency in the short term, it can also cause subacute rumen acidosis (SARA), a common metabolic disorder, when administered for long periods (2). The feeding of excessive high-concentrate diets caused an increase in short-chain fatty acids and lactate and resulted in a high reduction in ruminal pH (3, 4). This decline in ruminal pH can enhance the lysis of gram-negative bacteria and release lipopolysaccharide (LPS) that translocate into the bloodstream, which results in damage of the ruminal epithelium (5). Several studies have demonstrated that low pH can affect the integrity and permeability of the rumen epithelium (6–8). Moreover, the negative changes in barrier function of the rumen epithelium facilitate the translocation of LPS, spreading it into the circulatory system and resulting in systemic inflammation (9). Oxidative stress is related to the pathogenesis of many pathological conditions, including sepsis, mastitis, enteritis, and pneumonia (10). Therefore, it is a novel thought to consider the relationship between inflammation and oxidative stress in ruminal epithelial barrier function protection.
The tight junctions (TJs) are composed of transmembrane proteins that play a crucial role in regulating the permeability of the epithelial barrier and mediate adhesive function (11, 12), including the transmembrane proteins claudins and the adaptor proteins ZO-1, zonula occludin-2 (ZO-2), and zonula occludin-3 (ZO-3) (13–15). Previous studies have determined that the variation of TJ protein expression and distribution is the dominant reason for the disruption of the epithelial TJ barrier (16, 17), whereas the impairment of TJs causes a “leaky” epithelial barrier and then invasion of susceptible LPS, resulting in inflammatory responses (18). Occludin and ZO-1 are relevant to various junctional functions (19). The change of expression for occludin and ZO-1 induces an increase in transepithelial electrical resistance (20), resulting in an increase in paracellular permeability (21).
The damage in the epithelial barrier is closely linked with the trigger of inflammation and oxidative stress. Previous studies have demonstrated that excess feeding of a high-concentrate diet intensifies the activation of NFκB via LPS/TLR4 signaling (4, 22). Meanwhile, the transcription factor NFκB can be activated by oxidative stress and causes cytotoxicity and recruitment of apoptotic machinery (23–25). The suppression of the antioxidant system was caused by the high-grain diet through the influx of LPS concentration in peripheral blood (26). The gene Nrf2 takes a significant role in regulating the activation of the defense system, which leads to the protection of cells against the adverse impacts of oxidative stress and suppresses apoptosis (27). Accumulation of LPS causes the lower expression of Nrf2, resulting in suppression of the antioxidant system (28). The coordination between Nrf2 and NFκB is indispensable to enhance body health (29).
Thiamine is also known as vitamin B1 and plays a crucial role in carbohydrate metabolism by participating in the pentose phosphate pathway and tricarboxylic acid cycle. Thiamine also increases antioxidant formation and NADPH levels (30). Some researchers showed that thiamine might act as a potent antioxidant by scavenging free radicals to apoptotic inhibition (31). Simultaneously, exogenous thiamine supplementation reduced inflammation in ruminal epithelium during high-concentrate feeding via the suppression of NFκB activation (4, 32). A careful review of literature revealed that there are no data on the protective effect of thiamine on the rumen epithelium oxidative damage induced by a high-concentrate diet. Therefore, this present study aimed to investigate whether thiamine feeding increases TJ expression in rumen epithelium by modulating the Nrf2–NF-κB pathways, thereby protecting the rumen epithelial barrier function.
Materials and Methods
All trial plans and methods have been authorized by the Animal Ethics Committee of Yangzhou University, China. The study was conducted under the Care and Use of Laboratory Animals guidelines (SXXY 2015-0054).
Animals, Diet, and Experimental Design
There were 24 healthy Boer goats with no clinical sign, averaging 35.62 ± 2.4 kg in body weight (BW), at the beginning of the trial. The goats were randomly assigned into three treatments, with eight goats in each treatment, consuming one of three diets: (1) low-concentrate diet (CON; concentrate/forage, 30:70), (2) high-concentrate diet (HC; concentrate/forage, 70:30), and (3) high-concentrate diet with 200 mg of thiamine/kg of dry matter intake (DMI) (HCT; concentrate/forage, 70:30) for 12 weeks. The dietary composition is presented in Table 1. The added dose of thiamine was based on our previous study wherein thiamine ameliorates inflammation (4).
The goats were housed in small individual pens. The goats were fed at 0700 h and 1,800 h, at one-half of the allowed amount daily at each feeding, and had free access to freshwater during the trial period. Thiamine was mixed with the concentrate to be licked by the goats. The feed ingestion and physical condition of the animals were observed daily. All goats were slaughtered at the end of the trial, at 3 h following the final feeding.
Sample Collection and Analysis
After the goats were slaughtered, a representative sample of ~50 ml rumen fluid was collected to calculate the pH value instantly with a pH meter (Sartorius, Goettingen, Germany). The ruminal fluid was filtered through a four-layer cheesecloth, centrifuged at 10,000 × g for 15 min at 4°C, and stored at −20°C for later analysis. Blood samples were collected from the jugular vein using 5-ml vacuum tubes with sodium heparin (Lab Anim Tech Develp Co., Ltd., Beijing, China). Plasma was obtained via centrifugation at 3,000 × g at 4°C for 15 min and then stored at −20°C until further analysis. Within 5 min of slaughter, representative ventral epithelial tissues were exteriorized from the rumen and washed three times in precooled phosphate-buffered saline. A part of the sample (~50 cm2) was used for the Ussing chamber measurement. Approximately 10 g epithelium tissue was dissected into smaller pieces and transferred into liquid nitrogen for protein and RNA analysis. For histological analysis, the whole ventral sac rumen tissues were also fixed in either 4% paraformaldehyde or 2.5% glutaraldehyde (Sigma, St. Louis, MO) for histomorphometric microscopic analysis.
Measurements of Physiological Parameters in Rumen Fluid Analysis
Free LPS in the preprocessed rumen fluid was measured (33) by a chromogenic end-point Tachypleus amebocyte lysate assay kit (Rongbai Biological Technology Co., Ltd., Shanghai, China) with a minimum detection limit value of 0.01 endotoxin units (EU)/ml. Briefly, the rumen fluid was centrifuged at 10,000 × g for 45 min, and then the collected rumen fluid was diluted 100,000-fold for measurement of LPS. The detailed determination methods refer to Dai's previous studies (34). The thiamine concentration in ruminal fluid was tested with a micro method ELISA kit (Xin Yu Biotech Co., Ltd. Shanghai, China) following the manufacturer's explanatory memorandum. For the determination of volatile fatty acid (VFA), the rumen fluid mixtures were centrifuged at 3,000 g for 15 min, and then 25% (w/v) metaphosphoric acid was added into the collected supernatants. The VFA concentration was measured by gas chromatography (GC-14B, Shimadzu, Kyoto, Japan; Qin, 1982).
The Concentration Analyses of Thiamine, LPS, LBP, Cytokine, and IgA in the Blood
Thiamine and LPS in plasma were determined using similar methods as described above for rumen fluid and a lower measurement limit of LPS as 0.1 endotoxin units/ml. The LBP concentrations were performed using an ELISA kit test (Chinese Horseshoe Crab Reagent Manufactory, Xiamen, China) with a detection range of 15.6–1,000 ng/ml. The determination of IgA in serum was via an immunoturbidimetry assay kit (Bethyl Lab., Inc., Montgomery, USA). The cytokine in plasma was determined using the following commercial kits according to the manufacturer's instructions: TNF-α (Bio Source/Med Probe, Camarillo, CA), IL-1β (MyBioSource Inc., San Diego, CA), IL6 (MyBioSource Inc., San Diego, CA), and IL10 (Bethyl Lab., Inc., Montgomery, USA). The absorbance of all samples was read at 450 nm using a microplate reader (BioTek Instruments, Winooski, VT). The concentrations of TNF-α, IL-1β, IL6, and IL-10 had a detection range of 15.6–500 ng/ml, 31.25–2,000 pg/ml, 15.6–500 ng/ml, and 5–1,000 pg/ml, respectively, and the variation coefficients for inter- and intra-assay were no more than 10%.
Oxidative Enzyme Activities in Rumen Epithelial Tissue Measurement
The oxidative enzyme activities in rumen epithelial tissue were measured using commercial kits (Jiehuigao Biological Technology Co., Ltd., Beijing, China) according to the manufacturer's test specification for the following indicators: malondialdehyde (MDA), GSH and oxidized glutathione, glutathione reductase, GSH-Px, SOD, and T-AOC. All estimated values were standardized to the concentration of the total protein. BCA Protein Assay Kit (Beyotime Biotechnology Institute, Shanghai, China) was used to assess the protein concentrations.
Oxidative Enzyme Activities in Plasma Measurement
The oxidative enzyme activities in plasma were determined using the same assay kit described above for rumen epithelial tissue according to the manufacturer's instructions. The absorbance of all samples was read at 550 nm using a microplate reader (BioTek Instruments, Winooski, VT). The coefficient of variation was <2%.
Ussing Chamber Measurements in Ruminal Epithelial Sample
According to Klevenhusen's methods (8), the Ussing chamber technique was used to measure electrophysiological properties and the permeability of rumen epithelium tissue. Briefly, an ~50-cm2 ruminal epithelium tissue in ventral sac was isolated, the ventral rumen epithelium tissues was cleaned by immersion in buffer solution firstly, and then the epithelium was peeled away from the muscle layer. The isolated epithelium tissues were picked to pieces of about 2 cm2 and mounted between the two halves of the incubation chambers. Both halves of the chambers were instantly filled with buffer solution (8) and were gassed with carbogen gas (95% O2 and 5% CO2). Buffer temperature was maintained stationary at 39°C during the measurement process. Fluorescein 5(6)-isothiocyanate (FITC) and horseradish peroxidase (HRP) (Sigma-Aldrich, Schnelldorf, Austria) as markers were used to evaluate permeability. FITC and HRP were added to the mucosal side of each chamber, and hourly samples were taken from the serosal side to measure the flux through the epithelium. The electrophysiological parameters for Isc, tissue conductance (Gt), and transmembrane potential difference (PD) were determined by the Ussing chamber system and Acquire and Analyze 2.3 software.
Histological and Microscopic Analysis
Exemplars of the rumen wall were fixed via 4% paraformaldehyde solution and then embedded in paraffin, sectioned, and dyed by hematoxylin and eosin (H&E) for histological observation. A scoring criterion was adopted for determining histological damage as described previously (35). Briefly, the damage score was translated into three levels from 0 to 3, including superficial epithelial injury, moderate including focal erosions, and severe including multifocal erosions. Microscopic analysis via scanning electron microscopy (SEM) was adapted from Liu et al. (36). Briefly, the washed rumen tissue was immediately fixed in 2.5% glutaraldehyde for 24 h, transferred to 1% osmium for 1 h, then dehydrated via ethanol solutions, and coated with gold after having been kept under critical-point drying. The sample went through a series of treatments, cut into ultrathin sections (70–90 nm), and observed using transmission electron microscopy (Hitachi H-7650, Hitachi Technologies, Tokyo, Japan).
TUNEL Analysis
Apoptotic epithelial cells in rumen epithelium tissue were analyzed using TUNEL staining kits (Abcam, Shanghai, China) according to manufacturer's test specification. In TUNEL staining, the nucleotides attached by TdT were tagged either directly with a fluorescent label or with a chemical label that can be indirectly linked to either a fluorescent label or an enzyme. TUNEL-positive nuclei were dyed as brown-stained nuclei, which stated the existence of DNA fragmentation as a result of apoptosis. The positive rate was determined in randomly selected fields adapted from Tao's method (37).
Immunohistochemistry of Ruminal Epithelium Tight Junction Protein Analysis
The expression and distribution of ruminal epithelium tight junction proteins were performed via immunohistochemistry. The following antibodies were used in the immunohistochemistry analysis: claudin-1 (ab211737; Abcam), claudin-4 (ab210796; Abcam), occludin (ab167161, Abcam), and ZO-1 (ab214228, Abcam). Specimens were embedded in paraffin after having been fixed via 4% paraformaldehyde solution, sectioned and incubated with antibodies, and dyed by hematein for light microscope observation. Image Pro Plus v.6.0 software was used to choose the same brown color as the consistent criterion for estimating all photos. Each photo was determined to acquire the cumulative optical density of each image.
Total RNA Extraction and Real-Time PCR Analysis
Total RNA was extracted from 100 mg of rumen epithelium tissue via Tissue Total RNA Isolation Kit V2 (Vazyme Biotech Co., Ltd. Nanjing, China) according to the manufacturer's instructions. RNA integrity was quantified on A260/A280 ratio by Nano Drop 2000 (Thermo Fisher Scientific, Inc., Waltham, MA, USA). Only those samples with ratio A260 to A280 between 1.8 and 2.1 were subsequently adopted for cDNA trials. Then, cDNA synthesis was performed using 500 ng of the total RNA template by HiScript III RT SuperMix for qPCR (+gDNA wiper) (Vazyme Biotech Co., Ltd). Target primers were designed using Primer Premier Software 5.0 and synthesized by Sangon Biotech (Shanghai, China). The primer details are presented in Table 2. Real-time PCR was performed using ChamQTM SYBR® qPCR Master Mix Kit (Vazyme, Nanjing, China) with 7500 Fast Real-Time PCR System (Applied Bio-systems, CA, USA) according to the manufacturer's instructions. All processes were measured in triplicate. The quantitative PCR results were analyzed using the 2−ΔΔCt method (38). GAPDH gene expression was constant and was the only internal control gene in all samples.
Western Blotting Analysis
The total protein was fetched from the ruminal epithelium in all samples by mercantile kit (Thermofisher Scientific, New York, USA) based on the manufacturer's protocol. After that, the concentration of protein was measured via a special protein assay kit (Beyotime Biotechnology, Jiangsu, China). In this study, information on primary antibodies is shown in the following: anti-ZO-1 (diluted at 1:1,000, Abcam), anti-occludin (diluted at 1:1,000, Abcam), anti-claudin-1 (diluted at 1:1,000, Abcam), anti-catalase (anti-CAT, diluted at 1:2,000, Protein Tech), anti-heme oxygenase-1 (anti-HO-1, diluted at 1:1,000, Abcam), anti-superoxide dismutase 2 (anti-SOD2, diluted at 1:5,000, Novus), anti-p65 (diluted at 1:300, Cell Signaling), anti-pp65 (diluted at 1:300, Cell Signaling), anti-Akt (diluted at 1:2,000, Santa Cruz Biotechnology), anti-pAkt (diluted at 1:2,000, Santa Cruz Biotechnology), anti-nuclear factor erythroid-2 related factor 2 (anti-Nrf2, diluted at 1:2,000, Protein Tech), anti-pNrf2 (diluted at 1:2,000, Protein Tech), and anti-β-actin (diluted at 1:1,500, Santa Cruz). Thereafter, the protein was incubated for 45 min via the secondary antibody horseradish peroxidase (HRP-conjugated goat anti-rabbit IgG, 1:1,000, Beyotime), and then signals were visualized using the enhanced chemiluminescence kit (Thermofisher Scientific, USA) followed by a Bio-Rad imaging detection system. The data were analyzed via Image J software. All gray values were quantified to β-actin and were expressed relative to the control (39). Each trial was repeated six times.
Statistical Analysis
Data were analyzed using SPSS 21.0 software (SPSS Inc., Chicago, IL). The sex effect was involved in the original statistical model, giving no significant result (P > 0.05). Thus, the sex effect was eliminated from the final model in which treatment was only the fixed effect. Statistical significance was assessed using one-way analysis of variance and Tukey's post hoc test between different treatments. Data were deemed statistically significant when P < 0.05.
Results
Ruminal pH, Metabolite Content, Cytokine, and IgA
As shown in Table 3, Boer goat had a markedly decreased ruminal pH value in the HC treatment compared with the HCT treatment (p < 0.05). The HC diet feeding induced the increase of free LPS and the decrease of thiamine in rumen fluid and plasma, while thiamine supplementation reversed the results, and the same trend as LPS has happened to LPB in plasma (p < 0.05). Furthermore, the contents of butyrate, propionate, and total VFA were significantly lower, whereas the acetate content was significantly higher in the HCT treatment than in the HC treatment (p < 0.05).
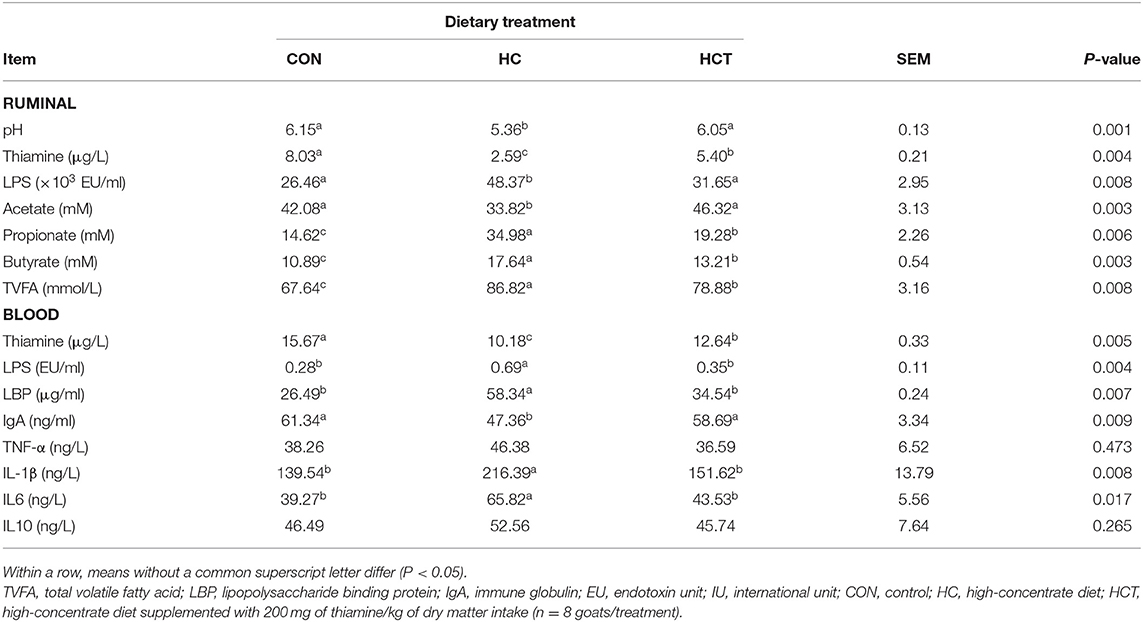
Table 3. Effects of thiamine supplementation on metabolites in the rumen and contents of thiamine, lipopolysaccharide, cytokine, and IgA in the blood of goats fed with a high-concentrate diet.
Goats fed with the HCT treatment had lower concentrations of cytokines TNF-α, IL-1β, IL6, and IL10 in blood than those in the HC treatment (p < 0.05). Moreover, the IgA concentration in the HCT treatment was significantly higher than in the HC treatment (p < 0.05).
Morphological and Ultrastructural Analysis of Rumen Papillae
Representative light micrographs of rumen papillae cross-sections are shown in Figure 1. The result showed that the rumen papillae of the CON and HCT treatments remained intact, whereas the stratum corneum of the epithelium papillae was injured seriously in the HC treatment. Meanwhile, the histological damage score was significantly higher in the HC treatment compared with the CON and HCT treatment (p < 0.05).
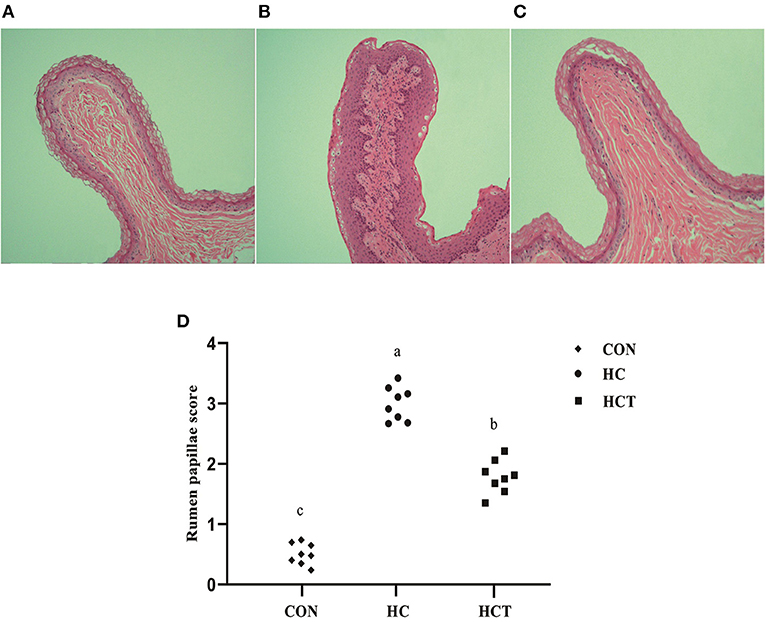
Figure 1. Representative histology sections of the rumen papillae of goats from the low-concentrate diet (CON), high-concentrate diet (HC), and high-concentrate diet with thiamine (HCT) treatment. Representative photomicrographs with H&E staining are shown. (A) The rumen papillae in the CON group were intact and showed no disruption. (B) In the HC treatment, the stratum corneum of the rumen papillae was severely damaged. (C) The rumen papillae showed a slight damage in the HCT treatment. (D) Rumen papillae injury score. All sections were stained with H&E and measured at ×400 magnification (n = 8 goats/treatment). The mean values in the figure without a common superscript letter differ (P < 0.05).
Scanning electron micrographs of rumen papillae demonstrated that the HC diet feeding caused desquamation, indentations, and dead keratinized cells throughout the surface of the papillae, where profound cellular injury also appeared. Nevertheless, the CON and the HCT treatments showed normal histological morphology (Figure 2).
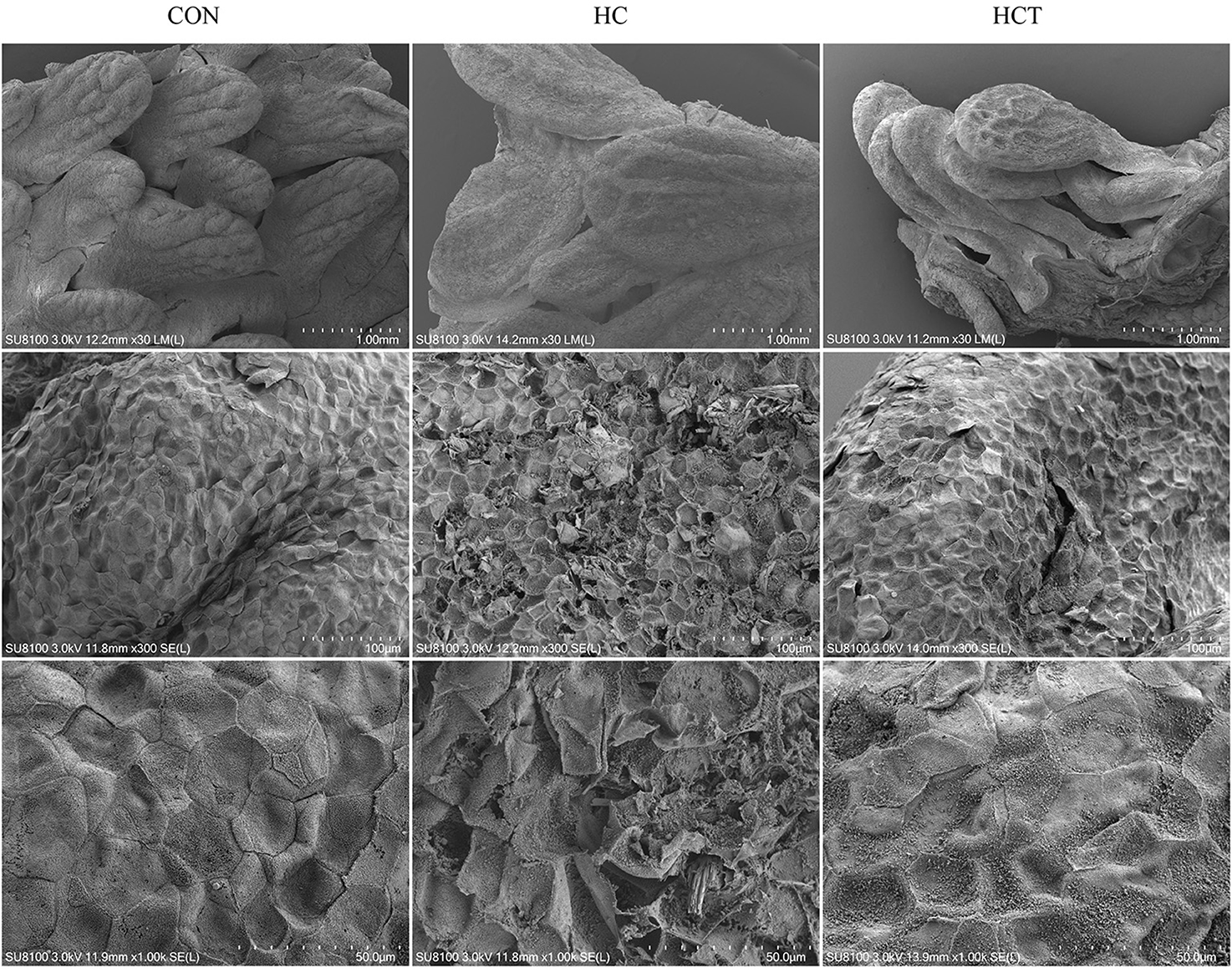
Figure 2. Comparisons of rumen papillae ultrastructure from the low-concentrate diet (CON), high-concentrate diet (HC), and high-concentrate diet with thiamine (HCT) treatments. Scanning electron microscopy (SEM) of the papillae surface in the three treatments with different scale bars (scale bar = 1.00 mm, 100 μm, and 50 μm, respectively).
Ussing Chamber Experiment
The HC diet treatment showed a significant up-regulation in Isc and Gt value and a significant down-regulation in PD values, whereas the HCT had a significant decrease in Isc and a significant increase in PD than the treatment only feeding a high-concentrate diet (p < 0.05; Figure 3). There remained no significant difference for Gt between the HC and HCT treatments. For the changing trend of permeability in ruminal epithelium, the HRP and FITC were found to be significantly lower in the HCT treatment than that in the HC treatment (p < 0.05), while their value was similar in the HCT and the CON treatments.
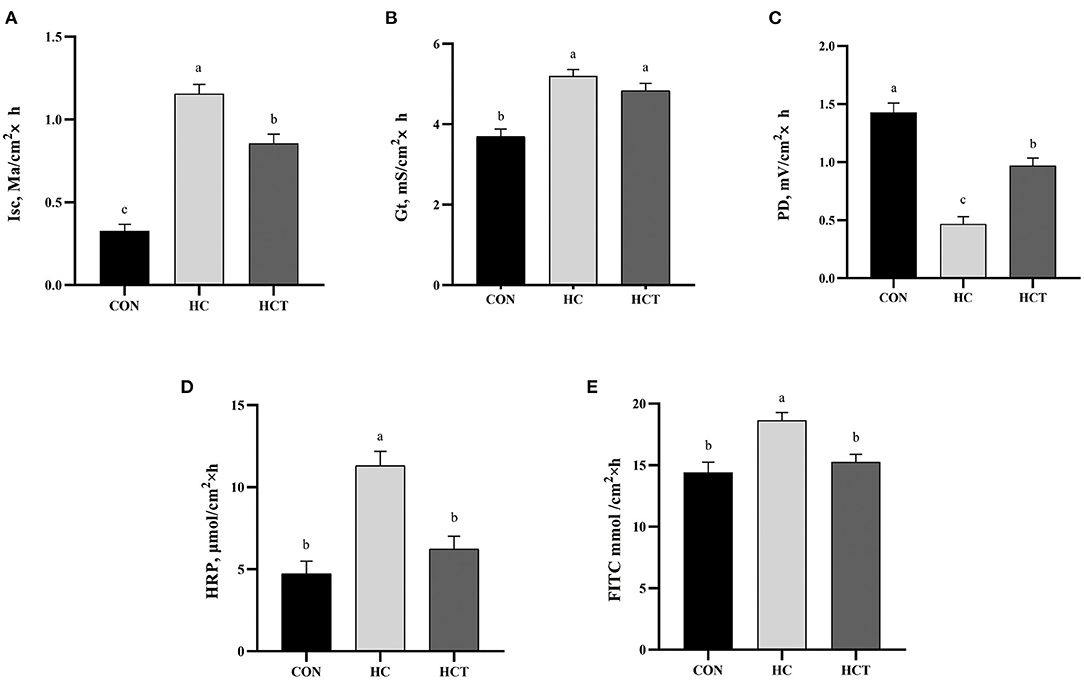
Figure 3. Effects of dietary thiamine supplementation on the electrophysiological properties: (A) short-circuit current (Isc), (B) tissue conductance (Gt), (C) transmembrane potential difference and tissue permeability measured as flux rate of (D) horseradish peroxidase and (E) fluorescein 5(6)-isothiocyanate. CON, low-concentrate diet; HC, high-concentrate diet; HCT, high-concentrate diet supplemented with 200 mg of thiamine/kg of dry matter intake (n = 8 goats/treatment). The mean values in columns without a common superscript letter differ (P < 0.05).
Apoptotic Index
As shown in Figure 4, the apoptotic percentage in the HC treatment was greater than that of the CON treatment (p < 0.05). In contrast, the apoptotic percentage was significantly down-regulated after feeding an HCT diet (p < 0.05).
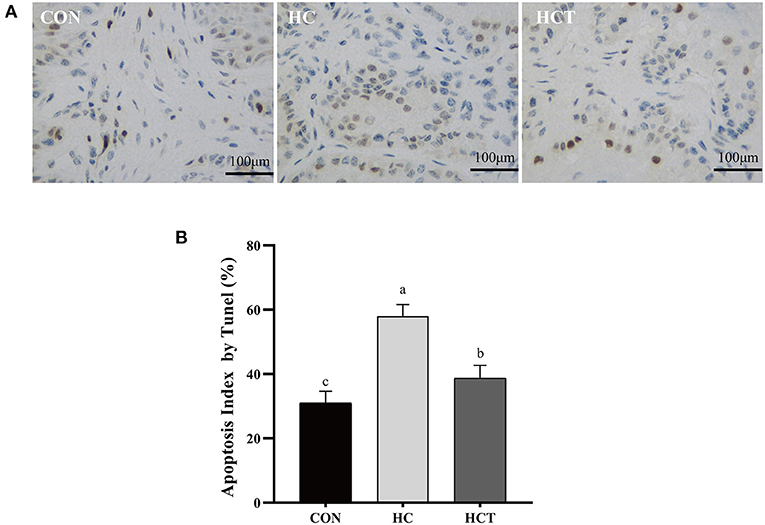
Figure 4. TUNEL comparison of the ruminal epithelium from the low-concentrate diet (CON), high-concentrate diet (HC), and high-concentrate diet with thiamine (HCT) treatments. Ruminal epithelium samples from each treatment were processed for evaluation of TUNEL-positive apoptotic cells. (A) Representative sections of rumen from the different treatments (CON, HC, and HCT treatments). (B) Apoptosis index analysis. All scale bars represent 25 μm (n = 8 goats/treatment). The mean values in columns without a common superscript letter differ (P < 0.05).
Oxidative Stress Status
The plasma MDA enzyme activity was significantly higher, whereas GSH, GSH-PX, SOD, and T-AOC enzyme activities were significantly lower in the HC treatment than in the CON treatment (p < 0.05; Table 4), while there was no significant variation between the HC and the HCT treatments (p < 0.05). These indicators remained at a trend nearly similar to that observed in the plasma.
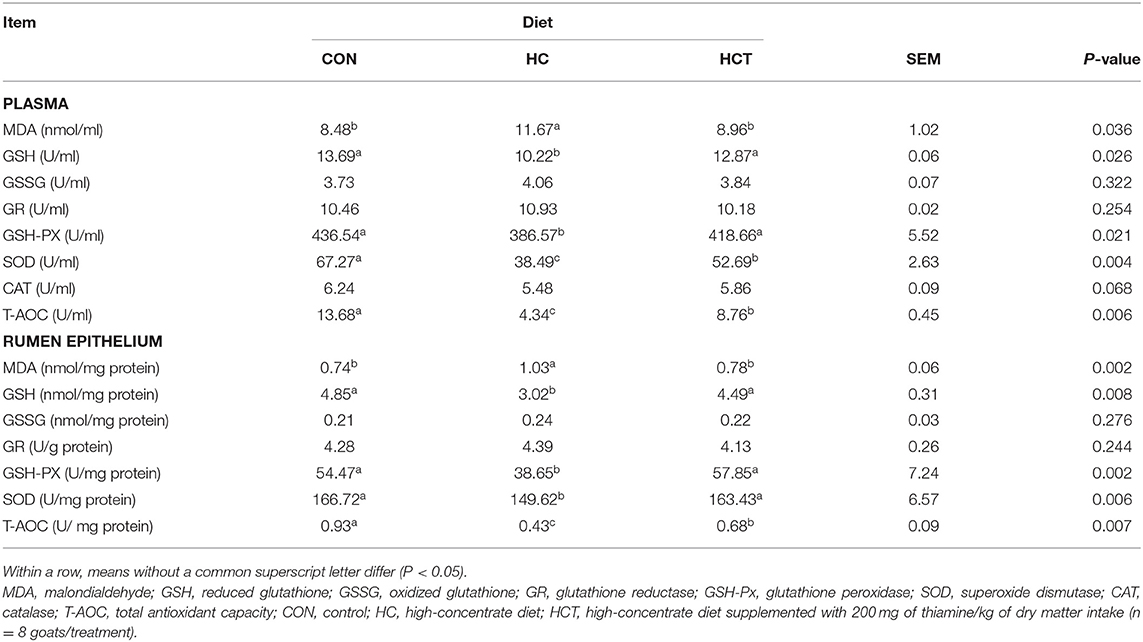
Table 4. Effects of thiamine supplementation on concentration of oxidative status index of plasma and rumen epithelium in goats with high concentrate diet.
Immunohistochemistry of Ruminal Epithelium Tight Junction
To investigate the differences in ruminal epithelium physical barrier with thiamine supplementation, we examined the expression and distribution of TJs (ZO-1, occludin, claudin-1, and claudin-4). The immunohistochemical analysis results showed that the protein expression levels of ZO-1, occludin, claudin-1, and claudin-4 were significantly lower in the HC diet compared with the CON treatment. The expression levels of ZO-1, occludin, claudin-1, and claudin-4 were also higher (p < 0.05; Figure 5) in the HCT diet than in the HC.
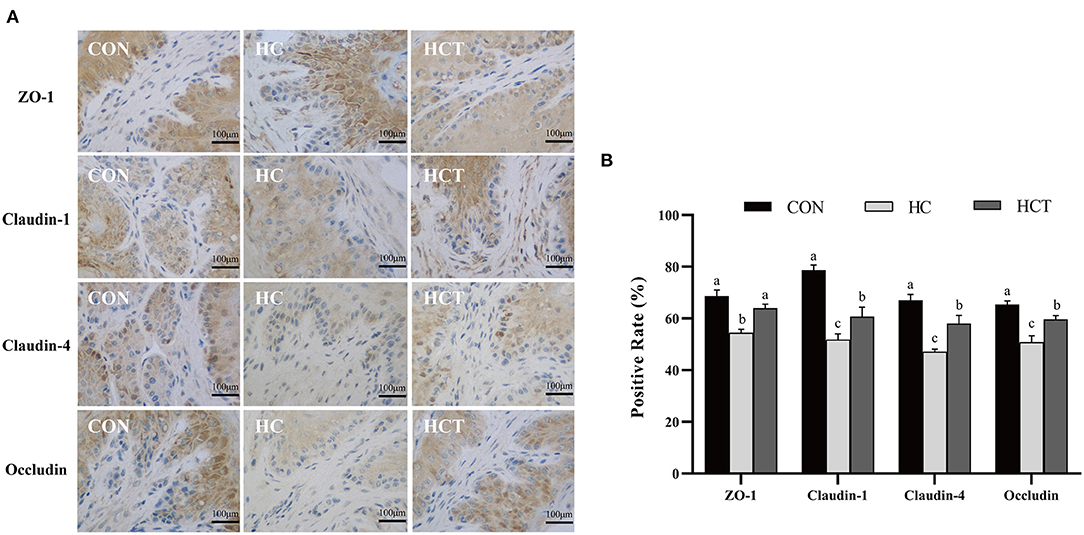
Figure 5. Effects of dietary thiamine supplementation on the expression and distribution of tight junction proteins during HC diet feeding. (A) Immunohistochemistry analysis of ZO-1, claudin-1, claudin-4, and occludin in the ruminal epithelium. (B) Positive rate analysis. All scale bars represent 100 μm (n = 8 goats/treatment). CON, low-concentrate diet; HC, high-concentrate diet; HCT, high-concentrate diet supplemented with 200 mg of thiamine/kg of dry matter intake. The mean values in columns without a common superscript letter differ (P < 0.05).
Gene Expression
The mRNA expression of ZO-1, occludin, claudin-1, Bcl-2, Nrf2, SOD2, GPX1, NQO1, and HO-1 in the HCT treatment significantly increased in comparison with the HC diet treatment (p < 0.05; Figure 6). Meanwhile, the mRNA expression of caspase 3, caspase 8, caspase 9, Bax, LBP, TLR-4, NFκB, TNF-α, IL-1β, IL-6, and TNF receptor-associated factor six had a significant decrease in the HCT treatment in comparison with the HC diet treatment (p < 0.05; Figure 6).
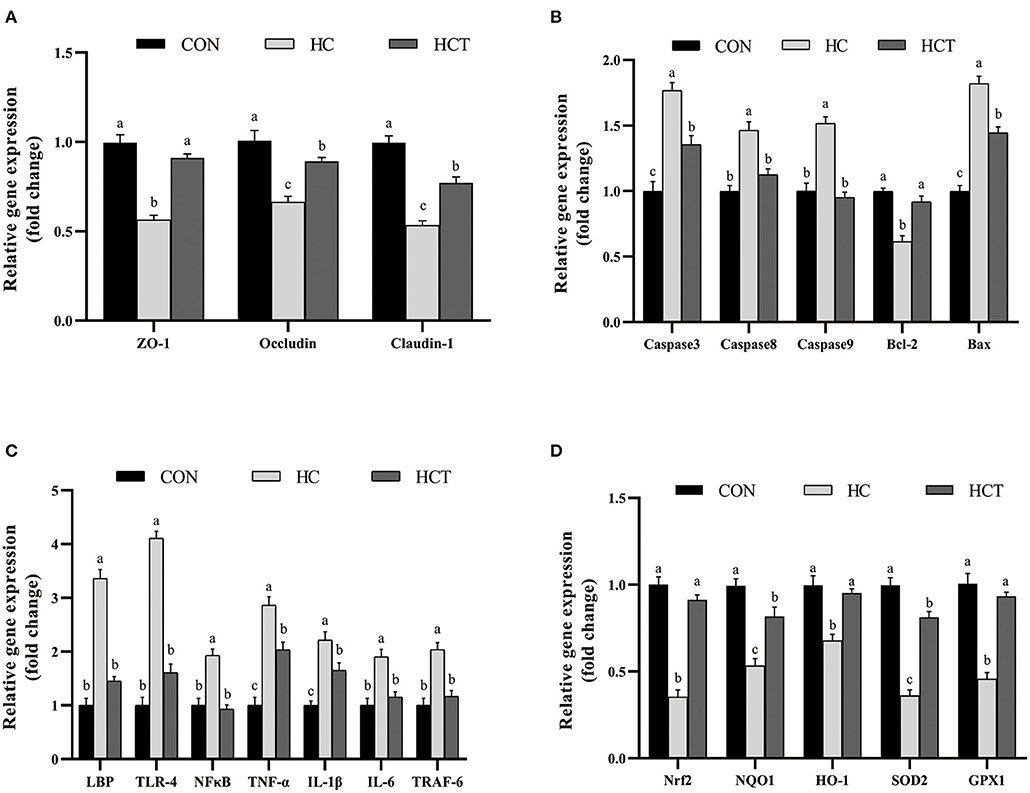
Figure 6. Effects of dietary thiamine supplementation on the mRNA abundance of genes during HC diet feeding. (A) Expression abundance of the tight junction-related gene. (B) Expression abundance of apoptosis-related genes. (C) Expression abundance of immune-related genes. (D) Expression abundance of oxidation-related genes (n = 8 goats/treatment). CON, low-concentrate diet; HC, high-concentrate diet; HCT, high-concentrate diet supplemented with 200 mg of thiamine/kg of dry matter intake. The mean values in columns without a common superscript letter differ (P < 0.05).
Protein Expression
Compared with the CON, the HC diet significantly reduced the protein expression of ZO-1, occludin, claudin-1, NQO1, HO-1, SOD2, Akt, p-Akt, Nrf2, and p-Nrf2; conversely, the expression of NFκB-related proteins p65 and pp65 was significantly increased (p < 0.05; Figures 7–9). Compared with the HC treatment, dietary thiamine supplementation reversed this change for those indicators (p < 0.05).
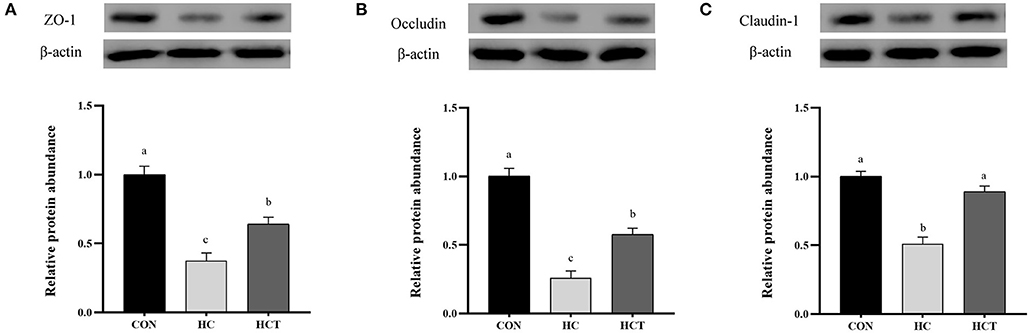
Figure 7. Effects of thiamine supplementation on the tight junction relative protein (A) ZO-1, (B) Occludin and (C) Claudin-1 expression levels in the ruminal epithelium of goats during the HC diet feeding (n = 8 goats/treatment). CON, low-concentrate diet; HC, high-concentrate diet; HCT, high-concentrate diet supplemented with 200 mg of thiamine/kg of dry matter intake. The mean values in columns without a common superscript letter differ (P < 0.05).
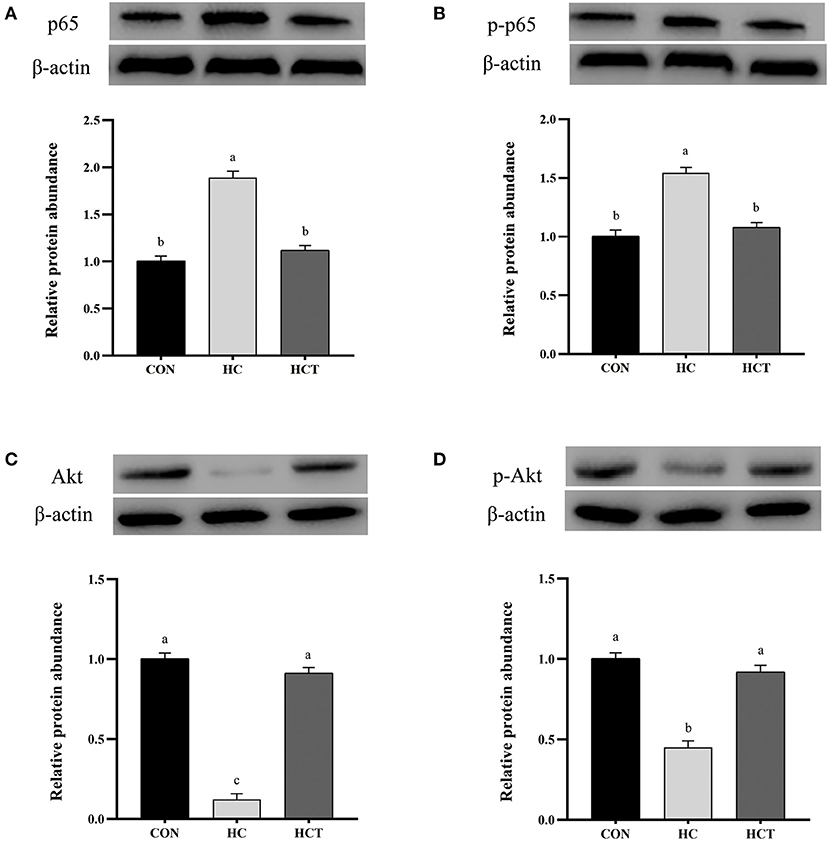
Figure 8. Effects of thiamine supplementation on the immune relative protein (A) p65, (B) p-p65, (C) Akt, and (D) p-Akt expression levels in the ruminal epithelium of goats during the HC diet feeding (n = 8 goats/treatment). CON, low-concentrate diet; HC, high-concentrate diet; HCT, high-concentrate diet supplemented with 200 mg of thiamine/kg of dry matter intake. The mean values in columns without a common superscript letter differ (P < 0.05).
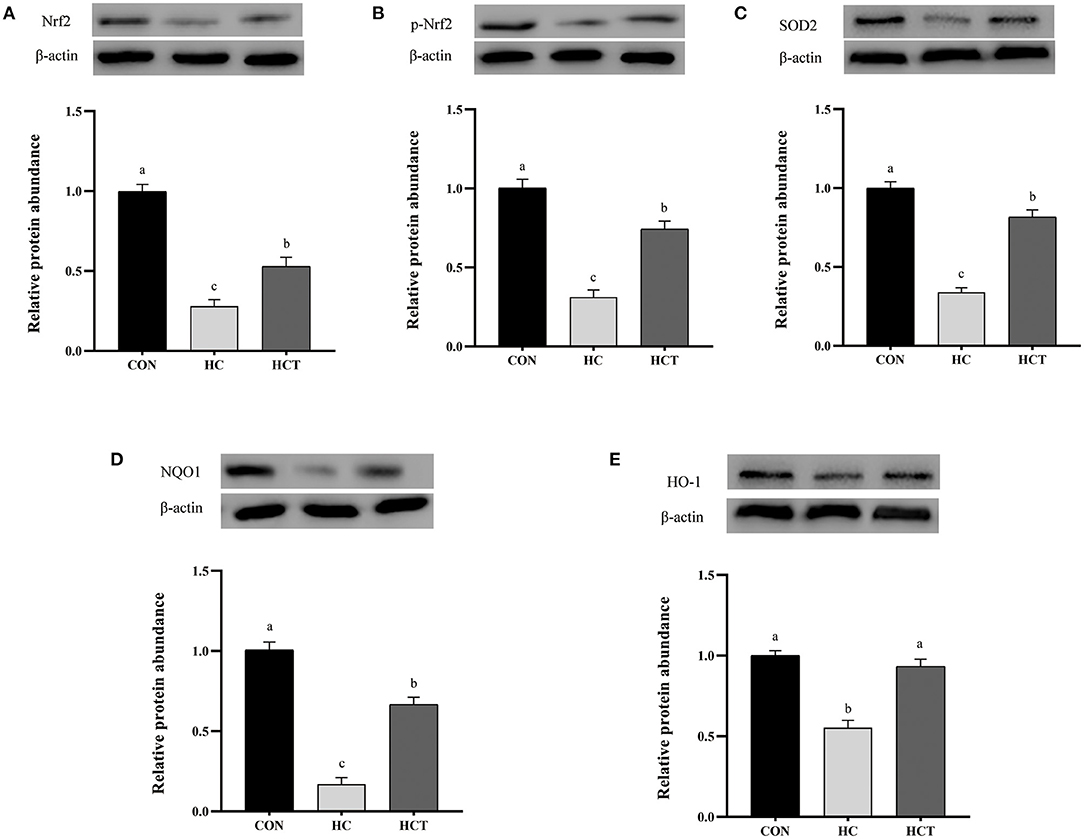
Figure 9. Effects of thiamine supplementation on the oxidative relative protein (A) Nrf2, (B) p-Nrf2, (C) SOD2, (D) NQO1 and (E) HO-1 expression levels in the ruminal epithelium of goats during the HC diet feeding (n = 8 goats/treatment). CON, low-concentrate diet; HC, high-concentrate diet; HCT, high-concentrate diet supplemented with 200 mg of thiamine/kg of dry matter intake. The mean values in columns without a common superscript letter differ (P < 0.05).
Discussion
Although the excessive amounts of high-concentrate diet in ruminants can enhance growth and milk yield for the short term, the accumulation of organic acids and plummeting of ruminal pH generate translocation of LPS, resulting in the damage of the rumen epithelium barrier (40, 41). In the current study, the HC diet feeding for 12 weeks exhibited a low pH and high VFA content compared to the CON treatment, which are characteristics of SARA. We found that the HCT diet induced a higher ruminal pH than that in the HC diet and reversed the negative change of VFA and LPS. The results indicated that thiamine supplementation changed the rumen fermentation state, which could be partially attributed to the evolution in microbial composition. Thiamine is indispensable for the proliferation of some ruminal bacteria strains of Ruminococcus, such as Ruminococcus albus and Ruminococcus flavefasciens (42). Meanwhile, relevant studies also uncovered that greater ruminal thiamine concentration could facilitate the increase in pH, which helped the growth of Succinivibrio (43). These results may provide more indirect clues for us to study the protective effect of thiamine on the epithelial barrier.
Correlative research has demonstrated that ruminal barrier function in ruminants has a molecular adaptation mechanism for HC diet feeding in the short term (44), and no impairment can be detected in cell layers during a short exposure (did not exceed 21 days) to the HC diet (6, 45). Thus, we fed the goats with the HC diet (70% grain) lasting for 12 weeks to monitor the structure of the rumen epithelium. The histological analysis indicated that the HC diet with thiamine supplementation for a long-term feeding regime protects the ruminal barrier. Furthermore, the thiamine effectiveness evidence in ruminal barrier function protection was demonstrated by SEM of the papillae surface.
Epithelial tight junction proteins are highly dynamic structures that contribute to the physical barrier function and play an important role in maintaining cell–cell interactions and stabilizing the paracellular and transcellular pathways (46). Occludin contributes to the maintenance of the TJ barrier as the large-channel TJ pathway, the pathway responsible for the macromolecule flux (47), with ZO-1 as an organizing component of the tight junction link occludin to the cortical actin cytoskeleton (11). Therefore, the coordination of occludin and ZO-1 plays a crucial role in maintaining the epithelium barrier. Claudins consist of multiple gene families, which have the ability to regulate cell proliferation (48). Our immunohistochemistry results demonstrated that the HCT diet defended the rumen epithelial physical barrier function compared to the HC diet as evidenced by the significantly altered expression and distribution of rumen epithelial tight junction proteins (ZO-1, occludin, claudin-1, and claudin-4). The expression of TJ gene and protein was also in accordance with the results of immunohistochemistry. Recent studies showed that the HC diet increased the ruminal epithelial permeability in goats by affecting the expression of tight junction proteins (36, 49). Thus, the Ussing chamber trial was chosen in this study for analysis of permeability and electrophysiology with thiamine supplementation.
Electrophysiology is associated with a balanced ruminal concentration of total VFA. Klevenhusen et al. indicated that a drop in pH causes an increase in the capacity of Isc (8). An excess of butyrate is toxic and can cause hyperkeratosis (50). Our study demonstrates that the HC diet increased Isc and Gt as well as decreased PD of the ruminal epithelium of goats and increased the permeability through the ruminal wall of the marker molecules FITC and HRP. Paracellular permeability can be estimated by the transepithelial transport of molecules, which usually are not present in the extracellular domain. Therefore, the value rise in Isc and PD, coupled with lower permeability to FITC and HRP, indicates an increased epithelial barrier function with thiamine supplementation. Thiamine supplementation changed the components of VFA as described above, which could be ascribed to the improvement in microbial community.
Apoptosis and damage of rumen epithelium are simultaneous during long-term high-concentrate diet feeding (51). Apoptosis plays a vital role in tissue homeostasis and development. Nevertheless, excessive apoptosis harms the barrier equilibrium of epithelium (52). Apoptosis may be triggered through the death receptor pathway, and the intrinsic apoptosis pathway depends on the mitochondria (53). In the present study, the HCT diet downregulated the proapoptotic genes such as Bax, caspase 3, caspase 8, and caspase 9 but upregulated the antiapoptotic gene Bcl2. The expression of Fas tends to decline, although the change was not statistically significant, which indicates a close relationship between apoptosis mediated by both the Fas and mitochondria. The change in the expression of caspase family members genes indicated a decrease in apoptosis rate with thiamine. The result of TUNEL analysis is also evidence that supports the change of gene expression. It has been reported that oxidative stress has great effects on mediating apoptosis (54). The ability of oxidative stress to trigger apoptosis as a result of a large number of cellular damages has been related to lipid peroxidation and alterations in proteins and nuclei (55). The fact has been revealed that thiamine deficiency caused an upregulation of apoptosis, inducing factor gene expression and leading to caspase 3-mediated apoptosis (56), while supplementation of thiamine ameliorated apoptosis by increasing the levels of GPX, SOD, and GSH (57). All these shreds of evidence point to the link between epithelial barrier function and oxidative stress with thiamine supplementation.
Our results have suggested that feeding a high-concentrate diet to goats for a long period results in the accumulation of LPS and VFA in the rumen. Previous studies have uncovered that suppression of the antioxidant system and activation of the immune system were caused by the high-concentrate diet through an accumulated LPS concentration in peripheral blood (4, 28), which is consistent with our findings for the expression of inflammatory and oxidative genes. We have previously reported that thiamine activates the immune system during high-concentrate feeding (4, 32). The increase of inflammatory factors in plasma indicates the occurrence of inflammation; regulation of IL-6 release by IgA may be among the anti-inflammatory mechanisms preventing the uncontrolled release of potentially noxious levels of inflammatory cytokines during acute or chronic responses (58). The NFkB pathway is known to regulate the expression of TJ proteins. It has been shown that decreases of TJ proteins produced chronic inflammation, in which NFkB activation plays a critical role (59).
Although oxidative reactions are crucial for animals, an excess of this reaction may cause tissue damage, while the occurrences of ROS exceeding the capacity of antioxidant defenses may cause oxidative stress (60). In the current study, we observed that MDA activity in the HCT treatment was significantly lower and that GSH, GSH-PX, SOD, and T-AOC were significantly higher than those in the HC treatment. These responses were similar for these indicators in epithelial tissue. Abaker et al. (61) demonstrated that a high-concentrate diet caused low levels of GPX and T-AOC and high levels of MDA in plasma. Watson et al. (26) proved the decreased SOD and GPX activities in male rats following endotoxin administration. Sokołowska et al. (62) also discovered that LPS induced oxidative stress and inflammatory reaction in the rat striatum. These studies give us an implication that LPS may induce oxidative stress through increasing of LPS in a high-concentrate feeding period. Previous research has shown that oxidative damage occurs along with oxidative stress caused by an increased level of MDA in serum (63). Multifarious antioxidant enzymes like SOD and GSH-PX play a vitally important role in protecting organisms from oxidative damage (64). The deficiency of thiamine may significantly impair the activity of transketolase, which is indispensable for the maintenance of GSH activity, a crucial antioxidant and free radical scavenger. Meanwhile, a previous study also showed that thiamine normalizes lipid peroxidation levels and elevates glutathione reductase activity (65).
Nrf2 acts as a sensor for oxidative stress, which regulates antioxidant defense systems (66). Nrf2 is restrained by Kelch-like ECH-associated protein 1 (keap1) in the cytoplasm under a situation of normal physiology; keap1 inhibits the function of Nrf2 by retaining Nrf2 in the cytoplasm under normal physiological situations. Nrf2 forms heterodimers with small Maf proteins and binds to the antioxidant response elements of target genes including GSH-Px, CAT, and SOD together with NQO1 and HO-1 (51) when cells are exposed in stress conditions (67). As a gene of anti-inflammatory and antioxidant properties, HO-1 is an Nrf2 target gene in the public eye (68). NQO1 can cope with oxidative lesions by weakening the activity of NADPH oxidase as well as the secretion of ROS, which is an Nrf2-mediated phase II metabolizing enzyme (69). The redox-regulated ubiquitous transcription factor NFκB can be activated by oxidative stress and inhibited by various antioxidants (23). The equilibrium state of both Nrf2 and NFκB regulation is vitally important to maintain the redox homeostasis in the health and performance of the body (29). Chen et al. revealed that Nrf2 deficiency impairs barrier function by disrupting the integrity of energy-dependent TJ (70), while the Nrf2 activator, quercetin, significantly enhanced intestinal barrier function through upregulation of claudin-4 in Caco-2 cells (71). Previous research has also shown that NFκB can damage barrier function through motivating the release of inflammatory factors (72).
The NFκB and Nrf2 pathways interface at several points to regulate transcription or the function of their downstream targets. On one hand, Nrf2 and NFκB can be functionally antagonistic. The down-regulated expression of Nrf2 induces more intense inflammation through activation of NFκB and down-stream proinflammatory cytokines (73). On the other hand, NFκB and Nrf2 both regulate a subset of target genes such as HO1 and IL-8. In the present study, thiamine increased the Nrf2 protein level and up-regulated the levels of antioxidant enzymes such as SOD2 together with phase II metabolizing enzymes (like NQO1 as well as HO-1), all of which were conducive to relieve oxidative lesions in the ruminal epithelium for high-concentrate diet feeding. At the same time, our research also showed the decline of NFκB-associated proteins p65 and pp65 as well as the increase of Akt and pAkt during thiamine supplement. Some studies also point out that NFκB-p65 and Nrf2 had synergy in regulating the antioxidative response in these cells (74). Moreover, the PI3K/AKT pathway is involved in the induction of Nrf2-driven gene expression (75). These studies all point to one piece of evidence that thiamine reduces oxidative stress by regulating the Nrf2–NFκB pathways during a high-concentrate feeding.
In conclusion, the current study results revealed that the rumen epithelial barrier function and the immune function were impaired, which are associated with the apoptotic and oxidative status that occurred during 12 weeks of feeding with the high-concentrate diet. Dietary thiamine supplementation was able to alleviate those harmful effects through the NFκB and Nrf2 signaling pathways. Thus, these findings provide that thiamine could potentially serve as a dietary supplementation to help in the alleviation of the negative effects of SARA in high-grain, intensive ruminant production.
Data Availability Statement
The raw data supporting the conclusions of this article will be made available by the authors, without undue reservation.
Ethics Statement
The animal study was reviewed and approved by Yangzhou city.
Author Contributions
YM and HW designed the research. YM and HZ conducted the research. YM and YZ analyzed the data. YM wrote the paper and had primary responsibility for the final content. ME revised the language of the current paper and carried out the experiment. All authors read and approved the final manuscript.
Funding
The research was supported by the Project of the National Natural Science Foundation of China (No. 31872988, Beijing), National Key Research and Development Program of China (2017YFD0500504, Beijing), and the Top Talents Award Plan of Yangzhou University (2016).
Conflict of Interest
The authors declare that the research was conducted in the absence of any commercial or financial relationships that could be construed as a potential conflict of interest.
Acknowledgments
We thank all the members of HW's laboratory who contributed to sample determination. Furthermore, we also thank Mawda Elmhadi for the language revision of this article that made this paper possible.
References
1. Kennelly JJ, Robinson B, Khorasani GR. Influence of carbohydrate source and buffer on rumen fermentation characteristics, milk yield, and milk composition in early-lactation holstein cows. J Dairy Sci. (1999) 82:2486–96. doi: 10.3168/jds.S0022-0302(99)75500-1
2. Mutsvangwa T, Wright TC. Sub-Acute Ruminal Acidosis (SARA) in Dairy Cows. Ontario: Ministry of Agriculture and Food (2003).
3. Zebeli Q, Metzler-Zebeli B. Interplay between rumen digestive disorders and diet-induced inflammation in dairy cattle. Res Vet Sci. (2012) 93:1099–108. doi: 10.1016/j.rvsc.2012.02.004
4. Zhang H, Peng AL, Zhao FF, Yu LH, Wang MZ, Osorio JS, et al. Thiamine ameliorates inflammation of the ruminal epithelium of saanen goats suffering from subacute ruminal acidosis. J Dairy Sci. (2020) 103:1931–43. doi: 10.3168/jds.2019-16944
5. Khafipour E, Krause DO, Plaizier JC. A grain-based subacute ruminal acidosis challenge causes translocation of lipopolysaccharide and triggers inflammation. J Dairy Sci. (2009) 92:1060–70. doi: 10.3168/jds.2008-1389
6. Steele MA, Croom J, Kahler M, AlZahal O, Hook SE, Plaizier K, et al. Bovine rumen epithelium undergoes rapid structural adaptations during grain-induced subacute ruminal acidosis. Am J Physiol-Reg I. (2011) 300:R1515–23. doi: 10.1152/ajpregu.00120.2010
7. Rackwitz R, Gbel G. Effects of dissolved carbon dioxide on the integrity of the rumen epithelium: an agent in the development of ruminal acidosis. J Anim Physiol An N. (2017) 102:12752. doi: 10.1111/jpn.12752
8. Klevenhusen F, Hollmann M, Podstatzky-Lichtenstein L, Krametter-Frotscher R, Aschenbach JR, Zebeli Q. Feeding barley grain-rich diets altered electrophysiological properties and permeability of the ruminal wall in a goat model. J Dairy Sci. (2013) 96:2293–302. doi: 10.3168/jds.2012-6187
9. Dong HS, Wang Y, Jia Y, Ni Y, Zhang S, Zhuang X, et al. Long-term effects of subacute ruminal acidosis (SARA) on milk quality and hepatic gene expression in lactating goats fed a high-concentrate diet. PLoS ONE. (2013) 8:e82850. doi: 10.1371/journal.pone.0082850
10. Lykkesfeldt J, Svendsen O. Oxidants and antioxidants in disease: oxidative stress in farm animals. Vet J. (2007) 173:502–511. doi: 10.1016/j.tvjl.2006.06.005
11. Fanning AS, Jameson BJ, Jesaitis LA, Anderson JM. The tight junction protein ZO-1 establishes a link between the transmembrane protein occludin and the actin cytoskeleton. J Biol Chem. (1998) 273:29745–53. doi: 10.1074/jbc.273.45.29745
12. Graham C, Simmons NL. Functional organization of the bovine rumen epithelium. Am J Physiol Regul Integr Comp Physiol. (2005) 288:R173–81. doi: 10.1152/ajpregu.00425.2004
13. Takano K, Kojima T, Sawada N, Himi T. Role of tight junctions in signal transduction: an update. Excli J. (2014) 13:1145–62. doi: 10.17877/DE290R-7042
14. Feldman GJ, Mullin JM, Ryan MP. Occludin: structure, function and regulation. Adv Drug Deliver Rev. (2005) 57:883–917. doi: 10.1016/j.addr.2005.01.009
15. Poritz LS, Harris LR, Kelly AA, Koltun WA. Increase in the tight junction protein claudin-1 in intestinal inflammation. Digest Dis Sci. (2011) 56:2802–2809. doi: 10.1007/s10620-011-1688-9
16. Heiskala M, Peterson PA, Yang Y. The roles of claudin superfamily proteins in paracellular transport. Traffic. (2001) 2:93–8. doi: 10.1034/j.1600-0854.2001.020203.x
17. John LJ, Fromm M, Schulzke JD. Epithelial barriers in intestinal inflammation. Antioxid Redox Sign. (2011) 15:1255–70. doi: 10.1089/ars.2011.3892
18. Turner JR. Intestinal mucosal barrier function in health and disease. Nat Rev Immunol. (2009) 9:799–809. doi: 10.1038/nri2653
19. Matter K, Balda MS. Occludin and the functions of tight junctions. Int Rev Cytol. (1998) 186:117–46. doi: 10.1016/S0074-7696(08)61052-9
20. Balda MS, Whitney JA, Flores C, Gonzalez S, Cereijido M, Matter K. Functional dissociation of paracellular permeability and transepithelial electrical resistance and disruption of the apical-basolateral intramembrane diffusion barrier by expression of a mutant tight junction membrane protein. J Cell Biol. (1996) 134:1031–49. doi: 10.1083/jcb.134.4.1031
21. Rao RK, Basuroy S, Rao VU, Karnaky KJ, Gupta A. Jr. Tyrosine phosphorylation and dissociation of occludin-ZO-1 and E-cadherin-beta-catenin complexes from the cytoskeleton by oxidative stress. Biochem J. (2002) 368:471–81. doi: 10.1042/bj20011804
22. Kurashima Y, Goto Y, Kiyono H. Mucosal innate immune cells regulate both gut homeostasis intestinal inflammation. Eur. J. Immunol. (2013) 43:3108–15. doi: 10.1002/eji.201343782
23. Brennan P, O'Neill LA. Inhibtion of nuclear factor KappaB by direct modification in whole cells-mechanism of action of nordihydroguaiaritic acid, curcumin and thiol modifiers. Biochem Pharmacol. (1998) 55:965–73. doi: 10.1016/S0006-2952(97)00535-2
24. Bonilla-Porras AR, Jimenez-Del-Rio M, Velez-Pardo M. Vitamin K3 and vitamin Calone or in combination induced apoptosis in leukemia cells by a similar oxidative stress signalling mechanism. Cancer Cell Int. (2011) 10:11–9. doi: 10.1186/1475-2867-11-19
25. Hunot S, Brugg B, Ricard D, Michel PP, Muriel MP, Ruberg M. Nuclear translocation of NF-kappaB is increased in dopaminergic neurons of patients with parkinson disease. Proc Natl Acad Sci USA. (1997) 94:7531–6. doi: 10.1073/pnas.94.14.7531
26. Watson AM, Warren G, Howard G, Shedlofsky SI, Blouin RA. Activities of conjugating and antioxidant enzymes following endotoxin exposure. J Biochem Mol Toxicol. (1999) 13:63–9. doi: 10.1002/(SICI)1099-0461(1999)13:2<
27. Manna K, Khan A, Das DK, Kesh SB, Das U, Ghosh S, et al. Protective effect of coconut water concentrate and its active component shikimic acid against hydroperoxide mediated oxidative stress through suppression of NF-κB and activation of Nrf2 pathway. J Ethnopharmacol. (2014) 155:132–46. doi: 10.1016/j.jep.2014.04.046
28. Memon MA, Wang Y, Xu T, Ma N, Zhang H, Roy AC, et al. Lipopolysaccharide induces oxidative stress by triggering MAPK and Nrf2 signalling pathways in mammary glands of dairy cows fed a high-concentrate diet. Microb Pathogenesis. (2019) 128:268–75. doi: 10.1016/j.micpath.2019.01.005
29. Jayasooriya RG, Lee KT, Lee HJ, Choi YH, Jeong JW, Kim GY. Anti-inflammatory effects of beta-hydroxyisovalerylshikonin in BV2 microglia are mediated through suppression of the PI3K/Akt/NF-kB pathway and activation of the Nrf2/HO-1 pathway. Food Chem Toxicol. (2014) 65:82–9. doi: 10.1016/j.fct.2013.12.011
30. Hamano Y, Okada S, Tanaka T. Effects of thiamine and clenbuterol on plasma metabolites and hepatic oxygen consumption in broiler chicks. Br Poult Sci. (1999) 40:127–30. doi: 10.1080/00071669987953
31. Vidhya A, Renjugopal V, Indira M. Impact of thiamine supplementation in the reversal of ethanol induced toxicity in rats. Indian J Physiol Pharmacol. (2013) 57:406–17.
32. Pan XH, Yang L, Beckers Y, Xue FG, Tang ZW, Jiang LS, et al. Thiamine supplementation facilitates thiamine transporter expression in the rumen epithelium and attenuates high-grain-induced inflammation in low-yielding dairy cows. J Dairy Sci. (2017) 100:5329–42. doi: 10.3168/jds.2016-11966
33. Gozho GN, Plaizier JC, Krause DO, Kennedy AD, Wittenberg KM. Subacute ruminal acidosis induces ruminal lipopolysaccharide endotoxin release and triggers an inflammatory response. J Dairy Sci. (2005) 88:1399–403. doi: 10.3168/jds.S0022-0302(05)72807-1
34. Dai HY, Liu X, Yan J, Aabdin ZU, Bilal MS, She X. Sodium butyrate ameliorates high-concentrate diet-induced inflammation in the rumen epithelium of dairy goats. J Agr Food Chem. (2017) 65:596–604. doi: 10.1021/acs.jafc.6b04447
35. Wu X, Vallance BA, Boyer L, Bergstrom KS, Walker J, Madsen K, et al. Saccharomyces boulardii ameliorates Citrobacter rodentium-induced colitis through actions on bacterial virulence factors. Am J Physiol Gastr L. (2008) 294:G295–306. doi: 10.1152/ajpgi.00173.2007
36. Liu JH, Xu TT, Liu YJ, Zhu WY, Mao SY. A high-grain diet causes massive disruption of ruminal epithelial tight junctions in goats. Am J Physiol-Reg I. (2013) 305:R232–41. doi: 10.1152/ajpregu.00068.2013
37. Tao SY, Jing T. Activation of cellular apoptosis in the caecal epithelium is associated with increased oxidative reactions in lactating goats after feeding a high-concentrate diet. Exp Physiol. (2015) 100:278–87. doi: 10.1113/expphysiol.2014.083352
38. Livak KJ, Schmittgen TD. Analysis of relative gene expression data using real-time quantitative PCR and the 2–ΔΔCT method. Methods. (2001) 25:402–8. doi: 10.1006/meth.2001.1262
39. Hnasko TS, Hnasko RM. The western Blot. Methods Mol Biol. (2015) 1318:87–96. doi: 10.1007/978-1-4939-2742-5_9
40. Gaebel G, Martens H, Bell M. The effect of low mucosal pH on sodium and chloride movement across the isolated rumen mucosa of sheep. Q J Exp Phys Transl Integr. (1989) 74:35–44. doi: 10.1113/expphysiol.1989.sp003237
41. Zebeli Q, Ametaj BN. Relationships between rumen lipopolysaccharide and mediators of inflammatory response with milk fat production and efficiency in dairy cows. J Dairy Sci. (2009) 92:3800–9. doi: 10.3168/jds.2009-2178
42. Bryant M, Robinson IM. Some nutritional requirements of the genus Ruminococcus. Appl Microbiol. (1961) 9:91–5. doi: 10.1128/AM.9.2.91-95.1961
43. Wetzels SU, Mann E, Metzler-Zebeli BU, Pourazad P, Qumar M, Klevenhusen F. Epimural indicator phylotypes of transiently-induced subacute ruminal acidosis in dairy cattle. Front. Microbiol. (2016) 7:274. doi: 10.3389/fmicb.2016.00274
44. Penner GB, Oba M, Gabel G, Aschenbach JR. A single mild episode of subacute ruminal acidosis does not affect ruminal barrier function in the short term. J Dairy Sci. (2010) 93:4838–45. doi: 10.3168/jds.2010-3406
45. Steele MA, AlZahal O, Hook SE, Croom J, McBride BW. Ruminal acidosis and the rapid onset of ruminal parakeratosis in a mature dairy cow: a case report. Acta Vet Scan. (2009) 51:39. doi: 10.1186/1751-0147-51-39
46. Steed E, Balda MS, Matter K. Dynamics and functions of tight junctions. Trends Cell Biol. (2010) 20:142–9. doi: 10.1016/j.tcb.2009.12.002
47. Al-Sadi R, Khatib K, Guo S, Ye D, Youssef M, Ma T. Occludin regulates macromolecule flux across the intestinal epithelial tight junction barrier. AM J Physiol-Gastr L. (2011) 300:G1054–64. doi: 10.1152/ajpgi.00055.2011
48. Tsukita S, Yamazaki Y, Katsuno T, Tamura A, Tsukita S. Tight junction-based epithelial microenvironment and cell proliferation. Oncogene. (2008) 27:6930–6938. doi: 10.1038/onc.2008.344
49. Lodemann U, Martens H. Effects of diet and osmotic pressure on Na+ transport and tissue conductance of sheep isolated rumen epithelium. Exp Physiol. (2006) 91:539–50. doi: 10.1113/expphysiol.2005.032078
50. Gäbel G, Aschenbach JR, Müller F. Transfer of energy substrates across the ruminal epithelium: implications and limitations. Anim Health Res Rev. (2002) 3:15–30. doi: 10.1079/AHRR200237
51. Dai H, Ma NN, Chang G, Aabdin ZU, Shen X. Long-term high-concentrate diet feeding induces apoptosis of rumen epithelial cells and inflammation of rumen epithelium in dairy cows. Anim Biotechnol. (2020) 3:1–8. doi: 10.1080/10495398.2020.1806073
52. Günther C, Neumann H, Neurath MF, Becker C. Apoptosis, necrosis and necroptosis: cell death regulation in the intestinal epithelium. Gut. (2013) 62:1062–71. doi: 10.1136/gutjnl-2011-301364
53. Su M, Yu T, Zhang H, Wu Y, Wang X, Li G. The antiapoptosis effect of glycyrrhizate on HepG2 cells induced by hydrogen peroxide. Oxid Med Cell Longevity. (2016) 2016:1–9. doi: 10.1155/2016/6849758
54. Buttke TM, Sandstrom PA. Oxidative stress as a mediator of apoptosis. Immunol Today. (1994) 15:7–10. doi: 10.1016/0167-5699(94)90018-3
55. Korsmeyer SJ, Yin XM, Oltvai ZN, Veisnovack DJ, Linette GP. Reactive oxygen species and the regulation of cell death by the Bcl-2 gene family. Biochim Biophys Acta. (1995) 1271:63–6. doi: 10.1016/0925-4439(95)00011-R
56. Nandi D, Patra RC, Swarup D. Effect of cysteine, methionine, ascorbic acid and thiamine on arsenic-induced oxidative stress and biochemical alterations in rats. Toxicology. (2005) 211:26–35. doi: 10.1016/j.tox.2005.02.013
57. Giguere JF, Butterworth RF. Activities of thiamine-dependent enzymes in two experimental models of thiamine deficiency encephalopathy. Neurochem Res. (1987) 12:305–310.
58. Wolf HM, Fischer MB, Puhringer H, Samstag A, Vogel E, Eibl MM. Human serum IgA downregulates the release of inflammatory cytokines (tumor necrosis factor-alpha, interleukin-6) in human monocytes. Blood. (1994) 83:1278–88. doi: 10.1182/blood.V83.5.1278.bloodjournal8351278
59. Saitou M, Furuse M, Sasaki H, Schulzke JD, Fromm M, Takano H, et al. Complex phenotype of mice lacking occludin, a component of tight junction strands. Mol Biol Cell. (2000) 11:4131–42. doi: 10.1091/mbc.11.12.4131
60. Zebeli Q, Dunn S, Ametaj B. Perturbations of plasma metabolites correlated with the rise of rumen endotoxin in dairy cows fed diets rich in easily degradable carbohydrates. J Dairy Sci. (2011) 94:2374–2382. doi: 10.3168/jds.2010-3860
61. Abaker J, Xu T, Jin D, Chang G, Zhang K, Shen X. Lipopolysaccharide derived from the digestive tract provokes oxidative stress in the liver of dairy cows fed a high-grain diet. J Dairy Sci. (2017) 100:666–78. doi: 10.3168/jds.2016-10871
62. Noworyta-Sokołowska K, Górska A, Gołembiowska K. LPS-induced oxidative stress and inflammatory reaction in the rat striatum. Pharmacol Rep. (2013) 65:863–9. doi: 10.1016/S1734-1140(13)71067-3
63. Yin BS, Li JJ, Hu XS, Cui YZ, Song T. Study on relationship between free radical oxidative damage and cow mastitis. Prog Vet Med. (2011) 32:52–3.
65. Tolstykh OI, Khmelevskii IV. The role of alpha-tocopherol and thiamine in the correction of lipid peroxidation in compensatory myocardial hypertrophy. Vopr Pitan. (1991) 3:38–42.
66. Das J, Ghosh J, Roy A, Sil PC. Mangiferin exerts hepatoprotective activity against D-galactosamine induced acute toxicity and oxidative/nitrosative stress via Nrf2-NFkappaB pathways. Toxicol Appl Pharm. (2012) 260:35–47. doi: 10.1016/j.taap.2012.01.015
67. Kensler TW, Wakabayashi N, Biswal S. Cell survival responses to environmental stresses via the Keap1-Nrf2-ARE pathway. Annu Rev Pharmacol Toxicol. (2007) 47:89–116. doi: 10.1146/annurev.pharmtox.46.120604.141046
68. Li H, Tang Z, Chu P, Song Y, Yang Y, Sun B, et al. Neuroprotective effect of Phosphocreatine on oxidative stress and mitochondrial dysfunction induced apoptosis in vitro and in vivo: Involvement of dual PI3K/Akt and Nrf2/HO-1 pathways. Free Radical Biol Med. (2018) 120:228–38. doi: 10.1016/j.freeradbiomed.2018.03.014
69. Mizunoe Y, Watanabe S, Sudo Y, Kobayashi M, Higami Y. Trehalose protects against oxidative stress by regulating the Keap1-Nrf2 and autophagy pathways. Redox Biol. (2018) 15:115–24. doi: 10.1016/j.redox.2017.09.007
70. Chen H, Fang Y, Li W, Orlando RC, Shaheen N, Chen XL. NFkB and Nrf2 in esophageal epithelial barrier function. Tissue Barriers. (2013) 1:e27463. doi: 10.4161/tisb.27463
71. Suzuki T, Hara H. Quercetin enhances intestinal barrier function through the assembly of zonula occludens-2, occludin, and claudin-1 and the expression of claudin-4 in Caco-2 cells. J Nutr. (2009) 139:965–74. doi: 10.3945/jn.108.100867
72. Fang Y, Chen H, Hu Y, Djukic Z, Tevebaugh W, Shaheen NJ, et al. Gastroesophageal reflux activates the NF-κB pathway and impairs esophageal barrier function in mice. Am J Physiol Gastrointest Liver Physiol. (2013) 305:G58–G65. doi: 10.1152/ajpgi.00438.2012
73. Pan H, Wang H, Wang X, Zhu L, Mao L. The absence of Nrf2 enhances NF-κB-dependent inflammation following scratch injury in mouse primary cultured astrocytes. Mediat Inflamm. (2012) 2012:1–9. doi: 10.1155/2012/217580
74. George LE, Lokhandwala MF, Asghar M. Novel role of NF-κB-p65 in antioxidant homeostasis in human kidney-2 cells. Am J Physiol Renal Physiol. (2012) 302:F1440–6. doi: 10.1152/ajprenal.00006.2012
Keywords: thiamine, goats, subacute rumen acidosis, apoptosis, oxidative stress, immune function, tight junction proteins
Citation: Ma Y, Zhang Y, Elmhadi M, Zhang H and Wang H (2021) Thiamine Alleviates High-Concentrate-Diet-Induced Oxidative Stress, Apoptosis, and Protects the Rumen Epithelial Barrier Function in Goats. Front. Vet. Sci. 8:663698. doi: 10.3389/fvets.2021.663698
Received: 03 February 2021; Accepted: 06 April 2021;
Published: 20 May 2021.
Edited by:
Pietro Celi, Adisseo, FranceReviewed by:
Shiyu Tao, Huazhong Agricultural University, ChinaSurinder Singh Chauhan, The University of Melbourne, Australia
Copyright © 2021 Ma, Zhang, Elmhadi, Zhang and Wang. This is an open-access article distributed under the terms of the Creative Commons Attribution License (CC BY). The use, distribution or reproduction in other forums is permitted, provided the original author(s) and the copyright owner(s) are credited and that the original publication in this journal is cited, in accordance with accepted academic practice. No use, distribution or reproduction is permitted which does not comply with these terms.
*Correspondence: Hongrong Wang, hrwang@yzu.edu.cn