- Department of General Psychiatry, Center for Psychosocial Medicine, University of Heidelberg, Heidelberg, Germany
Extending beyond the motor domain, the cerebellum is involved in various aspects of cognition and affect. Multidisciplinary evidence has demonstrated topographic organization of higher-order cognitive functions within the cerebellum. We here review recent neuroimaging research that indicates cerebellar contributions to major depressive disorder (MDD). At the structural level, increased volume of lobule IX has been demonstrated in MDD patients, independent of acute or remitted disease state. Successful treatment with electroconvulsive therapy has been associated with increased lobule VIIA volume in depressed patients. At the functional level, connectivity analyses have shown reduced cerebro-cerebellar coupling of lobules VI and VIIA/B with prefrontal, posterior parietal, and limbic regions in patients with MDD. As a limitation, most of this evidence is based on smaller patient samples with incomplete phenotypic and neuropsychological characterization and with heterogenous medication. Some studies did not apply cerebellum-optimized data analysis protocols. Taken together, MDD pathophysiology affects distinct subregions of the cerebellum that communicate with cortical networks subserving cognitive and self-referential processing. This mini-review synthesizes research evidence from cerebellar structural and functional neuroimaging in depression, and provides future perspectives for neuroimaging of cerebellar contributions to MDD.
Introduction
Lesions of the cerebellar posterior hemispheres and the cerebellar vermis frequently result in cognitive and/or affective symptoms, sometimes referred to as the “cerebellar cognitive affective syndrome” (1). It is characterized by deficits in executive function and linguistic processing, as well as by emotion dysregulation (emotional lability, impaired social cognition, apathy, or depressed mood) (2). Electrical stimulation experiments in animal models have additionally related cerebellar neural activity to anxious and impulsive behavior (3–5). Over the past decade, cerebellar contributions to non-motor functions have attracted increasing scientific interest. In particular, structural and functional neuroimaging has allowed for a more detailed understanding of the “cognitive/affective cerebellum” (6, 7). Against this background, the question has been raised whether abnormal cerebellar structure or function may contribute to major depressive disorder (MDD) (8).
In this mini-review, we briefly summarize the available structural and functional neuroimaging data in humans regarding the role of the cerebellum in depression. We identify current research limitations and discuss future perspectives for neuroimaging of cerebellar contributions to MDD. To describe cerebellar anatomy, we use a revised version of the Larsell nomenclature (9), as suggested by Schmahmann et al. (10).
Functional Neuroanatomy of the “Cognitive-Affective Cerebellum”
The cerebellum's histology is uniform throughout cortex, i.e., unlike the cerebral cortex, it has no discernable areal boundaries (11). The spatial organization of somatosensory, cognitive and affective representations within the cerebellum relies on polysynaptic connections that different subregions of the cerebellum form with functionally distinct regions of the cerebrum (12). MRI-Analyses of intrinsic functional connectivity (fcMRI) provide a means to discern theses cerebro-cerebellar circuits, thereby revealing the functional topography of the cerebellum. This evidence is corroborated by older electrophysiological and tract tracing experiments in animal models (13, 14). In healthy human subjects, several fcMRI studies of cerebro-cerebellar coupling are available (15–18). So far, the most comprehensive cerebellar mapping has been performed by Buckner et al. (17). Including 1,000 healthy subjects and employing different fcMRI approaches, the authors demonstrated that approximately half of the cerebellar cortex is associated with higher-level cognitive and affective functions (17). Non-motor functions are represented in the posterior lobe of the cerebellum, i.e., within cerebellar lobules VI–IX. Figure 1 shows an illustration of the unfolded cerebellar cortex. The intrinsic connectivity networks (ICN) (19) in which the non-motor cerebellum participates, include the “cognitive control network” (CCN) (20), the “salience network” (SN) (20), and the “default mode network” (DMN) (21). Additionally, a “cerebello-amygdaloid network” (CAN) has been suggested (18). Consistent evidence for cerebellar functional topography has emerged, especially within the cognitive domain. All available MRI studies of cerebellar connectivity in healthy subjects suggest that the anterior part of lobule VIIA, crus I and II, is related to the CCN (15–17, 22, 23). Two studies suggest that major connections to the DMN originate from the posterior part of crus I and II of lobule VIIA (15, 17); one study suggests that lobule IX is an essential cerebellar representation of the DMN (22). Cerebellar contributions to the SN seem to come from lobule VI (18, 23) and from lobules VIIB and VIII (23). Finally, lobules VI and VIII (18, 23), as well as the vermal portions of lobules VIIB, VIII, and IX (23) seem to have functional connections to the amygdala, potentially representing an independent ICN (18). In summary, there are functional subregions within, and sometimes extending beyond, each of the lobules VI–IX. Within one cerebellar lobule, segregated subregions are associated with distinct functional networks, differentially supporting cognitive or affective processing. An open research question is whether the hemispheric and the vermal portions of one lobule have the same (17) or separate (23) connectivity patterns.
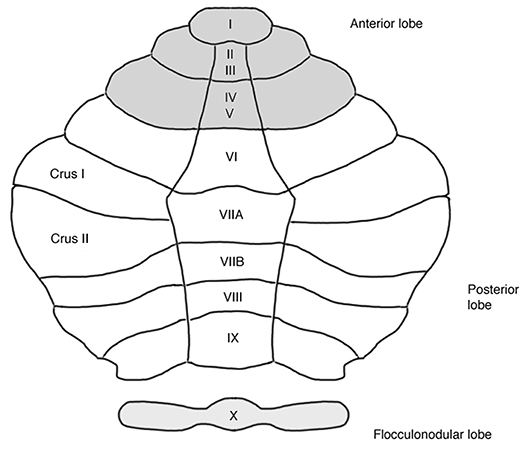
Figure 1. Unfolded view of the cerebellar cortex showing lobes and lobules (I–X). Anatomical labeling according to a revised version of the Larsell nomenclature (9), as described by Schmahmann et al. (10). Each cerebellar lobule comprises an unpaired medial (i.e., vermal) portion and a bilateral hemispheric portion. The posterior lobe represents the “cognitive/affective” cerebellum. Approximately half of the cerebellar cortex is associated with non-motor functions (17), see text for details.
Task-related cerebellar activations have been investigated in several MRI and PET studies. Verbal working memory, executive functions and emotional functions have been probed. These studies build on a cumulative number of ~100–150 subjects per task category. Stoodley and Schmahmann (6) conducted an “activation likelihood estimate” (ALE) meta-analysis of these findings. Consistent with the fcMRI studies, lobule VIIA, crus I was associated with cognitive functions (6). Further cognitive representations were detected in lobules VI and VIIB. The substrates of emotional processing were localized in lobule VI and in the vermal portion of lobule VII, in agreement with the functional connectivity results by Sang et al. (23) and Habas (18). Additional affective representations were localized in lobule VIIA, crus I (6).
In summary, the topographic organization of cognitive and affective representations in the cerebellum is complex. The cerebellar lobules within the “cognitive/affective cerebellum” consist of subregions with distinct connectivity patterns and functions. Across different imaging methodologies, there is most consistent evidence for the involvement of the anterior part of lobule VIIA, crus I and II, in cognitive processing (6, 15–18, 23). Clearly, there are also cerebellar contributions to the DMN, likely involving the posterior part of lobule VIIA, crus I and II (15, 17, 23), and potentially also lobule IX (15, 23). Furthermore, consistent evidence suggests that that lobule VI is associated with emotional processing (6, 18, 23). Finally, there is preliminary evidence for lobule VI involvement in the salience network (22, 23). Convergent findings are summarized in Table 1.
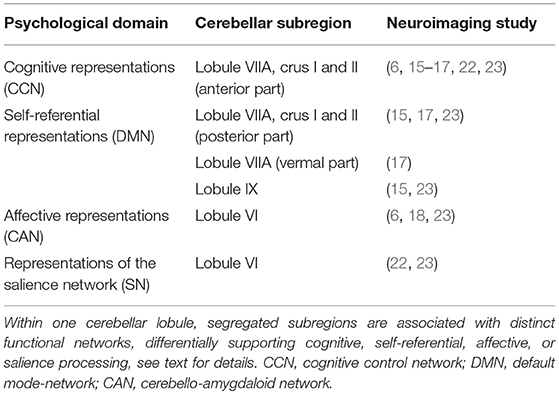
Table 1. Topographic organization of the non-motor cerebellum, as suggested by cerebellar neuroimaging.
Abnormal Cerebellar Structure and Function in Patients With Depression
Structural and functional changes of non-motor cerebellar regions in patients with depression have been subject to several MRI studies: Cerebellar connectivity has been investigated by six studies (24–29), cerebellar resting-state blood flow has been studied by one study (30), and cerebellum-optimized structural data analysis have been applied by two investigations (31, 32). Task-based imaging studies of the cerebellum in depressed patients are lacking at present.
Cognitive Representations
Consistent findings indicate that lobule VIIA, crus I and II, shows reduced connectivity to the cerebral components of the CCN in patients with depression, particularly to the dorsolateral prefrontal cortex (dlPFC) (24, 25, 27), but also to the ventromedial prefrontal cortex (vmPFC) (24, 28). In further support for the pathophysiological relevance of abnormal cerebellar-CCN coupling in depression, reduced lobule VIIA-vmPFC connectivity has been found to significantly correlate with impaired verbal working memory performance in depressed individuals (24). Finally, treatment with electroconvulsive therapy (ECT) seems to modulate the structure of an anterior subregion within lobule VIIA, crus I, in patients with severe acute depression (32). Notably, this structural change was associated with the antidepressant efficacy of ECT (32).
Self-Referential Representations (DMN)
Aberrant activity of the DMN's cerebellar components has been repeatedly demonstrated in patients with depression (24, 25, 27, 28), however, the specific spatial distribution and the direction of change is unclear at present. In depressed patients, Guo et al. (27) reported reduced connectivity of lobule VII, crus I, to the inferior parietal cortex and to the inferior temporal cortex, Liu et al. (25) reported reduced connectivity of lobule VIIA, crus II, to the posterior cingulate cortex (PCC), while Alalade et al. (24) reported increased connectivity of the vermal portion of lobule VIIA to the PCC. The latter finding was associated with the severity of depressive symptoms (24). Aberrant functional connectivity of the cerebellar vermis with key components of the DMN, i.e., with the PCC (25), as well as with the anterior cingulate cortex and the vmPFC (28) have also been described. However, it is important to note that these studies did not consider the functional segmentation of the vermis. Taking into consideration that vermal topography is highly complex (7, 17, 23), only limited conclusions can be drawn from these investigations. In patients with depression, more sophisticated approaches are required to specify aberrant cerebellar DMN activity. Finally, preliminary evidence has been presented for structural abnormalities of a cerebellar DMN component in depression. Independent of acute or remitted disease stage, lobule IX volume appears to be increased in patients with recurrent depression (31).
Representations of Other Domains
In depressed patients, no studies have yet been published on the structure or connectivity of cerebellar affective representations or of cerebellar representations of the salience network.
The Cerebellum in Depression-State of Knowledge And Future Perspectives
There is a special role for lobule VII within the “affective/cognitive cerebellum.” Unlike all other cerebellar lobules, lobule VII is not connected with the somatomotor system. Lobule VII communicates exclusively with cognitive and affective cerebral association cortices and with (para-) limbic structures (17). Cerebellar lobule VII contains subregions that are associated with cognitive, self-referential, and affective processing. In particular, strong evidence has accumulated for the involvement of lobule VII in cognitive processes. Convincing evidence has been provided for abnormal structure and function of lobule VII in patients with MDD. Multiple studies report decreased connectivity of lobule VII with cortical components of the CCN (24, 25, 27, 28). Preliminary findings also highlight the neuropsychological relevance of abnormal lobule VII-CCN coupling in patients with depression, i.e., an association between lobule VII-CCN connectivity and delayed memory recall (24). There are also cerebellar representations of the DMN and of the SN, as well as a putative cerebro-cerebellar ICN involving the amygdala (18), but their functional neuroanatomy is incompletely characterized at present.
There are two major limitations in current imaging approaches toward understanding the role of the cerebellum in depression. First, due to methodological constraints, the complex functional topography of the cerebellar vermis has been insufficiently addressed. At present, there is a considerable dearth of knowledge on dysfunction of the cerebellar vermis in depression. This is in contrast to the significance of the vermis for affective processes as illustrated by lesion studies (1). To accurately map the vermis' functional topography in depression, cerebellum-optimized data analysis protocols should be used by future MRI investigations. Cerebellum-optimized protocols have proven to result in superior neuroanatomical precision compared to conventional methods (33). Second, it has been a key observation of recent MRI studies that cerebellar lobules are polymodal structures with segregated, functionally distinct subregions. In fcMRI studies, it is of critical importance to consider these topographical details when placing the seed regions for connectivity analyses. The available fcMRI studies of cerebro-cerebellar connectivity in depression may not have been sufficiently accurate in this regard, potentially explaining the inconsistent cerebellar DMN findings in patients with MDD. Future studies should prefer data-driven extraction of cerebro-cerebellar ICNs. Validation of these findings by means of seed-based connectivity analyses may be performed, if accurate seed placement can be guaranteed based on previous data-driven identification of cerebellar ICN components (17).
Some general limitations of available brain imaging data in depression also apply to cerebellar imaging in MDD patients. First, depression is a clinically and biologically heterogeneous disorder, yet only few brain imaging studies have attempted to subtype patients based on clinical, neuropsychological or neurophysiological features (34). Second, in many studies, heterogeneity of psychotropic medication is a potential confound. Third, there is a lack of longitudinal brain imaging data in depression research. As a consequence, a significant number of fundamental research questions may not be answered. In particular, there is a need to discern the causal influence of depression risk factors on brain morphology and function. These limitations should be addressed when designing future studies to investigate cerebellar contributions to major depression.
This review emphasizes the role of non-motor cerebellar regions in patients with depression. Psychomotor disturbances, particularly psychomotor slowing, can be an important feature of major depression (35). The cerebral correlates of aberrant psychomotor functioning have been subject of several neuroimaging investigations (36–38). It should not be forgotten that future studies of the cerebellum in MDD will clearly benefit from investigating cerebro-cerebellar motor systems.
Author Contributions
MD and RW wrote the manuscript. MS and KK contributed to the interpretation of data.
Conflict of Interest Statement
The authors declare that the research was conducted in the absence of any commercial or financial relationships that could be construed as a potential conflict of interest.
Acknowledgments
We acknowledge financial support by Deutsche Forschungsgemeinschaft (funding programme Open Access Publishing), by the Baden-Württemberg Ministry of Science, Research and the Arts, and by Ruprecht-Karls-Universität Heidelberg. RW was supported by Deutsche Forschungsgemeinschaft, grant number WO1883/4-1.
References
1. Schmahmann JD, Weilburg JB, Sherman JC. The neuropsychiatry of the cerebellum - insights from the clinic. Cerebellum (2007) 6:254–67. doi: 10.1080/14734220701490995
2. Hoche F, Guell X, Vangel MG, Sherman JC, Schmahmann JD. The cerebellar cognitive affective/Schmahmann syndrome scale. Brain (2018) 141:248–70. doi: 10.1093/brain/awx317
3. Moers-Hornikx VM, Sesia T, Basar K, Lim LW, Hoogland G, Steinbusch HW, et al. Cerebellar nuclei are involved in impulsive behaviour. Behav Brain Res. (2009) 203:256–63. doi: 10.1016/j.bbr.2009.05.011
4. Moers-Hornikx VM, Vles JS, Lim LW, Ayyildiz M, Kaplan S, Gavilanes AW, et al. Periaqueductal grey stimulation induced panic-like behaviour is accompanied by deactivation of the deep cerebellar nuclei. Cerebellum (2011) 10:61–9. doi: 10.1007/s12311-010-0228-z
5. Huguet G, Kadar E, Temel Y, Lim LW. Electrical stimulation normalizes c-Fos expression in the deep cerebellar nuclei of depressive-like rats: implication of antidepressant activity. Cerebellum (2017) 16:398–410. doi: 10.1007/s12311-016-0812-y
6. Stoodley CJ, Schmahmann JD. Functional topography in the human cerebellum: a meta-analysis of neuroimaging studies. Neuroimage (2009) 44:489–501. doi: 10.1016/j.neuroimage.2008.08.039
7. Stoodley CJ, Schmahmann JD. Evidence for topographic organization in the cerebellum of motor control versus cognitive and affective processing. Cortex (2010) 46:831–44. doi: 10.1016/j.cortex.2009.11.008
8. Konarski JZ, Mcintyre RS, Grupp LA, Kennedy SH. Is the cerebellum relevant in the circuitry of neuropsychiatric disorders? J Psychiatry Neurosci. (2005) 30:178–86.
9. Larsell O. The Comparative Anatomy and Histology of the Cerebellum From Monotremes Through Apes. Minneapolis, MN: The University of Minnesota Press (1970).
10. Schmahmann JD, Doyon J, Mcdonald D, Holmes C, Lavoie K, Hurwitz AS, et al. Three-dimensional MRI atlas of the human cerebellum in proportional stereotaxic space. Neuroimage (1999) 10:233–60. doi: 10.1006/nimg.1999.0459
12. Strick PL, Dum RP, Fiez JA. Cerebellum and nonmotor function. Annu Rev Neurosci. (2009) 32:413–34. doi: 10.1146/annurev.neuro.31.060407.125606
13. Heath RG, Harper JW. Ascending projections of the cerebellar fastigial nucleus to the hippocampus, amygdala, and other temporal lobe sites: evoked potential and histological studies in monkeys and cats. Exp Neurol. (1974) 45:268–87. doi: 10.1016/0014-4886(74)90118-6
14. Kelly RM, Strick PL. Cerebellar loops with motor cortex and prefrontal cortex of a nonhuman primate. J Neurosci. (2003) 23:8432–44. doi: 10.1523/JNEUROSCI.23-23-08432.2003
15. Krienen FM, Buckner RL. Segregated fronto-cerebellar circuits revealed by intrinsic functional connectivity. Cereb Cortex (2009) 19:2485–97. doi: 10.1093/cercor/bhp135
16. O'reilly JX, Beckmann CF, Tomassini V, Ramnani N, Johansen-Berg H. Distinct and overlapping functional zones in the cerebellum defined by resting state functional connectivity. Cereb Cortex (2010) 20:953–65. doi: 10.1093/cercor/bhp157
17. Buckner RL, Krienen FM, Castellanos A, Diaz JC, Yeo BT. The organization of the human cerebellum estimated by intrinsic functional connectivity. J Neurophysiol. (2011) 106:2322–45. doi: 10.1152/jn.00339.2011
18. Habas C. Research note: a resting-state, cerebello-amygdaloid intrinsically connected network. Cereb Ataxias (2018) 5:4. doi: 10.1186/s40673-018-0083-0
19. Yeo BT, Krienen FM, Sepulcre J, Sabuncu MR, Lashkari D, Hollinshead M, et al. The organization of the human cerebral cortex estimated by intrinsic functional connectivity. J Neurophysiol. (2011) 106:1125–65. doi: 10.1152/jn.00338.2011
20. Seeley WW, Menon V, Schatzberg AF, Keller J, Glover GH, Kenna H, et al. Dissociable intrinsic connectivity networks for salience processing and executive control. J Neurosci. (2007) 27:2349–56. doi: 10.1523/JNEUROSCI.5587-06.2007
21. Greicius MD, Krasnow B, Reiss AL, Menon V. Functional connectivity in the resting brain: a network analysis of the default mode hypothesis. Proc Natl Acad Sci USA. (2003) 100:253–8. doi: 10.1073/pnas.0135058100
22. Habas C, Kamdar N, Nguyen D, Prater K, Beckmann CF, Menon V, et al. Distinct cerebellar contributions to intrinsic connectivity networks. J Neurosci. (2009) 29:8586–94. doi: 10.1523/JNEUROSCI.1868-09.2009
23. Sang L, Qin W, Liu Y, Han W, Zhang Y, Jiang T, et al. Resting-state functional connectivity of the vermal and hemispheric subregions of the cerebellum with both the cerebral cortical networks and subcortical structures. Neuroimage (2012) 61:1213–25. doi: 10.1016/j.neuroimage.2012.04.011
24. Alalade E, Denny K, Potter G, Steffens D, Wang L. Altered cerebellar-cerebral functional connectivity in geriatric depression. PLoS ONE (2011) 6:e20035. doi: 10.1371/journal.pone.0020035
25. Liu L, Zeng LL, Li Y, Ma Q, Li B, Shen H, et al. Altered cerebellar functional connectivity with intrinsic connectivity networks in adults with major depressive disorder. PLoS ONE (2012) 7:e39516. doi: 10.1371/journal.pone.0039516
26. Guo W, Liu F, Liu J, Yu L, Zhang Z, Zhang J, et al. Is there a cerebellar compensatory effort in first-episode, treatment-naive major depressive disorder at rest? Prog Neuropsychopharmacol Biol Psychiatry (2013) 46:13–8. doi: 10.1016/j.pnpbp.2013.06.009
27. Guo W, Liu F, Xue Z, Gao K, Liu Z, Xiao C, et al. Abnormal resting-state cerebellar-cerebral functional connectivity in treatment-resistant depression and treatment sensitive depression. Prog Neuropsychopharmacol Biol Psychiatry (2013) 44:51–7. doi: 10.1016/j.pnpbp.2013.01.010
28. Ma Q, Zeng LL, Shen H, Liu L, Hu D. Altered cerebellar-cerebral resting-state functional connectivity reliably identifies major depressive disorder. Brain Res. (2013) 1495:86–94. doi: 10.1016/j.brainres.2012.12.002
29. Guo W, Liu F, Liu J, Yu M, Zhang Z, Liu G, et al. Increased cerebellar-default-mode-network connectivity in drug-naive major depressive disorder at rest. Medicine (2015) 94:e560. doi: 10.1097/MD.0000000000000560
30. Depping MS, Wolf ND, Vasic N, Sosic-Vasic Z, Schmitgen MM, Sambataro F, et al. Aberrant resting-state cerebellar blood flow in major depression. J Affect Disord. (2018) 226:227–31. doi: 10.1016/j.jad.2017.09.028
31. Depping MS, Wolf ND, Vasic N, Sambataro F, Hirjak D, Thomann PA, et al. Abnormal cerebellar volume in acute and remitted major depression. Prog Neuropsychopharmacol Biol Psychiatry (2016) 71:97–102. doi: 10.1016/j.pnpbp.2016.06.005
32. Depping MS, Nolte HM, Hirjak D, Palm E, Hofer S, Stieltjes B, et al. Cerebellar volume change in response to electroconvulsive therapy in patients with major depression. Prog Neuropsychopharmacol Biol Psychiatry (2017) 73:31–5. doi: 10.1016/j.pnpbp.2016.09.007
33. Diedrichsen J. A spatially unbiased atlas template of the human cerebellum. Neuroimage (2006) 33:127–38. doi: 10.1016/j.neuroimage.2006.05.056
34. Drysdale AT, Grosenick L, Downar J, Dunlop K, Mansouri F, Meng Y, et al. Resting-state connectivity biomarkers define neurophysiological subtypes of depression. Nat Med. (2017) 23:28–38. doi: 10.1038/nm.4246
35. Buyukdura JS, Mcclintock SM, Croarkin PE. Psychomotor retardation in depression: biological underpinnings, measurement, and treatment. Prog Neuropsychopharmacol Biol Psychiatry (2011) 35:395–409. doi: 10.1016/j.pnpbp.2010.10.019
36. Bracht T, Federspiel A, Schnell S, Horn H, Hofle O, Wiest R, et al. Cortico-cortical white matter motor pathway microstructure is related to psychomotor retardation in major depressive disorder. PLoS ONE (2012) 7:e52238. doi: 10.1371/journal.pone.0052238
37. Liberg B, Rahm C. The functional anatomy of psychomotor disturbances in major depressive disorder. Front Psychiatry (2015) 6:34. doi: 10.3389/fpsyt.2015.00034
Keywords: major depression, cerebellum, cerebro-cerebellar networks, VBM, intrinsic connectivity
Citation: Depping MS, Schmitgen MM, Kubera KM and Wolf RC (2018) Cerebellar Contributions to Major Depression. Front. Psychiatry 9:634. doi: 10.3389/fpsyt.2018.00634
Received: 08 August 2018; Accepted: 08 November 2018;
Published: 29 November 2018.
Edited by:
Martin Walter, University of Tubingen, GermanyReviewed by:
Aislinn Joanmarie Williams, University of Iowa, United StatesSebastian Walther, Universität Bern, Switzerland
Copyright © 2018 Depping, Schmitgen, Kubera and Wolf. This is an open-access article distributed under the terms of the Creative Commons Attribution License (CC BY). The use, distribution or reproduction in other forums is permitted, provided the original author(s) and the copyright owner(s) are credited and that the original publication in this journal is cited, in accordance with accepted academic practice. No use, distribution or reproduction is permitted which does not comply with these terms.
*Correspondence: Robert C. Wolf, christian.wolf@med.uni-heidelberg.de