- 1School of Landscape and Ecological Engineering, Hebei University of Engineering, Handan, China
- 2Institute of Pomology, Shandong Academy of Agricultural Sciences, Tai’an, China
- 3School of Basic Medical Sciences, Hubei University of Chinese Medicine, Wuhan, China
- 4Key Laboratory of Plant Germplasm Enhancement and Specialty Agriculture, Wuhan Botanical Garden, Chinese Academy of Sciences, Wuhan, China
The plant hormone ABA (abscisic acid) plays an extremely important role in plant growth and adaptive stress, including but are not limited to seed germination, stomatal closure, pathogen infection, drought and cold stresses. Reactive oxygen species (ROS) are response molecules widely produced by plant cells under biotic and abiotic stress conditions. The production of apoplast ROS is induced and regulated by ABA, and participates in the ABA signaling pathway and its regulated plant immune system. In this review, we summarize ABA and ROS in apoplast ROS production, plant response to biotic and abiotic stresses, plant growth regulation, ABA signal transduction, and the regulatory relationship between ABA and other plant hormones. In addition, we also discuss the effects of protein post-translational modifications on ABA and ROS related factors.
Introduction
In nature, plants must adapt to their living environment to survive, which achieve the goal of individual growth and population reproduction, especially when plants are under stress conditions, including abiotic and biotic stresses. With the deepening of research, it has been found that ABA, as an important plant hormone. The main source of ABA is a de novo synthesis pathway that relies on carotenoids in higher plants (Nambara and Marion-Poll, 2005). ABA signal transduction begins with the ABA receptor perceiving the ABA signal, and then transmits the ABA signal downwards through a series of regulatory factors in the cell, forming a complex intracellular signal transduction network, and finally transformed into a visible physiological effect (Hauser et al., 2011). The regulatory network downstream of the ABA signal has protein phosphatase Type-2C Protein Phosphatase (PP2C) as the main node in the cell. In plants, PP2C is a serine/threonine protein phosphatase as a negative regulator in the ABA signaling pathway (Santiago et al., 2009; Umezawa et al., 2009). Studies have reported that PP2C functionally acquired mutants abi1-1 and abi2-1 are highly insensitive to ABA in seed germination and seedling growth (Gosti et al., 1999). In addition, the important regulatory factors downstream of ABA signal also include a large number of transcription factors that transmit and realize the gene functions regulated by ABA signal, such as ABI3, ABI4, ABI5, etc. these are all important transcription factors in ABA affecting seed germination and seedling morphology (Finkelstein et al., 2002).
In plants, ROS are stress-responsive substance, such as hydrogen peroxide (H2O2), superoxide anion (O2-), hydroxyl radical (OH-). In the process of aerobic metabolism, such as respiration and photosynthesis could inevitably lead to the production of various ROS in mitochondria, chloroplast and peroxisome, and a large amount of cellular ROS will also be caused in various biotic and abiotic stresses, and then leading to programmed cell death (PCD). A common feature of these different sources and types of ROS is that they cause oxidative damage to living substances such as protein, DNA, and lipid. Therefore, the balance of ROS in plants will also be strictly regulated. Plants have two ROS scavenging systems, namely the enzymatic scavenging system and the non-enzymatic scavenging system (Mittler, 2002; Mittler, 2017). The enzyme scavenging system is mainly through the reduction of enzymatic peroxides such as superoxide dismutase (SOD) and peroxidase (POD) to eliminate ROS, and the non-enzymatic scavenging system uses the ascorbate-glutathione cycle to eliminate ROS (Mittler, 2002). Interestingly, some studies found that there are many different mechanisms in plants that link ABA signaling with redox balance. Firstly, ABA induces the transcription of genes related to the ascorbate-glutathione cycle in the ROS scavenging system (Ghassemian et al., 2008). Secondly, the oxidation-decyclization from xanthine to zeaxanthin requires ascorbate oxidize to dehydroascorbate in ABA biosynthesis (Nambara and Marion-Poll, 2005; Hartung, 2010), which indirectly affects the level of ascorbate and the content of ROS (Ye et al., 2012), and also explains why ABA deficient mutants have lower ascorbate content and higher ROS enrichment (Ton et al., 2009).
ABA mediates the response of plants to a variety of environmental stresses, including abiotic stresses such as drought, salt, osmotic and cold stresses (Yoshida et al., 2014; Arif et al., 2018; Huang et al., 2018; Silva et al., 2018). Simultaneously, the degree of stress hormones response is also related to the adaptive capacity of plants to these adversity conditions (Nambara and Marion-Poll, 2005). Meanwhile, the role of ROS in plants which subjected to adversity stresses has been widely reported. Some studies believe that the accumulation of ROS is an adaptive response of plants under stress conditions (Suzuki et al., 2011), and it is not simply toxicity of metabolism. As a by-product, more and more evidences show that ROS also plays a role as a signaling molecule to regulate plant development and the expression of stress response genes, including encoding antioxidant enzyme genes, in order to regulate the production of H2O2 (Neill, 2002; Gechev et al., 2003; Choudhury et al., 2017; Mittler, 2017; Xue et al., 2020).
In addition, the function of ABA in plant-pathogen interactions has also been intensively studied (Ton et al., 2009; Cao et al., 2011). When facing multiple stresses, plants will initiate responses in the order of stresses or severity. In many cases though, ABA could promote the process of abiotic stress response to accelerate the initiation of defense responses to biotic stresses, such as pathogenic fungi (Robert-Seilaniantz et al., 2007; AbuQamar et al., 2017; Darma et al., 2019). In the early view, ROS would damage plant cell and accelerate PCD, as a signal molecule involved in the normal disease resistance process of plants, considered a universal early response substance to the infection of pathogenic microorganisms (Wong et al., 2008; Chi et al., 2009).
ABA not only promoted the shedding of plant organs, but also participates in the other plant growth and development processes, such as seed maturation, dormancy and germination, root growth and flower development (Leung and Giraudat, 1998; White et al., 2000; Finkelstein et al., 2002; Yoshida et al., 2006; Fujii et al., 2007). With the continuous progress of research, it has been found that under certain stress conditions, plants could generate a large amount of ROS to inhibit plant growth and development (Mittler, 2002; Agurla et al., 2018; Muhlemann et al., 2018), affect plant cell differentiation, root growth and stomata closure, etc. (Dietz et al., 2016; Simmons and Bergmann, 2016; Xu et al., 2017a).
At present, most researches concentrate on the role of ABA as a signal molecule in the response of plants to multiple environmental factors, and focus on the crosstalk between ABA and the response to biotic or abiotic stress in plants (Ma et al., 2009; Lee and Luan, 2012; Skubacz et al., 2016). ROS not only strengthens the cell wall through protein cross-linking to achieve antibacterial effect, but also could be used as a signal molecule to induce plant hypersensitivity (HY) and systemic acquired resistance (SAR) (Apel and Hirt, 2004; Xu et al., 2017b). In turn, plant pathogen associated molecular patterns, bacterial effector, signal transcription cascade, ion movement and protein kinase activation, etc., these biological processes will further promote the production of ROS. Meanwhile, another molecular mechanism has also been proposed to explain the inhibitory effect of ABA on ROS enrichment (Grant and Jones, 2009), that ABA may affect the stability of DELLA protein and induce the expression of genes which encoding ROS scavenging system related enzymes to inhibit the accumulation of ROS, among them, DELLA protein is one of the key regulators that integrate the plant hormones and environmental signals (Achard et al., 2008; Yang et al., 2012), indicating that there is a complex regulatory relationship between ABA and ROS.
The roles of ABA and ROS in plant resistances
In adversities, such as low temperature, high light intensity, osmotic stress, etc., the content of plant endogenous hormones will change significantly and affect the physiological state of plants. Research reported that plant endogenous hormones have undergone great changes under low temperature stress, such as the decrease of gibberellin content and the sharp increase of ABA content (Shu et al., 2018). In the research on the changes of plant hormones under low temperature stress, which had more attention to ABA, showing that ABA is closely related to the cold resistance of plants (Huang et al., 2017; Agurla et al., 2018; Lv et al., 2018). It is generally believed that the ABA content of plant varieties with strong cold resistance is higher than that of varieties with weak cold resistance, and the ABA content is positively correlated with the cold resistance of plants (Huang et al., 2017; Rubio et al., 2019). Meanwhile, the rate of calvin cycle metabolism decreases under low temperature stress, which will lead to excessive consumption of photoreaction and accumulation of ROS in photosynthesis (Ensminger et al., 2006). Studies had suggested that ABA could improve oxidase activity and induce stomatal closure to reduce CO2 fixation, thereby inhibit the accumulation of ROS (Lim et al., 2015). However, a recent study has shown that OPEN STOMATA 1 (OST1), as an important kinase in the ABA signaling pathway, can phosphorylate the photosynthetic oxygen-producing protein PPD5 of chloroplasts in the photosynthesis to reduce the accumulation of ROS, promote stomatal opening, and lead to weaken the photo-protective mechanism of plants, that reflects the negative regulation effect of ABA in regulating stomata opening and drought resistance (Hong et al., 2020). In plants, proline is considered to be a molecular substance with multiple functions, many studies have shown that the enrichment of proline can promote the response of plants to different abiotic stresses (Haudecoeur et al., 2009; Szabados and Savouré, 2010; Kavi Kishor and Sreenivasulu, 2014). As a substance related to osmotic regulation, proline has been recognized as a protective molecule that protects plant cells from osmotic stress, and proline has the ability to increase the activity of antioxidant enzymes, implying its positive regulation of ROS scavenging activity function (Matysik et al., 2002). In addition, with the increase of ROS levels during different metabolic changes caused by abiotic stresses (Foyer and Noctor, 2005), ABA signaling and ABA-dependent proline enrichment have been shown as an important part of the tolerance signal network while the plants resist different stresses (Pastori and Foyer, 2002). Under osmotic and salt stresses, the activities of proline biosynthesis related enzymes pyrroline-5-carboxylate synthetase (P5CS) and P5C reductase (P5CR) (Hu et al., 1992) are activated by ABA (Verslues et al., 2007; Szabados and Savouré, 2010). Research finding that the reduction of ROS enrichment is accompanied by the increase of proline content in the P5CS over-expression transgenic tobacco (Hong et al., 2000; Siripornadulsil et al., 2002). Correspondingly, the decrease of proline level promotes the ROS enrichment and leads to oxidative damage of plant cells in the p5cs1 insertion mutant (Szekely et al., 2008). Previous reports have also shown that salt stress-induced proline enrichment is dependent on the presence of ABA, which is consistent with the regulation of proline on redox, indicating that proline may act as an antioxidant in plants (Frank and James, 2006; Hoque et al., 2008). In addition, ABA also participates in ROS clearance process induced by salt stress in rice, however, ABA not only induces the expression of OsP5CR to generate proline through OsMADS25, but also induces the expression of OsGST4 to generate glutathione S–transferase (GST), thereby promotes ROS clearance and enhances the salt tolerance of rice, simultaneously, this study also shows that auxin may play a very important role in the ABA regulation of ROS clearance (Xu et al., 2018) (Figure 1).
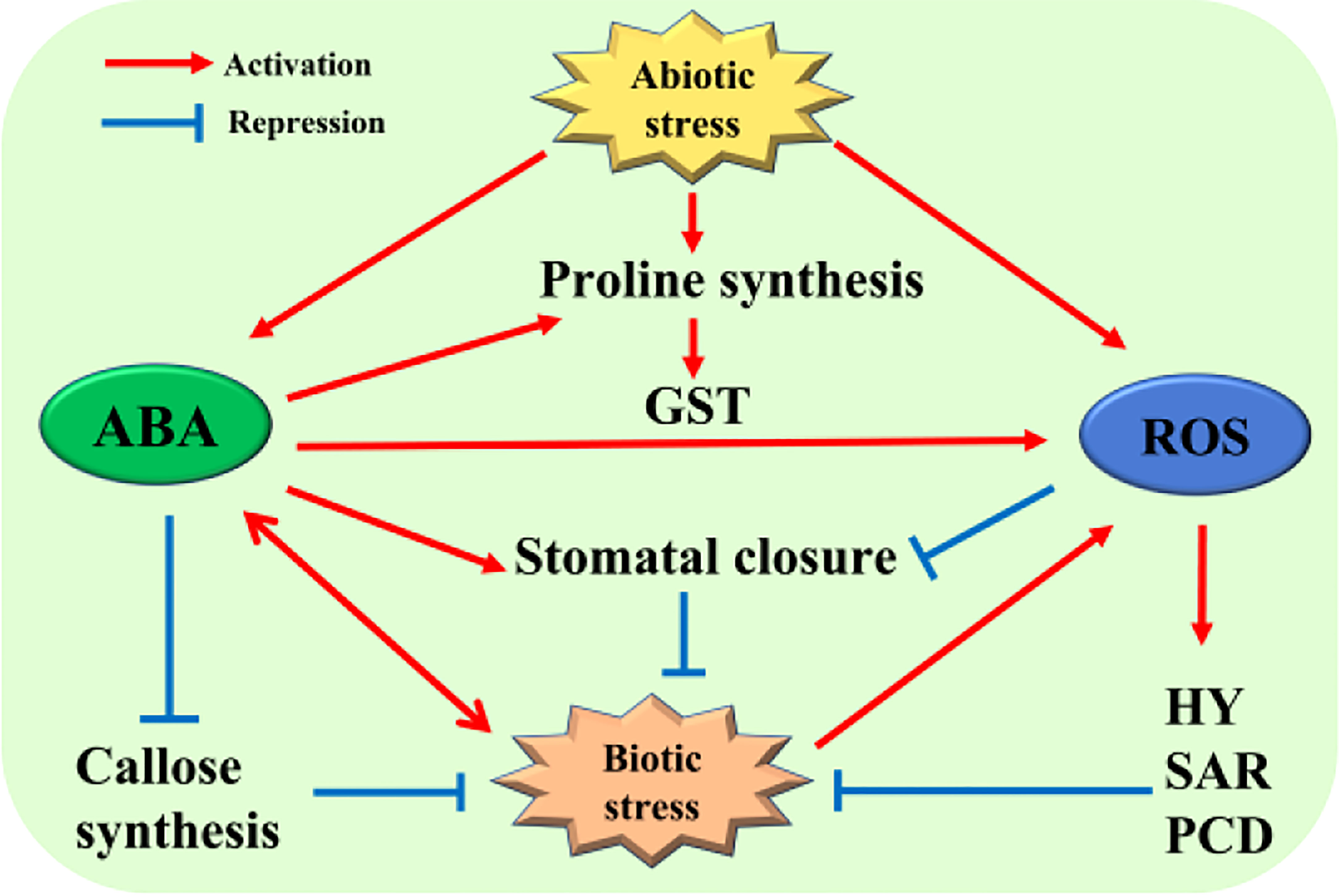
Figure 1 Regulatory network of the plant response to stress between ABA and ROS. Both biotic and abiotic stresses can cause the accumulation of ABA and ROS in plants, and ABA and ROS can also inhibit or adapt to external stress by regulating the content of some substances in plants or regulating the defense response of plants to maintain normal growth and development. For detailed explanation, please see text.
In the process of plant disease resistance and defense response, ABA exhibits dual characteristics in the process of pathogen infection and reproduction. For example, when plants are infected by the bacterial pathogen Pseudomonas syringae pv. tomato strain DC3000 (DC3000), ABA inhibits callose deposition and the expression of related genes induced by pathogen-associated molecular pattern (PAMP), that plays a negative regulatory role (de Torres Zabala et al., 2009). Meanwhile, when plant leaves are infected by bacterial pathogens, ABA will promote the closure of stomata to prevent the pathogens from spreading further in the plant (Melotto et al., 2006). In addition, ABA can promote the formation of callosum to block pathogenic bacteria continue to invade plants (Oide et al., 2013), that plays a positive regulatory role (Figure 1). The two-way regulation function is a hotspot on the interaction between plants and pathogens, and the kinetics of pathogen infection in the current research. When plants are infected by pathogens, that will induce the production of ROS and act as a signal molecule to activate plant hypersensitivity, which further starts systemic acquired resistance (SAR) (Apel and Hirt, 2004), or triggers off PCD to prevent further spread of the pathogens (Suzuki and Katano, 2018) (Figure 1). Interestingly, ABA can become the member of the infection strategy of pathogens in plants. For example, DC3000 can induce the enrichment of ABA and the expression of ABA signaling elements in Arabidopsis thaliana, this result is beneficial to the propagation of bacteria and the acceleration of the infection process (de Torres-Zabala et al., 2007). In addition, some pathogenic fungi, such as Botrytis cinerea and Magnaporthe grisea, directly promote the production of ABA to further accelerate the infection process and efficiency of pathogens (Audenaert et al., 2002; Cao et al., 2011).
The relationship between ABA and ROS in plant growth and development
In plants, the root development is attributed to the elongation and growth of root cells, as well as the continuous division and differentiation of cells (Ramirez‐Parra et al., 2017), which is affected by the homeostasis of ROS in plants (Tsukagoshi et al., 2010). As an important signal molecule, ROS affects many aspects of plant growth and development, such as cell cycle, PCD, hormone signaling, and plant response to environmental stress (Xie et al., 2014; You et al., 2014; Leng et al., 2017; Tognetti et al., 2017), if the balance of ROS is disturbed, the growth and development of plants will be affected (Zhang et al., 2014). As an important hormone that regulates plant metabolism, ABA also plays an important role in the growth and development of plant roots. In Arabidopsis, the ABA hypersensitive mutant abo6 accumulates a high concentration of ROS in the root tip, which slows down the growth rate of the root tip and reduced the activity of the root meristem (He et al., 2012; Yang et al., 2014). However, the exogenous application of GSH can partially restore the phenotype of root growth in abo6 mutant, it indicates that ROS generated in root tissues have a negative regulatory effect on root growth and development (He et al., 2012; Yang et al., 2014). Other reports also show the same regulatory relationship, exogenous application of GSH promotes the growth of Arabidopsis roots, while reducing the content of related antioxidants will inhibit the development of roots (Sanchez-Fernandez et al., 1997; Vernoux et al., 2000). Study has found that the domesticated mung bean seedling is detected the high content of ABA and the hypersensitivity to ABA, and showing a faster stomatal closure response, enhancing extracellular ROS production, and elevating antioxidant enzyme activity to adapt to environmental stresses, such as the damage caused by the burst of oxidation generated by osmotic stress, that is beneficial to the growth and development of mung bean seedlings (Sahu and Kar, 2018). Although the molecular interaction mechanism of ABA, ROS and antioxidant in the process of plant domestication is not clear, it still shows that there is a possible cross network which regulates plant stress tolerance and growing development.
Plant roots are often exposed to a variety of abiotic stresses, among them, high salt stress is the most common adversity condition, which strongly restricts the growth of plant roots and acts as a stress signal to promote the production of root tissue ROS. In plants, overmuch concentration of ROS will be toxic to plant cells. Therefore, the level of ROS must be strictly controlled (Qi et al., 2017). In order to reduce the oxidative damage of ROS to cells and maintain the intracellular redox balance, plants have evolved many defense systems, including ROS enzyme scavenging systems, such as ascorbate peroxidase (APX), superoxide dismutase (SOD), catalase (CAT), and glutathione peroxidase (GPX) (Foyer and Noctor, 2005). A large number of studies have shown that the ROS enzyme scavenging system is related to the tolerance and growth status of plants under abiotic stress (Dong et al., 2013; Huda et al., 2013; You et al., 2014). Constitutive overexpression of APX2 in the gain-of-function mutants can significantly enhance plant drought tolerance, improve plant water utilization efficiency, and maintain H2O2 homeostasis in plant cells, play a role in chloroplast protection and plant growing development (Rossel et al., 2006; Wu et al., 2018). Overexpression of APX also shows high tolerance to salt stress in transgenic plants (Lu et al., 2007; Yan et al., 2016). Similarly, constitutive overexpression of OsGSTU4 (glutathione S-transferase) in Arabidopsis can increase plant tolerance to salt and oxidative stresses, thereby promoting plant growth and development under adversity conditions (Sharma et al., 2014).
Under abiotic stresses, plants will cause premature senility of leaf through the changes of endogenous factors, which are important limiting factors for plant growth and crop yields. In the processes of these endogenous factors regulate leaf premature senility, ABA plays an important role in the connection between the oxidative damage of cell and the signal molecule response to abiotic stresses. ABA signaling mediates the expression of NYC, bZIP, WRKY and other transcription factors on transcription level, and indirectly affects the premature senility of leaf. In addition, Ca2+ signaling, ROS generation, and protein degradation also lead to leaf premature senility (Asad et al., 2019). Both exogenous environmental stimulating factors and endogenous senility factors can induce the activation of plant antioxidant systems and promote NADPH oxidase to catalyze the generation of ROS (Jiang and Zhang, 2002; Foreman et al., 2003; Ma et al., 2012). At the same time, ABA-mediated ROS production by NADPH oxidase acts as a second messenger in the ABA signal transduction pathway, negatively regulating the speed of ABA signal transmission, and then inducing leaf senescence (Kwak, 2003). Reports have shown that exogenous ABA can activate the NOX activity of genes encoding OsNox2, OsNox5, OsNox6, OsNox7, etc. in rice, and then promote the generation of ROS in guard cells. (Jiang and Zhang, 2002; Zhang et al., 2009). Thereinto, the expression of OsNox5 and OsNox7 are dependent on low and high concentrations of ABA respectively, indicating that OsNox5 and OsNox7 have a significant correlation with the level of ABA in plant tissues (Li et al., 2018).
In the process of plant photosynthesis, excessive light damage will lead to the degradation of the photosynthetic reaction center binding protein D1 by increasing the level of ROS and reducing the activity of PSII. In the light-dependent leaf senescence process, ABA can promote the degradation of D1 protein to trigger light damage (Gan and Amasino, 1997), however, partial shielding of light will cause the accumulation of low concentration ABA in local leaves and delay the senescence of leaves (Wang et al., 2016), which indicate that low horizontal ROS accumulation can cause lower photoinhibition and oxidative damage, and induce the degradation of D1 protein. On the contrary, low concentration ABA can not only inhibit the degradation of D1 protein, but also accelerate the biosynthesis of D1 protein. Study has found that ABA can induce the expression of the D1 protein-encoding gene PsbA during leaf senescence, thereby increase the content of D1 protein (Asad et al., 2019), indicating that low concentration ABA and ROS have opposite effects on the regulation of leaf senescence. In the darkness, the accumulation of D1 protein in rice leaves can reduce the level of ABA and further promote the photodamage repair of the PSII system.
Studies have reported that the transcription factor OsMADS25 regulates the elongation of main root and the number of lateral roots in rice through mediating the ABA signal pathway and the ROS scavenging system. Meanwhile, OsMADS25 can specifically activate the expression of OsP5CR, a key element of proline synthesis, in addition, OsMADS25 can activate the expression of OsYUC4 to promote auxin signaling, which in turn regulates root growth (Xu et al., 2018). In the process of plant root development, in addition to auxin and glucose, ROS also acts as a key signal molecule to regulate the activity of plant meristems. In particular, ROS is continuously generated in the root tip. The level of ROS controls the direction and scope of root tip growth (Liszkay et al., 2004). As a byproduct of plant cell metabolism, low level of ROS play a key role as a second messenger, regulating many important growth and development processes, including the division and differentiation of root tip meristem cells (Dunand et al., 2007; del Pozo, 2016). However, excessive ROS accumulation can cause oxidative damage to root tip cells. In mutants with altered ROS production or disrupted redox balance, the growth statuses of the taproots show significant differences (Dunand et al., 2007; Tsukagoshi et al., 2010; Yu et al., 2016; Zeng et al., 2017). The latest research showed that the increase of Ca2+ signal level in the cytoplasm caused by the external environment or growing development can indirectly inhibits the ABI4-mediated ABA signal pathway, thereby promoting the germination of Arabidopsis seeds (Kong et al., 2015). ROS also can promote the increase of Ca2+ content in the cytoplasm (Schroeder and Hagiwara, 1990; Pei et al., 2000; Kwak, 2003). Meanwhile, ABI4 can promote the generation of ROS through inhibiting the expression of VTC2 (Yu et al., 2019), and ABA-induced H2O2 production to activate Ca2+ channels in the plasma membrane (Pei et al., 2000). Therefore, whether ROS becomes an intermediate between Ca2+ signal and ABA signal through feedback effect in this regulatory network remains to be studied.
The roles of ABA and ROS in plant signaling
In plant cells, ABA is recognized and bound by ABA receptor RCAR/PYR1/PYL (Ma et al., 2009; Park et al., 2009), which interacts with downstream type 2C protein phosphatases (PP2Cs) to activate SNF1 (Sucrose-Non-Fermenting Kinase 1)-related protein kinase OPEN STOMATA1 (OST1)/SnRK2, which is inhibited by PP2C that promotes the dephosphorylation of Ser/Thr residues of OST1/SnRK2 to inactivate their activity (Umezawa et al., 2009; Mittler and Blumwald, 2015). Once activated, OST1 could be directly or indirectly combined, phosphorylate anion channel protein SLOW ANION CHANNEL-ASSOCIATED1, mediate ion release, cause guard cell movement, and promote stomatal closure (Geiger et al., 2009; Lee et al., 2009; Geiger et al, 2010; Brandt et al., 2012). Nicotinamide adenine dinucleotide phosphate (NADPH) oxidase is known as respiratory burst oxidase homologs (RBOHs), which is the main enzyme that promotes the generation of ROS in terrestrial plants (Umezawa et al., 2010; Mittler et al., 2011), and that is a homologue with mammalian NADPH oxidase in structure and function. RBOHs cross the plasma membrane to transport electrons from the inside to outside of the cell to form O2.-, and then generates H2O2 spontaneously or through superoxide dismutase (SOD) (Suzuki et al., 2011). At present, studies have reported that there are 10 RBOHs members in Arabidopsis, among them, RBOHD and RBOHF are the most representative members, and they play a very key role in the process of apoplast ROS synthesis, such as, caused by the interaction between plants and pathogens (Torres et al., 2002; Nühse et al., 2007; Zhang et al., 2007a). OST1 targets to bind RBOHF on the plasma membrane, phosphorylates the N-terminal Ser-13 and Ser-174 residues of RBOHF, and then generates H2O2 through the SOD outside the plasma membrane, whereas the regulation of ABA to NADPH through OST1 is ineffective in guard cells of the ost1 knockout mutant, and then resulting in the hindrance of ROS production (Wang and Song, 2008; Sirichandra et al., 2009). Interestingly, H2O2 production through OST1 regulation can be used as a feedback signal to inactivate PP2C activity, thereby further promoting the activity of OST1, forming a positive feedback loop (Raghavendra et al., 2010).
While plants live in drought and high salt environments, osmotic stress can induce a large amount of endogenous ABA enrichment to regulate the expression of stress resistance genes and the physiological processes in cells, which plays a very important role in the response of plants to adversity stress (Shi et al., 2013; Xie et al., 2016). Research evidence shows that ROS as a second messenger is an indispensable signal molecule in the ABA signal transduction pathway (Kwak, 2003; He et al., 2012; Shi et al., 2013; Suzuki et al., 2013; Gong et al., 2020). ABA stimulates the production of ROS through the NADPH oxidase of guard cells, and then induces the closure of stomata to adapt to drought stress condition (Xie et al., 2016). However, the mechanism disappears that ABA induces H2O2 production and stomata closure in the rbohD/rbohF double mutant, and reduces the inhibitory effect of ABA on root growing development in Arabidopsis (Kwak, 2003). The downstream transcription factor ABI1 (ABA INSENSITIVE1, ABI1) of ABA signal transduction pathway forms a complex with phosphatidic acid, which interacts with RBOHD/F to stimulate the generation of ROS (Zhang et al., 2009). Nevertheless, ABA loses the ability to induce ROS generation in the guard cells of the phospholipase Da1 mutant (PLDa1) (Zhang et al., 2004). ABA insensitivity factor ABI2 (ABA INSENSITIVE2, ABI2) also can interact with GPX3 to regulate the redox balance in guard cells (Miao et al., 2006). Research findings have confirmed that ROS is an important second messenger in the ABA signal transduction pathway.
ABA induces the opening of Ca2+ channels caused by hyperpolarization in the cytoplasmic membrane of guard cells, thereby increasing the concentration of Ca2+ in the cytoplasm (Schroeder and Hagiwara, 1990; Pei et al., 2000). As a signal molecule, ROS, especially H2O2, plays a very important role in ABA-mediated activation of Ca2+ channels (Pei et al., 2000; Kwak, 2003). The increase of the Ca2+ concentration in the cytoplasm activates the anion channel which binding the plasma membrane, leading to the depolarization of the plasma membrane, resulting in inhibition of the KAT1 K+ channel (Osakabe et al., 2014). Interestingly, OST1 can phosphorylate the C-terminal domain of KAT1, thereby inhibiting K+ channel activity (Sato et al., 2009). Therefore, it is hypothesized that ABA and Ca2+ play a synergistic role in regulating K+ channel closure. Ca2+ is very important for the activation of RBOHD in the cell. The combination of Ca2+ and EF hand is necessary for the activation of RBOHD (Ogasawara et al., 2008). In addition, the activation of CPKs promoted by Ca2+ is also essential for the activation of RBOHD (Boudsocq et al., 2010), such as, CPK5 phosphorylates the N-terminus of RBOHD to promote its activity (Dubiella et al., 2013). Although Ca2+ and CPKs are upstream of RBOHD-induced ROS generation, ROS can promote intracellular Ca2+ accumulation and activate CPK5 in turn (Dubiella et al., 2013). Studies have reported that ROS and Ca2+ may play an important role in the signal transmission between cells, and the long-distance transmission of Ca2+ and ROS is called Ca2+ wave or ROS wave, which may be effective in the system response of plants to biotic or abiotic stress as an important signaling molecule (Mittler et al., 2011; Gilroy et al., 2016).
In the ABA signal transduction pathway, OST1 is an essential signal element in the process of ABA-induced ROS generation (Mustilli et al., 2002). Moreover, studies have found that OST1 can phosphorylate the N-terminus of RBOHF (Sirichandra et al., 2009), but it is unclear whether this phosphorylation is necessary for OST1-mediated signal transduction. The latest research shows that OST1 persulfidation can enhance ABA-induced intracytoplasmic Ca2+ signal transduction and stomatal closure, and this persulfidation is related to the two Cys residues of OST1, which are very close to the kinase catalytic region and phosphorylation site of OST1 (Chen et al., 2020). However, it is interesting that recent studies have found that the phosphorylation of OST1 by BAK1 (BRI1-associated receptor kinase1, BAK1) is necessary for the process of ABA-induced ROS generation in guard cells (Shang et al., 2016). Considering that BAK1 is a co-receptor for LRR-RLKs (leucine-rich repeats receptor-like kinases, LRR-RLKs), it is speculated that OST1 may also be regulated by a certain LRR-RLKs. Excitingly, the latest research has discovered for the first time that the plant H2O2 receptor HPCA1 is an LRR receptor kinase located on the plasma membrane of the cell with two special Cys residues in its extracellular domain. Because the sulfhydryl group on the Cys residue is the target of H2O2 oxidation, H2O2 will cause the oxidation of the extracellular Cys residues of HPCA1 in guard cells, thereby activating the intracellular kinase activity of HPCA1, and further triggering the activation of Ca2+ channels and causing Ca2+ internal flow, which subsequently causes the stomatal closure. HPCA1-mediated H2O2-induced activation of Ca2+ channels is necessary for stomatal closure in guard cells (Wu et al., 2020). This receptor kinase-mediated H2O2 sensing mechanism is not similar to any known H2O2 receptor or sensor protein reported in other organisms. However, whether the endogenous H2O2 regulates the Cys residue on OST1, and then regulates ABA signal pathway, or exists a feedback signal synergy pathway between ABA and H2O2, maybe that is a new research entry point. Meanwhile, studies have found that H2O2 promoted by ABA inhibits the interaction of PP45 and DMI3 in rice, thereby activating DMI3, which can combine with calmodulin induced by the ABA signal pathway to regulate the ABA signal transduction process (Ni et al., 2019). It can be seen that H2O2 regulates the ABA signaling pathway in different ways.
The crosstalk between ABA and ROS in plant hormones network
Plant hormones can bind and induce downstream regulatory factors to affect plant growth and development in biotic and abiotic stresses, such as ABA, ethylene and brassinolide. Among them, ABA and ethylene, as two vital plant hormones, play important roles in many aspects of plant growth and development, including the response to adversity stresses (Bleecker and Kende, 2000; Finkelstein et al., 2002; Vishwakarma et al., 2017; Yin et al., 2017). Reports have shown that the accumulation of ROS in plants can not only regulate ABA-induced stomata closure and the activity of root meristems mediated by salicylic acid, but also play an important role in the Na balance mechanism in buds regulated by ethylene (Pei et al., 2000; Jiang et al., 2012; Jiang and Bel, 2013; Xu et al., 2017a). Therefore, it is speculated that there may be an important regulatory relationship between ROS and the plant hormone network, which plays an important role in the growing development of plants (Xia et al., 2015). For example, ABA induces the accumulation of ROS, which leads to the increase of Ca2+ content in guard cells, regulates stomatal closure in turn (Pei et al., 2000; Wang and Song, 2008). On the contrary, exogenous ABA treatment will decrease the level of ROS in rice seed germination embryos (Ye et al., 2012) (Figure 2). Ethylene can trigger the accumulation of ROS in the ozone-induced PCD and response to pathogenic bacteria to achieve the primary response of plant immunity (Overmyer et al., 2000; Overmyer et al., 2003; Mersmann et al., 2010; Tintor et al., 2013). Recent studies have shown that ethylene can regulate the MPK pathway by promoting RBOHF-mediated accumulation of ROS, which in turn leads to the accumulation of NO in plants and promotes the closure of stomata, in addition, as an important factor in the MPK pathway, MPK3/6 plays a role in the upstream of EIN2, indicating that ROS directly participates in the signal transduction regulation of ethylene (Zhang et al., 2021). Conversely, ethylene replies to the seedlings’ response to salt stress and photooxidation damage by reducing the level of ROS (Zhong et al., 2009; Peng et al., 2014). The results of these studies indicate that there may be a mechanism by which ABA and ethylene co-regulate the accumulation of ROS, which is a key element in elucidating the regulatory network between plant hormones and ROS. Interestingly, many reports show that ABA and ethylene exhibit two relationships in different biological processes, synergy and antagonism (Figure 2). For example, ethylene inhibits the growth of rice roots by promoting the biosynthesis of ABA (Yin et al., 2015). In addition, when plants respond to nutrient stress, the endoderm of roots will form plugging, while the effects of ABA and ethylene on root plugging are completely opposite (Barberon et al., 2016). ABA and ethylene jointly regulate the histone acetylation of SWI-INDEPENDENT 3 LIKE1 and SWI-INDEPENDENT 3 LIKE2 to achieve an antagonistic effect on seed dormancy (Wang et al., 2013).
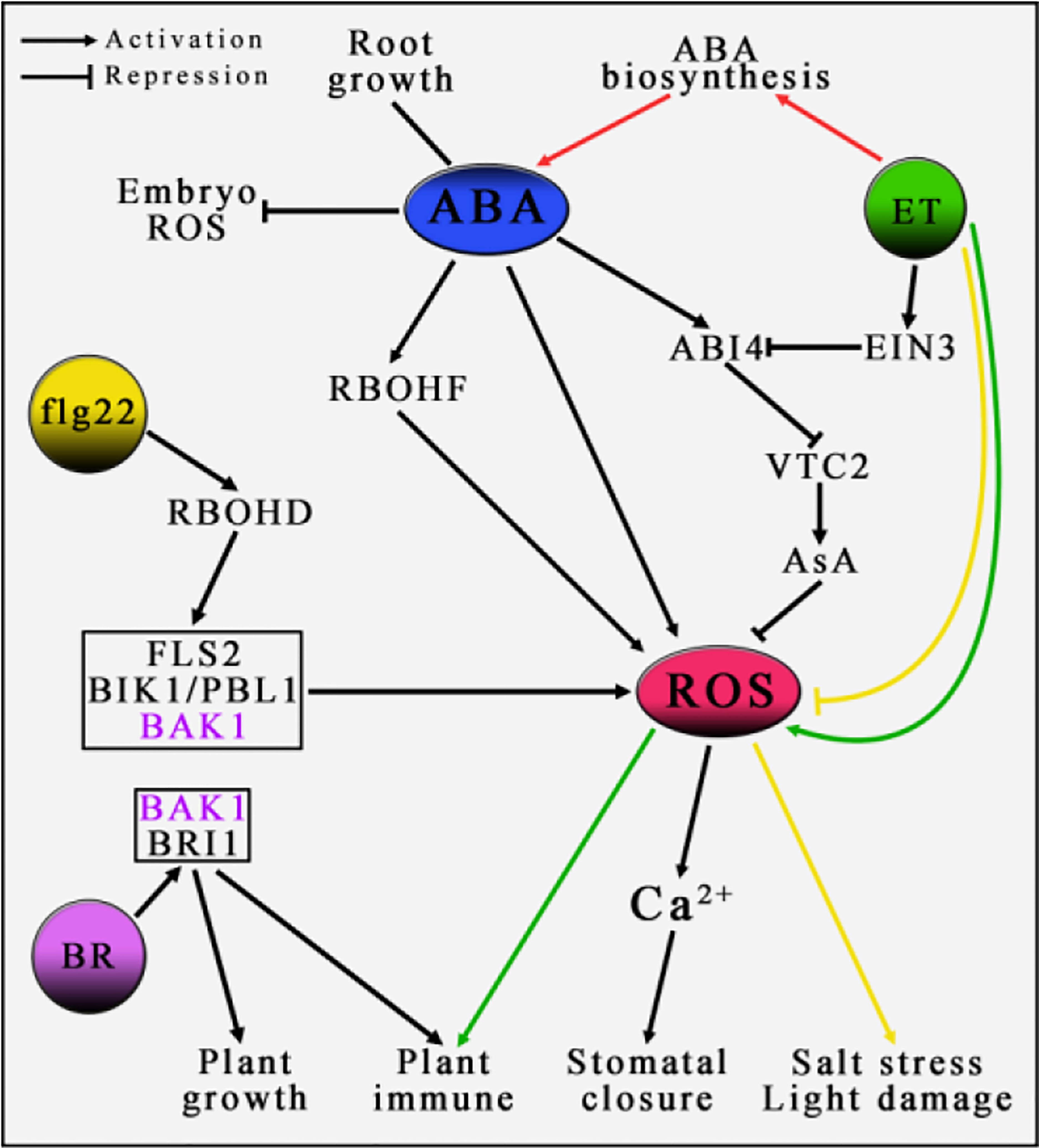
Figure 2 The role of ROS in the regulation of plant by hormones. In the process of plant growth and development, plant hormones play an important regulatory role, such as ABA, ET, BR, etc. In the entire regulatory network, ROS may play a central pivotal role. It remains to be elucidated whether BAK1, as an important element in the pathway of ROS generation induced by flg22, is the key site associated with the BR signaling pathway. Lines of the same color indicate the same regulatory pathway. For detailed explanation, please see text.
Ascorbate acid (AsA), as an important and highly effective non-enzymatic antioxidant, not only removes ROS generated during photosynthesis and growing development, but also plays an important role in the response of plants to biotic and abiotic stresses (Davey et al., 2000; Smirnoff and Wheeler, 2000; Gallie, 2013; Akram et al., 2017). With the discovery of multiple roles of AsA in the growing development of various plants, AsA and its regulatory mechanisms have received more and more attention (De Tullio and Arrigoni, 2004; Gallie, 2013; Bulley and Laing, 2016). Studies have reported that AsA plays an important role in the antagonistic relationship between ABA and ethylene. The AsA synthesis gene VTC2 is antagonistically regulated by the downstream factors ABI4 and EIN3 in the ABA and ethylene signal pathway respectively. EIN3 directly binds to the promoter of ABI4 to inhibit its expression, and ABI4 directly binds to the promoter of VTC2 to inhibit its expression, revealing a new mechanism of ABA and ethylene antagonistic regulation, that is to say, regulating the biosynthesis of AsA through the transcriptional cascade antagonism of EIN3-ABI4-VTC2, thereby affecting the accumulation of ROS in Arabidopsis thaliana seedlings (Yu et al., 2019) (Figure 2). With continuous literature reports, the regulatory relationship between ABA and ethylene on plant growing development has shown a complex regulatory network. However, the detailed mechanisms and key connections are still poorly understood.
Similar to the pathway that ABA induces RBOHF to trigger apoplast ROS generation, triggering apoplast ROS generation by the activation of RBOHD induced by bacterial flagellin epitope flg22 (Chinchilla et al., 2006), and the combination of receptors on the plasma membrane is composed of FLS2 (FLAGELLIN-SENSING-2), BIK1 (BOTRYTIS-INDUCED KINASE1) and PBL1 (PBS1-Like 1) (Lu et al., 2010; Zhang et al., 2010). Among them, BIK1 and PBL1 are germane cytoplasmic protein kinases, and FLS2 is a leucine-rich repeat receptor kinases (LRR-RLKs). When flg22 induces apoplast ROS generation, another LRR-RLK, BAK1 (BRI1-ASSOCIATED RECEPTOR KINASE 1) acts as a co-receptor to sense flg22 signal and jointly promote the transphosphorylation of FLS2, BAK1 and BIK1/PBL1 (Chinchilla et al., 2007; Heese et al., 2007; Lu et al., 2010; Zhang et al., 2010), thereby triggering a transient burst of Ca2+ in the cytoplasm (Li et al., 2014; Ranf et al., 2014; Monaghan et al., 2015), apoplast ROS generation (Lu et al., 2010; Zhang et al., 2010), and resistance to bacteria and pathogenic fungi (Veronese et al., 2006; Zhang et al., 2010). Interestingly, BAK1 acts as a brassinolide (BR) signaling regulatory element interacts with BR receptor BRI1 (Li et al., 2002; Nam and Li, 2002; Russinova et al., 2004), and co-phosphorylated to perceive and transmit BR signals (Wang et al., 2005; Wang et al., 2008), thereby mediate BR-regulated plant growth and development. Studies have found that BAK1 is not necessary in the FLS2-mediated plant immune signal pathway and BR-induced plant PAMP signal to pathogenic fungi, indicating that BAK1 may not be an intermediate element in the ABA, ROS and BR signaling networks (Figure 2). However, BAK1 can phosphorylate SnRK2.6 (Shang et al., 2016), and BIN2 kinase interacts with SnRK2.2 (Belin et al., 2006). It remains to be proved that SnRK2s and BIN2 may link ABA and BR signaling pathways, and mediate ROS generation as the key element.
Future perspective
Under normal physiological conditions, the production and consumption of ROS in cells is in a dynamic equilibrium state. An appropriate amount of ROS is of great significance to the signal transduction in plant cells and the immunity of pathogenic microorganisms. However, excessive ROS will lead to oxidative stresses, and the generation of stresses will be accompanied by a series of negative effects, such as irreversible damage to proteins, biofilms, DNA and RNA. Recent study has found that increased ROS can cause DNA breakage in maize sperm cells, resulting in haploid maize plants (Jiang et al., 2022). There are many factors that cause plant cells to produce excessive ROS, such as ABA, high light, adversity stress, and pathogen infiltration. Among them, apoplast ROS is mainly induced by the plasma membrane binding protein NADPH oxidase (RBOH). In addition to the NADPH oxidase of apoplasts, there are also some oxidases located on the plasma membrane of chloroplasts, peroxisomes, and mitochondria, which are also related to the production of ROS, especially during the interaction between plants and pathogens (Bolwell and Wojtaszek, 1997; Apel and Hirt, 2004). The main elements involved in the generation of apoplast ROS are rich in leucine and belong to the LRR-RLK class of protein molecules. The newly discovered plant H2O2 receptor HPCA1 is also an LRR-RLK protein molecule located on the cytomembrane. Cys residues exposed outside the cytomembrane are covalent modification by H2O2, leading to HPCA1 autophosphorylation of the part inside cell, and then gets activated (Wu et al., 2020). H2O2 is an important molecule for cells to respond to external stresses and internal signals. H2O2 enters into the cell through the water channel membrane proteins and covalently modified cytoplasmic proteins to regulate signal transduction and cellular processes (Figure 3).
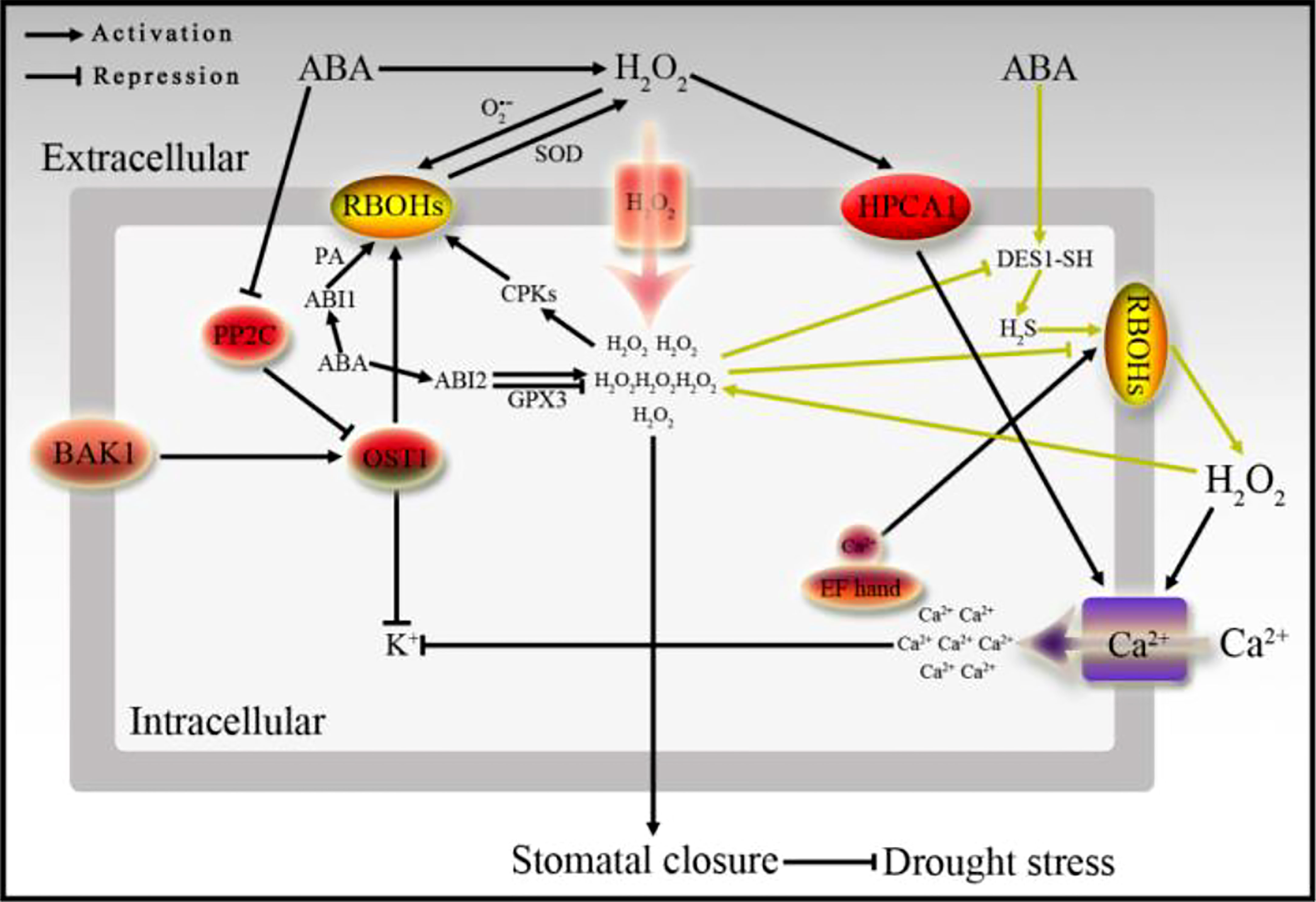
Figure 3 The relationship between ABA signaling and H2O2 in stomatal movement. ABA signal is transmitted downward through PP2C and OST1, or ABI1 and ABI2, meanwhile, ABA can promote the generation of H2O2 induced by RBOHs on the plasma membrane, and forms a microcirculation by H2S. H2O2 is sensed by the H2O2 receptor HPCA1, and the H2O2 signal is transmitted into the cell to promote Ca2+ influx, inhibit K+ accumulation, and then causes stomata to close.
It has been reported in the literature that H2S is involved in ABA-regulated ROS production and stomatal closure, conversely, excessive accumulation of ROS inhibits H2S production, thus forming a mini-regulatory circuit (Jie et al., 2020) (Figure 3). In addition, hydrogen sulfide (H2S) modifies SnRK2.6/OST1 through S-nitrosylation to participate in the molecular mechanism of regulating ABA signaling to induce plant stomatal closure (Chen et al., 2020). Interestingly, two S-sulfhydryl modification sites-Cys residues are found in SnRK2.6, when these two Cys residues are modified by S-sulfhydryl, the activity of SnRK2.6 and its interaction with transcription factors downstream of ABA signaling all will be promoted (Chen et al., 2020). Meanwhile, ABA and H2S-induced stomatal closure and Ca2+ influx will be less sensitive, and leading to water loss and decreased drought resistance in the ost1-3 mutant (Chen et al., 2020). In addition, ABA induces the DES1 enzyme in the guard cells to catalyze the production of H2S, in turn, the H2S positively regulates ABA signal transduction through the persulfidation of SnRK2.6 to affect calcium influx, and finally regulates stomatal movement, therefore, a new ABA signal transduction mechanism is proposed that relies on the regulation of sulfhydryl modification (Chen et al., 2020) (Figure 3). As mentioned above, the N-terminus of RBOHs contain multiple regulatory sites or motifs, including phosphorylation sites, EF hand, and phosphatidic acid binding elements, etc, which make RBOHs with binding and regulating effects (Ogasawara et al., 2008) (Figure 3). In addition to phosphorylation and sulfhydrylation, ubiquitination modification which is generally modified by E3 ubiquitin ligases is also an important regulation of ABA perception, signal transduction and response. PYR/PRLs, PP2Cs, PP2As, transcription factors and proteins encoded by ABA response genes are all targets of E3 ubiquitin ligase-mediated ubiquitination modification, and will be further degraded by the 26S proteasome. At present, it has been confirmed that a variety of E3 ubiquitin ligases are related to ABA signaling, including CRLs, RING and U-box types of ubiquitin ligases (Yu et al., 2015), and another type of E6-AP ubiquitin ligases have not been reported to be related to ABA signaling. Many ABA-related factors affect ABA signal transduction and stress resistance through the regulation of E3 ubiquitin ligases in plant growing, for example, ABA synthesis elements ABA1 and ABA2 (Lee et al., 2010), ABA receptor molecules PYL1 and PYL4 (Bueso et al., 2014), PYL8 (Irigoyen et al., 2014), ABA signaling factor ABI1 (Park et al., 2009; Kong et al., 2015), ABI2 and HAB2 (Wu et al., 2016), ABA signaling downstream transcription factor ABI3 (Kurup et al., 2000), ABI5 (Smalle et al., 2003; Lee et al., 2010; Seo et al., 2014), ABF1 (Chen et al., 2013), ABF3 (Chen et al., 2013), ATH86 (Lechner et al., 2011), SDIRIP1 (Zhang et al., 2007b; Zhang et al., 2015), and ABA downstream response gene RD21 (Kim and Kim, 2013), etc. In addition, recent studies have reported that the new E2 ubiquitin coupling enzyme UBC27 and the RING type E3 ubiquitin ligase AIRP3 form a specific E2-E3 complex and activate the ubiquitin ligase activity of AIRP3. Meanwhile, both UBC27 and AIRP3 can directly interact with the ABA co-receptor ABI1 to regulate ABA signaling and plant drought stress response (Pan et al., 2020). Ubiquitination modification basically regulates all the related fields of ABA in plants, and further affects plant growth and development, stress resistance, and interaction with pathogens. Although the modification and regulation mechanisms of many ABA-related elements have been studied and reported, the detailed ABA signaling network still needs to be verified and supplemented.
The photosynthesis of plants will be stimulated by high light to generate ROS in the cells, thereby causing light damage to plant cells. The latest research has found that the phytochrome-related transcription factor PHYTOCHROME-INTERACTING FACTORS (PIFs) directly binds to the promoter of the ABI5 and activating the expression of ABI5 specifically to regulate the ABA signaling pathway in the dark, meanwhile, ABA receptors PYL8/PYL9 can directly interact with PIFs and mediate this transcriptional regulation of ABI5 (Qi et al., 2020). PIFs are the key factors that integrate multiple signal pathways to regulate plant growth and development, and widely involved in a variety of plant hormones, such as gibberellin, ethylene, auxin, brassinolide, etc. meanwhile, mediated by external environmental factors, such as high temperature, high light, etc. in the signal regulation network (Paik et al., 2017). Whether the ROS generated by high light is involved in the signal transduction network that regulated by these plant hormones, we believe that the discovery of more ROS functions will play a very important role in the improvement of the signal regulation network. In addition, the interaction between ABA and auxin has been proven, ABA can promote auxin-mediated plant growth inhibition (Tiryaki and Staswick, 2002; Monroe-Augustus et al., 2003; Belin et al., 2009). In turn, auxin can promote ABA-mediated inhibition of seed germination and leaf senescence under different oxidative stress conditions (Liu et al., 2007; Mahmood et al., 2016). It shows that ABA and auxin co-promote the process of seed germination and seedling growth (Belin et al., 2009; Tsukagoshi et al., 2010). It is interesting to report that ABA can make use of ROS from mitochondria to antagonize the regulation of auxin in Arabidopsis. In turn, auxin can also attenuate the inhibitory effect of ABA on seed germination (He et al., 2012), indicating that there is both synergy and antagonism between ABA and auxin, which may be related to the concentration of plant hormones, and ROS plays an important role therein. In terms of plant defenses against pathogens, ABA may coordinate or antagonize the SA, JA, ET and other signal pathways through direct or indirect mechanisms, thereby regulating the antibacterial properties of plants (Mohr and Cahill, 2007; Robert-Seilaniantz et al., 2007; Derksen et al., 2013). This seems to be regarded as the main mechanism by which ABA induces the sensitivity of plants to a variety of pathogens. However, the role of ROS in these mechanisms has not been fully elucidated, this is also future research hotspots in plant disease resistance.
Studies have found that just restoring the expression of RBOHD in the xylem parenchyma or phloem cells in the rbohD mutant can restore the systemic ROS signal in plant. The transcriptional expression and the adaptive mechanism cause by systemic stress response to deal with the high light stimulation applied to a single leaf, indicating that RBOHD plays a key role in regulating the generation of the vascular bundles ROS, inducing systemic signal transduction and adaptation in Arabidopsis. Meanwhile, the signal integration of ROS and calcium, which are important signaling molecules for long-distance transport between cells or in plants, and electricity and hydraulic pressure occurs in the vascular bundle along with the process of systemic signal transduction (Zandalinas et al., 2020) (Figure 3). A recent review article also reported that ROS is produced, enriched, converted and transported across the membrane in several organelles of plant cells, including chloroplasts, mitochondria, endoplasmic reticulum, peroxisomes and vacuoles, etc. Meanwhile, ROS is transported across the membrane between plant cells to achieve ROS signal transmission throughout the plant (Mittler et al., 2022). This study suggests that we can express RBOHD/F specifically in different tissues, cells and organelles to perfect the understanding of RBOHD/F in mediating ROS fluctuations, and understanding of plant systemic responses induced by ROS. Improving plant ABA and ROS signal regulation components and pathways will help to further understand how plants adjust their endogenous signals and regulation strategies according to environmental changes, so as to obtain better survival ability in the natural. Additionally, that will provide new insights for the elucidation of plant hormone signal network and regulatory mechanism.
Author contributions
SHL, SL and LW designed this research and wrote the manuscript. LW, QZ, MC and MZ contributed to the provided guidance of the whole manuscript. LW, SL, QZ and RW reviewed and revised the manuscript. SHL, and LW prepared the figures. NL, SW, YC and LZ checked and revised the language and format of the manuscript. All authors contributed to the article and approved the submitted version.
Funding
This study were funded by Hebei Natural Science Foundation (C2022402021, C2022402026, C2019402343, C2019402430); The Science and Technology Research Project of University in Hebei Province (QN2020205); Science and Technology Research and Development Plan Project of Handan (19422011008–49, 21422012331).
Conflict of interest
The authors declare that the research was conducted in the absence of any commercial or financial relationships that could be construed as a potential conflict of interest.
Publisher’s note
All claims expressed in this article are solely those of the authors and do not necessarily represent those of their affiliated organizations, or those of the publisher, the editors and the reviewers. Any product that may be evaluated in this article, or claim that may be made by its manufacturer, is not guaranteed or endorsed by the publisher.
References
AbuQamar, S., Moustafa, K., Tran, L. S. (2017). Mechanisms and strategies of plant defense agains botrytis cinerea. Crit. Rev. Biotechnol. 37, 262–274. doi: 10.1080/07388551.2016.1271767
Achard, P., Renou, J. P., Berthomé, R., Harberd, N. P., Genschik, P. (2008). Plant DELLAs restrain growth and promote survival of adversity by reducing the levels of reactive oxygen species. Curr. Biol. 18, 656–660. doi: 10.1016/j.cub.2008.04.034
Agurla, S., Gahir, S., Munemasa, S., Murata, Y., Raghavendra, A. S. (2018). Mechanism of stomatal closure in plants exposed to drought and cold stress. Adv. Exp. Med. Biol. 1081, 215–232. doi: 10.1007/978-981-13-1244-1_12
Akram, N. A., Shafiq, F., Ashraf, M. (2017). Ascorbic acid-a potential oxidant scavenger and its role in plant development and abiotic stress tolerance. Front. Plant Sci. 8. doi: 10.3389/fpls.2017.00613
Apel, K., Hirt, H. (2004). REACTIVE OXYGEN SPECIES: Metabolism, oxidative stress, and signal transduction. Annu. Rev. Plant Biol. 55, 373–399. doi: 10.1146/annurev.arplant.55.031903.141701
Arif, M. A., Alseekh, S., Harb, J., Fernie, A., Frank, W. (2018). Abscisic acid, cold and salt stimulate conserved metabolic regulation in the moss physcomitrella patens. Plant Biol. J. 20, 1014–1022. doi: 10.1111/plb.12871
Asad, M., Zakari, S., Zhao, Q., Zhou, L., Ye, Y., Cheng, F. (2019). Abiotic stresses intervene with ABA signaling to induce destructive metabolic pathways leading to death: premature leaf senescence in plants. IJMS 20, 256. doi: 10.3390/ijms20020256
Audenaert, K., Meyer, G. B. D., Höfte, M. M. (2002). Abscisic acid determines basal susceptibility of tomato to botrytis cinerea and suppresses salicylic acid-dependent signaling mechanisms. Plant Physiol. 128, 491–501. doi: 10.1104/pp.010605
Barberon, M., Vermeer, J. E. M., De Bellis, D., Wang, P., Naseer, S., Andersen, T. G., et al. (2016). Adaptation of root function by nutrient-induced plasticity of endodermal differentiation. Cell 164, 447–459. doi: 10.1016/j.cell.2015.12.021
Belin, C., de Franco, P. O., Bourbousse, C., Chaignepain, S., Schmitter, J. M., Vavasseur, A., et al. (2006). Identification of features regulating OST1 kinase activity and OST1 function in guard cells. Plant Physiol. 141, 1316–1327. doi: 10.1104/pp.106.079327
Belin, C., Megies, C., Hauserová, E., Lopez-Molina, L. (2009). Abscisic acid represses growth of the arabidopsis embryonic axis after germination by enhancing auxin signaling. Plant Cell 21, 2253–2268. doi: 10.1105/tpc.109.067702
Bleecker, A. B., Kende, H. (2000). Ethylene: A gaseous signal molecule in plants. Annu. Rev. Cell Dev. Biol. 16, 1–18. doi: 10.1146/annurev.cellbio.16.1.1
Bolwell, G. P., Wojtaszek, P. (1997). Mechanisms for the generation of reactive oxygen species in plant defence–a broad perspective. Physiol. Mol. Plant Pathol. 51, 347–366. doi: 10.1006/pmpp.1997.0129
Boudsocq, M., Willmann, M. R., McCormack, M., Lee, H., Shan, L., He, P., et al. (2010). Differential innate immune signalling via Ca2+ sensor protein kinases. Nature 464, 418–422. doi: 10.1038/nature08794
Brandt, B., Brodsky, D. E., Xue, S., Negi, J., Iba, K., Kangasjarvi, J., et al. (2012). Reconstitution of abscisic acid activation of SLAC1 anion channel by CPK6 and OST1 kinases and branched ABI1 PP2C phosphatase action. Proc. Natl. Acad. Sci. U.S.A. 109, 10593–10598. doi: 10.1073/pnas.1116590109
Bueso, E., Rodriguez, L., Lorenzo-Orts, L., Gonzalez-Guzman, M., Sayas, E., Muñoz-Bertomeu, J., et al. (2014). The single-subunit RING-type E3 ubiquitin ligase RSL1 targets PYL4 and PYR1 ABA receptors in plasma membrane to modulate abscisic acid signaling. Plant J. 80, 1057–1071. doi: 10.1111/tpj.12708
Bulley, S., Laing, W. (2016). The regulation of ascorbate biosynthesis. Curr. Opin. Plant Biol. 33, 15–22. doi: 10.1016/j.pbi.2016.04.010
Cao, F. Y., Yoshioka, K., Desveaux, D. (2011). The roles of ABA in plant–pathogen interactions. J. Plant Res. 124, 489–499. doi: 10.1007/s10265-011-0409-y
Chen, S., Jia, H., Wang, X., Shi, C., Wang, X., Ma, P., et al. (2020). Hydrogen sulfide positively regulates abscisic acid signaling through persulfidation of SnRK2.6 in guard cells. Mol. Plant 13, 732–744. doi: 10.1016/j.molp.2020.01.004
Chen, Y., Liu, H., Stone, S., Callis, J. (2013). ABA and the ubiquitin E3 ligase KEEP ON GOING affect proteolysis of the arabidopsis thaliana transcription factors ABF1 and ABF3. Plant J. 75, 965–976. doi: 10.1111/tpj.12259
Chinchilla, D., Bauer, Z., Regenass, M., Boller, T., Felix, G. (2006). The arabidopsis receptor kinase FLS2 binds fig22 and determines the specificity of flagellin perception. Plant Cell 18, 465–476. doi: 10.2307/20076607
Chinchilla, D., Zipfel, C., Robatzek, S., Kemmerling, B., Nürnberger, T., Jones, J. D. G., et al. (2007). A flagellin-induced complex of the receptor FLS2 and BAK1 initiates plant defence. Nature 448, 497–500. doi: 10.1038/nature05999
Chi, M. H., Park, S. Y., Kim, S., Lee, Y. H. (2009). A novel pathogenicity gene is required in the rice blast fungus to suppress the basal defenses of the host. PloS Pathog. 5, e1000401. doi: 10.1371/journal.ppat.1000401
Choudhury, F. K., Rivero, R. M., Blumwald, E., Mittler, R. (2017). Reactive oxygen species, abiotic stress and stress combination. Plant J. 90, 856–867. doi: 10.1111/tpj.13299
Darma, R., Lutz, A., Elliott, C. E., Idnurm, A. (2019). Identification of a gene cluster for the synthesis of the plant hormone abscisic acid in the plant pathogen leptosphaeria maculans. Fungal Genet. Biol. 130, 62–71. doi: 10.1016/j.fgb.2019.04.015
Davey, M. W., Montagu, M. V., Inz, D., Sanmartin, M., Kanellis, A., Smirnoff, N., et al. (2000). PlantL-ascorbic acid: chemistry, function, metabolism, bioavailability and effects of processing. J. Sci. Food Agric. 80, 825–860. doi: 10.1002/(SICI)1097-0010(20000515)80:7<825::AID-JSFA598>3.0.CO;2-6
del Pozo, J. C. (2016). Reactive oxygen species: From harmful molecules to fine-tuning regulators of stem cell niche maintenance. PloS Genet. 12, e1006251. doi: 10.1371/journal.pgen.1006251
Derksen, H., Rampitsch, C., Daayf, F. (2013). Signaling cross-talk in plant disease resistance. Plant Sci. 207, 79–87. doi: 10.1016/j.plantsci.2013.03.004
de Torres Zabala, M., Bennett, M. H., Truman, W. H., Grant, M. R. (2009). Antagonism between salicylic and abscisic acid reflects early host-pathogen conflict and moulds plant defence responses. Plant J. 59, 375–386. doi: 10.1111/j.1365-313X.2009.03875.x
de Torres-Zabala, M., Truman, W., Bennett, M. H., Lafforgue, G., Mansfield, J. W., Rodriguez Egea, P., et al. (2007). Pseudomonas syringae pv. tomato hijacks the arabidopsis abscisic acid signalling pathway to cause disease. EMBO J. 26, 1434–1443. doi: 10.1038/sj.emboj.7601575
De Tullio, M. C., Arrigoni, O. (2004). Hopes, disillusions and more hopes from vitamin c. Cell Mol. Life Sci. 61, 209–219. doi: 10.1007/s00018-003-3203-8
Dietz, K. J., Turkan, I., Krieger-Liszkay, A. (2016). Redox and reactive oxygen species-dependent signaling into and out of the photosynthesizing chloroplast. Plant Physiol. 171, 1541–1550. doi: 10.1104/pp.16.00375
Dong, W., Wang, M., Xu, F., Quan, T., Peng, K., Xiao, L., et al. (2013). Wheat oxophytodienoate reductase gene TaOPR1 confers salinity tolerance via enhancement of abscisic acid signaling and reactive oxygen species scavenging. Plant Physiol. 161, 1217–1228. doi: 10.1104/pp.112.211854
Dubiella, U., Seybold, H., Durian, G., Komander, E., Lassig, R., Witte, C. P., et al. (2013). Calcium-dependent protein kinase/NADPH oxidase activation circuit is required for rapid defense signal propagation. Proc. Natl. Acad. Sci. U.S.A. 110, 8744–8749. doi: 10.1073/pnas.1221294110
Dunand, C., Crèvecoeur, M., Penel, C. (2007). Distribution of superoxide and hydrogen peroxide in arabidopsis root and their influence on root development: possible interaction with peroxidases. New Phytol. 174, 332–341. doi: 10.1111/j.1469-8137.2007.01995.x
Ensminger, I., Busch, F., Huner, N. P. A. (2006). Photostasis and cold acclimation: sensing low temperature through photosynthesis. Physiol. Plant 126, 28–44. doi: 10.1111/j.1399-3054.2006.00627.x
Finkelstein, R. R., Gampala, S. S. L., Rock, C. D. (2002). Abscisic acid signaling in seeds and seedlings. Plant Cell 14, S15–S45. doi: 10.1105/tpc.010441
Foreman, J., Demidchik, V., Bothwell, J. H. F., Mylona, P., Miedema, H., Torres, M. A., et al. (2003). Reactive oxygen species produced by NADPH oxidase regulate plant cell growth. Nature 422, 442–446. doi: 10.1038/nature01485
Foyer, C. H., Noctor, G. (2005). Redox homeostasis and antioxidant signaling: A metabolic interface between stress perception and physiological responses. Plant Cell 17, 1866–1875. doi: 10.1105/tpc.105.033589
Frank, V. B., James, F. D. (2006). Reactive oxygen species in plant cell death. Plant Physiol. 141, 384–390. doi: 10.1104/pp.106.078295
Fujii, H., Verslues, P. E., Zhu, J. K. (2007). Identification of two protein kinases required for abscisic acid regulation of seed germination, root growth, and gene expression in arabidopsis. Plant Cell 19, 485–494. doi: 10.1105/tpc.106.048538
Gallie, D. R. (2013). L-ascorbic acid: A multifunctional molecule supporting plant growth and development. Scientifica 2013, 1–24. doi: 10.1155/2013/795964
Gan, S., Amasino, R. M. (1997). Making sense of senescence (Molecular genetic regulation and manipulation of leaf senescence). Plant Physiol. 113, 313–319. doi: 10.1104/pp.113.2.313
Gechev, T., Willekens, H., Van Montagu, M., Inzé, D., Van Camp, W., Toneva, V., et al. (2003). Different responses of tobacco antioxidant enzymes to light and chilling stress. J. Plant Physiol. 160, 509–515. doi: 10.1078/0176-1617-00753
Geiger, D., Scherzer, S., Mumm, P., Marten, I., Ache, P., Matschi, S., et al. (2010). Guard cell anion channel SLAC1 is regulated by CDPK protein kinases with distinct Ca2+ affinities. Proc. Natl. Acad. Sci. U.S.A. 107, 8023–8028. doi: 10.1073/pnas.0912030107
Geiger, D., Scherzer, S., Mumm, P., Stange, A., Marten, I., Bauer, H., et al. (2009). Activity of guard cell anion channel SLAC1 is controlled by drought-stress signaling kinase-phosphatase pair. Proc. Natl. Acad. Sci. U.S.A. 106, 21425–21430. doi: 10.1073/pnas.0912021106
Ghassemian, M., Lutes, J., Chang, H. S., Lange, I., Chen, W., Zhu, T., et al. (2008). Abscisic acid-induced modulation of metabolic and redox control pathways in arabidopsis thaliana. Phytochemistry 69, 2899–2911. doi: 10.1016/j.phytochem.2008.09.020
Gilroy, S., Białasek, M., Suzuki, N., Górecka, M., Devireddy, A. R., Karpiński, S., et al. (2016). ROS, calcium, and electric signals: Key mediators of rapid systemic signaling in plants. Plant Physiol. 171, 1606–1615. doi: 10.1104/pp.16.00434
Gong, Z., Xiong, L., Shi, H., Yang, S., Herrera-Estrella, L. R., Xu, G., et al. (2020). Plant abiotic stress response and nutrient use efficiency. Sci. China Life Sci. 63, 635–674. doi: 10.1007/s11427-020-1683-x
Gosti, F., Beaudoin, N., Serizet, C., Webb, A. A., Vartanian, N., Giraudat, J. (1999). ABI1 protein phosphatase 2C is a negative regulator of abscisic acid signaling. Plant Cell 11, 1897–1910. doi: 10.1105/tpc.11.10.1897
Grant, M. R., Jones, J. D. G. (2009). Hormone (Dis) harmony moulds plant health and disease. Science 324, 750–752. doi: 10.1126/science.1173771
Hartung, W. (2010). The evolution of abscisic acid (ABA) and ABA function in lower plants, fungi and lichen. Funct. Plant Biol. 37, 806. doi: 10.1071/FP10058
Haudecoeur, E., Planamente, S., Cirou, A., Tannieres, M., Shelp, B. J., Morera, S., et al. (2009). Proline antagonizes GABA-induced quenching of quorum-sensing in agrobacterium tumefaciens. Proc. Natl. Acad. Sci. U.S.A. 106, 14587–14592. doi: 10.1073/pnas.0808005106
Hauser, F., Waadt, R., Schroeder, J. I. (2011). Evolution of abscisic acid synthesis and signaling mechanisms. Curr. Biol. 21, R346–R355. doi: 10.1016/j.cub.2011.03.015
He, J., Duan, Y., Hua, D., Fan, G., Wang, L., Liu, Y., et al. (2012). DEXH box RNA helicase–mediated mitochondrial reactive oxygen species production in arabidopsis mediates crosstalk between abscisic acid and auxin signaling. Plant Cell 24, 1815–1833. doi: 10.1105/tpc.112.098707
Heese, A., Hann, D. R., Gimenez-Ibanez, S., Jones, A. M. E., He, K., Li, J., et al. (2007). The receptor-like kinase SERK3/BAK1 is a central regulator of innate immunity in plants. Proc. Natl. Acad. Sci. U.S.A. 104, 12217–12222. doi: 10.1073/pnas.0705306104
Hong, Z., Lakkineni, K., Zhang, Z., Verma, D. P. S. (2000). Removal of feedback inhibition of delta(1)-pyrroline-5- carboxylate synthetase results in increased proline accumulation and protection of plants from osmotic stress. Plant Physiol. 122, 1129–1136. doi: 10.1104/pp.122.4.1129
Hong, Y., Wang, Z., Liu, X., Yao, J., Kong, X., Shi, H., et al. (2020). Two chloroplast proteins negatively regulate plant drought resistance through separate pathways. Plant Physiol. 182, 1007–1021. doi: 10.1104/pp.19.01106
Hoque, M., Banu, M. N.A., Nakamura, Y., Shimoishi, Y., Murata, Y. (2008). Proline and glycinebetaine enhance antioxidant defense and methylglyoxal detoxification systems and reduce NaCl-induced damage in cultured tobacco cells. J. Plant Physiol. 165, 813–824. doi: 10.1016/j.jplph.2007.07.013
Huang, X., Hou, L., Meng, J., You, H., Li, Z., Gong, Z., et al. (2018). The antagonistic action of abscisic acid and cytokinin signaling mediates drought stress response in arabidopsis. Mol. Plant 11, 970–982. doi: 10.1016/j.molp.2018.05.001
Huang, X., Shi, H., Hu, Z., Liu, A., Amombo, E., Chen, L., et al. (2017). ABA is involved in regulation of cold stress response in bermudagrass. Front. Plant Sci. 8. doi: 10.3389/fpls.2017.01613
Huda, K. M. K., Banu, M. S. A., Garg, B., Tula, S., Tuteja, R., Tuteja, N. (2013). OsACA6 , a p-type IIB Ca2+ ATPase promotes salinity and drought stress tolerance in tobacco by ROS scavenging and enhancing the expression of stress-responsive genes. Plant J. 76, 997–1015. doi: 10.1111/tpj.12352
Hu, C. A., Delauney, A. J., Verma, D. P. (1992). A bifunctional enzyme (delta 1-pyrroline-5-carboxylate synthetase) catalyzes the first two steps in proline biosynthesis in plants. Proc. Natl. Acad. Sci. U.S.A. 89, 9354–9358. doi: 10.1073/pnas.89.19.9354
Irigoyen, M. L., Iniesto, E., Rodriguez, L., Puga, M. I., Yanagawa, Y., Pick, E., et al. (2014). Targeted degradation of abscisic acid receptors is mediated by the ubiquitin ligase substrate adaptor DDA1 in arabidopsis. Plant Cell 26, 712–728. doi: 10.1105/tpc.113.122234
Jiang, C., Bel, E. J. (2013). An arabidopsis Soil-Salinity–tolerance mutation confers ethylene-mediated enhancement of Sodium/Potassium HomeostasisW. Plant Cell 25, 3535–3552. doi: 10.2307/23598366
Jiang, C., Belfield, E. J., Mithani, A., Visscher, A., Ragoussis, J., Mott, R., et al. (2012). ROS-mediated vascular homeostatic control of root-to-shoot soil Na delivery in arabidopsis: ROS-mediated control of Na homeostasis. EMBO J. 31, 4359–4370. doi: 10.1038/emboj.2012.273
Jiang, C., Sun, J., Li, R., Yan, S., Chen, W., Guo, L., et al. (2022). A reactive oxygen species burst causes haploid induction in maize. Mol. Plant 15, 943–955. doi: 10.1016/j.molp.2022.04.001
Jiang, M., Zhang, J. (2002). Involvement of plasma-membrane NADPH oxidase in abscisic acid- and water stress-induced antioxidant defense in leaves of maize seedlings. Planta 215, 1022–1030. doi: 10.1007/s00425-002-0829-y
Jie, S., Jing, Z., Mingjian, Z., Heng, Z., Beimi, C., Cecilia, G., et al. (2020). Persulfidation-based modification of cysteine desulfhydrase and the NADPH oxidase RBOHD controls guard cell abscisic acid signaling. Plant Cell 32, 1000–1017. doi: 10.1105/tpc.19.00826
Kavi Kishor, P. B., Sreenivasulu, N. (2014). Is proline accumulation perse correlated with stress tolerance or is proline homeostasis a more critical issue? Plant Cell Environ. 37, 300–311. doi: 10.1111/pce.12157
Kim, J. H., Kim, W. T. (2013). The arabidopsis RING E3 ubiquitin ligase AtAIRP3/LOG2 participates in positive regulation of high-salt and drought stress responses. Plant Physiol. 162, 1733–1749. doi: 10.1104/pp.113.220103
Kong, L., Cheng, J., Zhu, Y., Ding, Y., Meng, J., Chen, Z., et al. (2015). Degradation of the ABA co-receptor ABI1 by PUB12/13 U-box E3 ligases. Nat. Commun. 6, 1–13. doi: 10.1038/ncomms9630
Kurup, S., Jones, H. D., Holdsworth, M. J. (2000). Interactions of the developmental regulator ABI3 with proteins identied from developing arabidopsis seeds. Plant J. 21, 143–155. doi: 10.1046/j.1365-313x.2000.00663.x
Kwak, J. M. (2003). NADPH oxidase AtrbohD and AtrbohF genes function in ROS-dependent ABA signaling in arabidopsis. EMBO J. 22, 2623–2633. doi: 10.1093/emboj/cdg277
Lechner, E., Leonhardt, N., Eisler, H., Parmentier, Y., Alioua, M., Jacquet, H., et al. (2011). MATH/BTB CRL3 receptors target the homeodomain-leucine zipper ATHB6 to modulate abscisic acid signaling. Dev. Cell 21, 1116–1128. doi: 10.1016/j.devcel.2011.10.018
Lee, S. C., Lan, W., Buchanan, B. B., Luan, S. (2009). A protein kinase-phosphatase pair interacts with an ion channel to regulate ABA signaling in plant guard cells. Proc. Natl. Acad. Sci. U.S.A. 106, 21419–21424. doi: 10.1073/pnas.0910601106
Lee, S. C., Luan, S. (2012). ABA signal transduction at the crossroad of biotic and abiotic stress responses: ABA in drought and pathogen responses. Plant Cell Environ. 35, 53–60. doi: 10.1111/j.1365-3040.2011.02426.x
Lee, J. H., Yoon, H. J., Terzaghi, W., Martinez, C., Dai, M., Li, J., et al. (2010). DWA1 and DWA2, two arabidopsis DWD protein components of CUL4-based E3 ligases, act together as negative regulators in ABA signal transduction. Plant Cell 22, 1716–1732. doi: 10.1105/tpc.109.073783
Leng, Y., Yang, Y., Ren, D., Huang, L., Dai, L., Wang, Y., et al. (2017). A rice PECTATE LYASE-LIKE gene is required for plant growth and leaf senescence. Plant Physiol. 174, 1151–1166. doi: 10.1104/pp.16.01625
Leung, J., Giraudat, J. (1998). Abscisic acid signal transducyion. Annu. Rev. Plant Physiol. Plant Mol. Biol. 49, 199–222. doi: 10.1146/annurev.arplant.49.1.199
Li, L., Li, M., Yu, L., Zhou, Z., Liang, X., Liu, Z., et al. (2014). The FLS2-associated kinase BIK1 directly phosphorylates the NADPH oxidase RbohD to control plant immunity. Cell Host Microbe 15, 329–338. doi: 10.1016/j.chom.2014.02.009
Lim, C., Baek, W., Jung, J., Kim, J. H., Lee, S. (2015). Function of ABA in stomatal defense against biotic and drought stresses. Int. J. Mol. Sci. 16, 15251–15270. doi: 10.3390/ijms160715251
Liszkay, A., van der Zalm, E., Schopfer, P. (2004). Production of reactive oxygen intermediates (O2–, H2O2, and OH-) by maize roots and their role in wall loosening and elongation growth. Plant Physiol. 136, 3114–3123. doi: 10.1104/pp.104.044784
Liu, P. P., Montgomery, T. A., Fahlgren, N., Kasschau, K. D., Nonogaki, H., Carrington, J. C. (2007). Repression of AUXIN RESPONSE FACTOR10 by microRNA160 is critical for seed germination and post-germination stages: microRNA in arabidopsis seed germination. Plant J. 52, 133–146. doi: 10.1111/j.1365-313X.2007.03218.x
Li, Z., Wang, F., Zhao, Q., Liu, J., Cheng, F. (2018). Involvement of NADPH oxidase isoforms in the production of O2– manipulated by ABA in the senescing leaves of early-senescence-leaf (esl) mutant rice (Oryza sativa). PloS One 13, e0190161. doi: 10.1371/journal.pone.0190161
Li, J., Wen, J., Lease, K. A., Doke, J. T., Tax, F. E., Walker, J. C. (2002). BAK1, an arabidopsis LRR receptor-like protein kinase, interacts with BRI1 and modulates brassinosteroid signaling. Cell 110, 213–222. doi: 10.1016/S0092-8674(02)00812-7
Lu, Z., Liu, D., Liu, S. (2007). Two rice cytosolic ascorbate peroxidases differentially improve salt tolerance in transgenic arabidopsis. Plant Cell Rep. 26, 1909–1917. doi: 10.1007/s00299-007-0395-7
Lu, D., Wu, S., Gao, X., Zhang, Y., Shan, L., He, P. (2010). A receptor-like cytoplasmic kinase, BIK1, associates with a flagellin receptor complex to initiate plant innate immunity. Proc. Natl. Acad. Sci. U.S.A. 107, 496–501. doi: 10.1073/pnas.0909705107
Lv, X., Li, H., Chen, X., Xiang, X., Guo, Z., Yu, J., et al. (2018). The role of calcium-dependent protein kinase in hydrogen peroxide, nitric oxide and ABA-dependent cold acclimation. J. Exp. Bot. 69, 4127–4139. doi: 10.1093/jxb/ery212
Mahmood, K., El-Kereamy, A., Kim, S. H., Nambara, E., Rothstein, S. J. (2016). ANAC032 positively regulates age-dependent and stress-induced senescence in arabidopsis thaliana. Plant Cell Physiol. 57, 2029–2046. doi: 10.1093/pcp/pcw120
Ma, Y., Szostkiewicz, I., Korte, A., Moes, D., Yang, Y., Christmann, A., et al. (2009). Regulators of PP2C phosphatase activity function as abscisic acid sensors. Science 324, 1064–1068. doi: 10.1126/science.1172408
Matysik, J., Alia, Bhalu, B., Mohanty, P. (2002). Molecular mechanisms of quenching of reactive oxygen species by proline under stress in plants. Curr. Sci. 82, 525–532.
Ma, L., Zhang, H., Sun, L., Jiao, Y., Zhang, G., Miao, C., et al. (2012). NADPH oxidase AtrbohD and AtrbohF function in ROS-dependent regulation of Na+/K+ homeostasis in arabidopsis under salt stress. J. Exp. Bot. 63, 305–317. doi: 10.1093/jxb/err280
Melotto, M., Underwood, W., Koczan, J., Nomura, K., He, S. Y. (2006). Plant stomata function in innate immunity against bacterial invasion. Cell 126, 969–980. doi: 10.1016/j.cell.2006.06.054
Mersmann, S., Bourdais, G., Rietz, S., Robatzek, S. (2010). Ethylene signaling regulates accumulation of the FLS2 receptor and is required for the oxidative burst contributing to plant immunity. Plant Physiol. 154, 391–400. doi: 10.1104/pp.110.154567
Miao, Y., Lv, D., Wang, P., Wang, X. C., Chen, J., Miao, C., et al. (2006). An arabidopsis glutathione peroxidase functions as both a redox transducer and a scavenger in abscisic acid and drought stress responses. Plant Cell 18, 2749–2766. doi: 10.1105/tpc.106.044230
Mittler, R. (2002). Oxidative stress, antioxidants and stress tolerance. Trends Plant Sci. 7, 405–410. doi: 10.1016/S1360-1385(02)02312-9
Mittler, R., Blumwald, E. (2015). The roles of ROS and ABA in systemic acquired acclimation. Plant Cell 27, 64–70. doi: 10.1105/tpc.114.133090
Mittler, R., Vanderauwera, S., Suzuki, N., Miller, G., Tognetti, V. B., Vandepoele, K., et al. (2011). ROS signaling: the new wave? Trends Plant Sci. 16, 300–309. doi: 10.1016/j.tplants.2011.03.007
Mittler, R., Zandalinas, S. I., Fichman, Y., Van Breusegem, F. (2022). Reactive oxygen species signalling in plant stress responses. Nat. Rev. Mol. Cell Biol. 23, 1–17. doi: 10.1038/s41580-022-00499-2
Mohr, P. G., Cahill, D. M. (2007). Suppression by ABA of salicylic acid and lignin accumulation and the expression of multiple genes, in arabidopsis infected with pseudomonas syringae pv. tomato. Funct. Integr. Genomics 7, 181–191. doi: 10.1007/s10142-006-0041-4
Monaghan, J., Matschi, S., Romeis, T., Zipfel, C. (2015). The calcium-dependent protein kinase CPK28 negatively regulates the BIK1-mediated PAMP-induced calcium burst. Plant Signal Behav. 10, e1018497. doi: 10.1080/15592324.2015.1018497
Monroe-Augustus, M., Zolman, B. K., Bartel, B. (2003). IBR5, a dual-specificity phosphatase-like protein modulating auxin and abscisic acid responsiveness in arabidopsis. Plant Cell 15, 2979–2991. doi: 10.1105/tpc.017046
Muhlemann, J. K., Younts, T. L. B., Muday, G. K. (2018). Flavonols control pollen tube growth and integrity by regulating ROS homeostasis during high-temperature stress. Proc. Natl. Acad. Sci. U.S.A. 115, E11188–E11197. doi: 10.1073/pnas.1811492115
Mustilli, A. C., Merlot, S., Vavasseur, A., Fenzi, F., Giraudat, J. (2002). Arabidopsis OST1 protein kinase mediates the regulation of stomatal aperture by abscisic acid and acts upstream of reactive oxygen species production. Plant Cell 14, 3089–3099. doi: 10.1105/tpc.007906
Nambara, E., Marion-Poll, A. (2005). Abscisic acid biosynthesis and catabolism. Annu. Rev. Plant Biol. 56, 165–185. doi: 10.1146/annurev.arplant.56.032604.144046
Nam, K. H., Li, J. (2002). BRI1/BAK1, a receptor kinase pair mediating brassinosteroid signaling. Cell 110, 203–212. doi: 10.1016/S0092-8674(02)00814-0
Neill, S. (2002). Hydrogen peroxide signalling. Curr. Opin. Plant Biol. 5, 388–395. doi: 10.1016/S1369-5266(02)00282-0
Ni, L., Fu, X., Zhang, H., Li, X., Cai, X., Zhang, P., et al. (2019). Abscisic acid inhibits rice protein phosphatase PP45 via H2O2 and relieves repression of the Ca2+/CaM-dependent protein kinase DMI3. Plant Cell 31, 128–152. doi: 10.1105/tpc.18.00506
Nühse, T. S., Bottrill, A. R., Jones, A. M. E., Peck, S. C. (2007). Quantitative phosphoproteomic analysis of plasma membrane proteins reveals regulatory mechanisms of plant innate immune responses: Quantitative phosphoproteomics for signalling pathways. Plant J. 51, 931–940. doi: 10.1111/j.1365-313X.2007.03192.x
Ogasawara, Y., Kaya, H., Hiraoka, G., Yumoto, F., Kimura, S., Kadota, Y., et al. (2008). Synergistic activation of the arabidopsis NADPH oxidase AtrbohD by Ca2+ and phosphorylation. J. Biol. Chem. 283, 8885–8892. doi: 10.1074/jbc.M708106200
Oide, S., Bejai, S., Staal, J., Guan, N., Kaliff, M., Dixelius, C. (2013). Novel role of PR2 in abscisic acid (ABA) mediated, pathogen-induced callose deposition in arabidopsis thaliana. New Phytol. 200, 1187–1199. doi: 10.1111/nph.12436
Osakabe, Y., Yamaguchi-Shinozaki, K., Shinozaki, K., Tran, L. P. (2014). ABA control of plant macroelement membrane transport systems in response to water deficit and high salinity. New Phytol. 202, 35–49. doi: 10.1111/nph.12613
Overmyer, K., Brosché, M., Kangasjärvi, J. (2003). Reactive oxygen species and hormonal control of cell death. Trends Plant Sci. 8, 335–342. doi: 10.1016/S1360-1385(03)00135-3
Overmyer, K., Tuominen, H., Kettunen, R., Betz, C., Langebartels, C., Sandermann, H., et al. (2000). Ozone-sensitive arabidopsis rcd1 mutant reveals opposite roles for ethylene and jasmonate signaling pathways in regulating superoxide-dependent cell death. Plant Cell 12, 1849–1862. doi: 10.2307/3871197
Paik, I., Kathare, P. K., Kim, J. I., Huq, E. (2017). Expanding roles of PIFs in signal integration from multiple processes. Mol. Plant 10, 1035–1046. doi: 10.1016/j.molp.2017.07.002
Pan, W., Lin, B., Yang, X., Liu, L., Xia, R., Li, J., et al. (2020). The UBC27–AIRP3 ubiquitination complex modulates ABA signaling by promoting the degradation of ABI1 in arabidopsis. Proc. Natl. Acad. Sci. U.S.A. 117, 27694–27702. doi: 10.1073/pnas.2007366117
Park, S. Y., Fung, P., Nishimura, N., Jensen, D. R., Fujii, H., Zhao, Y., et al. (2009). Abscisic acid inhibits type 2C protein phosphatases via the PYR/PYL family of START proteins. Science 324, 1068–1071. doi: 10.1126/science.1173041
Pastori, G. M., Foyer, C. H. (2002). Common components, networks, and pathways of cross-tolerance to stress. the central role of “redox” and abscisic acid-mediated controls. Plant Physiol. 129, 460–468. doi: 10.1104/pp.011021
Pei, Z. M., Murata, Y., Benning, G., Thomine, S., Klüsener, B., Allen, G. J., et al. (2000). Calcium channels activated by hydrogen peroxide mediate abscisic acidsignalling in guard cells. Nature 406, 731–734. doi: 10.1038/35021067
Peng, J., Li, Z., Wen, X., Li, W., Shi, H., Yang, L., et al. (2014). Salt-induced stabilization of EIN3/EIL1 confers salinity tolerance by deterring ROS accumulation in arabidopsis. PloS Genet. 10, e1004664. doi: 10.1371/journal.pgen.1004664
Qi, L., Liu, S., Li, C., Fu, J., Jing, Y., Cheng, J., et al. (2020). PHYTOCHROME-INTERACTING FACTORS interact with the ABA receptors PYL8 and PYL9 to orchestrate ABA signaling in darkness. Mol. Plant 13, 414–430. doi: 10.1016/j.molp.2020.02.001
Qi, J., Wang, J., Gong, Z., Zhou, J. M. (2017). Apoplastic ROS signaling in plant immunity. Curr. Opin. Plant Biol. 38, 92–100. doi: 10.1016/j.pbi.2017.04.022
Raghavendra, A. S., Gonugunta, V. K., Christmann, A., Grill, E. (2010). ABA perception and signalling. Trends Plant Sci. 15, 395–401. doi: 10.1016/j.tplants.2010.04.006
Ramirez-Parra, E., Perianez-Rodriguez, J., Navarro-Neila, S., Gude, I., Moreno-Risueno, M. A., del Pozo, J. C. (2017). The transcription factor OBP4 controls root growth and promotes callus formation. New Phytol. 213, 1787–1801. doi: 10.1111/nph.14315
Ranf, S., Eschen-Lippold, L., Fröhlich, K., Westphal, L., Scheel, D., Lee, J. (2014). Microbe-associated molecular pattern-induced calcium signaling requires the receptor-like cytoplasmic kinases, PBL1 and BIK1. BMC Plant Biol. 14, 1–15. doi: 10.1186/s12870-014-0374-4
Robert-Seilaniantz, A., Navarro, L., Bari, R., Jones, J. D. (2007). Pathological hormone imbalances. Curr. Opin. Plant Biol. 10, 372–379. doi: 10.1016/j.pbi.2007.06.003
Rossel, J. B., Walter, P. B., Hendrickson, L., Chow, W. S., Poole, A., Mullineaux, P. M., et al. (2006). A mutation affecting ASCORBATE PEROXIDASE 2 gene expression reveals a link between responses to high light and drought tolerance. Plant Cell Environ. 29, 269–281. doi: 10.1111/j.1365-3040.2005.01419.x
Rubio, S., Noriega, X., Pérez, F. J. (2019). Abscisic acid (ABA) and low temperatures synergistically increase the expression of CBF/DREB1 transcription factors and cold-hardiness in grapevine dormant buds. Ann. Bot. 123, 681–689. doi: 10.1093/aob/mcy201
Russinova, E., Borst, J. W., Kwaaitaal, M., Caño-Delgado, A., Yin, Y., Chory, J., et al. (2004). Heterodimerization and endocytosis of arabidopsis brassinosteroid receptors BRI1 and AtSERK3 (BAK1). Plant Cell 16, 3216–3229. doi: 10.1105/tpc.104.025387
Sahu, M., Kar, R. K. (2018). Possible interaction of ROS, antioxidants and ABA to survive osmotic stress upon acclimation in vigna radiata l. wilczek seedlings. Plant Physiol. Biochem. 132, 415–423. doi: 10.1016/j.plaphy.2018.09.034
Sanchez-Fernandez, R., Fricker, M., Corben, L. B., White, N. S., Sheard, N., Leaver, C. J., et al. (1997). Cell proliferation and hair tip growth in the arabidopsis root are under mechanistically different forms of redox control. Proc. Natl. Acad. Sci. U.S.A. 94, 2745–2750. doi: 10.1073/pnas.94.6.2745
Santiago, J., Dupeux, F., Round, A., Antoni, R., Park, S. Y., Jamin, M., et al. (2009). The abscisic acid receptor PYR1 in complex with abscisic acid. Nature 462, 665–668. doi: 10.1038/nature08591
Sato, A., Sato, Y., Fukao, Y., Fujiwara, M., Umezawa, T., Shinozaki, K., et al. (2009). Threonine at position 306 of the KAT1 potassium channel is essential for channel activity and is a target site for ABA-activated SnRK2/OST1/SnRK2.6 protein kinase. Biochem. J. 424, 439–448. doi: 10.1042/BJ20091221
Schroeder, J. I., Hagiwara, S. (1990). Repetitive increases in cytosolic Ca2+ of guard cells by abscisic acid activation of nonselective Ca2+ permeable channels. Proc. Natl. Acad. Sci. U.S.A. 87, 9305–9309. doi: 10.1073/pnas.87.23.9305
Seo, K. I., Lee, J. H., Nezames, C. D., Zhong, S., Song, E., Byun, M. O., et al. (2014). ABD1 is an arabidopsis DCAF substrate receptor for CUL4-DDB1–based E3 ligases that acts as a negative regulator of abscisic acid signaling. Plant Cell 26, 695–711. doi: 10.1105/tpc.113.119974
Shang, Y., Dai, C., Lee, M. M., Kwak, J. M., Nam, K. H. (2016). BRI1-associated receptor kinase 1 regulates guard cell ABA signaling mediated by open stomata 1 in arabidopsis. Mol. Plant 9, 447–460. doi: 10.1016/j.molp.2015.12.014
Sharma, R., Sahoo, A., Devendran, R., Jain, M. (2014). Over-expression of a rice tau class glutathione s-transferase gene improves tolerance to salinity and oxidative stresses in arabidopsis. PloS One 9, e92900. doi: 10.1371/journal.pone.0092900
Shi, Y., Wang, Z., Meng, P., Tian, S., Zhang, X., Yang, S. (2013). The glutamate carboxypeptidase AMP1 mediates abscisic acid and abiotic stress responses in arabidopsis. New Phytol. 199, 135–150. doi: 10.1111/nph.12275
Shu, K., Zhou, W., Chen, F., Luo, X., Yang, W. (2018). Abscisic acid and gibberellins antagonistically mediate plant development and abiotic stress responses. Front. Plant Sci. 9. doi: 10.3389/fpls.2018.00416
Silva, N. C. Q., de Souza, G. A., Pimenta, T. M., Brito, F. A. L., Picoli, E. A. T., Zsögön, A., et al. (2018). Salt stress inhibits germination of stylosanthes humilis seeds through abscisic acid accumulation and associated changes in ethylene production. Plant Physiol. Biochem. 130, 399–407. doi: 10.1016/j.plaphy.2018.07.025
Simmons, A. R., Bergmann, D. C. (2016). Transcriptional control of cell fate in the stomatal lineage. Curr. Opin. Plant Biol. 29, 1–8. doi: 10.1016/j.pbi.2015.09.008
Sirichandra, C., Gu, D., Hu, H. C., Davanture, M., Lee, S., Djaoui, M., et al. (2009). Phosphorylation of the arabidopsis AtrbohF NADPH oxidase by OST1 protein kinase. FEBS Lett. 583, 2982–2986. doi: 10.1016/j.febslet.2009.08.033
Siripornadulsil, S., Traina, S., Verma, D. P. S., Sayre, R. T. (2002). Molecular mechanisms of proline-mediated tolerance to toxic heavy metals in transgenic microalgae. Plant Cell 14, 2837–2847. doi: 10.1105/tpc.004853
Skubacz, A., Daszkowska-Golec, A., Szarejko, I. (2016). The role and regulation of ABI5 (ABA-insensitive 5) in plant development, abiotic stress responses and phytohormone crosstalk. Front. Plant Sci. 7. doi: 10.3389/fpls.2016.01884
Smalle, J., Kurepa, J., Yang, P., Emborg, T. J., Babiychuk, E., Kushnir, S., et al. (2003). The pleiotropic role of the 26S proteasome subunit RPN10 in arabidopsis growth and development supports a substrate-specific function in abscisic acid signaling. Plant Cell 15, 965–980. doi: 10.1105/tpc.009217
Smirnoff, N., Wheeler, G. L. (2000). Ascorbic acid in plants: biosynthesis and function. Crit. Rev. Biochem. Mol. Biol. 35, 291–314. doi: 10.1080/10409230008984166
Suzuki, N., Katano, K. (2018). Coordination between ROS regulatory systems and other pathways under heat stress and pathogen attack. Front. Plant Sci. 9. doi: 10.3389/fpls.2018.00490
Suzuki, N., Miller, G., Morales, J., Shulaev, V., Torres, M. A., Mittler, R. (2011). Respiratory burst oxidases: the engines of ROS signaling. Curr. Opin. Plant Biol. 14, 691–699. doi: 10.1016/j.pbi.2011.07.014
Suzuki, N., Miller, G., Salazar, C., Mondal, H. A., Shulaev, E., Cortes, D. F., et al. (2013). Temporal-spatial interaction between reactive oxygen species and abscisic acid regulates rapid systemic acclimation in plants. Plant Cell 25, 3553–3569. doi: 10.1105/tpc.113.114595
Szabados, L., Savouré, A. (2010). Proline: a multifunctional amino acid. Trends Plant Sci. 15, 89–97. doi: 10.1016/j.tplants.2009.11.009
Székely, G., Abrahám, E., Cséplo, A., Rigó, G., Zsigmond, L., Csiszár, J., et al. (2008). Duplicated P5CS genes of arabidopsis play distinct roles in stress regulation and developmental control of proline biosynthesis. Plant J. 53, 11–28. doi: 10.1111/j.1365-313X.2007.03318.x
Tintor, N., Ross, A., Kanehara, K., Yamada, K., Fan, L., Kemmerling, B., et al. (2013). Layered pattern receptor signaling via ethylene and endogenous elicitor peptides during arabidopsis immunity to bacterial infection. Proc. Natl. Acad. Sci. U.S.A. 110, 6211–6216. doi: 10.1073/pnas.1216780110
Tiryaki, I., Staswick, P. E. (2002). An arabidopsis mutant defective in jasmonate response is allelic to the auxin-signaling mutant axr1. Plant Physiol. 130, 887–894. doi: 10.1104/pp.005272
Tognetti, V. B., Bielach, A., Hrtyan, M. (2017). Redox regulation at the site of primary growth: auxin, cytokinin and ROS crosstalk: Apical meristems plasticity in response to stress. Plant Cell Environ. 40, 2586–2605. doi: 10.1111/pce.13021
Ton, J., Flors, V., Mauch-Mani, B. (2009). The multifaceted role of ABA in disease resistance. Trends Plant Sci. 14, 310–317. doi: 10.1016/j.tplants.2009.03.006
Torres, M. A., Dangl, J. L., Jones, J. D. G. (2002). Arabidopsis gp91phox homologues AtrbohD and AtrbohF are required for accumulation of reactive oxygen intermediates in the plant defense response. PNAS 99, 517–522. doi: 10.1073/pnas.012452499
Tsukagoshi, H., Busch, W., Benfey, P. N. (2010). Transcriptional regulation of ROS controls transition from proliferation to differentiation in the root. Cell 143, 606–616. doi: 10.1016/j.cell.2010.10.020
Umezawa, T., Nakashima, K., Miyakawa, T., Kuromori, T., Tanokura, M., Shinozaki, K., et al. (2010). Molecular basis of the core regulatory network in ABA responses: sensing, signaling and transport. Plant Cell Physiol. 51, 1821–1839. doi: 10.1093/pcp/pcq156
Umezawa, T., Sugiyama, N., Mizoguchi, M., Hayashi, S., Myouga, F., Yamaguchi-Shinozaki, K., et al. (2009). Type 2C protein phosphatases directly regulate abscisic acid-activated protein kinases in arabidopsis. Proc. Natl. Acad. Sci. U.S.A. 106, 17588–17593. doi: 10.1073/pnas.0907095106
Vernoux, T., Wilson, R. C., Seeley, K. A., Reichheld, J. P., Muroy, S., Brown, S., et al. (2000). The root MERISTEMLESS1/CADMIUM SENSITIVE2 gene defines a glutathione-dependent pathway involved in initiation and maintenance of cell division during postembryonic root development. Plant Cell 12, 97–109. doi: 10.1105/tpc.12.1.97
Veronese, P., Nakagami, H., Bluhm, B., AbuQamar, S., Chen, X., Salmeron, J., et al. (2006). The membrane-anchored BOTRYTIS-INDUCED KINASE1 plays distinct roles in arabidopsis resistance to necrotrophic and biotrophic pathogens. Plant Cell 18, 257–273. doi: 10.1105/tpc.105.035576
Verslues, P. E., Kim, Y. S., Zhu, J. K. (2007). Altered ABA, proline and hydrogen peroxide in an arabidopsis glutamate:glyoxylate aminotransferase mutant. Plant Mol. Biol. 64, 205–217. doi: 10.1007/s11103-007-9145-z
Vishwakarma, K., Upadhyay, N., Kumar, N., Yadav, G., Singh, J., Mishra, R. K., et al. (2017). Abscisic acid signaling and abiotic stress tolerance in plants: a review on current knowledge and future prospects. Front. Plant Sci. 8. doi: 10.3389/fpls.2017.00161
Wang, Z., Cao, H., Sun, Y., Li, X., Chen, F., Carles, A., et al. (2013). Arabidopsis paired amphipathic helix proteins SNL1 and SNL2 redundantly regulate primary seed dormancy via abscisic acid–ethylene antagonism mediated by histone deacetylation. Plant Cell 25, 149–166. doi: 10.1105/tpc.112.108191
Wang, X., Goshe, M. B., Soderblom, E. J., Phinney, B. S., Kuchar, J. A., Li, J., et al. (2005). Identification and functional analysis of in vivo phosphorylation sites of the arabidopsis BRASSINOSTEROID-INSENSITIVE1 receptor kinase. Plant Cell 17, 1685–1703. doi: 10.1105/tpc.105.031393
Wang, X., Kota, U., He, K., Blackburn, K., Li, J., Goshe, M. B., et al. (2008). Sequential transphosphorylation of the BRI1/BAK1 receptor kinase complex impacts early events in brassinosteroid signaling. Dev. Cell 15, 220–235. doi: 10.1016/j.devcel.2008.06.011
Wang, F., Liu, J., Chen, M., Zhou, L., Li, Z., Zhao, Q., et al. (2016). Involvement of abscisic acid in PSII photodamage and D1 protein turnover for light-induced premature senescence of rice flag leaves. PloS One 11, e0161203. doi: 10.1371/journal.pone.0161203
Wang, P., Song, C. (2008). Guard-cell signalling for hydrogen peroxide and abscisic acid. New Phytol. 178, 703–718. doi: 10.1111/j.1469-8137.2008.02431.x
White, C. N., Proebsting, W. M., Hedden, P., Rivin, C. J. (2000). Gibberellins and seed development in maize. i. evidence that gibberellin/abscisic acid balance governs germination versus maturation pathways. Plant Physiol. 122, 1081–1088. doi: 10.1104/pp.122.4.1081
Wong, H. L., Pinontoan, R., Hayashi, K., Tabata, R., Yaeno, T., Hasegawa, K., et al. (2008). Regulation of rice NADPH oxidase by binding of rac GTPase to its n-terminal extension. Plant Cell 19, 4022–4034. doi: 10.1105/tpc.107.055624
Wu, F., Chi, Y., Jiang, Z., Xu, Y., Xie, L., Huang, F., et al. (2020). Hydrogen peroxide sensor HPCA1 is an LRR receptor kinase in arabidopsis. Nature 578, 577–581. doi: 10.1038/s41586-020-2032-3
Wu, B., Li, L., Qiu, T., Zhang, X., Cui, S. (2018). Cytosolic APX2 is a pleiotropic protein involved in H2O2 homeostasis, chloroplast protection, plant architecture and fertility maintenance. Plant Cell Rep. 37, 833–848. doi: 10.1007/s00299-018-2272-y
Wu, Q., Zhang, X., Peirats-Llobet, M., Belda-Palazon, B., Wang, X., Cui, S., et al. (2016). Ubiquitin ligases RGLG1 and RGLG5 regulate abscisic acid signaling by controlling the turnover of phosphatase PP2CA. Plant Cell 28, 2178–2196. doi: 10.1105/tpc.16.00364
Xia, X. J., Zhou, Y. H., Shi, K., Zhou, J., Foyer, C. H., Yu, J. Q. (2015). Interplay between reactive oxygen species and hormones in the control of plant development and stress tolerance. J. Exp. Bot. 66, 2839–2856. doi: 10.1093/jxb/erv089
Xie, Y., Mao, Y., Duan, X., Zhou, H., Lai, D., Zhang, Y., et al. (2016). Arabidopsis HY1-modulated stomatal movement: an integrative hub is functionally associated with ABI4 in dehydration-induced ABA responsiveness. Plant Physiol. 170, 1699–1713. doi: 10.1104/pp.15.01550
Xie, H. T., Wan, Z. Y., Li, S., Zhang, Y. (2014). Spatiotemporal production of reactive oxygen species by NADPH oxidase is critical for tapetal programmed cell death and pollen development in arabidopsis. Plant Cell 26, 2007–2023. doi: 10.1105/tpc.114.125427
Xu, N., Chu, Y., Chen, H., Li, X., Wu, Q., Jin, L., et al. (2018). Rice transcription factor OsMADS25 modulates root growth and confers salinity tolerance via the ABA–mediated regulatory pathway and ROS scavenging. PloS Genet. 14, e1007662. doi: 10.1371/journal.pgen.1007662
Xue, L., Sun, M., Wu, Z., Yu, L., Yu, Q., Tang, Y., et al. (2020). LncRNA regulates tomato fruit cracking by coordinating gene expression via a hormone-redox-cell wall network. BMC Plant Biol. 20, 162. doi: 10.1186/s12870-020-02373-9
Xu, Z., Mahmood, K., Rothstein, S. J. (2017b). ROS induces anthocyanin production via late biosynthetic genes and anthocyanin deficiency confers the hypersensitivity to ROS-generating stresses in arabidopsis. Plant Cell Physiol. 58, 1364–1377. doi: 10.1093/pcp/pcx073
Xu, L., Zhao, H., Ruan, W., Deng, M., Wang, F., Peng, J., et al. (2017a). ABNORMAL INFLORESCENCE MERISTEM1 functions in salicylic acid biosynthesis to maintain proper reactive oxygen species levels for root meristem activity in rice. Plant Cell 29, 560–574. doi: 10.1105/tpc.16.00665
Yang, D. L., Yao, J., Mei, C. S., Tong, X. H., Zeng, L. J., Li, Q., et al. (2012). Plant hormone jasmonate prioritizes defense over growth by interfering with gibberellin signaling cascade. Proc. Natl. Acad. Sci. U.S.A. 109, E1192–E1200. doi: 10.1073/pnas.1201616109
Yang, L., Zhang, J., He, J., Qin, Y., Hua, D., Duan, Y., et al. (2014). ABA-mediated ROS in mitochondria regulate root meristem activity by controlling PLETHORA expression in arabidopsis. PloS Genet. 10, e1004791. doi: 10.1371/journal.pgen.1004791
Yan, H., Li, Q., Park, S. C., Wang, X., Liu, Y., Zhang, Y., et al. (2016). Overexpression of CuZnSOD and APX enhance salt stress tolerance in sweet potato. Plant Physiol. Biochem. 109, 20–27. doi: 10.1016/j.plaphy.2016.09.003
Ye, N., Zhu, G., Liu, Y., Zhang, A., Li, Y., Liu, R., et al. (2012). Ascorbic acid and reactive oxygen species are involved in the inhibition of seed germination by abscisic acid in rice seeds. J. Exp. Bot. 63, 1809–1822. doi: 10.1093/jxb/err336
Yin, C. C., Ma, B., Collinge, D. P., Pogson, B. J., He, S. J., Xiong, Q., et al. (2015). Ethylene responses in rice roots and coleoptiles are differentially regulated by a carotenoid isomerase-mediated abscisic acid pathway. Plant Cell 27, 1061–1081. doi: 10.1105/tpc.15.00080
Yin, C. C., Zhao, H., Ma, B., Chen, S. Y., Zhang, J. S. (2017). Diverse roles of ethylene in regulating agronomic traits in rice. Front. Plant Sci. 8. doi: 10.3389/fpls.2017.01676
Yoshida, T., Mogami, J., Yamaguchi-Shinozaki, K. (2014). ABA-dependent and ABA-independent signaling in response to osmotic stress in plants. Curr. Opin. Plant Biol. 21, 133–139. doi: 10.1016/j.pbi.2014.07.009
Yoshida, T., Nishimura, N., Kitahata, N., Kuromori, T., Ito, T., Asami, T., et al. (2006). ABA-hypersensitive germination3 encodes a protein phosphatase 2C (AtPP2CA) that strongly regulates abscisic acid signaling during germination among arabidopsis protein phosphatase 2Cs. Plant Physiol. 140, 115–126. doi: 10.1104/pp.105.070128
You, J., Zong, W., Hu, H., Li, X., Xiao, J., Xiong, L. (2014). A STRESS-RESPONSIVE NAC1-regulated protein phosphatase gene rice protein phosphatase18 modulates drought and oxidative stress tolerance through abscisic acid-independent reactive oxygen species scavenging in rice. Plant Physiol. 166, 2100–2114. doi: 10.1104/pp.114.251116
Yu, Q., Tian, H., Yue, K., Liu, J., Zhang, B., Li, X., et al. (2016). A p-loop NTPase regulates quiescent center cell division and distal stem cell identity through the regulation of ROS homeostasis in arabidopsis root. PloS Genet. 12, e1006175. doi: 10.1371/journal.pgen.1006175
Yu, Y., Wang, J., Li, S., Kakan, X., Zhou, Y., Miao, Y., et al. (2019). Ascorbic acid integrates the antagonistic modulation of ethylene and abscisic acid in the accumulation of reactive oxygen species. Plant Physiol. 179, 1861–1875. doi: 10.1104/pp.18.01250
Yu, F., Wu, Y., Xie, Q. (2015). Precise protein post-translational modifications modulate ABI5 activity. Trends Plant Sci. 20, 569–575. doi: 10.1016/j.tplants.2015.05.004
Zandalinas, S. I., Fichman, Y., Mittler, R. (2020). Vascular bundles mediate systemic reactive oxygen signaling during light stress. Plant Cell 32, 3425–3435. doi: 10.1105/tpc.20.00453
Zeng, J., Dong, Z., Wu, H., Tian, Z., Zhao, Z. (2017). Redox regulation of plant stem cell fate. EMBO J. 36, 2844–2855. doi: 10.15252/embj.201695955
Zhang, C., Bousquet, A., Harris, J. M. (2014). Abscisic acid and lateral root organ defective/numerous infections and polyphenolics modulate root elongation via reactive oxygen species in medicago truncatula. Plant Physiol. 166, 644–658. doi: 10.1104/pp.114.248542
Zhang, H., Cui, F., Wu, Y., Lou, L., Liu, L., Tian, M., et al. (2015). The RING finger ubiquitin E3 ligase SDIR1 targets SDIR1-INTERACTING PROTEIN1 for degradation to modulate the salt stress response and ABA signaling in arabidopsis. Plant Cell 27, 214–227. doi: 10.1105/tpc.114.134163
Zhang, J., Li, W., Xiang, T., Liu, Z., Laluk, K., Ding, X., et al. (2010). Receptor-like cytoplasmic kinases integrate signaling from multiple plant immune receptors and are targeted by a pseudomonas syringae effector. Cell Host Microbe 7, 290–301. doi: 10.1016/j.chom.2010.03.007
Zhang, T., Li, Z., Zhao, Y., Shen, W., Chen, M., Gao, H., et al. (2021). Ethylene-induced stomatal closure is mediated via MKK1/3–MPK3/6 cascade to EIN2 and EIN3. J. Integr. Plant Biol. 63, 1324–1340. doi: 10.1111/jipb.13083
Zhang, W., Qin, C., Zhao, J., Wang, X. (2004). Phospholipase d alpha 1-derived phosphatidic acid interacts with ABI1 phosphatase 2C and regulates abscisic acid signaling. Proc. Natl. Acad. Sci. U.S.A. 101, 9508–9513. doi: 10.1073/pnas.0402112101
Zhang, J., Shao, F., Li, Y., Cui, H., Chen, L., Li, H., et al. (2007a). A pseudomonas syringae effector inactivates MAPKs to suppress PAMP-induced immunity in plants. Cell Host Microbe 1, 175–185. doi: 10.1016/j.chom.2007.03.006
Zhang, Y., Yang, C., Li, Y., Zheng, N., Chen, H., Zhao, Q., et al. (2007b). SDIR1 is a RING finger E3 ligase that positively regulates stress-responsive abscisic acid signaling in arabidopsis. Plant Cell 19, 1912–1929. doi: 10.1105/tpc.106.048488
Zhang, Y., Zhu, H., Zhang, Q., Li, M., Yan, M., Wang, R., et al. (2009). Phospholipase Dα1 and phosphatidic acid regulate NADPH oxidase activity and production of reactive oxygen species in ABA-mediated stomatal closure in arabidopsis. Plant Cell 21, 2357–2377. doi: 10.1105/tpc.108.062992
Keywords: ABA, ROS, plant growth, resistances, signal regulation
Citation: Li S, Liu S, Zhang Q, Cui M, Zhao M, Li N, Wang S, Wu R, Zhang L, Cao Y and Wang L (2022) The interaction of ABA and ROS in plant growth and stress resistances. Front. Plant Sci. 13:1050132. doi: 10.3389/fpls.2022.1050132
Received: 21 September 2022; Accepted: 08 November 2022;
Published: 24 November 2022.
Edited by:
Ben Zhang, Shanxi University, ChinaReviewed by:
Yanwen Yu, Henan Agricultural University, ChinaHuapeng Zhou, Sichuan University, China
Liwei Zheng, Zhengzhou University, China
Copyright © 2022 Li, Liu, Zhang, Cui, Zhao, Li, Wang, Wu, Zhang, Cao and Wang. This is an open-access article distributed under the terms of the Creative Commons Attribution License (CC BY). The use, distribution or reproduction in other forums is permitted, provided the original author(s) and the copyright owner(s) are credited and that the original publication in this journal is cited, in accordance with accepted academic practice. No use, distribution or reproduction is permitted which does not comply with these terms.
*Correspondence: Lihu Wang, wanglihu@hebeu.edu.cn
†These authors have contributed equally to this work