- 1WHO Collaborating Centre for Gonorrhoea and Other STIs, National Reference Laboratory for Sexually Transmitted Infections, Department of Laboratory Medicine, Faculty of Medicine and Health, Örebro University, Örebro, Sweden
- 2Division of Clinical Chemistry, Department of Laboratory Medicine, Faculty of Medicine and Health, Örebro University, Örebro, Sweden
- 3Centre for Epidemiology and Biostatistics, Melbourne School of Population and Global Health, University of Melbourne, Melbourne, VIC, Australia
- 4Global Antibiotic Research and Development Partnership (GARDP), Geneva, Switzerland
- 5College of Medicine, Institute for Therapeutic Innovation, University of Florida, Orlando, FL, United States
- 6Institute for Global Health, University College London (UCL), London, United Kingdom
Antimicrobial resistance in the sexually transmitted bacterium Neisseria gonorrhoeae is compromising the management and control of gonorrhea globally. Optimized use and enhanced stewardship of current antimicrobials and development of novel antimicrobials are imperative. The first in class zoliflodacin (spiropyrimidinetrione, DNA Gyrase B inhibitor) is a promising novel antimicrobial in late-stage clinical development for gonorrhea treatment, i.e., the phase III randomized controlled clinical trial (ClinicalTrials.gov Identifier: NCT03959527) was recently finalized, and zoliflodacin showed non-inferiority compared to the recommended ceftriaxone plus azithromycin dual therapy. Doxycycline, the first-line treatment for chlamydia and empiric treatment for non-gonococcal urethritis, will be frequently given together with zoliflodacin because gonorrhea and chlamydia coinfections are common. In a previous static in vitro study, it was indicated that doxycycline/tetracycline inhibited the gonococcal killing of zoliflodacin in 6-h time-kill curve analysis. In this study, our dynamic in vitro hollow-fiber infection model (HFIM) was used to investigate combination therapies with zoliflodacin and doxycycline. Dose–range experiments using the three gonococcal strains WHO F (susceptible to relevant therapeutic antimicrobials), WHO X (extensively drug-resistant, including ceftriaxone-resistant; zoliflodacin-susceptible), and SE600/18 (zoliflodacin-susceptible strain with GyrB S467N substitution) were conducted simulating combination therapy with a single oral dose of zoliflodacin 0.5–4 g combined with a doxycycline daily oral dose of 200 mg administered as 100 mg twice a day, for 7 days (standard dose for chlamydia treatment). Comparing combination therapy of zoliflodacin (0.5–4 g single dose) plus doxycycline (200 mg divided into 100 mg twice a day orally, for 7 days) to zoliflodacin monotherapy (0.5–4 g single dose) showed that combination therapy was slightly more effective than monotherapy in the killing of N. gonorrhoeae and suppressing emergence of zoliflodacin resistance. Accordingly, WHO F was eradicated by only 0.5 g single dose of zoliflodacin in combination with doxycycline, and WHO X and SE600/18 were both eradicated by a 2 g single dose of zoliflodacin in combination with doxycycline; no zoliflodacin-resistant populations occurred during the 7-day experiment when using this zoliflodacin dose. When using suboptimal (0.5–1 g) zoliflodacin doses together with doxycycline, gonococcal mutants with increased zoliflodacin MICs, due to GyrB D429N and the novel GyrB T472P, emerged, but both the mutants had an impaired biofitness. The present study shows the high efficacy of zoliflodacin plus doxycycline combination therapy using a dynamic HFIM that more accurately and comprehensively simulate gonococcal infection and their treatment, i.e., compared to static in vitro models, such as short-time checkerboard experiments or time-kill curve analysis. Based on our dynamic in vitro HFIM work, zoliflodacin plus doxycycline for the treatment of both gonorrhea and chlamydia can be an effective combination.
Introduction
Treatment and control of gonorrhea is threatened by increasing antimicrobial resistance (AMR) in Neisseria gonorrhoeae. Resistance has evolved to all previously used classes of antibiotics recommended for treatment. During the recent decade, in vitro and clinical resistance to the last-line options for empirical treatment, ceftriaxone and particularly azithromycin, has begun to spread (Unemo and Shafer, 2014; Unemo et al., 2019; Unemo et al., 2020; Workowski et al., 2021; Day et al., 2022; Sánchez-Busó et al., 2022). Furthermore, the first treatment failure with dual-therapy, ceftriaxone and azithromycin, was reported in 2016 (Fifer et al., 2016), and the international spread of the ceftriaxone-resistant FC428 clone has been verified since 2015 (Nakayama et al., 2016; Golparian et al., 2018; Lahra et al., 2018; Poncin et al., 2019; Zhou et al., 2020; Day et al., 2022; Lin et al., 2022). Furthermore, the first strains with resistance to ceftriaxone combined with high-level azithromycin resistance were identified in 2018 (Whiley et al., 2018; Jennison et al., 2019) and 2022 (Golparian et al., 2022; Pleininger et al., 2022). To mitigate the risk that gonorrhea becomes untreatable, which might become a reality for many bacterial infections (Algammal et al., 2023), global surveillance of the spread and evolution of AMR needs to be improved. Additionally, an enhanced understanding of the pharmacokinetics (PK) and pharmacodynamics (PD) for the optimization of current treatments and the use of molecular assays for resistance/susceptibility-guided therapy are necessary (WHO, 2012; Seña et al., 2020). However, new treatment options will become essential, which has been powerfully stressed in the WHO Global Action Plan: To Control the Spread and Impact of Antimicrobial Resistance in N. gonorrhoeae (WHO, 2012) and in the corresponding response plans from ECDC and CDC (CDC, 2012; ECDC, 2019). However, only two novel antimicrobials, zoliflodacin (Taylor et al., 2018a) and gepotidacin (Taylor et al., 2018b), have reached a later clinical stage of development for uncomplicated gonorrhea treatment.
Zoliflodacin is a novel spiropyrimidinetrione inhibiting bacterial DNA synthesis through the inhibition of topoisomerase type IIA with a unique mode of binding to GyrB (Basarab et al., 2015; Kern et al., 2015). Evaluation of zoliflodacin as a novel treatment for uncomplicated gonorrhea was recently finalized in a global phase III randomized controlled clinical trial (RCT) (ClinicalTrials.gov Identifier: NCT03959527). In this phase III RCT, zoliflodacin 3 g single oral dose met the pre-specified statistical test for non-inferiority when compared to the internationally recommended treatment including ceftriaxone 500 mg single intramuscular dose plus azithromycin 1 g single oral dose (5.31%, 95% confidence interval 1.38%, 8.65%). Non-inferiority of zoliflodacin was demonstrated within the pre-specified 12% margin and, additionally, within the 10% margin specified by the United States Food and Drug Administration (https://gardp.org/positive-results-announced-in-largest-pivotal-phase-3-trial-of-a-first-in-class-oral-antibiotic-to-treat-uncomplicated-gonorrhoea/). Zoliflodacin previously showed promising results in a phase II RCT (Taylor et al., 2018a), and additionally, the PD of zoliflodacin has been evaluated in a dynamic hollow-fiber infection model (HFIM) to identify the optimal zoliflodacin dosing, i.e., for ideal killing of N. gonorrhoeae and to suppress the selection of zoliflodacin resistance during treatment (Jacobsson et al., 2021; Jacobsson et al., 2022a). These HFIM studies showed that a ≥3 g single oral dose of zoliflodacin is required to successfully eradicate N. gonorrhoeae strains harboring pre-existing GyrB mutations that are predisposed to the development of zoliflodacin resistance (Jacobsson et al., 2021; Jacobsson et al., 2022a).
Doxycycline is a second-generation tetracycline, which is bacteriostatic through disruption of bacterial protein synthesis by binding to the 30S ribosomal subunit (Suzuka et al., 1966). Doxycycline is the first-line treatment for chlamydia, a first-line option for empiric treatment of non-gonococcal urethritis and cervicitis, used for initial empiric treatment in resistance-guided sequential treatment for Mycoplasma genitalium, and an alternative regimen for the treatment of syphilis, especially for patients with penicillin hypersensitivity (Lanjouw et al., 2016; Janier et al., 2021; Workowski et al., 2021; Jensen et al., 2022). Furthermore, two recent studies evaluating doxycycline post-exposure prophylaxis (PEP), i.e., after a potential exposure to a sexually transmitted infection (STI), showed promising results by decreasing the incidences of STIs (especially syphilis and chlamydia) (Molina et al., 2018; Luetkemeyer et al., 2022). Subsequently, the San Francisco Public Health Unit has started to offer doxycycline PEP to men who have sex with men (MSM) and trans women at a higher risk for STIs (San Francisco Department of Public Health, 2022). Consequently, if zoliflodacin is approved for the treatment of gonorrhea, it is likely that zoliflodacin will frequently be given together with doxycycline, i.e., because these other bacterial STIs are frequent coinfections to gonorrhea (or may not have been excluded), and the use of doxycycline PEP (Molina et al., 2018; Luetkemeyer et al., 2022) might quickly expand. It is crucial that doxycycline will then not inhibit the N. gonorrhoeae killing of zoliflodacin. It is a concern that, in a previous in vitro study, doxycycline/tetracycline significantly decreased zoliflodacin bactericidal activity (kill rate) in a 6-h time-kill curve analysis (Foerster et al., 2019). However, it was also stated as important to appropriately and quality assuredly evaluate the potential interactions between zoliflodacin and doxycycline/tetracycline in a dynamic HFIM (Foerster et al., 2019).
The objectives of this study were to evaluate different combination therapies with zoliflodacin (0.5–4 g in single oral doses) plus doxycycline (100 mg twice a day, for 7 days) against N. gonorrhoeae in our dynamic HFIM and compare with the previous results using zoliflodacin monotherapy (0.5–8 g) in HFIM (Jacobsson et al., 2021; Jacobsson et al., 2022a) for killing of N. gonorrhoeae and suppression of emergence of zoliflodacin resistance. The biofitness of the selected mutant WHO X-T472P, including the novel GyrB T472P substitution that increased zoliflodacin MIC, compared to its zoliflodacin-susceptible parental strain (WHO X) was also evaluated in HFIM.
Material and methods
Bacterial strains
The N. gonorrhoeae international reference strains WHO F (susceptible to relevant therapeutical antimicrobials) and WHO X (extensively drug-resistant, including resistance to ceftriaxone and other extended-spectrum cephalosporins and fluoroquinolones; zoliflodacin-susceptible) (Unemo et al., 2016), and the clinical N. gonorrhoeae strain SE600/18 (zoliflodacin-susceptible with GyrB S467N substitution, cultured in Sweden in 2018) (Jacobsson et al., 2022a) (Table 1) were examined.
Antimicrobial susceptibility testing
Zoliflodacin MICs (mg/L) were determined by agar dilution, according to Clinical and Laboratory Standards Institute (CLSI) guidelines (M07-A9 and M100-S24; www.clsi.org), using GCVIT agar plates [3.6% Difco GC Medium Base agar (BD, Diagnostics, Sparks, MD, United States) supplemented with 1% IsoVitaleX (BD, Diagnostics)] and microbroth dilution [in triplicates in the medium used in the HFIM medium, i.e., modified Fastidious Broth (mFB)], as previously reported (Jacobsson et al., 2021; Jacobsson et al., 2022a). MICs (mg/L) of doxycycline, tetracycline, ceftriaxone, cefixime, azithromycin, and ciprofloxacin were determined with ETEST, in concordance with the instructions of the manufacturer (bioMérieux, Marcy-l’Etoile, France).
Hollow-fiber infection model
N. gonorrhoeae infections and PK/PD of therapeutic antimicrobials against N. gonorrhoeae were simulated using our dynamic HFIM with cellulosic cartridges (FiberCell Systems Inc., Frederick, MD, United States) (Jacobsson et al., 2021; Jacobsson et al., 2022a; Jacobsson et al., 2022b). A schematic drawing summarizing our HFIM can be seen in Supplementary Figure S1, and for more details regarding HFIM and two compartment models, see Cadwell (2012).
Briefly, zoliflodacin was co-administered with doxycycline to HFIM using syringe pumps, and peristaltic pumps isovolumetrically replaced the antibiotic-containing broth medium with the antibiotic-free medium to simulate different half-lives (t1/2) in the plasma of the two antibiotics and free (protein-unbound fraction) concentration-time profiles. Sampling for quantitative cultures (colony-forming units (CFUs)/mL) for total gonococcal burden and possible zoliflodacin-resistant and/or doxycycline-resistant gonococcal population, and the measurement of zoliflodacin and doxycycline concentrations were performed over 7 days. On the first day, 0.5 mL of gonococcal cultures (18–24 h) from GCAGP agar plates (3.6% Difco GC Medium Base agar (BD, Diagnostics) supplemented with 1% hemoglobin (BD, Diagnostics), 1% IsoVitaleX (BD, Diagnostics), and 10% horse serum) were inoculated in 49.5 mL of mFB and incubated at 36°C in a humidified 5% CO2-enriched atmosphere to a mid-log phase. A measure of 10 mL (∼105–106 CFUs/mL) of the gonococcal suspension was then inoculated into each HFIM cartridge to mimic a clinically relevant gonococcal load (Bissessor et al., 2011; Chow et al., 2016; Priest et al., 2017; Van Der Veer et al., 2020). Zoliflodacin was administered to simulate a PK plasma concentration-time profile in a human adult, following a zoliflodacin single oral dose (PK parameters for zoliflodacin 3 g oral dose were used: 17% fraction of free zoliflodacin in plasma, 6.47 h t1/2, and a 3-h infusion time, and were linearly adjusted for other doses) (O’Donnell et al., 2019), as previously described (Jacobsson et al., 2021; Jacobsson et al., 2022a). Doxycycline was administered to mimic an adult human PK plasma concentration-time profile, following a 100-mg oral dose of doxycycline twice a day for 7 days (PK parameters for doxycycline 100 mg oral dose were used: 15% fraction of free doxycycline in plasma, 17.1 h t1/2, and a 2-h infusion time) (Maesen et al., 1989; Nguyen et al., 1989; Alsarra et al., 2004; Gschwend et al., 2007; Binh et al., 2009). One HFIM cartridge for each investigated strain and experiment was used as a control of untreated growth.
Dose–range experiments (n = 2) were conducted simulating combination therapy with a single oral dose of 0.5, 1, 2, 3, or 4 g of zoliflodacin combined with a daily dose of 200 mg of doxycycline administered as 100 mg given at 0 h and at 12 h (q12 h) for 7 days, i.e., the recommended first-line treatment for Chlamydia trachomatis infections (Lanjouw et al., 2016; Workowski et al., 2021).
Quantification of viable bacterial populations
To determine the N. gonorrhoeae total population and potentially zoliflodacin-resistant subpopulations, bacterial samples (1 mL) were taken from the extra capillary space of each cartridge at time points 2, 3, 6.5, 12, 14, 24, 48, 72, 96, 120, 144, and 168 h. Samples were serially diluted in mFB and plated in a quantitative manner on GCAGP agar plates and GCAGP agar plates containing 2–3×MIC of zoliflodacin, which resulted in a detection limit of ≥100 CFUs per HFIM cartridge, as previously reported (Jacobsson et al., 2021; Jacobsson et al., 2022a). Colony counts (log10 CFUs/mL) were conducted subsequent to incubation for up to 72 h at 36°C in a humidified 5% CO2-enriched atmosphere using an automated colony counter (Scan 4000, INTERSCIENCE, Saint-Nom-la-Bretèche, France).
Biofitness experiments
To evaluate the biofitness of the mutant with increased zoliflodacin MICs selected in HFIM (WHO X-T472P) in comparison to the zoliflodacin-susceptible parental strain (WHO X), competition experiments using the coculture of WHO X-T472P and WHO X were performed in the HFIM, as previously described for the zoliflodacin-resistant mutant selected and its parental strain, i.e., SE600/18-D429N and SE600/18 (Jacobsson et al., 2022a; Jacobsson et al., 2022b). Briefly, gonococcal culture was collected from GCAGP agar plates and diluted in mFB to a quantity of ∼105–106 CFU/mL. Equal volumes (5 mL/strain) of the suspensions of each strain were inoculated into the identical HFIM cartridge. Aliquots (1 mL) were sampled at 24, 48, 72, 96, 120, 144, and 168 h, diluted in mFB in a serial manner and quantitatively plated on GCAGP agar plates and GCAGP agar plates containing 2×MIC of zoliflodacin, as previously reported (Jacobsson et al., 2022a; Jacobsson et al., 2022b). Colony counts (log10 CFU/mL) were conducted subsequent to incubation for up to 72 h at 36°C in a humidified 5% CO2-enriched atmosphere using an automated colony counter (Scan 4000, INTERSCIENCE, Saint-Nom-la-Bretèche, France). The competitive index (CI) was calculated by dividing the ratio of the mutant with increased zoliflodacin MIC, WHO X-T472P, to its zoliflodacin-susceptible parental strain, WHO X, at each time point with the ratio of the mutant to its wild-type parent strain in the initial inoculum (Vincent et al., 2019).
Zoliflodacin and doxycycline concentration determination
To verify that the predicted zoliflodacin and doxycycline PK profiles were observed in HFIM, broth specimens (500 µL) were sampled at time points 1, 2, 3, 6.5, 12, 14, 24, 26, 48, 50, 72, 74, 96, 98, 120, 122, 144, 146, and 168 h. Concentrations of zoliflodacin were determined from 100-μL sample aliquots by liquid chromatography–tandem mass spectrometry (LC–MS/MS), as previously reported (Jacobsson et al., 2021; Jacobsson et al., 2022a; Jacobsson et al., 2022b). Concentrations of doxycycline were determined from 100-μL sample aliquots by LCMS/MS with isotopically labeled doxycycline as the internal standard.
Comparative genomic analysis
Whole-genome sequencing (WGS) was conducted on colonies that were growing on the zoliflodacin-containing plates and that additionally exhibited enhanced zoliflodacin MICs as determined by agar dilution. The aim was to identify gyrB mutations associated with the increased MICs of zoliflodacin (Alm et al., 2015; Foerster et al., 2015; Foerster et al., 2019; Jacobsson et al., 2021; Jacobsson et al., 2022a). The WGS procedure followed the previously reported methodology (Jacobsson et al., 2016; Golparian et al., 2020). Briefly, all reads underwent quality control and trimming procedures, following the established CLC Genomics Workbench v20.0.4 workflow (Golparian et al., 2022). Subsequently, the quality-controlled reads were aligned to the WHO X reference genome obtained from Genbank (Accession: NZ_LT592155.1) using local alignment in CLC Genomics Workbench. The alignment employed a match score of 1, a mismatch cost of 2, and a linear gap cost of 3. Variants across the gene were identified with a minimum coverage of ×10 and a minimum frequency of 35%.
Additionally, the genomic sequences of the mutants with increased zoliflodacin MICs were investigated using the same procedure to identify or exclude other resistance-associated mutations selected in HFIM.
Raw reads associated with this study are accessible through the European Nucleotide Archive (ENA) with the accession number PRJEB64051.
Results
Phenotypic and genomic characteristics of investigated N. gonorrhoeae strains
In all examined strains, the MICs of zoliflodacin were similar (only one MIC doubling dilution higher) using the microbroth dilution method in comparison to the agar dilution method. The MICs of zoliflodacin and additional tested antimicrobials, and relevant genomic characteristics of the investigated gonococcal strains WHO F (susceptible to relevant therapeutical antimicrobials), WHO X (extensively drug-resistant; zoliflodacin-susceptible), and SE600/18 (zoliflodacin-susceptible with GyrB S467N substitution) are summarized in Table 1.
Pharmacodynamic interaction of zoliflodacin and doxycycline in the hollow-fiber infection model
HFIM dose–range experiments were performed to simulate the combination therapy of a single oral dose of 0.5–4 g of zoliflodacin combined with a daily oral dose of 200 mg doxycycline administered as 100 mg twice a day for 7 days (Figure 1). The untreated growth controls all grew well for the three examined strains obtaining a bacterial density of 109–1010 CFUs/mL, except for a temporary decrease in growth at days 2–4 for WHO F, throughout the experiments lasting for 7 days (Figure 1A). The coadministration of zoliflodacin and doxycycline resulted in a rapid initial bacterial kill in all experiments. After a single 0.5 g zoliflodacin dose combined with the doxycycline regimen, WHO F was killed after 12 h in both conducted experiments, while WHO X and SE600/18 recovered and regrew to approximately 109–1010 CFUs/mL at the 48-h time point (Figure 1B). When simulating a 1 g zoliflodacin dose combined with the doxycycline regime, WHO F was eradicated after 6.5 h, and WHO X and SE600/18 were both eradicated in one experiment and regrew in the other experiment reaching a bacterial density of 108–1010 CFUs/mL after 120 h and 72 h, respectively (Figure 1C). Doses of 2–4 g zoliflodacin combined with the doxycycline regimen successfully eradicated all three strains within 12 h (Figure 1D).
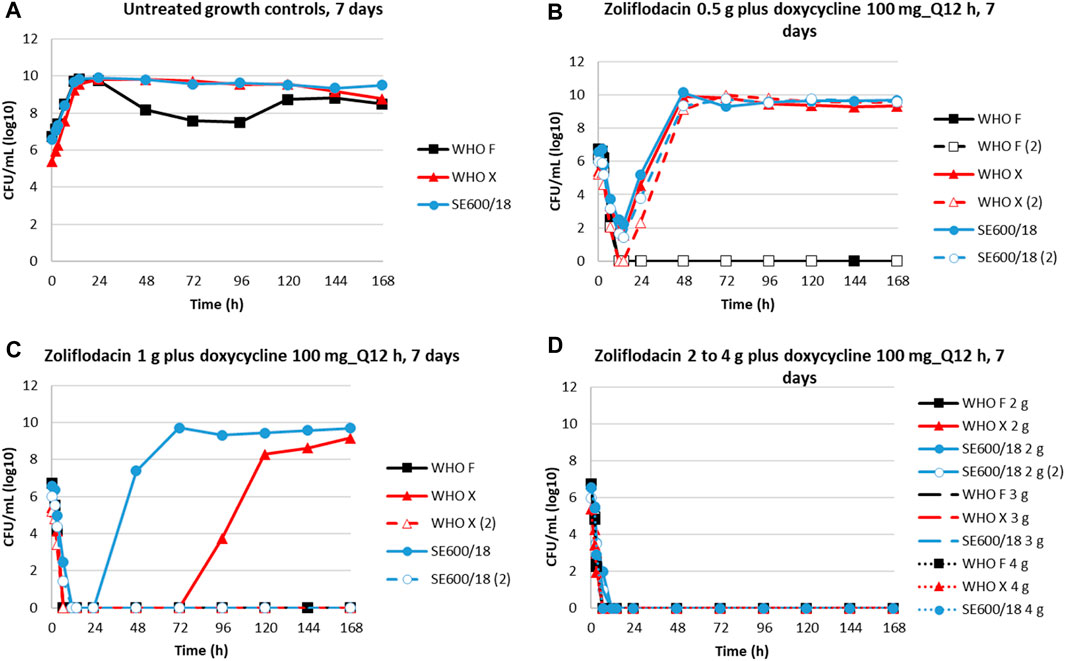
FIGURE 1. Growth curves of the total population of three N. gonorrhoeae strains (WHO F, WHO X, and SE600/18) in dose–range hollow-fiber infection Model (HFIM) experiments (performed in duplicate) showing untreated controls (A) and simulations of zoliflodacin single oral dose of 0.5 g (B), 1 g (C), 2 g, 3 g, and 4 g (D) combined with a daily dose of 200 mg doxycycline administered as 100 mg twice a day (Q12 h) for 7 days. HFIM experiments were monitored for 7 days.
On the zoliflodacin-containing agar plates, colonies were observed for WHO X and SE600/18 in the experimental arms of 0.5 g zoliflodacin combined with doxycycline and for SE600/18 also in the 1 g zoliflodacin combined with doxycycline arm. The WHO X colonies with a zoliflodacin MIC = 0.5 mg/L by agar dilution (parental strain MIC = 0.125 mg/L) harbored a GyrB T472P alteration, which has not been previously recognized to influence the MIC of zoliflodacin. The SE600/18 colonies displayed zoliflodacin MICs of 1–2 mg/L (parental strain MIC = 0.25 mg/L) and harbored the pre-existing GyrB S467N mutation plus a GyrB D429N alteration selected in the HFIM experiment, which has been previously shown to increase not only the zoliflodacin MIC but also to impair the growth and biofitness of the strain (Alm et al., 2015; Foerster et al., 2015; Foerster et al., 2019; Jacobsson et al., 2021; Jacobsson et al., 2022a).
When comparing N. gonorrhoeae eradication using dual therapy with the zoliflodacin–doxycycline regimen to our previous zoliflodacin monotherapy HFIM studies (Jacobsson et al., 2021; Jacobsson et al., 2022a) (Figure 2), we observed that 0.5 g zoliflodacin monotherapy failed to eradicate all three strains (Jacobsson et al., 2021; Jacobsson et al., 2022a), while WHO F was eradicated using the coadministration of zoliflodacin 0.5 g plus doxycycline in the present study. With 1 g zoliflodacin monotherapy, both WHO X and SE600/18 regrew and reached a bacterial density of 108 CFUs/mL 24 h previously compared to one of the two HFIM experiments with zoliflodacin 1 g combined with doxycycline. In the second HFIM experiment with zoliflodacin and 1 g doxycycline, both WHO X and SE600/18 were eradicated. Finally, SE600/18 also regrew in 2 g zoliflodacin monotherapy (Jacobsson et al., 2021; Jacobsson et al., 2022a), whereas it was eradicated by zoliflodacin 2 g plus doxycycline dual therapy. Accordingly, zoliflodacin–doxycycline dual therapy was slightly more effective in gonococcal killing compared to zoliflodacin monotherapy (Figure 2).
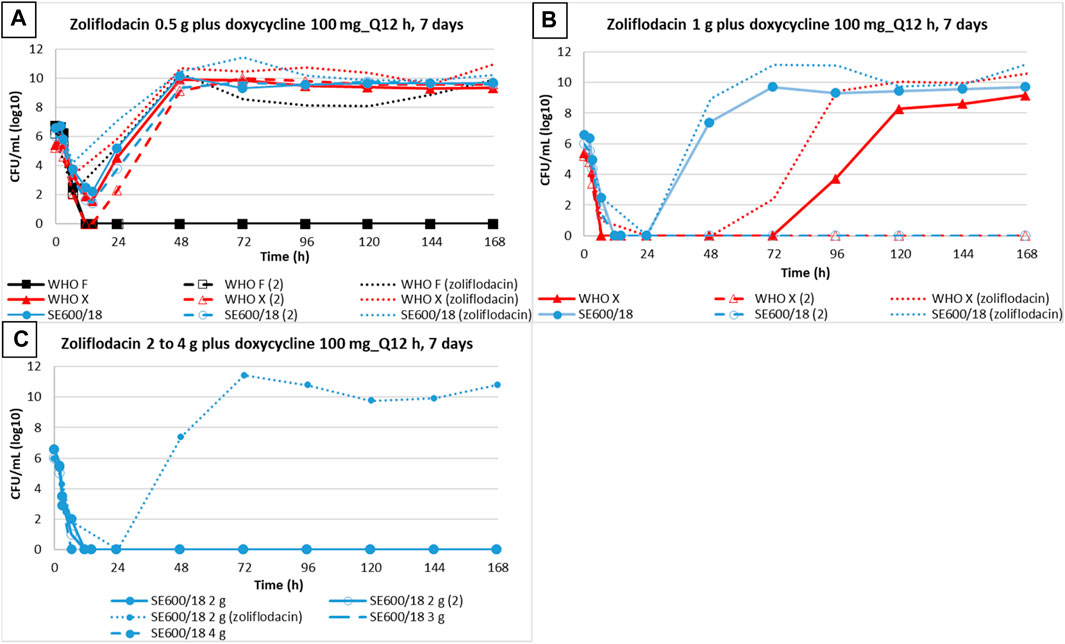
FIGURE 2. Growth curves of the total population of three N. gonorrhoeae strains (WHO F, WHO X, and SE600/18) simulating dual therapy with doxycycline (100 mg twice a day for 7 days) plus zoliflodacin 0.5 g (A), 1 g, (B) 2 g, 3 g, and 4 g (C) are represented with solid and dashed lines. Dotted lines depict results from zoliflodacin monotherapy (Jacobsson et al., 2021; Jacobsson et al., 2022a).
We also compared the emergence of N. gonorrhoeae populations with increased zoliflodacin MICs by dual therapy with the zoliflodacin–doxycycline regimen to our previous zoliflodacin monotherapy HFIM studies (Jacobsson et al., 2021; Jacobsson et al., 2022a) (Figure 3). In brief, exposure of WHO X to both 0.5 g and 1 g zoliflodacin monotherapy for WHO X (Jacobsson et al., 2021) selected zoliflodacin-resistant GyrB D429N-containing N. gonorrhoeae mutants that were maintained until the end of the 7-day experiment (Figure 3B). However, only the zoliflodacin 0.5 g dose selected mutants with increased zoliflodacin MICs (with GyrB T472P causing the zoliflodacin MIC to increase from 0.125 mg/L to 0.5 mg/L) when combined with the doxycycline regimen, and the mutants were not detected after 96 h (Figure 3A) This indicates that the mutants were outcompeted by WHO X wild-type cells due to a decreased biofitness. Similar suppression of the emergence of zoliflodacin-resistant mutants with adequate biofitness was observed for SE600/18 (data not shown).
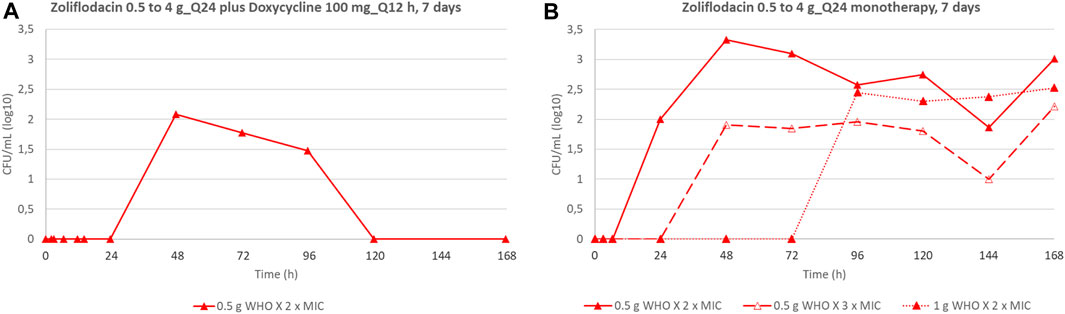
FIGURE 3. Total growth of populations with increased zoliflodacin MIC of the N. gonorrhoeae strain WHO X on the zoliflodacin-containing agar plates (2×MIC or 3×MIC) in the current experiments simulating dual-therapy with oral zoliflodacin and doxycycline (100 mg twice a day for 7 days) (A), compared to our previous studies evaluating zoliflodacin monotherapy (Jacobsson et al., 2021) (B).
Competition biofitness experiments (coculture) in HFIM
WHO X and the in vitro-selected WHO X-T472P mutant with increased zoliflodacin MICs were cocultured in the same HFIM cartridge for 7 days to investigate if the WHO X-T472P mutant selected in vitro displayed any altered growth and biofitness (Figure 4). The growth of both zoliflodacin-susceptible WHO X and WHO X-T472P with increased zoliflodacin MICs was maintained at approximately 108–1010 CFUs/mL during the experiment lasting for 7 days, i.e., similar to the bacterial density when monocultured (Figure 4A). However, the calculated competitive index (Figure 4B) displayed an impaired biofitness, especially during the first 72 h, of WHO X-T472P with increased zoliflodacin MIC compared to the zoliflodacin-susceptible WHO X parent strain. The HFIM experiments of the WHO F, WHO X, and SE600/18 strains and their results have been summarized in Supplementary Figure S2.
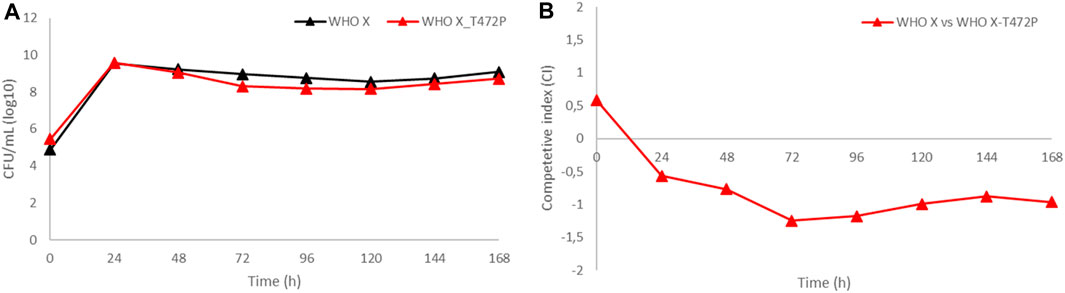
FIGURE 4. (A) Growth curves of the total population of the zoliflodacin-susceptible N. gonorrhoeae WHO X reference strain (black line) and the in vitro-selected WHO X-T472P mutant with increased zoliflodacin MIC (red line) when cocultured in the identical hollow-fiber infection model (HFIM) cartridge and monitored for 7 days. (B) Competitive index for the zoliflodacin-susceptible N. gonorrhoeae WHO X reference strain and the in vitro-selected WHO X-T472P mutant with increased zoliflodacin MIC, when cocultured in the identical HFIM cartridge and monitored for 7 days.
Discussion
Gonorrhea remains an important public health concern with 82 million new cases estimated among persons aged 15–49 years in 2020 globally (WHO, 2021). The high incidence and prevalence of infection combined with factors like its extraordinary capacity to develop resistance to all classes of antimicrobials introduced for treatment, the large number of asymptomatic infections, which constitutes a reservoir of infection, and the cultural stigma associated with the infection in some parts of the world contribute to the urgent need for improvement of the management and control of gonorrhea. Zoliflodacin, the first-in-class spiropyrimidinetrione, is bactericidal against N. gonorrhoeae with a low in vitro frequency of resistance and high antibacterial activity with an MIC90 value of 0.125 mg/L when global, including multi- and extensively drug-resistant, clinical N. gonorrhoeae isolates from the recent decades have been investigated (Jacobsson et al., 2014; Unemo et al., 2015; Bradford et al., 2020; Le et al., 2021; Unemo et al., 2021; Berçot et al., 2022; Pleininger et al., 2022). Results from the conducted phase II RCT using 3 g single oral dose of zoliflodacin demonstrated 100% microbiological cure for urogenital and rectal gonorrhea and 78% for pharyngeal gonorrhea, and no zoliflodacin-resistant gonococcal isolates were found (Taylor et al., 2018a). Furthermore, by using our dynamic HFIM for gonorrhea, we have previously demonstrated that to provide both effective killing of N. gonorrhoeae and suppression of the selection of zoliflodacin resistance, zoliflodacin should be given as a ≥3 g single dose. This dose also targets the rare zoliflodacin-susceptible N. gonorrhoeae strains harboring a pre-existing zoliflodacin-target GyrB S467N substitution which predisposes for the development of zoliflodacin resistance by a second-step mutation, i.e., GyrB D429N (Jacobsson et al., 2021; Jacobsson et al., 2022a; Golparian et al., 2022).
If zoliflodacin is approved for the treatment of gonorrhea, it is likely to be administered with doxycycline because other bacterial STIs, such as chlamydia, syphilis, or M. genitalium infections, are frequently diagnosed coinfections with gonorrhea (or may not have been excluded). Moreover, the use of doxycycline PEP (Molina et al., 2018; Luetkemeyer et al., 2022) will likely quickly expand. Consequently, the pharmacodynamic interaction between the two drugs was assessed in the present study. Zoliflodacin 0.5–4 g treatment in combination with the recommended 7-day doxycycline treatment regimen (100 mg twice a day for 7 days) was simulated in our dynamic in vitro HFIM. In brief, WHO F, susceptible to all relevant antimicrobials, was eradicated when simulating a single 0.5 g zoliflodacin dose combined with the doxycycline regimen. Both WHO X, extensively drug-resistant, including all extended-spectrum cephalosporins and fluoroquinolones, as well as SE600/18, a clinical zoliflodacin-susceptible N. gonorrhoeae strain with GyrB S467N substitution, were eradicated in one experiment simulating a single 1 g zoliflodacin dose combined with the doxycycline regimen, and recovered and regrew in one experiment. In the experiment where WHO X regrew, the novel GyrB T472P substitution, resulting in an increase in the zoliflodacin MIC from 0.125 mg/L to 0.5 mg/L, was identified. Notably, the WHO X-T472P mutant displayed a slightly decreased biofitness, which was observed by its eradication after 92 h in the HFIM experiment and in competitive coculture experiments. In the resistant populations of SE600/18, we identified the previously described GyrB D429N substitution that has been shown to increase the MICs of zoliflodacin (Alm et al., 2015; Foerster et al., 2015; Foerster et al., 2019; Jacobsson et al., 2021; Jacobsson et al., 2022a). However, compared to zoliflodacin monotherapy (Jacobsson et al., 2021; Jacobsson et al., 2022a), zoliflodacin plus doxycycline dual therapy further suppressed the emergence of zoliflodacin resistance, which only emerged when suboptimal doses of <3 g zoliflodacin were used. Consequently, adding doxycycline to the zoliflodacin treatment seemed advantageous for both the bacterial kill rate and resistance suppression compared to our previous studies, mimicking zoliflodacin monotherapy. With dual therapy, all three examined strains were eradicated by a lower zoliflodacin dose (WHO F 0.5 g compared to 1 g and SE600/18 2 g compared to a 3 g zoliflodacin dose) or the complete regrowth was observed later during the experiment (120 h compared to 96 h for WHO X using 1 g zoliflodacin dose). These results are in contrast to the results from a previous static in vitro study (Foerster et al., 2019), in which it was indicated that doxycycline/tetracycline inhibits the gonococcal killing of zoliflodacin in 6-h time-kill curve analysis. Accordingly, in the present study, we show the importance of using dynamic HFIM that more accurately and comprehensively simulate gonococcal infections and their treatment, i.e., compared to static in vitro models, such as short-time checkerboard experiments or time-kill curve analysis, which should be interpreted with caution.
Dual antimicrobial therapy can be a way to preserve an antibiotic and to prolong its usefulness; however, to evaluate if the combination of antibiotics is beneficial, if there is a synergistic effect or, at a minimum, an additive interaction so that the bacterial killing is enhanced and the likelihood of suppression of resistance is increased, various properties of the drug need to be considered. HFIM allows to alter the antimicrobial concentration, simulate various dosing regimens and different distribution schemes, single or multiple doses, and also simulate and combine antibiotics with differences in half-life, and all at the same time monitoring the efficacy in bacterial killing and the development of resistant subpopulations during treatment under the different administration schemes all to evaluate the best overall treatment effect (Nielsen and Friberg, 2013; Lodise et al., 2020; Hou et el., 2022). There are limitations linked to HFIM to be considered when interpreting these findings. For example, the efficacy of a specific drug represents a conservative estimate as the model does not account for the immune response of the host and therefore does not fully reflect the human response to bacteria and antibiotics. Furthermore, a limitation coupled to the present study is the nonexistence of human PK data from the major gonococcal infection sites, i.e., the urogenital tract, the anogenital tract, and the oropharynx. However, this is a limitation in most similar STI studies, i.e., for the evaluation of novel antibiotics and for antimicrobials currently recommended for treatment (Kong et al., 2019). Consequently, the HFIM simulations for gonorrhea treatment were in the present study based on concentrations of free zoliflodacin and doxycycline in human plasma that may not correspond to the systemic exposures at the urogenital and extragenital infection sites in an ideal manner. Despite this, antimicrobial plasma concentrations are often used as surrogates for infection site concentrations as these are unknown and have shown to serve well in linking drug exposure to effect (Drusano, 2004).
In conclusion, by examining the pharmacodynamics of zoliflodacin in combination therapy with doxycycline against N. gonorrhoeae in our dynamic in vitro gonococcal HFIM, we demonstrated that combination therapy including zoliflodacin (0.5 g–4 g single dose) and doxycycline (200 mg divided into 100 mg twice a day for 7 days) displays a high efficacy in the N. gonorrhoeae killing rate and suppression of resistance emergence, i.e., an increased efficacy compared to zoliflodacin monotherapy (Jacobsson et al., 2021; Jacobsson et al., 2022a). The future use of zoliflodacin for the treatment of gonorrhea will require additional evaluations, including the investigation of antagonism or synergism with other antibiotics potentially co-administered for the treatment of gonorrhea, additional concomitant STIs, or other infectious diseases.
Data availability statement
The datasets presented in this study can be found in online repositories. The names of the repository/repositories and accession number(s) can be found here: European Nucleotide Archive (ENA) with the accession number PRJEB64051.
Author contributions
SJ: writing–original draft and writing–review and editing. DG: writing–review and editing. JO: writing–review and editing. FK: writing–review and editing. RD: writing–review and editing. FF: writing–review and editing. DB: writing–review and editing. AL: writing–review and editing. GD: writing–review and editing. MU: writing–original draft and Writing–review and editing.
Funding
The author(s) declare financial support was received for the research, authorship, and/or publication of this article. This study was made possible with funding received by the Global Antibiotic Research and Development Partnership (GARDP) from the German Federal Ministry of Education and Research and the UK Department of Health and Social Care (DHSC) as part of the Global AMR Innovation Fund (GAMRIF) (2022). GAMRIF is a United Kingdom aid program that supports early-stage innovative research in underfunded areas of AMR research and development for the benefit of those in low- and middle-income countries (LMICs), who bear the greatest burden of AMR. Grants from the Örebro County Council Research Committee, Örebro, Sweden, and the Foundation for Medical Research at Örebro University Hospital, Örebro, Sweden (2022), were also used.
Acknowledgments
The authors would like to thank Entasis Therapeutics Inc. (Waltham, MA, United States), an affiliate of Innoviva Specialty Therapeutics, Inc., and particularly John Mueller and John O’Donnell, for provision of zoliflodacin, PK/PD data, and review of the manuscript.
Conflict of interest
The authors declare that the research was conducted in the absence of any commercial or financial relationships that could be construed as a potential conflict of interest.
Publisher’s note
All claims expressed in this article are solely those of the authors and do not necessarily represent those of their affiliated organizations, or those of the publisher, the editors, and the reviewers. Any product that may be evaluated in this article, or claim that may be made by its manufacturer, is not guaranteed or endorsed by the publisher.
Supplementary material
The Supplementary Material for this article can be found online at: https://www.frontiersin.org/articles/10.3389/fphar.2023.1291885/full#supplementary-material
Supplementary Figure S1 | Hollow fiber infection model (two compartment model). Neisseria gonorrhoeae cells replicate and are retained in the hollow fiber cellulosic cartridge. The central reservoir is continuously re-circulating the growth medium, and eliminates waste of the growth medium. Antibiotic is added in a controlled manner, associated with the examined dosing regimen, and the elimination kinetics are controlled by the addition of diluent to the central reservoir. The volume in the central reservoir is maintained constant.
Supplementary Figure S2 | Schematic flow chart summarizing the hollow fiber infection model (HFIM) experiments of the WHO F, WHO X, and SE600/18 strains and their results. MIC, minimum inhibitory concentration.
References
Algammal, A., Hetta, H. F., Mabrok, M., and Behzadi, P. (2023). Editorial: emerging multidrug-resistant bacterial pathogens "superbugs": a rising public health threat. Front. Microbiol. 14, 1135614. doi:10.3389/fmicb.2023.1135614
Alm, R. A., Lahiri, S. D., Kutschke, A., Otterson, L. G., McLaughlin, R. E., Whiteaker, J. D., et al. (2015). Characterization of the novel DNA gyrase inhibitor AZD0914: low resistance potential and lack of cross-resistance in Neisseria gonorrhoeae. Antimicrob. Agents Chemother. 59, 1478–1486. doi:10.1128/AAC.04456-14
Alsarra, I. A., Al-Said, M. S., Al-Khamis, K. I., Niazy, E. M., El-Sayed, Y. M., Al-Rashood, K. A., et al. (2004). Comparative bioavailability study of doxycycline hyclate (equivalent to 100 mg doxycycline) capsules (doxycin vs vibramycin) for bioequivalence evaluation in healthy adult volunteers. Int. J. Clin. Pharmacol. Ther. 42, 373–377. doi:10.5414/cpp42373
Basarab, G. S., Kern, G. H., McNulty, J., Mueller, J. P., Lawrence, K., Vishwanathan, K., et al. (2015). Responding to the challenge of untreatable gonorrhea: ETX0914, a first-in-class agent with a distinct mechanism-of-action against bacterial Type II topoisomerases. Sci. Rep. 5, 11827. doi:10.1038/srep11827
Berçot, B., Caméléna, F., Mérimèche, M., Jacobsson, S., Sbaa, G., Mainardis, M., et al. (2022). Ceftriaxone-resistant, multidrug-resistant Neisseria gonorrhoeae with a novel mosaic penA-237.001 gene. Euro Surveill. 27, 2200899. doi:10.2807/1560-7917.ES.2022.27.50.2200899
Binh, V. Q., Chinh, N. T., Thanh, N. X., Cuong, B. T., Quang, N. N., Dai, B., et al. (2009). Sex affects the steady-state pharmacokinetics of primaquine but not doxycycline in healthy subjects. Am. J. Trop. Med. Hyg. 81, 747–753. doi:10.4269/ajtmh.2009.09-0214
Bissessor, M., Tabrizi, S. N., Fairley, C. K., Danielewski, J., Whitton, B., Bird, S., et al. (2011). Differing Neisseria gonorrhoeae bacterial loads in the pharynx and rectum in men who have sex with men: implications for gonococcal detection, transmission, and control. J. Clin. Microbiol. 49, 4304–4306. doi:10.1128/JCM.05341-11
Bradford, P. A., Miller, A. A., O’Donnell, J., and Mueller, J. P. (2020). Zoliflodacin: an oral spiropyrimidinetrione antibiotic for the treatment of Neisseria gonorrheae, including multi-drug-resistant isolates. ACS Infect. Dis. 6, 1332–1345. doi:10.1021/acsinfecdis.0c00021
Cadwell, J. J. S. (2012). The hollow fiber infection model for antimicrobial pharmacodynamics and pharmacokinetics. Adv. Pharmacoepidem. Drug Saf. S1, 007. doi:10.4172/2167-1052.S1-007
Centers for Disease Control and Prevention (2012). Cephalosporin-resistant Neisseria gonorrhoeae public health response plan. Available at: http://www.cdc.gov/std/treatment/ceph-r-responseplanjuly30-2012.pdf.
Chow, E. P., Tabrizi, S. N., Phillips, S., Lee, D., Bradshaw, C. S., Chen, M. Y., et al. (2016). Neisseria gonorrhoeae bacterial DNA load in the pharynges and saliva of men who have sex with men. J. Clin. Microbiol. 54, 2485–2490. doi:10.1128/JCM.01186-16
Day, M., Pitt, R., Mody, N., Saunders, J., Rai, R., Nori, A., et al. (2022b). Detection of 10 cases of ceftriaxone-resistant Neisseria gonorrhoeae in the United Kingdom, december 2021 to june 2022. Euro Surveill. 27, 2200803. doi:10.2807/1560-7917.ES.2022.27.46.2200803
Day, M. J., Jacobsson, S., Spiteri, G., Kulishev, C., Sajedi, N., Woodford, N., et al. (2022a). Significant increase in azithromycin "resistance" and susceptibility to ceftriaxone and cefixime in Neisseria gonorrhoeae isolates in 26 European countries, 2019. BMC Infect. Dis. 22, 524. doi:10.1186/s12879-022-07509-w
Drusano, G. L. (2004). Antimicrobial pharmacodynamics: critical interactions of “bug and drug”. Nat. Rev. Microbiol. 2, 289–300. doi:10.1038/nrmicro862
European Centre for Disease Prevention and Control (2019). Response plan to control and manage the threat of multi- and extensively drug-resistant gonorrhoea in europe. 2019 update. Available at: https://www.ecdc.europa.eu/sites/default/files/documents/multi-and-extensively-drug-resistant-gonorrhoea-response-plan-Europe-2019.pdf.
Fifer, H., Natarajan, U., Jones, L., Alexander, S., Hughes, G., Golparian, D., et al. (2016). Failure of dual antimicrobial therapy in treatment of gonorrhea. N. Engl. J. Med. 374, 2504–2506. doi:10.1056/NEJMc1512757
Foerster, S., Drusano, G., Golparian, D., Neely, M., Piddock, L. J. V., Alirol, E., et al. (2019). In vitro antimicrobial combination testing of and evolution of resistance to the first-in-class spiropyrimidinetrione zoliflodacin combined with six therapeutically relevant antimicrobials for Neisseria gonorrhoeae. J. Antimicrob. Chemother. 74, 3521–3529. doi:10.1093/jac/dkz376
Foerster, S., Golparian, D., Jacobsson, S., Hathaway, L. J., Low, N., Shafer, W. M., et al. (2015). Genetic resistance determinants, in vitro time-kill curve analysis and pharmacodynamic functions for the novel topoisomerase II inhibitor ETX0914 (AZD0914) in Neisseria gonorrhoeae. Front. Microbiol. 6, 1377. doi:10.3389/fmicb.2015.01377
Golparian, D., Bazzo, M. L., Golfetto, L., Gaspar, P. C., Schorner, M. A., Schwartz Benzaken, A., et al. (2020). Genomic epidemiology of Neisseria gonorrhoeae elucidating the gonococcal antimicrobial resistance and lineages/sublineages across Brazil, 2015-16. J. Antimicrob. Chemother. 75, 3163–3172. doi:10.1093/jac/dkaa318
Golparian, D., Pleininger, S., Jacobsson, S., Indra, A., and Unemo, M. (2022). Complete reference genome sequence of the extensively drug-resistant strain Neisseria gonorrhoeae AT159, with ceftriaxone resistance and high-level azithromycin resistance, using Nanopore Q20+ chemistry and Illumina sequencing. Microbiol. Resour. Announc 11, e0074422. doi:10.1128/mra.00744-22
Golparian, D., Rose, L., Lynam, A., Mohamed, A., Bercot, B., Ohnishi, M., et al. (2018). Multidrug-resistant Neisseria gonorrhoeae isolate, belonging to the internationally spreading Japanese FC428 clone, with ceftriaxone resistance and intermediate resistance to azithromycin, Ireland. Euro Surveill. 23, 1800617. doi:10.2807/1560-7917.ES.2018.23.47.1800617
Gschwend, M. H., Martin, W., Erenmemişoğlu, A., Scherm, M., Dilger, C., Tamur, U., et al. (2007). Pharmacokinetics and bioequivalence study of doxycycline capsules in healthy male subjects. Arzneimittelforschung 57, 347–351. doi:10.1055/s-0031-1296629
Hou, Y., Mi, K., Sun, L., Zhou, K., Wang, L., Zhang, L., et al. (2022). The application of hollow fiber cartridge in biomedicine. Pharmaceutics 14, 1485. doi:10.3390/pharmaceutics14071485
Jacobsson, S., Golparian, D., Alm, R. A., Huband, M., Mueller, J., Jensen, J. S., et al. (2014). High in vitro activity of the novel spiropyrimidinetrione AZD0914, a DNA gyrase inhibitor, against multidrug-resistant Neisseria gonorrhoeae isolates suggests a new effective option for oral treatment of gonorrhea. Antimicrob. Agents Chemother. 58, 5585–5588. doi:10.1128/AAC.03090-14
Jacobsson, S., Golparian, D., Cole, M., Spiteri, G., Martin, I., Bergheim, T., et al. (2016). WGS analysis and molecular resistance mechanisms of azithromycin-resistant (MIC >2 mg/L) Neisseria gonorrhoeae isolates in Europe from 2009 to 2014. J. Antimicrob. Chemother. 71, 3109–3116. doi:10.1093/jac/dkw279
Jacobsson, S., Golparian, D., Oxelbark, J., Alirol, E., Franceschi, F., Gustafsson, T. N., et al. (2021). Pharmacodynamic evaluation of dosing, bacterial kill, and resistance suppression for zoliflodacin against Neisseria gonorrhoeae in a dynamic hollow fiber infection model. Front. Pharmacol. 12, 682135. doi:10.3389/fphar.2021.682135
Jacobsson, S., Golparian, D., Oxelbark, J., Franceschi, F., Brown, D., Louie, A., et al. (2022a). Pharmacodynamic evaluation of zoliflodacin treatment of Neisseria gonorrhoeae strains with amino acid substitutions in the zoliflodacin target GyrB using a dynamic hollow fiber infection model. Front. Pharmacol. 13, 874176. doi:10.3389/fphar.2022.874176
Jacobsson, S., Golparian, D., Oxelbark, J., Wicha, W. W., da Costa, R. M. A., Franceschi, F., et al. (2022b). Pharmacodynamic evaluation of lefamulin in the treatment of gonorrhea using a hollow fiber infection model simulating Neisseria gonorrhoeae infections. Front. Pharmacol. 13, 1035841. doi:10.3389/fphar.2022.1035841
Janier, M., Unemo, M., Dupin, N., Tiplica, G. S., Potočnik, M., and Patel, R. (2021). 2020 European guideline on the management of syphilis. J. Eur. Acad. Dermatol Venereol. 35, 574–588. doi:10.1111/jdv.16946
Jennison, A. V., Whiley, D., Lahra, M. M., Graham, R. M., Cole, M. J., Hughes, G., et al. (2019). Genetic relatedness of ceftriaxone-resistant and high-level azithromycin resistant Neisseria gonorrhoeae cases, United Kingdom and Australia. Euro Surveill. 24, 1900118. doi:10.2807/1560-7917.ES.2019.24.8.1900118
Jensen, J. S., Cusini, M., Gomberg, M., Moi, H., Wilson, J., and Unemo, M. (2022). 2021 European guideline on the management of Mycoplasma genitalium infections. J. Eur. Acad. Dermatol Venereol. 36, 641–650. doi:10.1111/jdv.17972
Kern, G., Palmer, T., Ehmann, D. E., Shapiro, A. B., Andrews, B., Basarab, G. S., et al. (2015). Inhibition of Neisseria gonorrhoeae type II topoisomerases by the novel spiropyrimidinetrione AZD0914. J. Biol. Chem. 290, 20984–20994. doi:10.1074/jbc.M115.663534
Kong, F. Y. S., Horner, P., Unemo, M., and Hocking, J. S. (2019). Pharmacokinetic considerations regarding the treatment of bacterial sexually transmitted infections with azithromycin: a review. J. Antimicrob. Chemother. 74, 1157–1166. doi:10.1093/jac/dky548
Lahra, M. M., Martin, I., Demczuk, W., Jennison, A. V., Lee, K.-I., Nakayama, S.-I., et al. (2018). Cooperative recognition of internationally disseminated ceftriaxone-resistant Neisseria gonorrhoeae strain. Emerg. Infect. Dis. 24, 735–740. doi:10.3201/eid2404.171873
Lanjouw, E., Ouburg, S., de Vries, H. J., Stary, A., Radcliffe, K., and Unemo, M. (2016). 2015 European guideline on the management of Chlamydia trachomatis infections. Int. J. STD AIDS 27, 333–348. doi:10.1177/0956462415618837
Le, W., Su, X., Lou, X., Li, X., Gong, X., Wang, B., et al. (2021). Susceptibility trends of zoliflodacin against multidrug-resistant Neisseria gonorrhoeae clinical isolates in nanjing, China, 2014 to 2018. Antimicrob. Agents Chemother. 65, 00863320–e920. doi:10.1128/AAC.00863-20
Lin, X., Chen, W., Xie, Q., Yu, Y., Liao, Y., Feng, Z., et al. (2022). Dissemination and genome analysis of high-level ceftriaxone-resistant penA60.001 Neisseria gonorrhoeae strains from the Guangdong Gonococcal antibiotics susceptibility Programme (GD-GASP), 2016-2019. Emerg. Microbes Infect. 11, 344–350. doi:10.1080/22221751.2021.2011618
Lodise, T. P., Smith, N. M., O'Donnell, N., Eakin, A. E., Holden, P. N., Boissonneault, K. R., et al. (2020). Determining the optimal dosing of a novel combination regimen of ceftazidime/avibactam with aztreonam against NDM-1-producing Enterobacteriaceae using a hollow-fibre infection model. J. Antimicrob. Chemother. 75, 2622–2632. doi:10.1093/jac/dkaa197
Luetkemeyer, A., Dombrowski, J., Cohen, S., Donnell, D., Grabow, C., Brown, C., et al. (2022). “Doxycycline post-exposure prophylaxis for STI prevention among MSM and transgender women on HIV PrEP or living with HIV: high efficacy to reduce incident STI's in a randomized trial,” in The 24th International AIDS Conference, Montreal, Canada, July, 2022.
Maesen, F. P., Davies, B. I., and van den Bergh, J. J. (1989). Doxycycline and minocycline in the treatment of respiratory infections: a double-blind comparative clinical, microbiological and pharmacokinetic study. J. Antimicrob. Chemother. 23, 123–129. doi:10.1093/jac/23.1.123
Molina, J. M., Charreau, I., Chidiac, C., Pialoux, G., Cua, E., Delaugerre, C., et al. (2018). Post-exposure prophylaxis with doxycycline to prevent sexually transmitted infections in men who have sex with men: an open-label randomised substudy of the ANRS IPERGAY trial. Lancet Infect. Dis. 18, 308–317. doi:10.1016/S1473-3099(17)30725-9
Nakayama, S., Shimuta, K., Furubayashi, K., Kawahata, T., Unemo, M., and Ohnishi, M. (2016). New ceftriaxone- and multidrug-resistant Neisseria gonorrhoeae strain with a novel mosaic penA gene isolated in Japan. Antimicrob. Agents Chemother. 60, 4339–4341. doi:10.1128/AAC.00504-16
Nguyen, V. X., Nix, D. E., Gillikin, S., and Schentag, J. J. (1989). Effect of oral antacid administration on the pharmacokinetics of intravenous doxycycline. Antimicrob. Agents Chemother. 33, 434–436. doi:10.1128/AAC.33.4.434
Nielsen, E. I., and Friberg, L. E. (2013). Pharmacokinetic-pharmacodynamic modeling of antibacterial drugs. Pharmacol. Rev. 65, 1053–1090. doi:10.1124/pr.111.005769
O'Donnell, J., Lawrence, K., Vishwanathan, K., Hosagrahara, V., and Mueller, J. P. (2019). Single-dose pharmacokinetics, excretion, and metabolism of zoliflodacin, a novel spiropyrimidinetrione antibiotic, in healthy volunteers. healthy volunt. Antimicrob. Agents Chemother. 63, e01808–e01818. doi:10.1128/AAC.01808-18
Ohnishi, M., Watanabe, Y., Ono, E., Takahashi, C., Oya, H., Kuroki, T., et al. (2010). Spread of a chromosomal cefixime-resistant penA gene among different Neisseria gonorrhoeae lineages. Antimicrob. Agents Chemother. 54, 1060–1067. doi:10.1128/AAC.01010-09
Pleininger, S., Indra, A., Golparian, D., Heger, F., Schindler, S., Jacobsson, S., et al. (2022). Extensively drug-resistant (XDR) Neisseria gonorrhoeae causing possible gonorrhoea treatment failure with ceftriaxone plus azithromycin in Austria, April 2022. Euro Surveill. 27, 2200455. doi:10.2807/1560-7917.ES.2022.27.24.2200455
Poncin, T., Merimeche, M., Braille, A., Mainardis, M., Bebear, C., Jacquier, H., et al. (2019). Two cases of multidrug-resistant Neisseria gonorrhoeae related to travel in south-eastern Asia, France. Euro Surveill. 24, 1900528. doi:10.2807/1560-7917.ES.2019.24.36.1900528
Priest, D., Ong, J. J., Chow, E. P. F., Tabrizi, S., Phillips, S., Bissessor, M., et al. (2017). Neisseria gonorrhoeae DNA bacterial load in men with symptomatic and asymptomatic gonococcal urethritis. Sex. Transm. Infect. 93, 478–481. doi:10.1136/sextrans-2016-052950
San Francisco Department of Public Health, (2022). Health update: doxycycline post-exposure prophylaxis reduces incidence of sexually transmitted infections. https://www.sfcdcp.org/wp-content/uploads/2022/10/Health-Update-Doxycycline-Post-Exposure-Prophylaxis-Reduces-Incidence-of-Sexually-Transmitted-Infections-SFDPH-FINAL-10.20.2022.pdf.
Sánchez-Busó, L., Cole, M. J., Spiteri, G., Day, M., Jacobsson, S., Golparian, D., et al. (2022). Europe-wide expansion and eradication of multidrug-resistant Neisseria gonorrhoeae lineages: a genomic surveillance study. Lancet Microbe 3, e452–e463. doi:10.1016/S2666-5247(22)00044-1
Seña, A. C., Bachmann, L., Johnston, C., Wi, T., Workowski, K., Hook, E. W., et al. (2020). Optimising treatments for sexually transmitted infections: surveillance, pharmacokinetics and pharmacodynamics, therapeutic strategies, and molecular resistance prediction. Lancet Infect. Dis. 20, e181–e191. doi:10.1016/S1473-3099(20)30171-7
Suzuka, I., Kaji, H., and Kaji, A. (1966). Binding of specific sRNA to 30S ribosomal subunits: effect of 50S ribosomal subunits. Proc. Natl. Acad. Sci. U. S. A. 55, 1483–1490. doi:10.1073/pnas.55.6.1483
Taylor, S. N., Marrazzo, J., Batteiger, B. E., Hook, E. W., Sena, A. C., Long, J., et al. (2018a). Single dose zoliflodacin (ETX0914) for treatment of urogenital gonorrhea. N. Engl. J. Med. 379, 1835–1845. doi:10.1056/NEJMoa1706988
Taylor, S. N., Morris, D. H., Avery, A. K., Workowski, K. A., Batteiger, B. E., Tiffany, C. A., et al. (2018b). Gepotidacin for the treatment of uncomplicated urogenital gonorrhea: a phase 2, randomized, dose-ranging, single-oral dose evaluation. Clin. Infect. Dis. 67, 504–512. doi:10.1093/cid/ciy145
Unemo, M., Ahlstrand, J., Sánchez-Busó, L., Day, M., Aanensen, D., Golparian, D., et al. (2021). High susceptibility to zoliflodacin and conserved target (GyrB) for zoliflodacin among 1209 consecutive clinical Neisseria gonorrhoeae isolates from 25 European countries, 2018. J. Antimicrob. Chemother. 76, 1221–1228. doi:10.1093/jac/dkab024
Unemo, M., Golparian, D., Sanchez-Buso, L., Grad, Y., Jacobsson, S., Ohnishi, M., et al. (2016). The novel 2016 WHO Neisseria gonorrhoeae reference strains for global quality assurance of laboratory investigations: phenotypic, genetic and reference genome characterization. J. Antimicrob. Chemother. 71, 3096–3108. doi:10.1093/jac/dkw288
Unemo, M., Lahra, M. M., Cole, M., Galarza, P., Ndowa, F., Martin, I., et al. (2019). World Health Organization Global Gonococcal Antimicrobial Surveillance Program (WHO GASP): review of new data and evidence to inform international collaborative actions and research efforts. Sex. Health. 16, 412–425. doi:10.1071/SH19023
Unemo, M., Ringlander, J., Wiggins, C., Fredlund, H., Jacobsson, S., and Cole, M. (2015). High in vitro susceptibility to the novel spiropyrimidinetrione ETX0914 (AZD0914) among 873 contemporary clinical Neisseria gonorrhoeae isolates from 21 European countries from 2012 to 2014. Antimicrob. Agents Chemother. 59, 5220–5225. doi:10.1128/AAC.00786-15
Unemo, M., Ross, J., Serwin, A. B., Gomberg, M., Cusini, M., and Jensen, J. S. (2020). 2020 European guideline for the diagnosis and treatment of gonorrhoea in adults. Int. J. STD AIDS 2020, 095646242094912. doi:10.1177/0956462420949126
Unemo, M., and Shafer, W. M. (2014). Antimicrobial resistance in Neisseria gonorrhoeae in the 21st century: past, evolution, and future. Clin. Microbiol. Rev. 27, 587–613. doi:10.1128/CMR.00010-14
van der Veer, B., Hoebe, C., Dukers-Muijrers, N., van Alphen, L. B., and Wolffs, P. F. G. (2020). Men and women have similar Neisseria gonorrhoeae bacterial loads: a comparison of three anatomical sites. J. Clin. Microbiol. 58, 01171120–e1220. doi:10.1128/JCM.01171-20
Vincent, L. R., Kerr, S. R., Tan, Y., Tomberg, J., Raterman, E. L., Dunning Hotopp, J. C., et al. (2018). In vivo-Selected compensatory mutations restore the fitness cost of mosaic penA alleles that confer ceftriaxone resistance in Neisseria gonorrhoeae. mBio 9, e01905-17. doi:10.1128/mBio.01905-17
Whiley, D. M., Jennison, A., Pearson, J., and Lahra, M. M. (2018). Genetic characterisation of Neisseria gonorrhoeae resistant to both ceftriaxone and azithromycin. Lancet Infect. Dis. 18, 717–718. doi:10.1016/S1473-3099(18)30340-2
Workowski, K. A., Bachmann, L. H., Chan, P. A., Johnston, C. M., Muzny, C. A., Park, I., et al. (2021). Sexually transmitted infections treatment guidelines, 2021. MMWR Recomm. Rep. 70, 1–187. doi:10.15585/mmwr.rr7004a1
World Health Organization, (2012). Global action plan to control the spread and Impact of antimicrobial resistance in Neisseria gonorrhoeae. Available at: https://apps.who.int/iris/handle/10665/44863.
World Health Organization, (2021a). Global progress report on HIV, viral hepatitis and sexually transmitted infections. Available at: https://www.who.int/publications/i/item/9789240027077.
World Health Organization, (2021b). Global progress report on HIV, viral hepatitis and sexually transmitted infections. Available at: https://www.who.int/
Keywords: Neisseria gonorrhoeae, hollow-fiber infection model, zoliflodacin, doxycycline, pharmacodynamics, antimicrobial treatment, pharmacokinetics
Citation: Jacobsson S, Golparian D, Oxelbark J, Kong FYS, Da Costa RMA, Franceschi F, Brown D, Louie A, Drusano G and Unemo M (2023) Pharmacodynamics of zoliflodacin plus doxycycline combination therapy against Neisseria gonorrhoeae in a gonococcal hollow-fiber infection model. Front. Pharmacol. 14:1291885. doi: 10.3389/fphar.2023.1291885
Received: 10 September 2023; Accepted: 20 November 2023;
Published: 07 December 2023.
Edited by:
Charlene Kahler, University of Western Australia, AustraliaReviewed by:
Payam Behzadi, Islamic Azad University, IranAdriana Le Van, Uniformed Services University of the Health Sciences, United States
Copyright © 2023 Jacobsson, Golparian, Oxelbark, Kong, Da Costa, Franceschi, Brown, Louie, Drusano and Unemo. This is an open-access article distributed under the terms of the Creative Commons Attribution License (CC BY). The use, distribution or reproduction in other forums is permitted, provided the original author(s) and the copyright owner(s) are credited and that the original publication in this journal is cited, in accordance with accepted academic practice. No use, distribution or reproduction is permitted which does not comply with these terms.
*Correspondence: Magnus Unemo, magnus.unemo@regionorebrolan.se